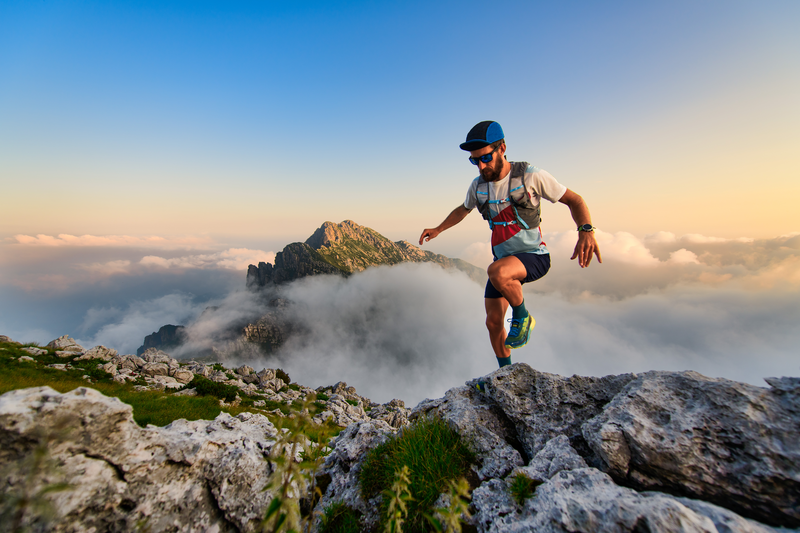
95% of researchers rate our articles as excellent or good
Learn more about the work of our research integrity team to safeguard the quality of each article we publish.
Find out more
MINI REVIEW article
Front. Cell. Infect. Microbiol. , 14 May 2019
Sec. Parasite and Host
Volume 9 - 2019 | https://doi.org/10.3389/fcimb.2019.00132
This article is part of the Research Topic Toxoplasma gondii Host Interactions: A Story of Immune Attack and Parasite Counterattack View all 19 articles
As an intracellular microbe, Toxoplasma gondii must establish a highly intimate relationship with its host to ensure success as a parasite. Many studies over the last decade-and-a-half have highlighted how the host reshapes its immunoproteome to survive infection, and conversely how the parasite regulates host responses to ensure persistence. The role of host non-protein-coding RNA during infection is a vast and largely unexplored area of emerging interest. The potential importance of this facet of the host-parasite interaction is underscored by current estimates that as much as 80% of the host genome is transcribed into non-translated RNA. Here, we review the current state of knowledge with respect to two major classes of non-coding RNA, microRNA (miRNA) and long non-coding RNA (lncRNA), in the host response to T. gondii infection. These two classes of regulatory RNA are known to have profound and widespread effects on cell function. However, their impact on infection and immunity is not well-understood, particularly for the response to T. gondii. Nevertheless, numerous miRNAs have been identified that are upregulated by Toxoplasma, and emerging evidence suggests a functional role during infection. While the field of lncRNA is in its infancy, it is already clear that Toxoplasma is also a strong trigger for this class of regulatory RNA. Non-coding RNA responses induced by T. gondii are likely to be major determinants of the host's ability to resist infection and the parasite's ability to establish long-term latency.
Toxoplasma gondii is one of the most prevalent human parasites in the world. It infects a wide range of species and establishes latent infection in brain and muscle tissue. In immune compromised individuals, as well as in the developing fetus, infection can result in severe disease (McLeod et al., 2013). Toxoplasma initiates strong protective Th1 immunity through induction of dendritic cell IL-12, while also inducing the activity of counter-regulatory cytokines such as IL-10 (Dupont et al., 2012; Sasai et al., 2018). In mouse models, parasite profilin functions as a pathogen-associated molecular pattern molecule triggering IL-12 through host Toll-like receptors 11 and 12 (Andrade et al., 2013; Raetz et al., 2013; Gazzinelli et al., 2014; Yarovinsky, 2014). From within the cell, Toxoplasma directly injects host-directed effector proteins such as ROP16, TgIST, GRA18, and GRA24 (Olias et al., 2016; Hakimi et al., 2017; He et al., 2018). These proteins seize control of host signaling responses through respective activation of STAT3/6, NFκB, and p38 MAPK molecules (Ong et al., 2010; Butcher et al., 2011; Rosowski et al., 2011; Braun et al., 2013). It is likely that a major factor in the success of Toxoplasma lies in its ability to produce host-directed effectors that act to ensure a balance between pro-inflammatory and anti-inflammatory responses. Host non-coding RNA responses are now emerging as important regulators of cell function. Regarding the host response to Toxoplasma, several microRNAs and a growing list of long non-coding RNAs are known to be triggered by infection. Here, we survey the current state of our knowledge of this important but still little understood class of host responses during infection with T. gondii.
Only a small percentage (<3%) of the genome codes for proteins, yet the majority (~80%) is actively transcribed (Spurlock et al., 2016; Atianand et al., 2017). The transcripts that do not code for proteins are collectively referred to as non-coding RNAs (ncRNAs) and they are separated into two general categories: shorter non-coding RNAs and longer non-coding RNAs (Xue et al., 2017; Zhang et al., 2017). Shorter non-coding RNAs are defined as being <200 nucleotides in length and include microRNAs (miRNAs), short interfering RNAs (siRNAs), transfer RNAs (tRNAs), piwi-interacting RNAs (piwiRNA), and small nucleolar RNAs (snoRNAs). Non-coding RNAs <200 nucleotides include ribosomal RNAs (rRNAs) and long non-coding RNAs (lncRNAs). Here, we focus on two types of non-coding RNAs: microRNAs and long non-coding RNAs. While several other classes of RNAs exist, miRNAs and lncRNAs are the two major classes of host ncRNA that have been examined during Toxoplasma infection.
MicroRNAs are ~18 to 22 nucleotides in length. While examples of miRNAs acting transcriptionally exist, they primarily function post-transcriptionally by directly binding to mRNAs through direct base pair interactions (Xue et al., 2017). This interaction leads to mRNA cleavage, mRNA degradation, or blocking of translation (Figure 1A). MicroRNAs play important roles in regulating both innate and adaptive immunity. For example, the miR-17-92 cluster regulates B-cell, T-cell, and monocyte development through downregulation of the proapoptotic protein Bim (Xiao et al., 2008). The miR-146 family is a negative regulator of the innate immune response and may target TRAF6 and IRAK1 (Taganov et al., 2006; Lindsay, 2008; Cannella et al., 2014). miR-155 is a regulator of T-cell and B-cell maturation, as well as the innate immune response (Lindsay, 2008; Cannella et al., 2014).
Figure 1. Comparison of miRNA and lncRNA function. (A) microRNAs function post-transcriptionally through direct base-pair interactions with mRNA. (B) Due to their larger size, lncRNAs have greater functional diversity and can interact with RNA, DNA, and protein. lncRNAs are known to influence gene expression at both the transcriptional and post-transcriptional level.
The largest group of RNA produced is long non-coding RNAs (lncRNAs), and it accounts for up to 68% of the transcriptome, not including ribosomal RNAs (Iyer et al., 2015; Chen et al., 2017). Compared to microRNAs, lncRNAs are much longer and more complex in structure and function. Thus, lncRNAs have multiple operational units and extensive functional diversity through their ability to interact with RNA, DNA, and protein (Guttman and Rinn, 2012; Fitzgerald and Caffrey, 2014; Chen et al., 2017). lncRNAs are widely involved in gene regulation at both transcriptional and post-transcriptional levels. Known functions of lncRNAs include transcriptional co-activation, recruitment of chromatin modifiers, miRNA sponges, regulation of splicing, and mRNA stabilization (Figure 1B; Fitzgerald and Caffrey, 2014; Szcześniak and Makałowska, 2016). The study of lncRNAs in the immune system is a relatively new field. In fact, the first study identifying a function for a particular lncRNA involved in the innate immune response was published as recently as 2013 (Carpenter et al., 2013). This lncRNA, lncRNA-Cox2, is broadly involved in both activation and repression of immune genes by performing functions both in cis at nearby genes and trans at genes on different chromosomes (Elling et al., 2018). In addition to lncRNA-Cox2, several other lncRNAs are now known to play a role in the immune response (Spurlock et al., 2016; Atianand et al., 2017; Chen et al., 2017). Recently, lncRNA responses were examined in epithelial cells infected with the intestinal pathogen Cryptosporidium parvum, an apicomplexan related to Toxoplasma. One lncRNA in particular, NR_045064, was found to regulate selected host defense genes through modifications in chromatin structure (Li et al., 2018).
Numerous studies have surveyed global host miRNA responses during T. gondii infection in different anatomical regions (liver, spleen, brain, plasma), cell types (primary human foreskin fibroblasts, neuroepitheliomal cells, peripheral blood mononuclear cell-derived macrophages), and mammalian species (mouse, human, cat, pig). Several different parasite strains and infectious stages of T. gondii have also been employed. These studies are summarized in Table 1. Here, we highlight some key findings as well as underscore some of the commonalities between the studies.
Table 1. Studies surveying host microRNAs and lncRNAs differentially expressed during T. gondii infection.
The first study profiling microRNAs differentially expressed during T. gondii infection was published almost a decade ago (Zeiner et al., 2010). It reported that 14% of the miRNAs on the microarray were differentially regulated, including members of the miR-17-92 and miR-106b-25 family that are upregulated after infection with RH in primary human foreskin fibroblasts (HFFs). Other studies have confirmed this finding in other cell types. The miR-17-92 polycistronic gene cluster was also found to be upregulated in human macrophages derived from peripheral blood mononuclear cells after infection with TgCtwh3 strain parasites (Cai et al., 2013, 2014). He et al. found that mir-17-5p (a member of miR-17-92 gene cluster) is upregulated in the mouse spleen after infection with the RH strain (He et al., 2016). Nevertheless, in an initial analysis knockdown of miR-17-92 in HFF had no observable effect on infection, parasite replication or host cell lysis (Zeiner et al., 2010).
Despite lack of an overt effect of miR-17-92 on the intracellular growth cycle of T. gondii, more recent work suggests a connection of this miRNA cluster with inhibition of host cell apoptosis. Blocking apoptosis is a well-documented survival strategy of Toxoplasma in the host cell (Lüder and Gross, 2005; Carmen and Sinai, 2007). Knocking down expression of miR-20a (a member of the miR-17-92 cluster) in infected macrophages resulted in increased sensitivity to apoptosis (Cai et al., 2013; Rezaei et al., 2018). The model proposed for how Toxoplasma induces miR-17-92 to inhibit apoptosis revolves around the parasite secretory kinase ROP16 (Figure 2). This protein is injected into the host cell cytoplasm where it tyrosine phosphorylates STAT3 (Saeij et al., 2007; Yamamoto et al., 2009). The miR-17-92 cluster contains STAT3 binding sites in its promoter and is therefore upregulated by STAT3 phosphorylation. Evidence for this comes from the observation that knockdown of STAT3 using siRNA prevents miR-17-92 upregulation. Furthermore, luciferase constructs with the binding site for STAT3 in the promoter region of miR-17-92 led to increased luciferase expression (Cai et al., 2013). STAT3-induced miR-17-92 then binds to the 3′ UTR of the Bim transcript, reducing BIM levels (Cai et al., 2014). The BIM protein is pro-apoptotic and, therefore, deficiency leads to the inhibition of apoptosis observed in host cells following infection with T. gondii.
Figure 2. Model for mir-17-92 inhibition of apoptosis during T. gondii infection. During intracellular infection, T. gondii injects the ROP16 protein into the host cell cytoplasm. In the cytoplasm, ROP16 phosphorylates STAT3. Phosphorylated STAT3 enters the nucleus of the host cell, binds to STAT3 sites in the promoter of the miR-17-92 cluster gene, and upregulates miR-17-92 miRNAs. miR-17-92 cluster miRNAs then bind to the Bim transcript and reduce BIM levels, thereby inhibiting the process of apoptosis. This model is based upon data reported in Cai et al. (2013, 2014).
The miRNA miR-132 has both neural and immune functions and dysregulation has been associated with numerous neurological disorders (Soreq and Wolf, 2011; Miller et al., 2012; Wanet et al., 2012). Xiao et al. surveyed miRNA expression profiles in neural cells (SK-N-MC cells) acutely infected with Type I, Type II, and Type III Toxoplasma strains (Xiao et al., 2014). They found that miR-132 was the only miRNA upregulated by >2-fold by all three T. gondii strains, and this upregulation was confirmed in the peritoneal cavity and striatum of infected mice. Among the predicted targets of miR-132, the strongest pathway enriched was that for dopamine signaling. Three genes (Drd1, Drd5, and Maoa) in the dopamine signaling pathway displayed decreased transcription and protein expression in T. gondii-infected mice. HPLC data also demonstrated increased production of dopamine, serotonin, and 5-hydroxyindoleacetic acid in the striatum of Toxoplasma-infected mice. miR-132 is known to be upregulated by LPS and several viruses (Taganov et al., 2006; Lagos et al., 2010). These combined results suggest that miR-132 may be a common target of a broad range of pathogens and may represent a general response to infection. It is also worth noting that other researchers have reported dysregulation of dopamine pathways during Toxoplasma infection, suggesting possible links to nervous system abnormalities (Syn et al., 2018; Alsaady et al., 2019).
In a recent study, expression of miR-132 in the mouse brain during chronic (5 month) infection with PRU strain parasites was investigated (Li et al., 2015). Contrary to acute infection, chronic infection resulted in decreased expression of miR-132 relative to non-infected mice. Correlation between levels of miR-132 in different brain regions and the number of parasites was weak, suggesting that the effects of Toxoplasma on miRNA expression were indirect.
Cannella et al. surveyed microRNAs differentially expressed between T. gondii high and low virulence strains to determine if differences in host pathogenesis correlated with specific microRNAs (Cannella et al., 2014). This resulted in identification of miR-146a and miR155. These investigators found that miR-146a was induced by Type II but not by Type I tachyzoites in several non-hematopoietic cell types. In addition, levels of miR-146a increased in the central nervous system of mice chronically infected with Type II Toxoplasma and were correlated with the presence of cysts. An independent study also found miR-146a was induced in the brain during chronic infection initiated with PRU oocysts (Hu et al., 2018).
In contrast to Type II T. gondii, Type I Toxoplasma strains were found to repress miR-146a levels. Furthermore, when ROP16 was deleted in Type I strains, expression of miR-146a was greatly enhanced. ROP16 deletion in Type II strains had no effect on miR-146a expression as predicted given the lack of kinase activity of this rhoptry protein. Interestingly, miR-146a−/− mice displayed increased resistance to Type II T. gondii (Cannella et al., 2014). The lower levels of IFN-γ in miR-146a knockout mice suggests that increased resistance results from avoidance of cytokine-induced inflammation that can lead to pathology and death when not appropriately controlled (Gazzinelli et al., 1996; Gavrilescu and Denkers, 2001; Mordue et al., 2001).
The miRNA miR-155 is known to target SOCS1 mRNA to promote inflammation (Rao et al., 2014). Cannella et al. reported that miR-155 was induced after T. gondii infection (Cannella et al., 2014). Induction was strain-independent during in vitro infection of stromal and phagocytic cells. In vivo, miR-155 was induced in the central nervous system of mice infected with Type II Toxoplasma strains. Another study found that miR-155 was induced in mouse spleens after infection with RH tachyzoites (He et al., 2016). However, it is worth noting that in a study in pigs, miR-155 was downregulated during chronic infection (Hou et al., 2019). The reason for this conflicting result is not clear and could be due to host species or T. gondii strain differences. Nevertheless, taken together the data indicate that both miR-146a and miR-155 are triggered by Toxoplasma. At least for the case of miR-146a, this appears to impact the outcome of infection.
Parasites exploit exosomes to influence host responses during infection (Gavinho et al., 2018; Wu et al., 2018; Atayde et al., 2019). It is, therefore, of interest that HFF infected with T. gondii release exosome-like vesicles containing several distinct miRNA species (Pope and Lässer, 2013). Thus, 10 miRNAs (miR-92a, miR-595, miR125b, miR-199a-3p, miR-125a-5p, miR-503, miR-320d, miR-1183, miR-99a-star, miR-23b) were identified within exosomes isolated from infected cells. The miRNA miR-23b is particularly of interest because it is a known negative regulator of IL-17 synthesis (Hu and O'Connell, 2012; Zhu et al., 2012). A more recent study identified as many as 64 distinct microRNAs that were present within exosomes derived from cells infected with RH vs. exosomes derived from uninfected cells (Kim et al., 2016). Additionally, the Cannella et al. (2014) study identified expression of a specific miRNA, miR-146a, in exosomes of Type II infected cells. Together, these data suggest that nearby uninfected cells (and not just infected cells) experience altered microRNA expression during infection. The influence of miRNA on exosome infection biology is likely to be a fruitful area of investigation in the near future.
To date, we know very little regarding expression of host long non-coding RNAs during Toxoplasma infection. Here, we highlight two studies that provide some interesting first glances at regulation of this class of RNA during T. gondii infection. While these studies are intriguing, direct comparison of the results is complicated by the small degree of sequence conservation between lncRNAs of different species (Spurlock et al., 2016).
One group of investigators examined lncRNAs differentially regulated in HFF cell lines during infection with Type II (ME49) T. gondii (Liu et al., 2018). They found that 1,206 lncRNAs were upregulated after infection, including 996 that were only induced by active infection relative to heat-inactivated parasites. Interestingly, inactivated parasites upregulated 392 lncRNAs when compared to uninfected cells, demonstrating that simply the presence of dead parasites can elicit effects on lncRNA expression. It was found that one particular lncRNA, NONHSAT022487, is highly upregulated after Type II infection. Knock down of NONHSAT022487 resulted in increased expression of cytokines IL-12, TNF-α, IL-1β, and IFN-γ in T. gondii-infected THP-1 monocytic cells. Overexpression of NONHSAT022487 resulted in decreased expression of these four cytokines. This lncRNA would therefore appear to be a prime candidate to further explore how it exerts its effects on proinflammatory cytokine gene expression.
We surveyed the expression of lncRNA in mouse bone marrow-derived macrophages during infection with both Type I (RH) and Type II (PTG) strains (Menard et al., 2018). We found that hundreds of putative lncRNAs were differentially regulated, and a substantially greater number were differentially regulated with RH rather than PTG infection (1,522 and 528, respectively). The total number of lncRNAs upregulated by infection was similar to the number down-regulated. Interestingly, we also found that Toxoplasma likely directly controls expression of host lncRNAs, as upregulation of two lncRNAs was ablated in parasite strains deleted for the STAT3/6-directed kinase ROP16. Functional studies are now required to identify biologically important lncRNAs in the response to infection.
We have only a nascent understanding of the role of non-coding RNA in host defense against infection. However, we now know that there is a large number of miRNA that are up or downregulated during T. gondii infection, including the miR-17-92 gene cluster, miR-132, miR-146a, miR-155, and miR-23b. At least some of these miRNAs play important roles in the response to T. gondii. Clearly, there are many miRNA yet to be characterized, and it is likely that some will prove to significantly impact host defense largely in ways yet to be discovered. Our knowledge of lncRNA responses to Toxoplasma is even more rudimentary. However, the finding that thousands of lncRNAs that are up or downregulated during T. gondii infection indicates that host lncRNA responses are extensive, and most likely biologically important. Future work is required to determine those regulatory RNAs that are paramount in the response to infection with Toxoplasma and other microbial pathogens.
All authors listed have made a substantial, direct and intellectual contribution to the work, and approved it for publication.
Our lncRNA work was supported by the National Institute of General Medical Sciences of the National Institutes of Health under award number P30 GM110907.
The authors declare that the research was conducted in the absence of any commercial or financial relationships that could be construed as a potential conflict of interest.
Alsaady, I., Tedford, E., Alsaad, M., Bristow, G., Kohli, S., Murray, M., et al. (2019). Downregulation of the central noradrenergic system by Toxoplasma gondii Infection. Infect. Immun. 87, 1–16. doi: 10.1128/IAI.00789-18
Andrade, W. A., Souza, M. D., Ramos-Martinez, E., Nagpal, K., Dutra, M. S., Melo, M. B., et al. (2013). Combined action of nucleic acid-sensing toll-like receptors and TLR11/TLR12 heterodimers imparts resistance to Toxoplasma gondii in mice. Cell Host Microbe 13, 42–53. doi: 10.1016/j.chom.2012.12.003
Atayde, V. D., da Silva Lira Filho, A., Chaparro, V., Zimmermann, A., Martel, C., Jaramillo, M., et al. (2019). Exploitation of the Leishmania exosomal pathway by Leishmania RNA virus 1. Nat. Microbiol. 4, 714–723. doi: 10.1038/s41564-018-0352-y
Atianand, M. K., Caffrey, D. R., and Fitzgerald, K. A. (2017). Immunobiology of long noncoding RNAs. Ann. Rev. Immunol. 35, 177–198. doi: 10.1146/annurev-immunol-041015-055459
Braun, L., Brenier-Pinchart, M. P., Yogavel, M., Curt-Varesano, A., Curt-Bertini, R. L., Hussain, T., et al. (2013). A Toxoplasma dense granule protein, GRA24, modulates the early immune response to infection by promoting a direct and sustained host p38 MAPK activation. J. Exp. Med. 210, 2071–2086. doi: 10.1084/jem.20130103
Butcher, B. A., Fox, B. A., Rommereim, L. M., Kim, S. G., Maurer, K. J., Yarovinsky, F., et al. (2011). Toxoplasma gondii rhoptry kinase ROP16 activates STAT3 and STAT6 resulting in cytokine inhibition and arginase-1-dependent growth control. PLoS Pathog. 7:e1002236. doi: 10.1371/journal.ppat.1002236
Cai, Y., Chen, H., Jin, L., You, Y., and Shen, J. (2013). STAT3-dependent transactivation of miRNA genes following Toxoplasma gondii infection in macrophage. Parasit. Vectors 6:356. doi: 10.1186/1756-3305-6-356
Cai, Y., Chen, H., Mo, X., Tang, Y., Xu, X., Zhang, A., et al. (2014). Toxoplasma gondii inhibits apoptosis via a novel STAT3-miR-17-92-Bim pathway in macrophages. Cell. Signal. 26, 1204–1212. doi: 10.1016/j.cellsig.2014.02.013
Cannella, D., Brenier-Pinchart, M. P., Braun, L., van Rooyen, J. M., Bougdour, A., Bastien, O., et al. (2014). miR-146a and miR-155 delineate a MicroRNA fingerprint associated with Toxoplasma persistence in the host brain. Cell Rep. 6, 928–937. doi: 10.1016/j.celrep.2014.02.002
Carmen, J. C., and Sinai, A. P. (2007). Suicide prevention: disruption of apoptotic pathways by protozoan parasites. Mol. Microbiol. 64, 904–916. doi: 10.1111/j.1365-2958.2007.05714.x
Carpenter, S., Aiello, D., Atianand, M. K., Ricci, E. P., Gandhi, P., Hall, L. L., et al. (2013). A long noncoding RNA mediates both activation and repression of immune response genes. Science 341, 789–792. doi: 10.1126/science.1240925
Chen, Y. G., Satpathy, A. T., and Chang, H. Y. (2017). Gene regulation in the immune system by long noncoding RNAs. Nat. Immunol. 18, 962–972. doi: 10.1038/ni.3771
Cong, W., Zhang, X. X., He, J. J., Li, F. C., Elsheikha, H. M., and Zhu, X. Q. (2017). Global miRNA expression profiling of domestic cat livers following acute Toxoplasma gondii infection. Oncotarget 8, 25599–25611. doi: 10.18632/oncotarget.16108
Dupont, C. D., Christian, D. A., and Hunter, C. A. (2012). Immune response and immunopathology during toxoplasmosis. Semin. Immunopathol. 34, 793–813. doi: 10.1007/s00281-012-0339-3
Elling, R., Robinson, E. K., Shapleigh, B., Liapis, S. C., Covarrubias, S., Katzman, S., et al. (2018). Genetic models reveal cis and trans immune-regulatory activities for lincRNA-Cox2. Cell Rep. 25, 1511–1524 e1516. doi: 10.1016/j.celrep.2018.10.027
Fitzgerald, K. A., and Caffrey, D. R. (2014). Long noncoding RNAs in innate and adaptive immunity. Curr. Opin. Immunol. 26, 140–146. doi: 10.1016/j.coi.2013.12.001
Gavinho, B., Rossi, I. V., Evans-Osses, I., Inal, J., and Ramirez, M. I. (2018). A new landscape of host-protozoa interactions involving the extracellular vesicles world. Parasitology 145, 1521–1530. doi: 10.1017/S0031182018001105
Gavrilescu, L. C., and Denkers, E. Y. (2001). IFN-g overproduction and high level apoptosis are associated with high but not low virulence Toxoplasma gondii infection. J. Immunol. 167, 902–909. doi: 10.4049/jimmunol.167.2.902
Gazzinelli, R. T., Mendonça-Neto, R., Lilue, J., Howard, J., and Sher, A. (2014). Innate resistance against Toxoplasma gondii: an evolutionary tale of mice, cats, and men. Cell Host Microbe 15, 132–138. doi: 10.1016/j.chom.2014.01.004
Gazzinelli, R. T., Wysocka, M., Hieny, S., Scharton-Kersten, T., Cheever, A., Kuhn, R., et al. (1996). In the absence of endogenous IL-10, mice acutely infected with Toxoplasma gondii succumb to a lethal immune response dependent upon CD4+ T cells and accompanied by overproduction of IL-12, IFN-g, and TNF-a. J. Immunol. 157, 798–805.
Guttman, M., and Rinn, J. L. (2012). Modular regulatory principles of large non-coding RNAs. Nature 482, 339–346. doi: 10.1038/nature10887
Hakimi, M. A., Olias, P., and Sibley, L. D. (2017). Toxoplasma effectors targeting host signaling and transcription. Clin. Microbiol. Rev. 30, 615–645. doi: 10.1128/CMR.00005-17
He, H., Brenier-Pinchart, M. P., Braun, L., Kraut, A., Touquet, B., Couté, Y., et al. (2018). Characterization of a Toxoplasma effector uncovers an alternative GSK3/beta-catenin-regulatory pathway of inflammation. Elife 7:e39887. doi: 10.7554/eLife.39887
He, J. J., Ma, J., Wang, J. L., Xu, M. J., and Zhu, X. Q. (2016). Analysis of miRNA expression profiling in mouse spleen affected by acute Toxoplasma gondii infection. Infect. Genet. Evol. 37, 137–142. doi: 10.1016/j.meegid.2015.11.005
Hou, Z., Liu, D., Su, S., Wang, L., Zhao, Z., Ma, Y., et al. (2019). Comparison of splenocyte microRNA expression profiles of pigs during acute and chronic toxoplasmosis. BMC Genom. 20:97. doi: 10.1186/s12864-019-5458-y
Hu, R., and O'Connell, R. M. (2012). MiR-23b is a safeguard against autoimmunity. Nat. Med. 18, 1009–1010. doi: 10.1038/nm.2849
Hu, R. S., He, J. J., Elsheikha, H. M., Zhang, F. K., Zou, Y., Zhao, G. H., et al. (2018). Differential brain microRNA expression profiles after acute and chronic infection of mice with Toxoplasma gondii oocysts. Front. Microbiol. 9:2316. doi: 10.3389/fmicb.2018.02316
Iyer, M. K., Niknafs, Y. S., Malik, R., Singhal, U., Sahu, A., Hosono, Y., et al. (2015). The landscape of long noncoding RNAs in the human transcriptome. Nat. Genet. 47, 199–208. doi: 10.1038/ng.3192
Jia, B., Chang, Z., Wei, X., Lu, H., Yin, J., Jiang, N., et al. (2014). Plasma microRNAs are promising novel biomarkers for the early detection of Toxoplasma gondii infection. Parasit. Vectors 7:433. doi: 10.1186/1756-3305-7-433
Kim, M. J., Jung, B. K., Cho, J., Song, H., Pyo, K. H., Lee, J. M., et al. (2016). Exosomes secreted by Toxoplasma gondii-infected L6 cells: their effects on host cell proliferation and cell cycle changes. Korean J. Parasitol. 54, 147–154. doi: 10.3347/kjp.2016.54.2.147
Lagos, D., Pollara, G., Henderson, S., Gratrix, F., Fabani, M., Milne, R. S., et al. (2010). miR-132 regulates antiviral innate immunity through suppression of the p300 transcriptional co-activator. Nat. Cell Biol. 12, 513–519. doi: 10.1038/ncb2054
Li, M., Gong, A. Y., Zhang, X. T., Wang, Y., Mathy, N. W., Martins, G. A., et al. (2018). Induction of a long noncoding RNA transcript, NR_045064, promotes defense gene transcription and facilitates intestinal epithelial cell responses against Cryptosporidium infection. J. Immunol. 201, 3630–3640. doi: 10.4049/jimmunol.1800566
Li, S., Yang, J., Wang, L., Du, F., Zhao, J., and Fang, R. (2019). Expression profile of microRNAs in porcine alveolar macrophages after Toxoplasma gondii infection. Parasit. Vectors 12:65. doi: 10.1186/s13071-019-3297-y
Li, Y. E., Kannan, G., Pletnikov, M. V., Yolken, R. H., and Xiao, J. (2015). Chronic infection of Toxoplasma gondii downregulates miR-132 expression in multiple brain regions in a sex-dependent manner. Parasitology 142, 623–632. doi: 10.1017/S003118201400167X
Lindsay, M. A. (2008). microRNAs and the immune response. Trends Immunol. 29, 343–351. doi: 10.1016/j.it.2008.04.004
Liu, W., Huang, L., Wei, Q., Zhang, Y., Zhang, S., Zhang, W., et al. (2018). Microarray analysis of long non-coding RNA expression profiles uncovers a Toxoplasma-induced negative regulation of host immune signaling. Parasit. Vectors 11:174. doi: 10.1186/s13071-018-2697-8
Lüder, C. G. K., and Gross, U. (2005). Apoptosis and its modulation during infection with Toxoplasma gondii: molecular mechanisms and role in pathogenesis. Curr. Top. Microbiol. Immunol. 289, 219–238. doi: 10.1007/3-540-27320-4_10
McLeod, R. M., van Tubbergen, C., Montoya, J. G., and Petersen, E. (2013). “Human Toxoplasma infection,” in Toxoplasma gondii. The Model Apicomplexan: Perspectives and Methods, eds L. M. Weiss and K. Kim (San Diego, CA: Academic Press), 100–159.
Menard, K. L., Haskins, B. E., Colombo, A. P., and Denkers, E. Y. (2018). Toxoplasma gondii manipulates expression of host long noncoding RNA during intracellular infection. Sci. Rep. 8:15017. doi: 10.1038/s41598-018-33274-5
Miller, B. H., Zeier, Z., Xi, L., Lanz, T. A., Deng, S., Strathmann, J., et al. (2012). MicroRNA-132 dysregulation in schizophrenia has implications for both neurodevelopment and adult brain function. Proc. Natl. Acad. Sci. U.S.A. 109, 3125–3130. doi: 10.1073/pnas.1113793109
Mordue, D. G., Monroy, F., La Regina, M., Dinarello, C. A., and Sibley, L. D. (2001). Acute toxoplasmosis leads to lethal overproduction of Th1 cytokines. J. Immunol. 167, 4574–4584. doi: 10.4049/jimmunol.167.8.4574
Ngô, H. M., Zhou, Y., Lorenzi, H., Wang, K., Kim, T. K., Zhou, Y., et al. (2017). Toxoplasma modulates signature pathways of human epilepsy, neurodegeneration and cancer. Sci. Rep. 7:11496. doi: 10.1038/s41598-017-10675-6
Olias, P., Etheridge, R. D., Zhang, Y., Holtzman, M. J., and Sibley, L. D. (2016). Toxoplasma effector recruits the Mi-2/NuRD complex to repress STAT1 transcription and block IFN-gamma-Dependent gene expression. Cell Host Microbe 20, 72–82. doi: 10.1016/j.chom.2016.06.006
Ong, Y. C., Reese, M. L., and Boothroyd, J. C. (2010). Toxoplasma rhoptry protein 16 (ROP16) subverts host function by direct tyrosine phosphorylation of STAT6. J. Biol. Chem. 285, 28731–28740. doi: 10.1074/jbc.M110.112359
Pope, S. M., and Lässer, C. (2013). Toxoplasma gondii infection of fibroblasts causes the production of exosome-like vesicles containing a unique array of mRNA and miRNA transcripts compared to serum starvation. J. Extracell. Vesicles 2, 1–8. doi: 10.3402/jev.v2i0.22484
Raetz, M., Kibardin, A., Sturge, C. R., Pifer, R., Li, H., Burstein, E., et al. (2013). Cooperation of TLR12 and TLR11 in the IRF8-dependent IL-12 response to Toxoplasma gondii profilin. J. Immunol. 191, 4818–4827. doi: 10.4049/jimmunol.1301301
Rao, R., Rieder, S. A., Nagarkatti, P., and Nagarkatti, M. (2014). Staphylococcal enterotoxin B-induced microRNA-155 targets SOCS1 to promote acute inflammatory lung injury. Infect. Immun. 82, 2971–2979. doi: 10.1128/IAI.01666-14
Rezaei, F., Daryani, A., Sharifi, M., Sarvi, S., Jafari, N., Pagheh, A. S., et al. (2018). miR-20a inhibition using locked nucleic acid (LNA) technology and its effects on apoptosis of human macrophages infected by Toxoplasma gondii RH strain. Microb. Pathog. 121, 269–276. doi: 10.1016/j.micpath.2018.05.030
Rosowski, E. E., Lu, D., Julien, L., Rodda, L., Gaiser, R. A., Jensen, K. D., et al. (2011). Strain-specific activation of the NF-kappaB pathway by GRA15, a novel Toxoplasma gondii dense granule protein. J. Exp. Med. 208, 195–212. doi: 10.1084/jem.20100717
Saeij, J. P., Coller, S., Boyle, J. P., Jerome, M. E., White, M. W., and Boothroyd, J. C. (2007). Toxoplasma co-opts host gene expression by injection of a polymorphic kinase homologue. Nature 445, 324–327. doi: 10.1038/nature05395
Sasai, M., Pradipta, A., and Yamamoto, M. (2018). Host immune responses to Toxoplasma gondii. Int. Immunol. 30, 113–119. doi: 10.1093/intimm/dxy004
Soreq, H., and Wolf, Y. (2011). NeurimmiRs: microRNAs in the neuroimmune interface. Trends Mol. Med. 17, 548–555. doi: 10.1016/j.molmed.2011.06.009
Spurlock, C. F. III., Crooke, P. S. III., and Aune, T. M. (2016). Biogenesis and transcriptional regulation of long noncoding RNAs in the human immune system. J. Immunol. 197, 4509–4517. doi: 10.4049/jimmunol.1600970
Syn, G., Anderson, D., Blackwell, J. M., and Jamieson, S. E. (2018). Epigenetic dysregulation of host gene expression in Toxoplasma infection with specific reference to dopamine and amyloid pathways. Infect. Genet. Evol. 65, 159–162. doi: 10.1016/j.meegid.2018.07.034
Szcześniak, M. W., and Makałowska, I. (2016). lncRNA-RNA interactions across the human transcriptome. PLoS ONE 11:e0150353. doi: 10.1371/journal.pone.0150353
Taganov, K. D., Boldin, M. P., Chang, K. J., and Baltimore, D. (2006). NF-kappaB-dependent induction of microRNA miR-146, an inhibitor targeted to signaling proteins of innate immune responses. Proc. Natl. Acad. Sci. U.S.A. 103, 12481–12486. doi: 10.1073/pnas.0605298103
Wanet, A., Tacheny, A., Arnould, T., and Renard, P. (2012). miR-212/132 expression and functions: within and beyond the neuronal compartment. Nucleic Acids Res. 40, 4742–4753. doi: 10.1093/nar/gks151
Wu, Z., Wang, L., Li, J., Wang, L., Wu, Z., and Sun, X. (2018). Extracellular vesicle-mediated communication within host-parasite interactions. Front. Immunol. 9:3066. doi: 10.3389/fimmu.2018.03066
Xiao, C., Srinivasan, L., Calado, D. P., Patterson, H. C., Zhang, B., Wang, J., et al. (2008). Lymphoproliferative disease and autoimmunity in mice with increased miR-17-92 expression in lymphocytes. Nat. Immunol. 9, 405–414. doi: 10.1038/ni1575
Xiao, J., Li, Y., Prandovszky, E., Karuppagounder, S. S., Talbot, C. C. Jr., Dawson, V. L., et al. (2014). MicroRNA-132 dysregulation in Toxoplasma gondii infection has implications for dopamine signaling pathway. Neuroscience 268, 128–138. doi: 10.1016/j.neuroscience.2014.03.015
Xu, M. J., Zhou, D. H., Nisbet, A. J., Huang, S. Y., Fan, Y. F., and Zhu, X. Q. (2013). Characterization of mouse brain microRNAs after infection with cyst-forming Toxoplasma gondii. Parasit. Vectors 6:154. doi: 10.1186/1756-3305-6-154
Xue, M., Zhuo, Y., and Shan, B. (2017). MicroRNAs, long noncoding RNAs, and their functions in human disease. Methods Mol. Biol. 1617, 1–25. doi: 10.1007/978-1-4939-7046-9_1
Yamamoto, M., Standley, D. M., Takashima, S., Saiga, H., Okuyama, M., Kayama, H., et al. (2009). A single polymorphic amino acid on Toxoplasma gondii kinase ROP16 determines the direct and strain-specific activation of Stat3. J. Exp. Med. 206, 2747–2760. doi: 10.1084/jem.20091703
Yarovinsky, F. (2014). Innate immunity to Toxoplasma gondii infection. Nat. Rev. Immunol. 14, 109–121. doi: 10.1038/nri3598
Zeiner, G. M., Norman, K. L., Thomson, J. M., Hammond, S. M., and Boothroyd, J. C. (2010). Toxoplasma gondii infection specifically increases the levels of key host microRNAs. PLoS ONE 5:e8742. doi: 10.1371/journal.pone.0008742
Zhang, Y., Cai, S., Jia, Y., Qi, C., Sun, J., Zhang, H., et al. (2017). Decoding noncoding RNAs: role of microRNAs and long noncoding RNAs in ocular neovascularization. Theranostics 7, 3155–3167. doi: 10.7150/thno.19646
Keywords: non-coding RNA, microRNA, miRNA, long non-coding RNA, lncRNA, Toxoplasma gondii, parasite
Citation: Menard KL, Haskins BE and Denkers EY (2019) Impact of Toxoplasma gondii Infection on Host Non-coding RNA Responses. Front. Cell. Infect. Microbiol. 9:132. doi: 10.3389/fcimb.2019.00132
Received: 07 March 2019; Accepted: 12 April 2019;
Published: 14 May 2019.
Edited by:
Nicolas Blanchard, INSERM U1043 Centre de Physiopathologie de Toulouse Purpan, FranceReviewed by:
Mohamed Ali Hakimi, Institut National de la Santé et de la Recherche Médicale (INSERM), FranceCopyright © 2019 Menard, Haskins and Denkers. This is an open-access article distributed under the terms of the Creative Commons Attribution License (CC BY). The use, distribution or reproduction in other forums is permitted, provided the original author(s) and the copyright owner(s) are credited and that the original publication in this journal is cited, in accordance with accepted academic practice. No use, distribution or reproduction is permitted which does not comply with these terms.
*Correspondence: Kayla L. Menard, a21lbmFyZEB1bm0uZWR1
Eric Y. Denkers, ZWRlbmtlcnNAdW5tLmVkdQ==
Disclaimer: All claims expressed in this article are solely those of the authors and do not necessarily represent those of their affiliated organizations, or those of the publisher, the editors and the reviewers. Any product that may be evaluated in this article or claim that may be made by its manufacturer is not guaranteed or endorsed by the publisher.
Research integrity at Frontiers
Learn more about the work of our research integrity team to safeguard the quality of each article we publish.