- 1Molecular Biology Department, Instituto de Parasitología y Biomedicina “López Neyra”, Consejo Superior de Investigaciones Científicas, Spain
- 2Programa de Estudio y Control de Enfermedades Tropicales, Facultad de Medicina, Universidad de Antioquia, Colombia
- 3Bionaturis Group, Bioorganic Research and Services, S.A., Spain
The host immunological response is a key factor determining the pathogenesis of cutaneous leishmaniasis. It is known that a Th1 cellular response is associated with infection control and that antigen-specific memory T cells are necessary for the development of a rapid and strong protective cellular response. The present manuscript reports the analysis of the functional and phenotypic profiles of antigen-specific CD4+ and CD8+ T cells from patients cured of cutaneous leishmaniasis (CL), patients with an active process of cutaneous leishmaniasis, asymptomatic individuals with a positive Montenegro test and healthy donors (HD). Peripheral blood mononuclear cells (PBMCs) from the patients exhibited a lymphoproliferative capacity after stimulation with total soluble protein from either Leishmania panamensis (SLpA) or Leishmania infantum (SLiA) or with a recombinant paraflagellar rod protein-1 (rPFR1). Higher frequencies of antigen-specific TNAIVE cells, mainly following stimulation with rPFR1, were observed in asymptomatic and cured patients than in patients with active cutaneous leishmaniasis, while T cells from patients with active cutaneous leishmaniasis showed a higher percentage of effector memory T cells (TEM for CD4+ T cells and TEMRA for CD8+ T cells). The amount of antigen-specific CD57+/CD8+ TEMRA cells in patients with active cutaneous leishmaniasis was higher than that in cured patients and asymptomatic subjects. Regarding functionality, a more robust multifunctional CD8+ T cell response was detected in cured patients than in those with active cutaneous leishmaniasis. Moreover, cured patients showed a significant increase in the frequency of cells expressing a Th1-type cytotoxic production profile (IFN-γ+/granzyme-B/+perforin+). Patients with an active leishmaniosis process had a significantly higher frequency of CD8+ T cells expressing the inhibitory CD160 and 2B4 receptors than did cured patients. The expression profile observed in cured patients could be indicative of an imbalance toward a CD8+ Th1 response, which could be associated with infection control; consequently, the determination of this profile could be a useful tool for facilitating the clinical follow-up of patients with cutaneous leishmaniasis. The results also suggest a possible exhaustion process of CD8+ T cells associated with the evolution of Leishmania infection.
Introduction
Leishmaniasis is caused by intracellular parasites belonging to Leishmania species, and 1.5–2 million new cases are reported annually worldwide (WHO, 2016). Therapeutic options are limited, and there is no effective vaccine (Alvar et al., 2012). Depending on the infecting Leishmania species, different clinical manifestations occur, with cutaneous leishmaniasis (CL) being the most prevalent clinical form (de Vries et al., 2015). In South and Central America, Leishmania parasites of the subgenus Viannia are the most prevalent etiologic agents of human CL (Castilho et al., 2010). Systematic studies carried out in different areas of Colombia since the 1980s showed the presence of six species belonging to the genus Leishmania, with a higher prevalence of Leishmania panamensis (50.8–74.5%) and Leishmania braziliensis (15.3–30.3%) isolates than isolates of the other species. It is thought that 97% of the pathologies caused by Leishmania spp. in Colombia correspond to CL (Corredor et al., 1990; Ovalle et al., 2006; Ramírez et al., 2016).
The infections caused by Leishmania species are, in many cases, self-healing, so it is assumed that the host immune response is a key factor that determines the pathogenesis of the infection. It has been widely reported that the Th1 response is critical for the control of Leishmania infection, since this response creates a cytokine environment that promotes the clearance of the parasite by macrophages (Kaye and Scott, 2011). The development of Leishmania infection in IFN-γ- and TNF-α-deficient murine models increased the lesion sizes and the parasite burdens (Theodos et al., 1991; Wilhelm et al., 2001; Pinheiro and Rossi-Bergmann, 2007). CD4+ and CD8+ T cells play a central role in the Th1 response by producing IFN-γ, TNF-α, and other Th1 cytokines that are essential for controlling parasite growth (da Silva Santos and Brodskyn, 2014). Thus, the cellular immune functions performed by these T cells are fundamental for eliminating the parasites, although there is evidence that CD8+ cytotoxic T lymphocytes (CTL) are involved in tissue damage in CL patients through cytotoxic mediators (Faria et al., 2009; Santos Cda et al., 2013). It is equally important to note that T lymphocytes play a critical role in protection against reinfection by Leishmania species. In this sense, after primary infection, long-lived memory T cell populations are maintained in the absence of antigens and are able to mediate immunity against a second infection (Glennie and Scott, 2016). It has been reported that cured patients who have overcome an episode of CL harbor specific effector memory T cells (TEMs) that produce IFN-γ and central memory T cells (TCMs) that produce IL-2 in response to stimulation with soluble leishmania antigens (Keshavarz Valian et al., 2013).
During the chronic stage of Leishmania infection, antigen-specific T cells become functionally impaired, as has been observed in other protozoan diseases (Gigley et al., 2012; Rodrigues et al., 2014). This dysfunctional process, known as T cell exhaustion, occurs gradually, with the upregulation of both the expression and coexpression of inhibitory receptor molecules in the membrane of T cells. It has been reported that CD8+ T cells from patients with visceral leishmaniasis exhibit an increased expression of the inhibitory receptors CTLA-4 and PD-1 (Gautam et al., 2014). In experimental models of Leishmania donovani infection, the blockade of the PD-1/PD-L1 pathway partially restored CD8+ T cell immune functions and significantly reduced the splenic parasite burden (Joshi et al., 2009; Hernández-Ruiz et al., 2010). Nevertheless, further information is needed to understand this exhaustion process in the context of Leishmania infection and its impact on the progression of leishmaniasis.
A systematic review of biomarkers for monitoring therapeutic responses in leishmaniasis (Kip et al., 2015) stated that sensitive and specific markers that are capable of assessing therapeutic efficacy and are able to predict long-term clinical outcomes using noninvasive sampling methods are urgently needed. The paraflagellar rod proteins (PFRs) represent a family of relevant trypanosomatid antigens that are located in the paraflagellar pocket of these parasites (Cachon et al., 1988). Knockout assays in Leishmania mexicana demonstrated that the proteins encoded by PFR genes play a critical role in the mobility and survival of the parasite (Santrich et al., 1997). Some members of the PFR antigen family stand out due to their high immunogenicity (Michailowsky et al., 2003). Additionally, a very recent study highlighted the potential of a recombinant PFR1 antigen for the serological diagnosis of Leishmania infantum infection (Ledesma et al., 2017). In the context of trypanosomatid infection by Trypanosoma cruzi, the immunization of mice with a protein from the PFR family (PFR2) fused to HSP70 as a DNA vaccine provided a protective response against T. cruzi experimental infection by inducing an increase in the expression of IL-2 and IFN-γ by splenic CD8+ T cells and by the generation of antigen-specific T cells (Morell et al., 2006).
In the present work, the cellular mechanisms that are triggered in human patients with different clinical statuses related to Leishmania infection were studied. For this purpose, a phenotypic and functional characterization of antigen-specific CD4+ and CD8+ T cells from patients cured of CL, patients with active CL (CL), asymptomatic individuals with a positive Montenegro test and healthy donors (HD) was conducted. Additionally, the degree of exhaustion and the senescence profile of these antigen-specific T cells were evaluated.
Materials and Methods
Peripheral Blood Mononuclear Cells Isolation of the Study Populations
Peripheral blood was collected from Montenegro-positive subjects (n = 4), cutaneous leishmaniasis patients (n = 4), patients cured of leishmaniasis (n = 5), and healthy donors from endemic (n = 4), and nonendemic areas (n = 6). All individuals included in this study live in Colombia. Peripheral blood (~20 mL) from each individual was collected by venipuncture into EDTA-containing tubes (BD Vacutainer), and peripheral blood mononuclear cells (PBMCs) were purified as previously described (Egui et al., 2012). The PBMCs were suspended in inactivated fetal bovine serum (iFBS) (Gibco, Grand Island, NY) containing 10% DMSO and cryopreserved in liquid nitrogen until use.
Individuals with evidence of exposure to Leishmania but without clinical manifestations of disease were identified by a positive Montenegro skin test. To this end, 0.1 mL of leishmanin (provided by PECET, Antioquia University, Medellin-Colombia) was subcutaneously inoculated into the arms of healthy subjects living in a leishmaniasis-endemic region of Colombia (Caldas Department) where the most widespread species of Leishmania is L. panamensis (Corredor et al., 1990; Ovalle et al., 2006; Ramírez et al., 2016). Subjects positive to Montenegro skin test were carefully clinically evaluated. They were considered asymptomatic when it was confirmed that they never presented lesions or scarring due to Leishmania infection in their clinical history, and were never treated against this disease. The diagnosis of CL patients was based on combined procedures, using direct microscopic examination, parasite growth, and molecular (PCR) methods. Smear preparations from the skin lesions were fixed and stained with Giemsa for microscopic observation and also cultivated in NNN media (Robinson et al., 2002). The identification of the Leishmania species causing the infection was carried out by PCR-RFLP following a previously published protocol (Montalvo et al., 2008). All the collected samples corresponded to the L. panamensis species. L. panamensis infection requires specific treatment. There are no data that report spontaneous cure in patients infected with L. panamensis in Colombia. All the cutaneous leishmaniasis patients presented from two to six cutaneous lesions. Blood was collected from these patients before the administration of antiparasitic treatment. The enrolled patients who had been cured of leishmaniasis had not presented any cutaneous lesions compatible with leishmaniasis for at least the previous 2 years.
Ethical Considerations
The Human Research Bioethics Committee of the University of Antioquia, Medellin (reference: 16-05-727) and the Consejo Superior de Investigaciones Científicas (CSIC) in Spain (reference: 094/2016) approved the protocols used in this study. All subjects gave written informed consent in accordance with the guidelines of the Declaration of Helsinki.
Overexpression and Purification of the Recombinant PFR1 Protein
The cloning, expression, and purification of the L. infantum PFR1 antigen were performed as described previously (Ledesma et al., 2017). The overexpression of the recombinant PFR1 protein was induced by the addition of 0.2 mM isopropyl-beta-D-thiogalactopyranoside (IPTG) for 3 h at 37°C. Protein was solubilized in a buffer containing 0.3 M NaCl and 50 mM Na2HPO4 and purified by Ni2+-NTA affinity chromatography. The purified protein was visualized via 10% SDS-PAGE followed by Coomassie blue staining. The protein concentration was measured using a Micro BCA Protein Assay Kit (Thermo Fisher Scientific). The purified PFR1 protein was tested by an E-Toxate reaction kit (Limulus amebocyte lysate [LAL], Sigma), which showed that the endotoxin levels were below the detection limit of the kit (0.1 endotoxin units/mL).
Isolation of Leishmania panamensis and Leishmania infantum Total Soluble Antigens
L. panamensis and L. infantum promastigotes were grown in modified RPMI 1640 medium supplemented with 20% FBS and gentamycin (50 μg/mL). Promastigotes were collected by centrifugation and washed with 1 × PBS at pH 7.2. The parasites were suspended at 109 parasites/mL in lysis buffer (50 mM Tris-HCl at pH 7.4, 50 mM NaCl, 0.005% NP-40, 1 mM PMSF, and 1 μg/mL leupeptin) and sonicated 3 times with pulses of 50–62 kHz for 40 s at time intervals of 20 s. The soluble protein extracts (SLpA and SLiA) were collected by centrifugation at 10,000 rpm for 20 min at 4°C. The protein concentration was determined using the Micro BCA Protein Assay Kit (Thermo Fisher Scientific), and the proteins were visualized by 10% SDS-PAGE followed by Coomassie blue staining (Gibco).
Cell Proliferation Assay
PBMCs (2 × 105 cells/well) from the subjects under study were split in 96-well flat-bottom plates containing 200 μL/well RPMI-20% iFBC medium in the presence of the recombinant PFR1 antigen (5 μg/mL), SLpA (10 μg/mL), SLiA (10 μg/mL), or 2 μg/mL concanavalin A (ConA) in triplicate. Polymyxin B (50 U/mL) was added to a parallel set of wells. The plates were incubated at 37°C in a CO2 atmosphere for 120 h. The cells stimulated with SLpA or SLiA were pulsed with BrdU (10 μM) and incubated for 20 h at 37°C. Cell proliferation was determined using a nonradioactive ELISA technique, according to the manufacturer's instructions (Cell Proliferation ELISA BiotrakTM System, version 2, GE Healthcare, AmershamTM, UK). The results were expressed as optical density (OD) values, and the stimulation index (SI) was calculated using the following formula:
The cells stimulated with rPFR1 were pulsed with methyl-3H] thymidine (0.5 μCi/well) and incubated for an additional 20 h at 37°C. The cells were immobilized in glass fiber filter mats using a FilterMate harvester (Perkin Elmer). 3H incorporation was measured in a Wallac 1450 MicroBeta counter device. The results were expressed as counts per minute (cpm), and the stimulation index (SI) was calculated using the following formula:
Monoclonal Antibodies for Cell Surface Staining
The following conjugated antibodies were included in the panel for cell surface staining: anti-CD3-Pacific Blue (clone UCHT1), anti-CD4-AlexaFluor700 (clone RPA-T4), anti-CD8-allophycocyanin-H7 (clone SK1), anti-CD27-FITC (clone M-T271), anti-CCR7-PE (clone 150503), anti-CD45RA-PerCP-Cy5.5 (clone HI100), anti-CD57-APC (clone NK-1), anti-2B4-FITC (clone 2-69), anti-TIM-3-PE-CF594 (clone 7D3), anti-CD160-AlexaFluor647 (clone BY55) (all from BD Pharmingen, San Diego, CA), and anti-PD-1-PE (clone J105; eBioscience, San Diego, CA). For the intracellular staining, the following conjugated antibodies were used: anti-CTLA-4-PE-Cy5 (clone BNI3), anti-IFN-γ-PE-Cy7 (clone B27), anti-granzyme B-PE-CF594 (clone GB11), anti-IL-2-APC (clone MQ1-17H12), anti-TNF-α-AlexaFluor488 (clone Mab11) (all from BD Pharmingen, San Diego, CA), and anti-perforin-PE (Clone B-D48; Abcam, Cambridge, U.K.). Live cells were isolated by using the Fixable Aqua Dead Cell Stain viability marker (LIVE/DEAD) (Invitrogen, Eugene, OR).
Detection of Intracellular Cytokines, Cytotoxic Molecules, and Inhibitory Receptors by Flow Cytometric Assays
PBMCs were cultured in RPMI 1640 supplemented with 2 mM L-glutamine, 10% iFBS, and 50 μg/mL gentamicin. PBMCs were cultured at 1 × 106 cells/mL with anti-CD28 (1 μg/mL) and anti-CD49d (1 μg/mL) antibodies (purified clones CD28.2 and 9F10, BD Pharmingen, San Diego, CA) in the presence of SLpA (1 μg/mL), in the absence of SLpA (considered the basal response) or in the presence of 10 μg/mL L. infantum recombinant PFR1 protein (with 30 μg/mL of polymyxin B). The cells were incubated for 14 h at 37°C in a humidified atmosphere with 5% CO2. For intracellular staining, cells were cultured in the presence of GolgiPlug and GolgiStop according to the manufacturer's instructions (BD Pharmingen, San Diego, CA). After stimulation, the cells were washed and incubated in PBS (containing 5% iFBS) for 10 min at RT. PBMCs were stained with a viability marker, namely, LIVE/DEAD Fixable Aqua (Invitrogen, Eugene, OR), for 20 min in the dark at RT. Prior to the addition of the surface staining antibodies, the cells were washed three times with PBS (containing 5% iFBS). For the memory and phenotypic characterizations, the cells were stained with anti-CD3, anti-CD4, anti-CD8, anti-CD45RA, anti-CCR7, anti-CD27, and anti-CD57 antibodies for 20 min in the dark at 4°C. To analyze inhibitory receptor expression, the cells were stained with anti-CD3, anti-CD4, anti-CD8, anti-PD-1, anti-2B4, anti-TIM-3, and anti-CD160 antibodies for 20 min in the dark at 4°C and washed with PBS (containing 5% iFBS). Then, the cells were fixed and permeabilized with Cytofix/Cytoperm (BD Biosciences) for 20 min at 4°C, washed following the manufacturer's instructions and stained with anti-CTLA-4 for 30 min in the dark at 4°C. To evaluate the intracellular production of cytokines and cytotoxic molecules, cells were stained with anti-CD3, anti-CD4, and anti-CD8 antibodies. After washing, the cells were fixed and permeabilized with Cytofix/Cytoperm (BD Biosciences). Intracellular staining was performed with anti-granzyme B, anti-IFN-γ, anti-IL-2, anti-TNF-α, and anti-perforin antibodies for 30 min at 4°C. Finally, after the last staining step, the cells were washed, suspended in 1 × PBS and analyzed in an LSRFortessa cytometer (BD Biosciences). At least 100,000 events were acquired, and analyses were performed using FlowJo 9.3.2 (TreeStar, Ashland, OR), Pestle 1.7 and SPICE 5.3 (National Institutes of Health, Bethesda, MD). Positivity for each marker was determined using fluorescence minus one (FMO) controls. Each antibody was titrated prior to the assays. Dead and doublet cells were excluded from the analysis.
Statistical Analysis
The statistical analysis was carried out using Prism version 6.0 (GraphPad Software, La Jolla, CA). Statistical significance was calculated using nonparametric tests, such as the Mann–Whitney U-test (pairwise comparisons) or the Kruskal–Wallis test with the Dunn correction to identify differences between groups of cellular subpopulations or between more than two groups of subjects (ANOVA was used for comparisons among > 2 groups). Differences were considered statistically significant for p < 0.05, and the symbols used are *p < 0.05, **p < 0.01, and ***p < 0.001. The box plot graphs were generated by GraphPad Prism version 6.0 software and represent all values (minimum to maximum). The boxes represent the 25–75th percentiles.
Results
Leishmanial Antigens and Lymphoproliferative Responses
The total soluble Leishmania proteins (SLpA and SLiA) and the recombinant PFR1 protein (rPFR1) used in the assays are shown in Supplementary Figure 1. A single and intensely stained band corresponding to the PFR1 antigen with an expected molecular mass of approximately 70 kDa was observed (lane 3). The purity was higher than 95%, as determined by a band densitometry analysis following Coomassie blue staining. PBMCs from patients with active leishmaniasis showed a proliferative response after stimulation with SLpA or SLiA, with proliferation indexes varying between 4.4 and 0.8 and between 4.3 and 0.8, respectively (Table 1). PBMCs from asymptomatic subjects with a positive Montenegro test showed proliferation indexes between 3.1 and 0.7 when stimulated with SLpA and between 1.5 and 0.1 when stimulated with SLiA (Table 1). The proliferation indexes of PBMCs from cured patients ranged within tighter intervals, between 1.3 and 0.1 and between 0.6 and 0.02 after stimulation with SLpA or SLiA, respectively (Table 1). A proliferative response was also detected after the stimulation of PBMCs with rPFR1. This response was stronger in cells from patients with active CL, with values ranging from 2.3 to 0.4, than in PBMCs from cured patients, with values between 0.8 and 0.02 (Table 1). Stimulation with rPFR1 did not lead to a detectable proliferative response in PBMCs from asymptomatic subjects. In general terms, and in spite of the limitation regarding to the wide range of proliferative response after antigen stimulation (SLpA SLiA or rPFR1), the obtained results allowed to detect which group of the subject responded with a higher and lower value of the proliferative index.
Phenotypic Characterization of Antigen-Specific Memory CD4+ and CD8+ T Cells
To evaluate the existence of a possible association between the distribution of antigen-specific memory T cells and the clinical status of CL patients, we analyzed the phenotypes of CD4+ and CD8+ T cells from PBMCs isolated from all recruited subjects except the HD (13 individuals). The expression of CD45RA, CCR7, CD27, and CD57 in PBMCs stimulated with either SLpA or rPFR1 was analyzed by flow cytometry after staining with conjugated antibodies.
As shown in Figure 1A, within the subset of SLpA-specific CD4+ T cells from either asymptomatic individuals with a positive Montenegro test or cured patients, the proportion of cells with a TNAIVE phenotype (CD45RA+CD27+CCR7+) was significantly higher than that of cells with a terminal effector memory phenotype (TEMRA cells, CD45RA+CD27−CCR7−) (p < 0.05). This pattern was also observed in SLpA-specific CD8+ T cells from both groups of subjects (Figure 1B), although the difference was not statistically significant. Patients with active leishmaniasis showed a higher percentage (statistically nonsignificant) of SLpA-specific CD8+ T cells with a TEMRA phenotype than of cells with a TNAIVE phenotype. Remarkably, the frequency of TEMRA CD8+ T cells was greater in patients with active CL than in cured patients (Figure 1B). In addition, the frequency of CD4+ and CD8+ effector memory T cells (TEM, CD45RA−CCR7−CD27−) was significantly higher than the frequency of CD4+ and CD8+ central memory T cells (TCM, CD45RA−CCR7+CD27+) in both asymptomatic and cured patients (p < 0.05 and p < 0.01, respectively; Figures 1A,B).
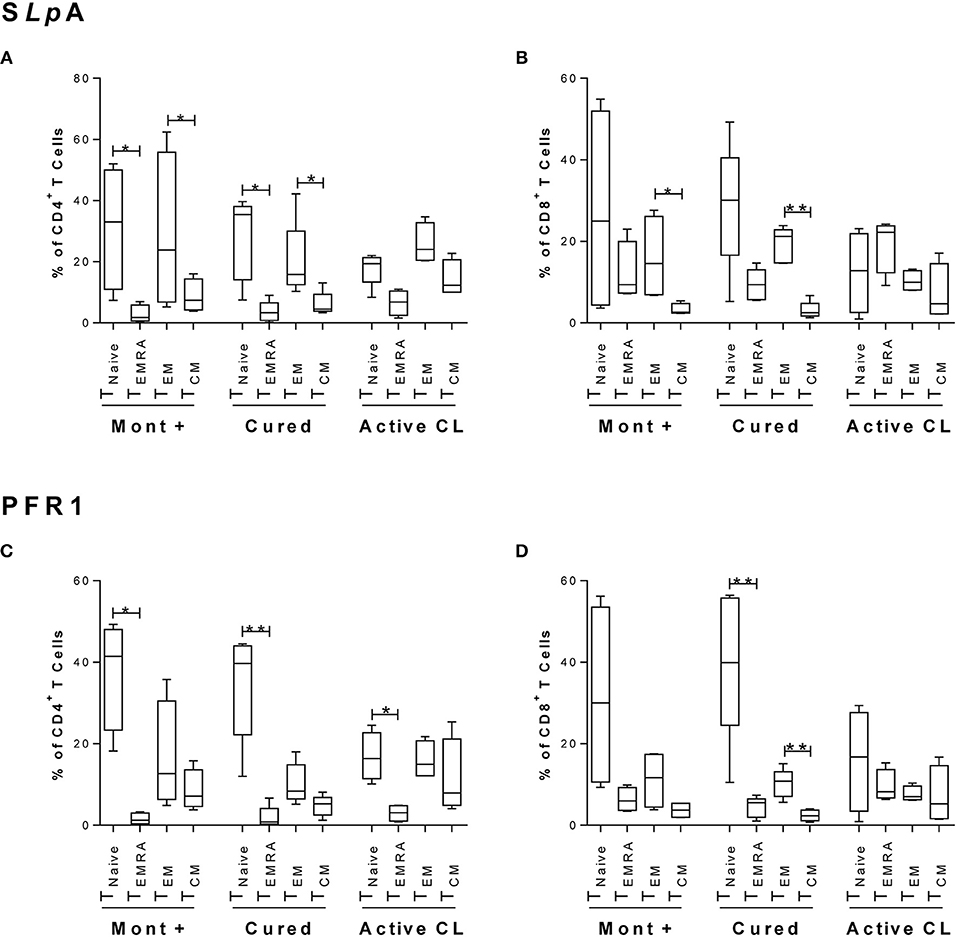
Figure 1. Immunophenotyping of antigen-specific CD4+ and CD8+ memory T cells. Phenotypic characterization of CD4+ (A,C) and CD8+ (B,D) T cells from asymptomatic subjects with a positive Montenegro skin test (Mont +, n = 4), patients cured of cutaneous leishmaniasis (Cured, n = 5) and patients with active cutaneous leishmaniasis (Active CL, n = 4). This profile was determined after stimulation with SLA from L. panamensis (A,B) or with the rPFR1 antigen (C,D). According to CD45RA, CD27, and CCR7 expression, the cells were grouped as TNAIVE (CD8+CD45RA+CCR7+), TEMRA (CD8+CD45RA+CCR7−), TEM (CD8+CD45RA−CCR7−), and TCM (CD8+CD45RA−CCR7+). The boxes (25th−75th percentiles) and whisker plots (using the Tukey's method) show the median frequency and range of the CD4+ and CD8+ T cells. Statistical analyses were carried out using the Mann–Whitney U-test. Statistically significant differences were indicated by *p < 0.05 and **p < 0.01.
Regarding the PFR1-specific CD4+ T cells, a similar phenotypic profile with a significantly higher percentage of TNAIVE cells than of TEMRA cells was observed in the three groups of subjects (p < 0.05 and p < 0.01; Figure 1C). Interestingly, in PFR1-specific CD8+ T cells, the difference between the proportions of TNAIVE and TEMRA cells was less pronounced in patients with active CL (Figure 1D) than in patients in the other two groups. Within this subset of CD8+ T cells, statistically significant differences between the proportions of TNAIVE and TEMRA cells were detected only in the group of cured patients (p < 0.01; Figure 1D). Furthermore, in this group of patients, a significantly higher frequency of CD8+ T cells with a TEM phenotype than of CD8+ T cells with a TCM phenotype was observed (p < 0.01; Figure 1D).
Since the expression of the CD57 marker has been associated with an irreversible senescence state (replicative senescence) in cells, which is more prevalent in effector memory T cells and especially in those with a highly differentiated phenotype (Xu and Larbi, 2017), CD57 expression was evaluated in effector memory T cells (TEM and TEMRA). The results indicate that the frequency of CD4+ and CD8+ T cells expressing CD57 tended to be higher (although not statistically significant) in TEMRA cells from patients with active CL than in TEMRA cells from either asymptomatic individuals with a positive Montenegro test or cured patients (Supplementary Figure 2). Remarkably, this difference was statistically significant within both subsets of SLpA- and PFR1-specific CD8+ T cells when the evaluation was made by considering the absolute number of CD57+ cells within the subset of TEMRA cells (p < 0.05; Figure 2). The CD4+ T cells exhibited a similar behavior, although the observed differences were not statistically significant.
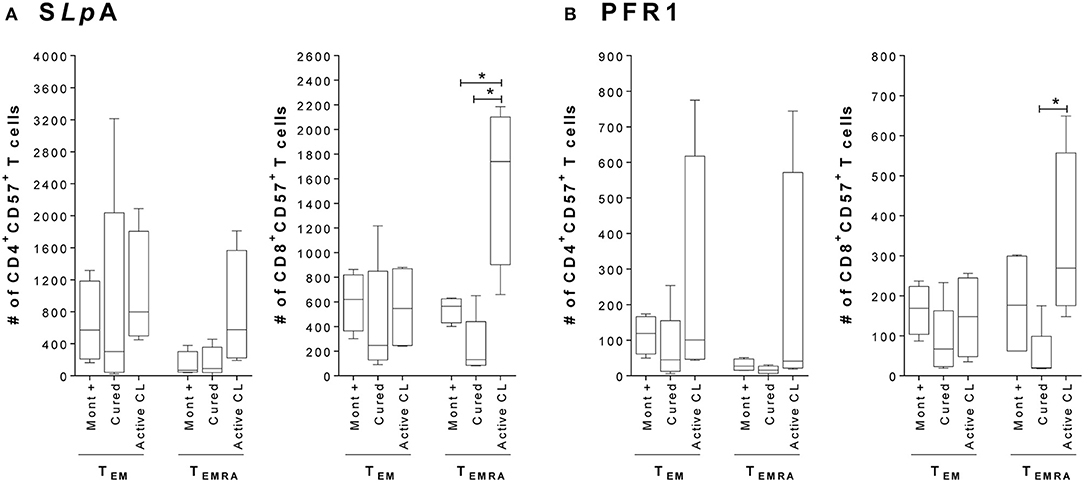
Figure 2. Expression of the senescence marker CD57 in CD4+ and CD8+ effector memory T cells. (A) Total number of SLpA-specific CD4+ and CD8+ T cells that expressed the marker CD57+ within the TEMRA and TEM effector memory subpopulations. (B) Total number of rPFR1-specific CD4+ and CD8+ T cells that expressed the marker CD57+ within the TEMRA and TEM effector memory subpopulations. Analyses were carried out in asymptomatic subjects with a positive Montenegro skin test (Mont +, n = 4), patients cured of cutaneous leishmaniasis (Cured, n = 5) and patients with active cutaneous leishmaniasis (Active CL, n = 4). The boxes (25th−75th percentiles) and whisker plots (using Tukey's method) show the median and range of the CD4+ and CD8+ T cells. Statistical analyses were carried out using the Mann–Whitney U-test. Statistically significant differences are indicated by *p < 0.05.
Functional Capacity of Circulating CD4+ and CD8+ T Cells in Response to Leishmania Antigens
To determine if there was a relationship between the pattern of functional responses and disease status, the functional responses of antigen-specific CD4+ and CD8+ T cells from asymptomatic subjects with a positive Montenegro skin test, patients cured of leishmaniasis and patients with active CL were evaluated. The production of intracellular cytokines (IL-2, IFN-γ, and TNF-α) and cytotoxic molecules (granzyme B and perforin) after stimulation with SLA from L. panamensis was determined. The results showed that the frequency of CD4+ T cells with multifunctional capacity tended to be greater in both asymptomatic individuals and patients with active CL than in cured patients (Figure 3), based on the greater proportions of antigen-specific CD4+ T cells performing four (1.3 and 3.6% vs. 0.9%, respectively) or three (10.5 and 15.8% vs. 6.5%, respectively) functions. Asymptomatic patients showed a higher frequency of bifunctional CD4+ T cells (40.7%) and of CD4+ T cells expressing both of the cytotoxic molecules, namely, granzyme B and perforin (Figure 3A). Remarkably, the evaluation of the multifunctional response in CD8+ T cells led to different results. The frequency of multifunctional CD8+ T cells was a greater although not statistically significant in cured patients than in either patients with active CL or asymptomatic patients. In fact, CD8+ T cells performing five functions (granzyme B+, IFN-γ+, IL-2+, perforin+, and TNF-α+) were detected only in the group of cured patients (Figure 3B). Similarly, higher frequencies of CD8+ T cells expressing four (3.3% vs. 1.7 and 0.8%) and three markers (18.5% vs. 6.7% and 7.5%) were observed in patients cured of CL than in either the asymptomatic Montenegro-positive individuals or those with active CL, respectively (Figure 3B). Furthermore, cured patients showed a greater frequency of CD8+ T cells expressing IFN-γ, perforin and granzyme B than did either asymptomatic Montenegro-positive individuals or patients with active CL (11.8 vs. 6.7 and 1.2%, respectively; Figure 3). In addition, while the subjects cured of leishmaniasis exhibited monofunctional cells that individually expressed all the analyzed molecules, CD8+ T cells from patients with active CL preferentially expressed granzyme B or IL-2 (Figure 3).
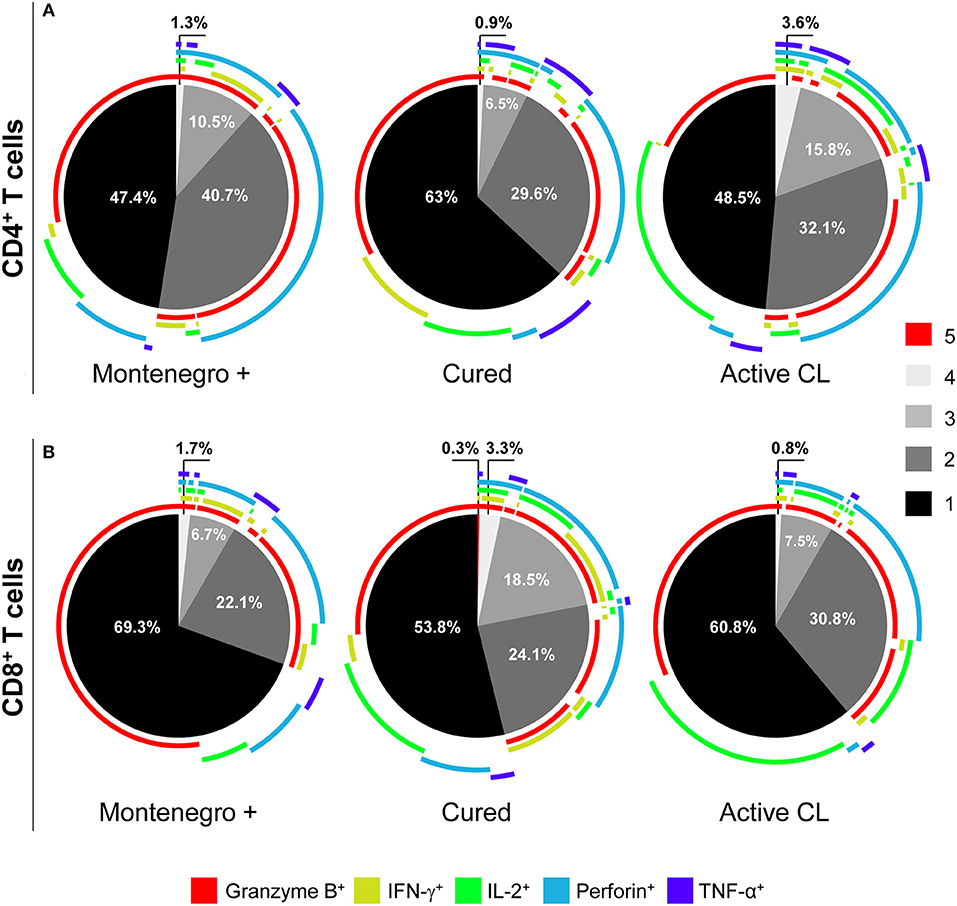
Figure 3. Multifunctional capacity of Leishmania-specific CD4+ and CD8+ T cells. Multifunctional activity of CD4+ (A) and CD8+ (B) T cells from asymptomatic subjects with a positive Montenegro skin test (Mont +, n = 4), patients cured of cutaneous leishmaniasis (Cured, n = 5) and patients with active cutaneous leishmaniasis (Active CL, n = 4). This profile was determined using a five-function assay to simultaneously measure the expression of IFN-γ, IL-2, TNF-α, granzyme B, and perforin after stimulation with SLA from L. panamensis. The color of each portion of the pie charts depicts the number of molecules produced by CD4+ and CD8+ T cells in response to L. panamensis antigens. The arcs of the pie charts represent the proportions of cells expressing each of the analyzed molecules. The percentage of unstimulated CD4+ and CD8+ T cells expressing these molecules (basal response) was subtracted from the value obtained following antigen stimulation.
As shown in Figure 4, patients with active CL had a higher proportion of CD4+ T cells that simultaneously produced the cytokines IFN-γ, TNF-α, and IL-2 than did either asymptomatic individuals or cured patients (8.2 vs. 0.8%; Figure 4A). In cured patients, the monofunctional CD4+ T cells mainly expressed TNF-α or IFN-γ (Figure 4A), while the frequency of CD8+ T cells expressing three (1.7% vs. 0.5 and 0.4%) or two cytokines (14.7% vs. 9.5 and 5.1%) was higher than that in either asymptomatic individuals or patients with active CL. Conversely, patients with active CL showed a greater frequency of monofunctional CD8+ T cells (94.6%) than did either asymptomatic or cured patients (90 and 83.6%, respectively; Figure 4B). Our results showed that the different groups of subjects under examination might be differentiated not only based on the frequency of multifunctional CD4+ or CD8+ T cells that produce several cytokines but also based on the patterns of the expressed cytokines. For example, compared with its production in either asymptomatic individuals or cured patients, IL-2 was mainly produced by monofunctional CD4+ and CD8+ T cell populations from patients with active CL (Figures 4A,B). Moreover, the frequency of CD8+ T cells expressing both of the cytotoxic molecules, namely, granzyme B and perforin, was significantly lower in cured patients than in either asymptomatic subjects or patients with active CL (11.8% vs. 25.5 and 31.3%, respectively; Figure 5).
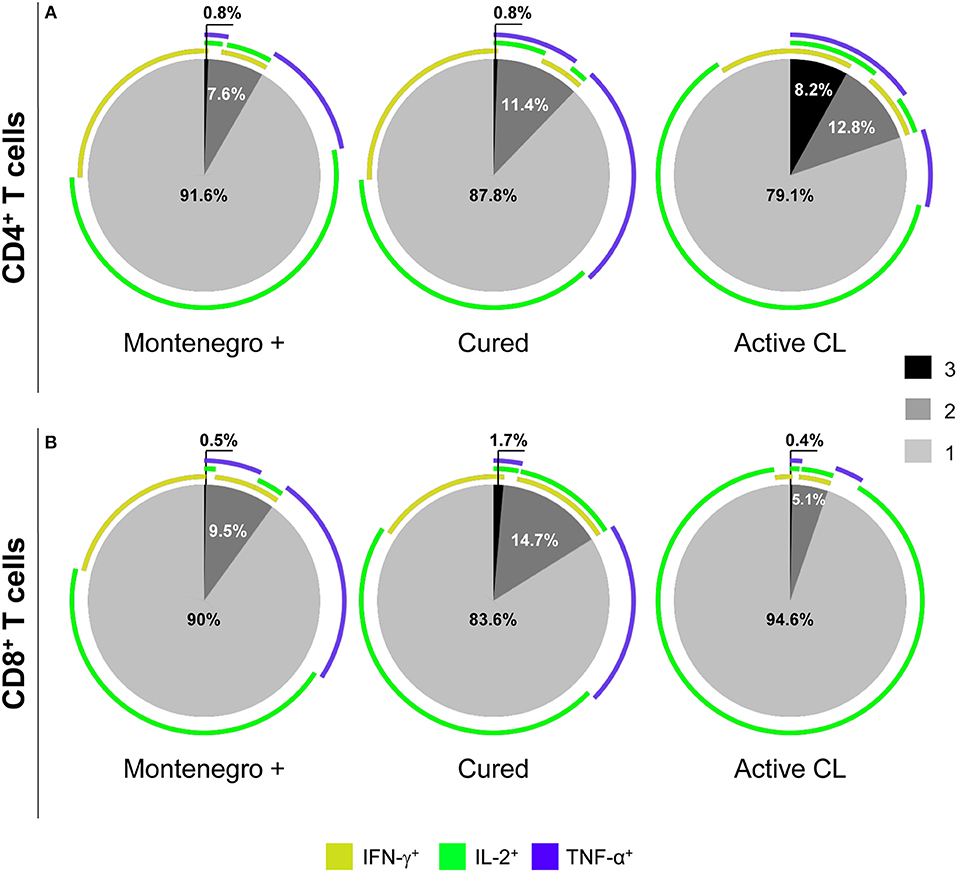
Figure 4. Functional capacity of Leishmania-specific CD4+ and CD8+ T cells. Cytokine production (IFN-γ, IL-2, and TNF-α) by CD4+ (A) and CD8+ (B) T cells from asymptomatic subjects with a positive Montenegro skin test (Mont +, n = 4), patients cured of cutaneous leishmaniasis (Cured, n = 5) and patients with active cutaneous leishmaniasis (Active CL, n = 4) in response to SLA from L. panamensis. The colors in the pie charts depict the presence of one, two, or three functions. The arcs of the pie charts represent the proportions of cells expressing each of the molecules under study.
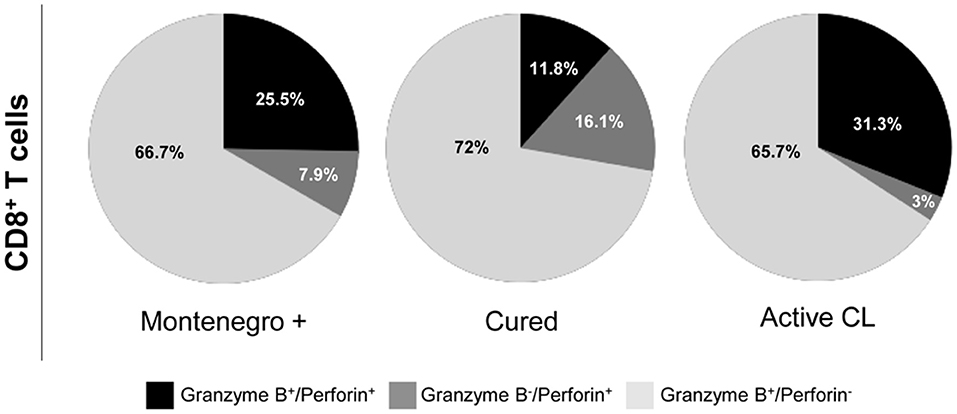
Figure 5. Cytotoxic profile of Leishmania-specific CD8+ T cells. Functional activity of CD8+ T cells determined by perforin and granzyme B expression after stimulation with SLA from L. panamensis in asymptomatic subjects with a positive Montenegro skin test (Mont +, n = 4), patients cured of cutaneous leishmaniasis (Cured, n = 5) and patients with active cutaneous leishmaniasis (Active CL, n = 4). The colors in the pie charts depict the proportion of cells expressing each of the analyzed molecules.
Expression of Inhibitory Receptors in Specific CD8+ T Cells
The next question to be evaluated was whether the functional response patterns of antigen-specific CD8+ T cells were associated with the expression or coexpression of inhibitory receptors. Consequently, the expression of PD-1, CTLA-4, 2B4, CD160, and TIM-3 was evaluated ex vivo in PBMCs from 10 HD, 4 asymptomatic subjects, 5 patients cured of cutaneous leishmaniasis and 4 patients with active CL. PBMCs were stimulated with SLpA and stained using conjugated antibodies, as described in section Materials and Methods. Patients with active CL showed significantly higher frequencies of CD8+ T cells expressing CD160 (p < 0.01), CTLA-4 (p < 0.05), PD-1 (p < 0.05), and TIM-3 (p < 0.01) than did HD (Figure 6A). The frequency of CD8+ T cells expressing CD160 was also significantly higher in patients with active CL than in cured patients (p < 0.05). The same pattern of differences between these two pairs of patient groups was found for the frequency of CD8+ T cells that expressed 2B4, although the differences were not statistically significant. Further examination of the differences among samples from the different subjects revealed that the frequency of CD8+ T cells expressing CTLA-4 (p < 0.05 and p < 0.01) and TIM-3 (p < 0.01) was higher in both asymptomatic individuals and cured patients than in HD (Figure 6A). An evaluation of the concurrent expression of inhibitory receptors showed a significantly higher frequency of CD8+ T cells that coexpressed two, three, or four inhibitory receptors in patients with active CL than in HD (p < 0.01; Figure 6B). There was a higher frequency of CD8+ T cells that coexpressed four molecules in asymptomatic individuals than there was in HD (p < 0.01; Figure 6B).
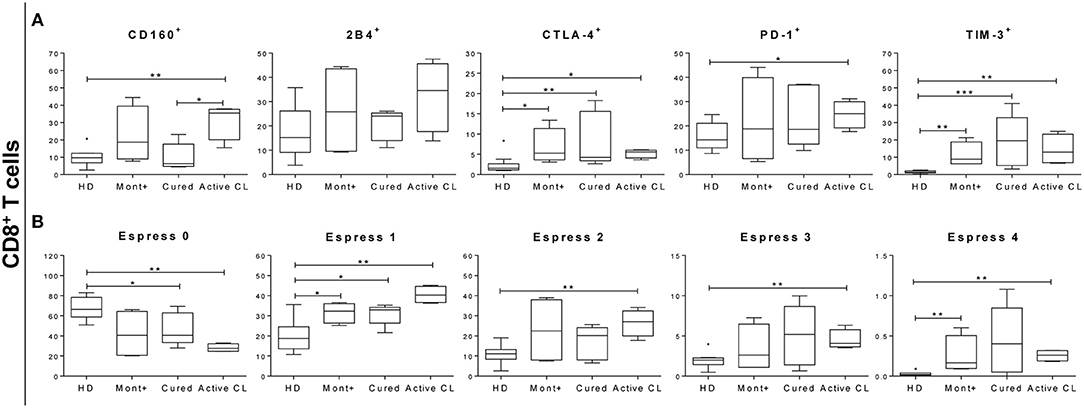
Figure 6. Percentage of CD8+ T cells expressing or coexpressing inhibitory receptors. (A) Frequency of CD8+ T cells expressing 2B4, CD160, CTLA-4, PD-1, and TIM-3 in asymptomatic subjects with a positive Montenegro skin test (Mont +, n = 4), patients cured of cutaneous leishmaniasis (Cured, n = 5), patients with active cutaneous leishmaniasis (Active CL, n = 4) and healthy donors (HD, n = 10). (B) Coexpression of 2B4, CD160, CTLA-4, PD-1, and TIM-3 by CD8+ T cells. The boxes (25th−75th percentiles) and whisker plots (using Tukey's method) show the median and range of the frequency of inhibitory receptors on T cells. Statistical analyses were carried out using the Mann–Whitney U-test. Statistically significant differences are indicated by *p < 0.05, **p < 0.01, and ***p < 0.001.
Discussion
Understanding the immunological mechanisms that underlie disease outcomes after the infection of a subject with Leishmania (Viannia) is essential given that there are asymptomatic patients, parasite persistence after treatment (de Oliveira Camera et al., 2006; Figueroa et al., 2009) and disease reactivation (Saravia et al., 1990). It has been reported that the clinical cure of leishmaniasis is mainly dependent on T cell populations that secrete Th1-related cytokines (Coutinho et al., 1998; Da-Cruz et al., 2005). Most knowledge obtained from the development of vaccines against CL comes from experimental infections with Leishmania major in murine models and cannot be fully extended to the subgenus Viannia. Likewise, clinical studies indicate that the human response to infection with Leishmania (Viannia) parasites differs from the response to infection caused by other members of the Leishmania subgenus (Laskay et al., 1991; Melby et al., 1994; Silveira et al., 2009). In addition, an evaluation of the treatment efficacy of several drugs against CL showed that healing is a dynamic process that involves changes in the frequency and quality of antigen-specific T cells (Da-Cruz et al., 1994; Lakhal-Naouar et al., 2015). These facts reinforce the importance of evaluating the quality of the immune response triggered by infection with Leishmania (Viannia) species (De Luca and Macedo, 2016).
In the present manuscript, the phenotype and functionality of CD4+ and CD8+ T cells from CL patients, cured patients and asymptomatic individuals with a positive Montenegro skin test were characterized. In addition, the existence of a possible CD8+ T cell exhaustion process associated with the evolution of Leishmania infection was evaluated. The results showed that cells from these patients exhibited lymphoproliferative capacity following stimulation with both SLpA and SLiA and, to a lesser extent, with the rPFR1 protein. In active CL patients, a similar proliferative response was observed in T cells stimulated with either SLpA or SLiA. In cured and asymptomatic patients, the greatest proliferative response was observed following cell stimulation with SLpA. The cell proliferation assay with soluble antigens of L. infantum showed a proliferative response in all subjects, although with lower values compared to those obtained with SLpA. The proliferative capacity of the subjects against the antigens of L. infantum, was performed as the rPFR1 protein came from L. infantum. These data are expected since all patients were infected with L. panamensis, the most prevalent infecting strain in the area where the patients under study live (Corredor et al., 1990; Ovalle et al., 2006; Ramírez et al., 2016). Consequently, subsequent cellular assays were performed using SLpA as the stimulation agent.
The analysis of the phenotype of the stimulated CD4+ and CD8+ T cells from asymptomatic individuals and cured patients showed that TNAIVE cells, which represented 30–40% of the total T cell population, were the subset with the highest frequency, mainly following stimulation with rPFR1. The effector memory (TEM) population represented ~10% of the total T cells, which was higher than the frequency of central memory T cells (TCM). This pattern was observed in T cells stimulated with both the soluble L. panamensis antigen (SLpA) and the L. infantum rPFR1 antigen. These central and effector memory T cell profiles have been previously reported in other parasitic and viral human infections. Thus, asymptomatic and cardiac Chagas disease patients have a significantly higher proportion of TEM cells than TCM cells in the population of CD4+ and CD8+ T cells (Fiuza et al., 2009) both ex vivo and after stimulation with T. cruzi antigens. A similar profile was also reported in memory HIV-specific CD8+ T cells, for which TEM cells comprised 71.8% of the population and TCM cells comprised 4.1% (Champagne et al., 2001). It has been reported that after resolution of Leishmania infection, a small number of persistent parasites can act as source of continuous antigen stimulation to host immune cells (Sacks and Noben-Trauth, 2002), which leads to the maintenance of effector memory T cells (Aebischer et al., 1993; Mendonça et al., 2004; Okwor and Uzonna, 2008). These effector memory T cells can be maintained for prolonged durations, exhibiting increased resistance to apoptosis and playing a key role in protection against reinfection (Sallusto et al., 2004). Correspondingly, it has been reported that both central and effector memory T cells mediate long-term resistance to leishmaniasis (Zaph et al., 2004). The T cells from the studied patients with active CL showed a higher proportion of effector memory T cells (TEM for CD4+ T cells and TEMRA for CD8+ T cells) than TNAIVE cells after stimulation with SLpA. These profiles are consistent with a shift in the activation phenotype associated with the persistence of the lesions. However, the patients with active CL had similar frequencies of CD4+ TNAIVE and TEM cells after stimulation with rPFR1. The rPFR1-specific CD8+ T cells presented a slightly higher proportion of TNAIVE cells than of TEMRA or TEM cells. The higher frequency of effector cells detected in patients with active CL than in either asymptomatic or cured patients could be caused by the existence of a high parasite load and the consequent activation of an antigen-specific immune response. In this context, it has been reported that chronic Chagas disease patients in the asymptomatic stage have a higher proportion of TNAIVE antigen-specific CD8+ T cells, while chronic Chagas patients with cardiac symptomatology present a greater frequency of effector memory cells (TEM and TEMRA) (Egui et al., 2015). The T cell-mediated immune response has also been analyzed in the skin lesions of CL patients by other authors. Thus, in CL patients infected with Leishmania braziliensis, a higher percentage of CD4+ and CD8+ TEM cells than others T cell subsets (TNAIVE and TCM cells) was found at the lesion level than in the blood (de Oliveira Mendes-Aguiar et al., 2016). The higher percentage of these cells together to activated cytotoxic cells was taken as an indication of the contribution of TEM to immune-mediated tissue damage. It is known that the proliferative capacity of effector T cells expressing CD57 is severely and irreversibly compromised. Interestingly, the number of antigen-specific CD8+ CD57+ TEMRA cells from patients with active CL was statistically significantly higher than that observed in cured patients. Likewise, a significantly higher number of CD4+ TEMRA cells that expressed the CD57 molecule was found in patients with active CL than in cured patients and asymptomatic subjects. These TEMRA cells, which have a terminally differentiated phenotype, are very susceptible to apoptosis and produce a large amount of cytotoxic molecules (Khamesipour et al., 2012). The expression of CD57 has been strongly correlated with the simultaneous production of granzyme and perforin (Chattopadhyay et al., 2009). This phenotypic pattern is consistent with the cytotoxic profile observed in antigen-specific CD8+ T cells from patients with active CL. These results suggest that persistent exposure to the parasite might push the effector cells toward a terminally differentiated and senescent cell phenotype. In active leishmaniasis, the prolonged parasite presence would lead to persistent cytotoxic activity against intracellular protozoa, which could lead to a higher prevalence of senescent effector CD8+ T cells. The results show that CD4+ T cells exhibit a similar behavior, although these differences are not statistically significant. This fact may be related to the CD8+ T cells, as they are the cells endowed with cytotoxic activity, which is the main characteristic of CD8+ T cells.
CD8+ T cells from cured patients showed a higher multifunctional capacity than did those from patients with active CL. A multifunctional capacity of antigen-specific CD8+ T cells, which was only observed in cured patients, was indicated by the ability of the cells to simultaneously perform the five examined functions (IFN-γ+, TNF-α+, IL-2+, granzyme B+, and perforin+). In addition, cured patients showed a higher proportion of CD8+ T cells expressing three or four of these cytokines and cytotoxic molecules. Similarly, cured patients, in particular, showed a higher frequency of CD8+ T cells expressing IFN-γ+ and TNF-α+ than did patients with active CL, suggesting a relevant role of these cytokines in infection control. In murine models of leishmaniasis, CD8+ T cells have been shown to exert a curative role, which has been attributed to the production of Th1-related cytokines such as IFN-γ (Ruiz and Becker, 2007; Jayakumar et al., 2011). Moreover, IFN-γ-deficient mice were more susceptible to Leishmania infection, with larger lesions and higher parasite burdens than their wild-type counterparts (Pinheiro and Rossi-Bergmann, 2007). Additionally, it has been reported that other mediators produced by CD8+ T cells (such as perforin, granzymes, and chemokines) contribute to host defense. Conversely, an exacerbation of the CD8+ T cell immune response and the high production of cytotoxic molecules has been associated with tissue damage (Santos Cda et al., 2013). In fact, it has been reported that the CD8+ T cell immune response is involved in both the resolution of disease and the pathology caused by Leishmania (Viannia) infection in humans, highlighting the functional heterogeneity of these cells (Coutinho et al., 1998; Bangham, 2009). Interestingly, herein, it was shown that only cured patients showed a Th1-type cytotoxic profile, as indicated by the simultaneous expression of cytotoxic molecules and IFN-γ (CD8+ T cells, IFN-γ+granzyme B+perforin+). It is well-documented that resistance to leishmaniasis is related to the development and production of Th1-type proinflammatory cytokines, which leads to the activation of macrophages and to parasite killing (Sacks and Noben-Trauth, 2002; Von Stebut et al., 2003). While cytotoxic activity induces target cell death, Th1 cytokines (such as IFN-γ and TNF-α) are involved in the development of an inflammatory response that modulates the activity of macrophages and dendritic cells. However, when these pathways are not properly regulated, inflammatory disorders and tissue damage could develop (Ribeiro-de-Jesus et al., 1998; Follador et al., 2002; Arias et al., 2014). At the lesion level, there is elevated expression of granzyme B, perforin and CD107a (Novais et al., 2013; Cardoso et al., 2015), which has been positively correlated with lesion size (Santos Cda et al., 2013). Correspondingly, CD8+ T cells from patients with active CL presented a higher expression level of cytotoxic molecules (granzyme B+perforin+) than did those from cured patients or asymptomatic subjects.
In the context of CD4+ T cells, different functional profiles of the immune response were detected among the different groups of studied patients. Thus, a higher frequency of CD4+ T cells with multifunctional activity was detected in patients with active CL than in cured patients. However, cured patients had more monofunctional cells expressing cytokines related to the Th1 immune response, such as IFN-γ and TNF-α. These results are consistent with those of studies carried out in CL patients, which showed that after treatment, there is a reduction in both the frequency of multifunctional CD4+ T cells and the amount of cytokines secreted by these cells (Lakhal-Naouar et al., 2015). In fact, the depletion of effector CD4+ T cells in murine models did not appear to influence resistance to infection, while the depletion of CD8+ T effector cells reversed the protective effect, which led to a Th2 cytokine environment related to infection susceptibility (Jayakumar et al., 2011). Although little is known regarding the role of the cytotoxic activity of CD4+ cells in Leishmania infection, it has been reported that a high frequency of CD4+ T cell subsets producing granzyme B is found in individuals who previously had contact with L. major (Naouar et al., 2014). Consistently, in the present study, the production of cytotoxic molecules by CD4+ T cells was mainly detected in asymptomatic subjects. However, further work is needed to determine the potential roles of these cells and to understand the implications for protection and damage.
It has been reported that the healing process of CL lesions at the end of therapy is associated with an increase in the proportion of L. braziliensis-reactive-CD8+ T cells and a decline in CD4+-specific-reactive T cells (Da-Cruz et al., 2002). Consistent with these findings, the results of this study show a higher proportion of multifunctional L. panamensis-specific CD8+ T cells in treated cured patients than in asymptomatic subjects and active CL patients. However, a lower frequency of multifunctional CD4+ T cells was found in cured patients than in asymptomatic subjects and active CL patients.
In chronic diseases, the persistence of pathogen-derived antigens induces an increase in the expression of inhibitory receptors and a gradual loss of the response capacity of antigen-specific T cells. This process, known as the exhaustion process, has been mainly described in CD8+ T cells (Kahan et al., 2015). Very little is known about the exhaustion process in infections caused by Leishmania (Viannia) subgenus species. In the context of Chagas disease, this process has been well-documented in CD8+ T cells, with a higher level of dysfunction associated with a severe stage of the disease (Lasso et al., 2015). It was also recently reported that anti-Trypanosoma cruzi treatment partially reverses the exhaustion process in CD8+ T cells, thus improving antigen-specific functionality (Mateus et al., 2017). The results obtained in the present study showed higher expression and coexpression of inhibitory receptors in CD8+ T cells from patients with L. panamensis infection than in HD. Patients with active CL showed higher expression of CD160 and PD-1 than did cured patients and asymptomatic subjects. A significantly higher coexpression of inhibitory receptors by CD8+ T cells was only observed when patients with active CL were compared with HD. The relatively lower level of inhibitory receptor coexpression in cured patients than in patients with active CL, which was associated with the high multifunctional capacity of the CD8+ T cells of cured patients, suggests the critical importance of the functional response of CD8+ T cells in the healing process. Even the impaired or dysfunctional CD8+ T cell response induced by the exhaustion process and/or the senescent cell phenotype could contribute to the maintenance of an active lesion. Thus, in experimental model of CL, mice lacking PD-L1 were markedly more resistant to infection, and the lesions they developed were smaller (Liang et al., 2006). Furthermore, it has been reported in diffuse cutaneous leishmaniasis that an increase in PD-L1 expression by monocytes is a possible mechanism used by the parasites to evade the immune response (Barroso et al., 2018). In patients with visceral leishmaniasis, elevated expression of the CTLA-4 and PD-1 inhibitory receptors has been reported (Esch et al., 2013; Gautam et al., 2014; Chiku et al., 2016). Interestingly, the expression of such markers decreased in patients with Leishmania infection after treatment (Gautam et al., 2014). In this context, the high production of IFN-γ and TNF-α by CD8+ T cells and the greater proportion of multifunctional CD8+ T cells observed in patients cured of CL could be associated with the lower expression of PD-1 found in these patients, which might be related to a partial reversion of the exhaustion process induced by treatment. Consistent with that theory, in experimental models of visceral leishmaniasis, it has been reported that the blockade of PD-1 reduces the parasite burden, restores the T cell proliferation capacity and increases the production of IFN-γ and TNF-α (Mou et al., 2013; Chiku et al., 2016).
The findings presented in this work improve our understanding of the poorly elucidated exhaustion process that occurs in the context of L. panamensis infection. In addition, the data provide evidence that antigen-specific immune response markers can be correlated with the clinical status of human patients with CL caused by L. panamensis and might consequently be a useful tool for facilitating the clinical follow-up of patients with CL.
Author Contributions
MCT carried out the conceptualization. MCT and MCL designed the study. DL, AM, AE, EP-A, and IG obtained the samples and materials. AE, DL, and EP-A performed the experiments. AE, EP-A, DL, and MCL analyzed the results. AE and MCL performed the data visualization. AE, EP-A, MCL, and MCT discussed the results. JI, MCL, MCT, and IV acquired funding. MCL and SR supervised the study. DL, AE, and EP-A wrote the original draft. MCT, MCL, and JI wrote and edited the final version of the manuscript. All authors read and approved the final manuscript.
Funding
This work was supported by grants RTC-2016-50005-1, SAF2016-80998-R, and SAF2016-81003-R from the Programa Estatal I+D+i (MINECO) and by the Network of Tropical Diseases Research RICET (RD16/0027/0005) funded by ISCIII and FEDER.
Conflict of Interest Statement
The authors declare that the research was conducted in the absence of any commercial or financial relationships that could be construed as a potential conflict of interest.
Acknowledgments
We thank the patients and healthy volunteers who participated in this study. We are very grateful to A. López-Barajas from IPBLN-CSIC for her technical assistance. DL was supported by an FPU predoctoral fellowship (FPU-AP2012-1662) from the Spanish Ministry of Education, Culture, and Sport, and EP-A was supported by a predoctoral fellowship from FUNCCET.
Supplementary Material
The Supplementary Material for this article can be found online at: https://www.frontiersin.org/articles/10.3389/fcimb.2018.00393/full#supplementary-material
References
Aebischer, T., Moody, S. F., and Handman, E. (1993). Persistence of virulent Leishmania major in murine cutaneous leishmaniasis: a possible hazard for the host. Infect. Immun. 61, 220–226.
Alvar, J., Vélez, I. D., Bern, C., Herrero, M., Desjeux, P., Cano, J., et al. (2012). Leishmaniasis worldwide and global estimates of its incidence. PLoS ONE 7:e35671. doi: 10.1371/journal.pone.0035671
Arias, M. A., Jiménez de Bagües, M. P., Aguiló, N., Menao, S., Hervás-Stubbs, S., de Martino, A., et al. (2014). Elucidating sources and roles of granzymes A and B during bacterial infection and sepsis. Cell Rep. 8, 420–429. doi: 10.1016/j.celrep.2014.06.012
Bangham, C. R. (2009). CTL quality and the control of human retroviral infections. Eur. J. Immunol. 39, 1700–1712. doi: 10.1002/eji.200939451
Barroso, D. H., Falcão, S. A. C., da Motta, J. O. C., Sevilha Dos Santos, L., Takano, G. H. S., Gomes, C. M., et al. (2018). PD-L1 may mediate T-cell exhaustion in a case of early diffuse leishmaniasis caused by Leishmania (L.) amazonensis. Front. Immunol. 9:1021. doi: 10.3389/fimmu.2018.01021
Cachon, J., Cachon, M., Cosson, M., and Cosson, J. (1988). The paraflagellar rod: a structure in search of a function. Biol. Cell 63, 169–181. doi: 10.1016/0248-4900(88)90056-1
Cardoso, T. M., Machado, Á., Costa, D. L., Carvalho, L. P., Queiroz, A., Machado, P., et al. (2015). Protective and pathological functions of CD8+ T cells in Leishmania braziliensis infection. Infect. Immun. 83, 898–906. doi: 10.1128/IAI.02404-14
Castilho, T. M., Goldsmith-Pestana, K., Lozano, C., Valderrama, L., Saravia, N. G., and McMahon-Pratt, D. (2010). Murine model of chronic L. (Viannia) panamensis infection: role of IL-13 in disease. Eur. J. Immunol. 40, 2816–2829. doi: 10.1002/eji.201040384
Champagne, P., Ogg, G. S., King, A. S., Knabenhans, C., Ellefsen, K., Nobile, M., et al. (2001). Skewed maturation of memory HIV-specific CD8 T lymphocytes. Nature 410, 106–111. doi: 10.1038/35065118
Chattopadhyay, P. K., Betts, M. R., Price, D. A., Gostick, E., Horton, H., Roederer, M., et al. (2009). The cytolytic enzymes granyzme A, granzyme B, and perforin: expression patterns, cell distribution, and their relationship to cell maturity and bright CD57 expression. J. Leukoc. Biol. 85, 88–97. doi: 10.1189/jlb.0208107
Chiku, V. M., Silva, K. L., de Almeida, B. F., Venturin, G. L., Leal, A. A., de Martini, C. C., et al. (2016). PD-1 function in apoptosis of T lymphocytes in canine visceral leishmaniasis. Immunobiology 221, 879–888. doi: 10.1016/j.imbio.2016.03.007
Corredor, A., Kreutzer, R. D., Tesh, R. B., Boshell, J., Palau, M. T., Caceres, E., et al. (1990). Distribution and etiology of leishmaniasis in Colombia. Am. J. Trop. Med. Hyg. 42, 206–214.
Coutinho, S. G., Da-Cruz, A. M., Bertho, A. L., Santiago, M. A., and De-Luca, P. (1998). Immunologic patterns associated with cure in human American cutaneous leishmaniasis. Braz. J. Med. Biol. Res. 31, 139–142.
da Silva Santos, C., and Brodskyn, C. I. (2014). The role of CD4 and CD8 T cells in human cutaneous Leishmaniasis. Front. Public Health 2:165. doi: 10.3389/fpubh.2014.00165
Da-Cruz, A. M., Bertho, A. L., Oliveira-Neto, M. P., and Coutinho, S. G. (2005). Flow cytometric analysis of cellular infiltrate from American tegumentary leishmaniasis lesions. Br. J. Dermatol. 153, 537–543. doi: 10.1111/j.1365-2133.2005.06647.x
Da-Cruz, A. M., Bittar, R., Mattos, M., Oliveira-Neto, M. P., Nogueira, R., Pinho-Ribeiro, V., et al. (2002). T-cell-mediated immune responses in patients with cutaneous or mucosal leishmaniasis: long-term evaluation after therapy. Clin. Diagn. Lab. Immunol. 9, 251–256. doi: 10.1128/CDLI.9.2.251-256.2002
Da-Cruz, A. M., Conceição-Silva, F., Bertho, A. L., and Coutinho, S. G. (1994). Leishmania-reactive CD4+ and CD8+ T cells associated with cure of human cutaneous leishmaniasis. Infect. Immun. 62, 2614–2618.
De Luca, P. M., and Macedo, A. B. (2016). Cutaneous Leishmaniasis vaccination: a matter of quality. Front. Immunol. 7:151. doi: 10.3389/fimmu.2016.00151
de Oliveira Camera, P., Junger, J., do Espírito Santo Silva Pires, F., Mattos, M., Oliveira-Neto, M. P., Fernandes, O., et al. (2006). Haematogenous dissemination of Leishmania (Viannia) braziliensis in human American tegumentary leishmaniasis. Trans. R. Soc. Trop. Med. Hyg. 100, 1112–1117. doi: 10.1016/j.trstmh.2006.02.014
de Oliveira Mendes-Aguiar, C., Vieira-Gonçalves, R., Guimarães, L. H., de Oliveira-Neto, M. P., Carvalho, E. M., and Da-Cruz, A. M. (2016). Effector memory CD4(+) T cells differentially express activation associated molecules depending on the duration of American cutaneous leishmaniasis lesions. Clin. Exp. Immunol. 185, 202–209. doi: 10.1111/cei.12798
de Vries, H. J., Reedijk, S. H., and Schallig, H. D. (2015). Cutaneous leishmaniasis: recent developments in diagnosis and management. Am. J. Clin. Dermatol. 16, 99–109. doi: 10.1007/s40257-015-0114-z
Egui, A., Thomas, M. C., Carrilero, B., Segovia, M., Alonso, C., Marañón, C., et al. (2015). Differential phenotypic and functional profiles of TcCA-2 -specific cytotoxic CD8+ T cells in the asymptomatic versus cardiac phase in Chagasic patients. PLoS ONE 10:e0122115. doi: 10.1371/journal.pone.0122115
Egui, A., Thomas, M. C., Morell, M., Marañón, C., Carrilero, B., Segovia, M., et al. (2012). Trypanosoma cruzi paraflagellar rod proteins 2 and 3 contain immunodominant CD8(+) T-cell epitopes that are recognized by cytotoxic T cells from Chagas disease patients. Mol. Immunol. 52, 289–298. doi: 10.1016/j.molimm.2012.05.021
Esch, K. J., Juelsgaard, R., Martinez, P. A., Jones, D. E., and Petersen, C. A. (2013). Programmed death 1-mediated T cell exhaustion during visceral leishmaniasis impairs phagocyte function. J. Immunol. 191, 5542–5550. doi: 10.4049/jimmunol.1301810
Faria, D. R., Souza, P. E., Durães, F. V., Carvalho, E. M., Gollob, K. J., Machado, P. R., et al. (2009). Recruitment of CD8(+) T cells expressing granzyme A is associated with lesion progression in human cutaneous leishmaniasis. Parasite Immunol. 31, 432–439. doi: 10.1111/j.1365-3024.2009.01125.x
Figueroa, R. A., Lozano, L. E., Romero, I. C., Cardona, M. T., Prager, M., Pacheco, R., et al. (2009). Detection of Leishmania in unaffected mucosal tissues of patients with cutaneous leishmaniasis caused by Leishmania (Viannia) species. J. Infect. Dis. 200, 638–646. doi: 10.1086/600109
Fiuza, J. A., Fujiwara, R. T., Gomes, J. A., Rocha, M. O., Chaves, A. T., de Araújo, F. F., et al. (2009). Profile of central and effector memory T cells in the progression of chronic human chagas disease. PLoS Negl. Trop. Dis. 3:e512. doi: 10.1371/journal.pntd.0000512
Follador, I., Araújo, C., Bacellar, O., Araújo, C. B., Carvalho, L. P., Almeida, R. P., et al. (2002). Epidemiologic and immunologic findings for the subclinical form of Leishmania braziliensis infection. Clin. Infect. Dis. 34, E54–E58. doi: 10.1086/340261
Gautam, S., Kumar, R., Singh, N., Singh, A. K., Rai, M., Sacks, D., et al. (2014). CD8 T cell exhaustion in human visceral leishmaniasis. J. Infect. Dis. 209, 290–299. doi: 10.1093/infdis/jit401
Gigley, J. P., Bhadra, R., Moretto, M. M., and Khan, I. A. (2012). T cell exhaustion in protozoan disease. Trends Parasitol. 28, 377–384. doi: 10.1016/j.pt.2012.07.001
Glennie, N. D., and Scott, P. (2016). Memory T cells in cutaneous leishmaniasis. Cell Immunol. 309, 50–54. doi: 10.1016/j.cellimm.2016.07.010
Hernández-Ruiz, J., Salaiza-Suazo, N., Carrada, G., Escoto, S., Ruiz-Remigio, A., Rosenstein, Y., et al. (2010). CD8 cells of patients with diffuse cutaneous leishmaniasis display functional exhaustion: the latter is reversed, in vitro, by TLR2 agonists. PLoS Negl. Trop. Dis. 4:e871. doi: 10.1371/journal.pntd.0000871
Jayakumar, A., Castilho, T. M., Park, E., Goldsmith-Pestana, K., Blackwell, J. M., and McMahon-Pratt, D. (2011). TLR1/2 activation during heterologous prime-boost vaccination (DNA-MVA) enhances CD8+ T Cell responses providing protection against Leishmania (Viannia). PLoS Negl. Trop. Dis. 5:e1204. doi: 10.1371/journal.pntd.0001204
Joshi, T., Rodriguez, S., Perovic, V., Cockburn, I. A., and Stäger, S. (2009). B7-H1 blockade increases survival of dysfunctional CD8(+) T cells and confers protection against Leishmania donovani infections. PLoS Pathog. 5:e1000431. doi: 10.1371/journal.ppat.1000431
Kahan, S. M., Wherry, E. J., and Zajac, A. J. (2015). T cell exhaustion during persistent viral infections. Virology 479–480, 180–193. doi: 10.1016/j.virol.2014.12.033
Kaye, P., and Scott, P. (2011). Leishmaniasis: complexity at the host-pathogen interface. Nat. Rev. Microbiol. 9, 604–615. doi: 10.1038/nrmicro2608
Keshavarz Valian, H., Nateghi Rostami, M., Tasbihi, M., Miramin Mohammadi, A., Eskandari, S. E., Sarrafnejad, A., et al. (2013). CCR7+ central and CCR7- effector memory CD4+ T cells in human cutaneous leishmaniasis. J. Clin. Immunol. 33, 220–234. doi: 10.1007/s10875-012-9788-7
Khamesipour, A., Nateghi Rostami, M., Tasbihi, M., Miramin Mohammadi, A., Shahrestani, T., Sarrafnejad, A., et al. (2012). Phenotyping of circulating CD8(+) T cell subsets in human cutaneous leishmaniasis. Microbes Infect. 14, 702–711. doi: 10.1016/j.micinf.2012.02.006
Kip, A. E., Balasegaram, M., Beijnen, J. H., Schellens, J. H., de Vries, P. J., and Dorlo, T. P. (2015). Systematic review of biomarkers to monitor therapeutic response in leishmaniasis. Antimicrob. Agents Chemother. 59, 1–14. doi: 10.1128/AAC.04298-14
Lakhal-Naouar, I., Slike, B. M., Aronson, N. E., and Marovich, M. A. (2015). The immunology of a healing response in cutaneous Leishmaniasis treated with localized heat or systemic antimonial therapy. PLoS Negl. Trop. Dis. 9:e0004178. doi: 10.1371/journal.pntd.0004178
Laskay, T., Mariam, H. G., Berhane, T. Y., Fehniger, T. E., and Kiessling, R. (1991). Immune reactivity to fractionated Leishmania aethiopica antigens during active human infection. J. Clin. Microbiol. 29, 757–763.
Lasso, P., Mateus, J., Pavía, P., Rosas, F., Roa, N., Thomas, M. C., et al. (2015). Inhibitory receptor expression on CD8+ T cells is linked to functional responses against Trypanosoma cruzi Antigens in chronic chagasic patients. J. Immunol. 195, 3748–3758. doi: 10.4049/jimmunol.1500459
Ledesma, D., Berriatua, E., Thomas, M. C., Bernal, L. J., Ortuño, M., Benitez, C., et al. (2017). Performance of Leishmania PFR1 recombinant antigen in serological diagnosis of asymptomatic canine leishmaniosis by ELISA. BMC Vet. Res. 13:304. doi: 10.1186/s12917-017-1224-z
Liang, S. C., Greenwald, R. J., Latchman, Y. E., Rosas, L., Satoskar, A., Freeman, G. J., et al. (2006). PD-L1 and PD-L2 have distinct roles in regulating host immunity to cutaneous leishmaniasis. Eur. J. Immunol. 36, 58–64. doi: 10.1002/eji.200535458
Mateus, J., Pérez-Antón, E., Lasso, P., Egui, A., Roa, N., Carrilero, B., et al. (2017). Antiparasitic treatment induces an improved CD8(+) T cell response in chronic chagasic patients. J. Immunol. 198, 3170–3180. doi: 10.4049/jimmunol.1602095
Melby, P. C., Andrade-Narvaez, F. J., Darnell, B. J., Valencia-Pacheco, G., Tryon, V. V., and Palomo-Cetina, A. (1994). Increased expression of proinflammatory cytokines in chronic lesions of human cutaneous leishmaniasis. Infect. Immun. 62, 837–842.
Mendonça, M. G., de Brito, M. E., Rodrigues, E. H., Bandeira, V., Jardim, M. L., and Abath, F. G. (2004). Persistence of leishmania parasites in scars after clinical cure of American cutaneous leishmaniasis: is there a sterile cure? J. Infect. Dis. 189, 1018–1023. doi: 10.1086/382135
Michailowsky, V., Luhrs, K., Rocha, M. O., Fouts, D., Gazzinelli, R. T., and Manning, J. E. (2003). Humoral and cellular immune responses to Trypanosoma cruzi-derived paraflagellar rod proteins in patients with Chagas' disease. Infect. Immun. 71, 3165–3171. doi: 10.1128/IAI.71.6.3165-3171.2003
Montalvo, A. M., Monzote, L., Fraga, J., Montano, I., Muskus, C., Marín, M., et al. (2008). [PCR-RFLP and RAPD for typing neotropical Leishmania]. Biomedica 28, 597–606. doi: 10.7705/biomedica.v28i4.66
Morell, M., Thomas, M. C., Caballero, T., Alonso, C., and López, M. C. (2006). The genetic immunization with paraflagellar rod protein-2 fused to the HSP70 confers protection against late Trypanosoma cruzi infection. Vaccine 24, 7046–7055. doi: 10.1016/j.vaccine.2006.07.006
Mou, Z., Muleme, H. M., Liu, D., Jia, P., Okwor, I. B., Kuriakose, S. M., et al. (2013). Parasite-derived arginase influences secondary anti-Leishmania immunity by regulating programmed cell death-1-mediated CD4+ T cell exhaustion. J. Immunol. 190, 3380–3389. doi: 10.4049/jimmunol.1202537
Naouar, I., Boussoffara, T., Ben Ahmed, M., Belhaj Hmida, N., Gharbi, A., Gritli, S., et al. (2014). Involvement of different CD4(+) T cell subsets producing granzyme B in the immune response to Leishmania major antigens. Mediat. Inflamm. 2014:636039. doi: 10.1155/2014/636039
Novais, F. O., Carvalho, L. P., Graff, J. W., Beiting, D. P., Ruthel, G., Roos, D. S., et al. (2013). Cytotoxic T cells mediate pathology and metastasis in cutaneous leishmaniasis. PLoS Pathog. 9:e1003504. doi: 10.1371/journal.ppat.1003504
Okwor, I., and Uzonna, J. (2008). Persistent parasites and immunologic memory in cutaneous leishmaniasis: implications for vaccine designs and vaccination strategies. Immunol. Res. 41, 123–136. doi: 10.1007/s12026-008-8016-2
Ovalle, C. E., Porras, L., Rey, M., Ríos, M., and Camargo, Y. C. (2006). [Geographic distribution of Leishmania species isolated from patients at the National Institute of Dermatology Federico Lleras Acosta E.S.E., 1995-2005]. Biomedica 26(Suppl. 1), 145–151.
Pinheiro, R. O., and Rossi-Bergmann, B. (2007). Interferon-gamma is required for the late but not early control of Leishmania amazonensis infection in C57Bl/6 mice. Mem. Inst. Oswaldo Cruz. 102, 79–82. doi: 10.1590/S0074-02762007000100013
Ramírez, J. D., Hernández, C., León, C. M., Ayala, M. S., Flórez, C., and González, C. (2016). Taxonomy, diversity, temporal and geographical distribution of Cutaneous Leishmaniasis in Colombia: a retrospective study. Sci. Rep. 6:28266. doi: 10.1038/srep28266
Ribeiro-de-Jesus, A., Almeida, R. P., Lessa, H., Bacellar, O., and Carvalho, E. M. (1998). Cytokine profile and pathology in human leishmaniasis. Braz. J. Med. Biol. Res. 31, 143–148.
Robinson, R. J., Agudelo, S., Muskus, C., Alzate, J. F., Berberich, C., Barker, D. C., et al. (2002). The method used to sample ulcers influences the diagnosis of cutaneous Leishmaniasis. Trans. R. Soc. Trop. Med. Hyg. 96(Suppl. 1), S169–S171. doi: 10.1016/S0035-9203(02)90071-4
Rodrigues, V., Cordeiro-da-Silva, A., Laforge, M., Ouaissi, A., Akharid, K., Silvestre, R., et al. (2014). Impairment of T cell function in parasitic infections. PLoS Negl. Trop. Dis. 8:e2567. doi: 10.1371/journal.pntd.0002567
Ruiz, J. H., and Becker, I. (2007). CD8 cytotoxic T cells in cutaneous leishmaniasis. Parasite Immunol. 29, 671–678. doi: 10.1111/j.1365-3024.2007.00991.x
Sacks, D., and Noben-Trauth, N. (2002). The immunology of susceptibility and resistance to Leishmania major in mice. Nat. Rev. Immunol. 2, 845–858. doi: 10.1038/nri933
Sallusto, F., Geginat, J., and Lanzavecchia, A. (2004). Central memory and effector memory T cell subsets: function, generation, and maintenance. Annu. Rev. Immunol. 22, 745–763. doi: 10.1146/annurev.immunol.22.012703.104702
Santos Cda, S., Boaventura, V., Ribeiro Cardoso, C., Tavares, N., Lordelo, M. J., Noronha, A., et al. (2013). CD8(+) granzyme B(+)-mediated tissue injury vs. CD4(+)IFNgamma(+)-mediated parasite killing in human cutaneous leishmaniasis. J. Invest. Dermatol. 133, 1533–1540. doi: 10.1038/jid.2013.4
Santrich, C., Moore, L., Sherwin, T., Bastin, P., Brokaw, C., Gull, K., et al. (1997). A motility function for the paraflagellar rod of Leishmania parasites revealed by PFR-2 gene knockouts. Mol. Biochem. Parasitol. 90, 95–109.
Saravia, N. G., Weigle, K., Segura, I., Giannini, S. H., Pacheco, R., Labrada, L. A., et al. (1990). Recurrent lesions in human Leishmania braziliensis infection–reactivation or reinfection? Lancet 336, 398–402.
Silveira, F. T., Lainson, R., De Castro Gomes, C. M., Laurenti, M. D., and Corbett, C. E. (2009). Immunopathogenic competences of Leishmania (V.) braziliensis and L. (L.) amazonensis in American cutaneous leishmaniasis. Parasite Immunol. 31, 423–431. doi: 10.1111/j.1365-3024.2009.01116.x
Theodos, C. M., Povinelli, L., Molina, R., Sherry, B., and Titus, R. G. (1991). Role of tumor necrosis factor in macrophage leishmanicidal activity in vitro and resistance to cutaneous leishmaniasis in vivo. Infect. Immun. 59, 2839–2842.
Von Stebut, E., Ehrchen, J. M., Belkaid, Y., Kostka, S. L., Molle, K., Knop, J., et al. (2003). Interleukin 1alpha promotes Th1 differentiation and inhibits disease progression in Leishmania major-susceptible BALB/c mice. J. Exp. Med. 198, 191–199. doi: 10.1084/jem.20030159
WHO (2016). Leishmaniasis Situation and Trends. Global Health Observatory. Available online at: http://www.who.int/gho/negleted_diseases/leishmaniasis/en/ (Accessed on March 4, 2016).
Wilhelm, P., Ritter, U., Labbow, S., Donhauser, N., Röllinghoff, M., Bogdan, C., et al. (2001). Rapidly fatal leishmaniasis in resistant C57BL/6 mice lacking TNF. J. Immunol. 166, 4012–4019. doi: 10.4049/jimmunol.166.6.4012
Xu, W., and Larbi, A. (2017). Markers of T cell senescence in humans. Int. J. Mol. Sci. 18:1742. doi: 10.3390/ijms18081742
Keywords: Leishmania, leishmaniasis, paraflagellar rod protein-1, biomarkers, CD8+ and CD4+ T-cells, phenotype, inhibitory receptors, Th1-cytokines
Citation: Egui A, Ledesma D, Pérez-Antón E, Montoya A, Gómez I, Robledo SM, Infante JJ, Vélez ID, López MC and Thomas MC (2018) Phenotypic and Functional Profiles of Antigen-Specific CD4+ and CD8+ T Cells Associated With Infection Control in Patients With Cutaneous Leishmaniasis. Front. Cell. Infect. Microbiol. 8:393. doi: 10.3389/fcimb.2018.00393
Received: 25 April 2018; Accepted: 19 October 2018;
Published: 19 November 2018.
Edited by:
Eugenia Carrillo, Instituto de Salud Carlos III, SpainReviewed by:
Hira Nakhasi, Center for Biologics Evaluation and Research (FDA), United StatesJavier Moreno, Instituto de Salud Carlos III, Spain
Vicente Larraga, Department of Cellular and Molecular Biology, Consejo Superior de Investigaciones Científicas (CSIC), Spain
Copyright © 2018 Egui, Ledesma, Pérez-Antón, Montoya, Gómez, Robledo, Infante, Vélez, López and Thomas. This is an open-access article distributed under the terms of the Creative Commons Attribution License (CC BY). The use, distribution or reproduction in other forums is permitted, provided the original author(s) and the copyright owner(s) are credited and that the original publication in this journal is cited, in accordance with accepted academic practice. No use, distribution or reproduction is permitted which does not comply with these terms.
*Correspondence: Manuel C. López, bWNsb3BlekBpcGIuY3NpYy5lcw==
M. Carmen Thomas, bWN0aG9tYXNAaXBiLmNzaWMuZXM=