- 1Programa de Estudio y Control de Enfermedades Tropicales (PECET), Facultad de Medicina, Universidad de Antioquia, Medellín, Colombia
- 2Grupo de Investigación en Inmunotoxicología, Departamento de Farmacia, Facultad de Ciencias, Universidad Nacional de Colombia, Bogotá, Colombia
Introduction: In cutaneous leishmaniasis, the host immune response is responsible for the development of skin injuries but also for resolution of the disease especially after antileishmanial therapy. The immune factors that participate in the regulation of inflammation, remodeling of the extracellular matrix, cell proliferation and differentiation may constitute biomarkers of diseases or response to treatment. In this work, we analyzed the production of the growth factors EGF, TGFβ1, PDGF, and FGF during the infection by Leishmania parasites, the development of the injuries and the early response to treatment.
Methodology: Golden hamsters were infected with L. (V) braziliensis. The growth factors were detected in skin scrapings and biopsies every 2 weeks after infected and then at day 7 of treatment with different drug candidates by RT-qPCR. The parasitic load was also quantified by RT-qPCR in skin biopsies sampled at the end of the study.
Results: The infection by L. (V) braziliensis induced the expression of all the growth factors at day 15 of infection. One month after infection, EGF and TGFβ1 were expressed in all hamsters with inverse ratio. While the EGF and FGF levels decreased between day 15 and 30 of infection, the TGFβ1 increased and the PGDF levels did not change. The relative expression of EGF and TGFβ1 increased notably after treatment. However, the increase of EGF was associated with clinical cure while the increase of TGFβ1 was associated with failure to treatment. The amount of parasites in the cutaneous lesion at the end of the study decreased according to the clinical outcome, being lower in the group of cured hamsters and higher in the group of hamsters that had a failure to the treatment.
Conclusions: A differential profile of growth factor expression occurred during the infection and response to treatment. Higher induction of TGFβ1 was associated with active disease while the higher levels of EGF are associated with adequate response to treatment. The inversely EGF/TGFβ1 ratio may be an effective biomarker to identify establishment of Leishmania infection and early therapeutic response, respectively. However, further studies are needed to validate the utility of the proposed biomarkers in field conditions.
Introduction
Leishmaniasis is a parasitic disease caused by protozoa of the genus Leishmania spp. which are transmitted by phlebotomies insects (Bates, 2007; Sharma and Singh, 2008). The disease is widely distributed in five continents and is endemic in low-income countries in tropical and subtropical regions (Alvar et al., 2012). The disease manifests itself in three main clinical forms known as cutaneous, mucosal, and visceral leishmaniasis. Mucosal and cutaneous leishmaniasis (ML and CL, respectively) are characterized by the presence of granulomatous lesions in the mucous and dermis, respectively (Herwaldt, 1999; Handler et al., 2015; Kevric et al., 2015), that may cause disfigurement accompanied by social stigmatization and psychological disorders, altering the economic well-being of patients (Weiss, 2008; Okwor and Uzonna, 2016). There are very few options to treat CL and ML and all have numerous disadvantages that evidence the urgent need to develop new and better therapeutic alternatives. It is known that CL is manifested as skin lesions that result from the exacerbated activation of the immune system through the recruitment of abundant monocytes, neutrophils, NK cells, and CD8+ and CD4+ T lymphocytes (Brewig et al., 2009; Hrdinka et al., 2011; Nylén and Eidsmo, 2012; Soong et al., 2012). The activation of all these cells induces a chronic inflammatory response that leads to the necrosis of the tissue and therefore to the skin damage and the appearance of ulcers (in most cases). This cutaneous lesion usually resolves after specific therapy that let the elimination of the antigenic stimulus and therefore, the resolution of the inflammatory response and repair of the damaged tissue (McGwire and Satoskar, 2014; Copeland and Aronson, 2015; de Menezes et al., 2015; Aronson et al., 2016).
Although the immune response to Leishmania has been described during infection and disease, this immune response during and after treatment has been poorly described. To date, no information is available about the production of dermal and epidermal growth factors during Leishmania infection or concerning the healing of lesions caused by Leishmania spp. Studies carried out with other types of ulcers reported that during healing, the epidermal tissue is formed after transformation of the dermis into fibrous tissue (scarring) accompanied by an increase in NK cells, a decrease in circulating T cells with depletion of CD8+ T cells, and a decrease of CD4+ T cells with a decrease in the production of IFNγ (Lakhal-Naouar et al., 2015) and expression of dermal and epidermal growth factors (Kiwanuka et al., 2012).
Growth factors are polypeptides that stimulate cell proliferation, migration, and differentiation as well as survival and apoptosis (Bennett and Schultz, 1993). The most important growth factors in this process of tissue repair are fibroblast growth factor (FGF), platelet derived growth factor (PDGF), transforming growth factor beta 1 (TGFβ1) and alpha (TGFα), epidermal growth factor (EGF), connective tissue growth factor (CTGF), and vascular endothelial growth factor (VEGF; (Robson, 1991; Steed, 1997)). The source and function of these growth factors are summarized in Table 1. While some observations suggest an important role for TGFβ during parasite establishment in the early stages of human CL (Barral et al., 1995) and treatment failure in patients with visceral leishmaniasis (Elmekki et al., 2016) there seem to be no reports of studies for the other growth factors in the context of leishmaniasis.
Based on the important role of growth factors during healing and tissue repair, this study aimed to identify the expression levels of EGF, FGF, PDGF, and TGFβ1 during: (i) infection by Leishmania (V) braziliensis, (ii) development of CL, and (iii) early response to treatment using the experimental model for CL in the golden hamster (Mesocricetus auratus). An useful RT-qPCR method to quantify EGF, FGF, PDGF, and TGFβ1 expression was also standardized.
Materials and Methods
Compounds
Propantheline bromide (CAS #50-34-0), limonin (CAS #1180-71-8), nomilin (CAS #1063-77-0), azadirachtin (CAS #11141-17-6), cryptolepine hydrate (CAS #480-26-2), glycyrrhizin (CAS #1405-86-3), and oleanolic acid (CAS #508-02-1) were purchased at Sigma Aldrich (St Louis, MO, USA) and were used as active pharmaceutical ingredients (API) for new formulations. Meglumine antimoniate (MA) (Sanofi-Aventis Bogotá, Colombia) was used as a reference antileishmanial drug.
Parasites
Green fluorescent protein (GFP)-transfected L. (V) braziliensis promastigotes (MHOM/CO/88/UA301-EGFP) were maintained in biphasic Novy-MacNeal-Nicholle (NNN) medium phosphate buffer saline (PBS) enriched with glucose at pH 6.9.
Formulations
An oil-in-water (O/W) semisolid emulsion was prepared which served as the matrix in which the API were embedded. The designed formulations were as follows: 0.5% propantheline bromide, 2.4% limonin, 2.2% nomilin, 1.1% azadirachtin, 1.4% cryptolepine hydrate, 5% glycyrrhizin, and 2.5% oleanolic acid. The concentration of the API was selected at convenience, according to the availability of the compound and previous results of the antileishmanial activity obtained in vitro.
Animals
Eighty-six week-old hamsters, male and female, with an average weight of 180 g (160–200 g) were used. Hamsters were maintained in specific pathogenic-free conditions in an animal facility at Universidad de Antioquia (Medellín, Colombia), housed in transparent cages in groups of three or four animals per cage, during the study.
Experimental Infection
Previous anesthesia with a mixture of ketamine (40 mg/kg) and xylazine (5 mg/kg), hamsters were injected in the dorsum with 5 × 108 promastigotes of L. braziliensis in the stationary phase of growth (day 6 in culture) in 100 μl PBS. After 15 and 30 days post-infection (ID15 or ID30, respectively), one group of eight hamsters each were humanely sacrificed. Skin biopsies from the inoculation site were obtained and stored in RNA later for the RNA extraction. The remaining hamsters were kept under observation until the development of ulcers. Skin biopsies of four uninfected hamsters were obtained and they corresponded to biopsies obtained before infection day (ID0).
Therapeutic Response
Four weeks after the infection, ulcers were formed, and treatment with each of the formulations had started. For this, the hamsters were randomly distributed in eight groups with eight hamsters each. Each group was treated topically during 20 days with 40 mg/day of one of the following formulations: 0.5% propantheline bromide, 2.4% limonin; 2.2% nomilin, 1.1% azadirachtin, 1.4% cryptolepine hydrate, 5% glycyrrhizin, and 2.5% oleanolic acid; or intramuscular MA (120 mg/kg/10 days). The hamsters were monitored daily during the study recording any change in behavior or death. The area of the injuries was measured before treatment (TD0) and 90 days after completion of the treatment (PTD90), which corresponded to the end of the study. The effectiveness of each treatment was determined according to the area of the lesion at PTD90 with respect to the size of the lesion before treatment. The response to the treatment was classified as cure (if healing of 100% of the area of the lesion did occur) or improvement (if the area of injury decreased more than 20%). On the contrary, the outcome was classified as failure (if the area of injury increased) or as relapse (if reactivation of the injury appeared after an initial cure). At the end of the study, hamsters were sacrificed humanely in a CO2 chamber and necropsied. Skin samples for parasite load analysis were obtained. Prior to treatment (TD0) and on day 7 of treatment (TD7), scraping samples were taken from the lesions and deposited in RNA later (for RNA extraction).
RNA Extraction
The RNA from the skin samples was extracted using Trizol®; (Invitrogen) following the manufacturer's instructions and then quantified in a Nanodrop 1000 (Thermo scientific).
Retrotranscription
One hundred grams of RNA were treated with 1 μl DNase I (Fermentas), 1 μl of buffer, and 8 μl of nuclease free water; the mixture was incubated in a thermocycler PTC 100TM (MJ research) for three cycles: 30 min at 37°C, 5 min at 4°C, and 10 min at 65°C. Using the maximum first strand cDNA synthesis kit, the RNA was transcribed into cDNA, following the manufacturer's instructions: 4 μl master mix reaction, 2 μl RT enzyme mix, 2 μl RNA DNase I treated, and 12 μl water were mixed and incubated in the PTC 100TM (MJ research) thermocycler for three cycles: 10 min at 25°C, 15 min at 50°C, and 5 min at 85°C.
Growth Factor Expression
Specific primers for EGF, PDGF, FGF, and TGFβ1, as well as fluorescent FAM-labeled hydrolysis probes, were designed. The sequences for each forward (Fw) and reverse (Rv) primer and probe used were as follows:
FGF,Fw,5′-GTGTCAAGGCTGCTAGGTTT-3′, Rv,5′-ACACATTGTATCCATCCTCAA-3′ and probe 5′-TCGCCTCACTTCGATCCCG-3′; EGF,Fw,5′-CAGAACAAAGCCAGAAAATC-3′, Rv,5′-CTGCAAGTACGTTCGTTTAACT-3′ and probe 5′-AGACTCGCGTTGCAAGGCG-3′; PDGF,Fw,5′-GGCTCGAAGTCAGATCCATA-3′,Rv,5′-CTTCTCCGGCACATGCTTAA-3′and probe 5′-TGGAGACAAGCCTGAGAGCC-3′;TGFβ1,Fw,5′-AGCCTGGACACACAGTACAGTA-3′,Rv,5′-CTTGCGACCCACGTAGTAC-3′and probe 5′-AACACAACCCGGGTGCTTC-3′;γActin,Fw,5′-ACAGAGAGAAGATGACGCA-3′,Rv,5′-GCCTGAATGGCCACGTAC-3′and probe 5′-TTGAAACCTTCAAATGACGCA-3′.
The reaction of amplification was carried out with 1 μl of the cDNA and using the following protocol: 600 s at 95°C, 40 cycles of 15 s at 95°C, and 60 s at 60°C in a Smart Cycler II (Cepheid, Sunnyvale, CA, USA). The efficiency of the amplification reaction was determined using the LinReg program and the expression levels were calculated using the ΔΔCT method, comparing the levels of expression for PI15, PI30 with respect to healthy skin before infection and TD7 with respect to TD0. Additionally, the levels of induction were compared in terms of clinical outcome, in terms of cure, improvement, relapse, or failure.
Parasite Load
The parasitic load on the skin biopsy samples taken at the end of the study (PRD90) was determined by RT-qPCR using the Vero 1-step RT-qPCR SYBR Green kit, a 123 bp fragment of the DNA polymerase I gene from Leishmania was amplified. To do this, 20 ng of RNA, 12.5 μl the mix, and 100 ng of the each primer Fw 5′-TGAGCGCATCGAGTACCT-3′ and Rv 5′-TCCCGCTTGCCATCCTC-3′, with a volume adjusted to 25 μl using nuclease-free water, were used to carry out the reaction with the Smart Cycler II (Cepheid, Sunnyvale, CA, USA): 50°C 15 min, 95°C 15 min and 40 cycles 95°C 15 s, 60°C 20 s and 72°C 20 s, a final cycle 72°C 300 s and a melting curve between 60 and 95°C. Absolute quantification was performed using a standard logarithmic scale from 1 to 1 million parasites.
Ethical Aspects
All the procedures were approved by the Ethics Committee for Animal Experimentation of the Universidad de Antioquia (Act No. 91 of 2014).
Statistical Analysis
The differences between the parasitic loads according to the clinical results were determined by a one-way ANOVA and a Tukey's test for multiple comparisons. The differences in the expression of growth factors between ID15 and ID30 vs. ID0 and between TD0 and TD7 were determined by two-way ANOVA and Bonferroni's test for multiple comparisons. In addition, differences in expression levels according to the clinical results were also obtained by two-way ANOVA and Bonferroni's test.
Results
Lesion Progression in Hamsters
After intradermal injection of promastigotes of L. (V) braziliensis promastigotes in dorsum, hamsters were monitored weekly for any sign of induration or skin damage at the site of inoculation. The diameter of the injury increased in size every week. In this way, the average size of the injuries was 1,29 ± 3.29 mm2 at ID8, 3.35 ± 7.92 mm2 at ID15, 12.75 ± 19 mm2 at ID21, and 39.93 ± 40.73 mm2 at ID30. The lesion on the skin was evidenced first as a nodule and then as an ulcer. The induration varied from mild in the second week post-infection to exacerbated in the 4 week after inoculation (Figure 1).
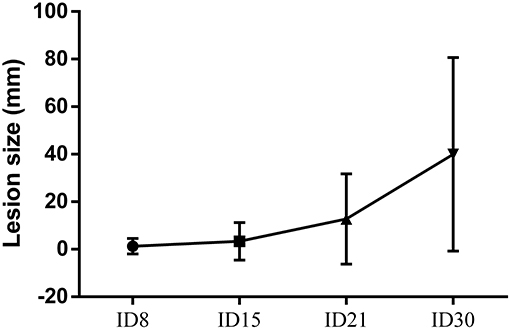
Figure 1. Cutaneous leishmaniasis progression. Quantification of lesion area in in golden hamsters experimentally infected with 5 × 108 promastigotes of L. (V) braziliensis. Data are presented as the mean ± SD of 24 hamsters at days 8, 15, 21, and 30 after infection.
Expression of PDGF, EGF, FGF, and TGFβ1 Associated With the Process of Infection by L. (V) braziliensis in the Hamster Model for Cutaneous Leishmaniasis
The expression levels of PDGF, EGF, FGF, and TGFβ1 were calculated by the ΔΔCT method and expressed as the mean ± SD of the number of times that each factor was induced with respect to healthy skin before infection (ID0), during the development of the lesion (ID15 and ID30). Expression levels lower than the constitutive gene were found for the PDGF, EGF, TGFβ1, and FGF before infection, and these basal levels were used for the calculation of ΔΔCT. Infection by L. (V) braziliensis induced the expression of all the growth factors in ID15, with levels of induction with respect to healthy hamsters of: PDGF 0.4 ± 0.1, EGF 3.9 ± 0.2, FGF 1.1 ± 0.5, and TGFβ1 0.4 ± 0.2. In ID30, PDGF was expressed in 3/8 hamsters with an expression value of 0.6 ± 0.1; EGF and TGFβ1 were expressed in all hamsters with an expression value of 1.5 ± 0.7 and 1.6 ± 0.5, respectively. Lastly, FGF was expressed in 2/8 hamsters and its expression value was 0.2 ± 0.0. The differences in EGF and TGFβ1 expression levels between ID15 and ID30 were statistically significant with p < 0.0001 and < 0.0442, respectively. On the contrary, the differences between PDGF and FGF were not significant (Figure 2).
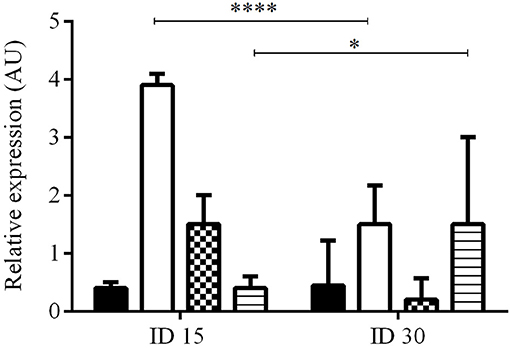
Figure 2. Relative expression of growth factors during infection and development of injury. The figure shows the relative expression at ID1 (n = 8) and ID30 (n = 8) vs. before infection (ID0) calculated by the ΔΔCT method. PDGF (black), EGF (white), FGF (pattern), and TGFβ1 (lines). ****p < 0.0001; *p < 0.0442.
Expression of PDGF, EGF, FGF, and TGFβ1 in Response to Treatment
Of 64 hamsters treated with the different compounds and followed up for 3 months, 10 hamsters (15.6%) cured, 24 hamsters (37.5%) showed improvement of their ulcers, 27 hamsters (42.2%) failed, and 3 hamsters (4.6%) relapsed. The appearance of the lesions according to the clinical outcomes after treatment are shown in Figure 3. The levels of expression of PDGF, EGF, FGF, and TGFβ1 at TD7 were compared with respect to the levels before treatment (TD0) using the ΔΔCT method. In the cured hamsters, the expression levels increased 0.9 ± 0.1 times for PDGF, 46.9 ± 34.2 for EGF, 0.7 ± 0.2 for FGF, and 0.8 ± 0.7 for TGFβ1 (Figure 4). In the hamsters with improvement of their ulcers, the expression levels increased 0.011 ± 0.017 times for PDGF, 17.5 ± 2.4, for EGF, 0.01 ± 0.03 for FGF, and 2.1 ± 0.1 for TGFβ1. In turn, in the hamster that relapsed, the levels for PDGF were 0.012 ± 0.01, 9.5 ± 8.8 for EGF, 0.8 ± 0.7 for TGFβ1, and FGF was not detected. Finally, in the hamsters that failed to the treatment, the expression levels for EGF and TGFβ1 increased 8.9 ± 2.1 and 3.1 ± 0.6 times, respectively, while PDGF and FGF were not detected. Significant differences in expression were only observed in EGF expression between cured hamsters vs. TD0 (p < 0.0001), improvement vs. TD0 (p < 0.0413), cured vs. improvement (p < 0.0003), cured vs. failure (p < 0.0001), and cured vs. relapsed (p < 0.0001) (Figure 4).
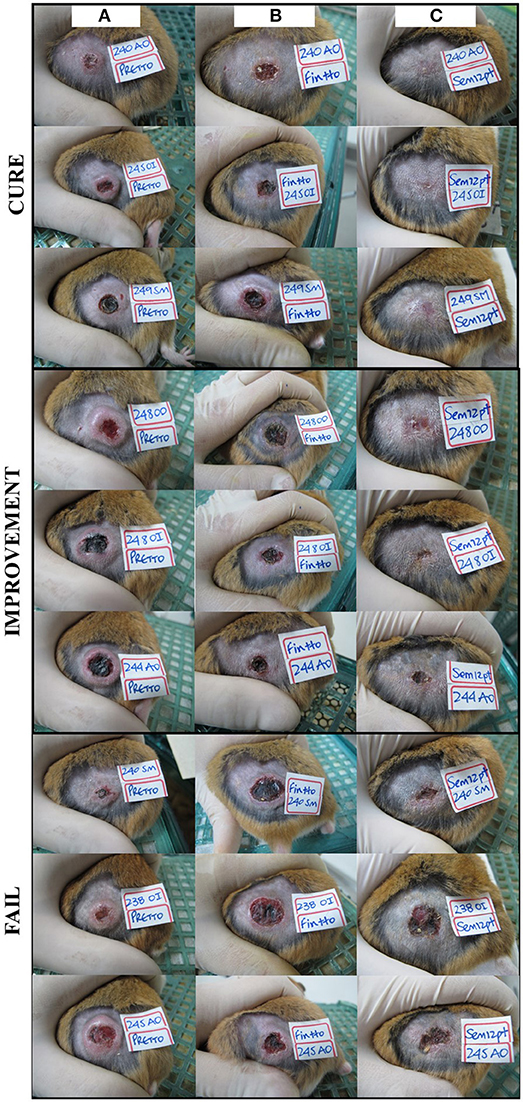
Figure 3. Treatment progress of cutaneous leishmaniasis in hamsters experimentally infected with L. (V) braziliensis. A representative photograph of lesion, (A) before treatment, (B) end of treatment, and (C) 90 days post-treatment. Note the complete re-epithelialization of the skin in cured hamsters, the decrease in size of the injury during improvement or the increases in the size of the injury during fail to treatment.
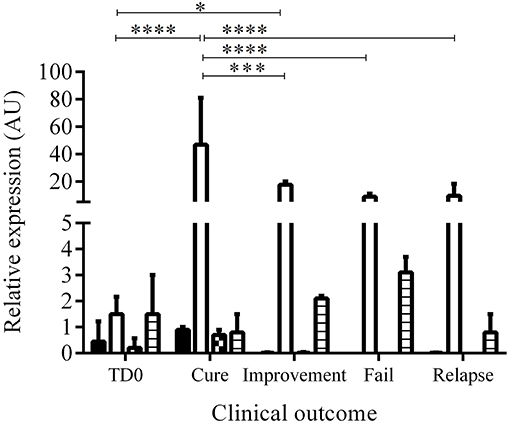
Figure 4. Relative expression of growth factors before and during treatment. The figure shows the induction of growth factors calculated by the ΔΔCT between TD0 and TD7 method according to the clinical outcome. Bars represent the mean value ± SD. PDGF (black), EGF (white), FGF (square), and TGFβ1 (lines). *p < 0.0413; ***p < 0,0003; ****p < 0.0001, cured (n = 10), improvement (n = 24), fail (n = 27), relapse (n = 3).
Only EGF and TGFβ1 showed expression levels >1. When compared the proportion of EGF vs. TGFβ1 we found that the EGF was expressed 58.6 times more than the TGFβ1 in cure and 8.3 in improvement while in relapses and fails the proportion was 11.8 and 2.9, respectively. Although the EGF increases during treatment, the increase is lower in relation to a poor therapeutic response. Thus, for example, the EGF was expressed 2.68 more times in cure vs. improvement, 4.93 times more in cure vs. relapse and 5.26 times more in cure vs. failure. In a similar way, the TGFβ1 was expressed 3.9 times more in failure vs. cure, 2.6 times more in improvement vs. cure.
The effect of each treatment in the expression of each growth factor in terms of increasing, reducing, or no changes with respect to the clinical outcome is shown in Table 2. The treatment with meglumine antimoniate and propantheline bromide, both drugs associated with cure of CL in hamster, showed the major increases in the expression of EGF.
Parasite Load During Infection by L. (V) braziliensis and Treatment in the Golden Hamster (Mesocricetus auratus)
The number of parasites in the injury during infection (ID15 and ID30) and at the end of the study (PTD90) was measured by RT-qPCR and was expressed as parasites per mg of tissue. The parasite load in the 10 cured hamsters was 76.8 ± 115.8, while in the 24 animals with clinical improvement the parasite load was 238.0 ± 57.0. In turn, in the 27 animals classed as failures, the parasite load was 710.4 ± 484.5, while in the 3 hamsters that relapsed the parasitic load was 3478.0 ± 388.6. Differences were statistically significant between cured vs. fail, cured vs. relapsed, improvement vs. failure, improvement vs. relapse, and failure vs. relapsed. In all cases, the p value was < 0.0001 (Figure 5).
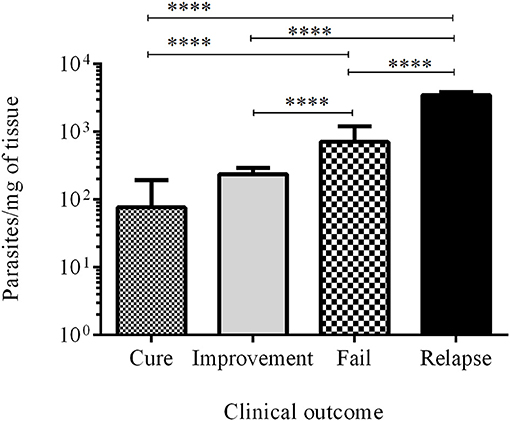
Figure 5. Parasite load. Bars represent the mean value ± SD of the number of parasites per mg of tissue in cured hamsters (white bar), improvement (gray bar), failure (square bar), and relapse (black bar). ****p < 0.0001.
Discussion
For several decades, there have only been five drugs used for the treatment of leishmaniasis. Although these are still effective, they are far from ideal due to factors such as costs, duration of treatment, toxicity, and the appearance of resistant strains (Oliveira et al., 2011; Monge-Maillo and López-Vélez, 2013; Wolf Nassif et al., 2017). The intensive work done in the last 15 years in the search for alternative treatments of leishmaniasis has allowed for the identification of numerous candidate molecules or compounds. Some of these have advanced in their development until the stage of clinical evaluation. However, only a few are considered as real candidates for the development of medicines to date. Many candidates fail because of the poor effectiveness demonstrated in phase II clinical trials (Singh et al., 2012). The extremely high cost of clinical trials is a bottleneck in the development of new drugs; therefore, it is necessary to identify markers that allow for early identification of the response to the treatment that is being evaluated (Frank and Hargreaves, 2003; Zwierzina, 2008).
Although multiple studies have analyzed susceptibility and resistance genes associated to the development of the lesions (Castellucci et al., 2012; Sohrabi et al., 2013; Abdoli et al., 2017), the role of EGF, FGF, and PDGF in the establishment of the infection, development of the ulcer, and in early response to treatment has not been established. In contrast, although the TGFβ1 has been related to susceptibility and chronicity of the infection (Balak et al., 1992; Barral-Netto and Barral, 1994; Barral et al., 1995; Bogdan and Röllinghoff, 1998; Nieto et al., 2011; Hejazi et al., 2012; Rodrigues et al., 2014; Bhattacharya et al., 2016), its role has not been described in the context of early therapeutic response. In this work, we described the dynamics of the production of EGF, FGF, PDGF, and TGFβ from initial infection and manifestation of skin lesions to the early stages of response to different treatment compounds. We demonstrated that the expression of PDGF, EGF, FGF, and TGFβ1 was induced at ID15 during the establishment of the experimental infection by L. (V) braziliensis. This result suggests that, during infection, the cells are activated to control the tissue damage as a result of inflammation (Mast and Schultz, 1996). At ID30 when the infection is already established and the ulcer is manifested, the expression of growth factors changes drastically, not only in the profile of factors, but also in the level of induction (Mast and Schultz, 1996). FGF and PDGF were no longer expressed in more than half of the animals, but EGF and TGFβ1 showed an interesting change reversing their induction levels by increasing TGFβ1 and decreasing EGF.
The decreased expression of PDGF and FGF correlates with the appearance of lesions by ID30. It has been confirmed in the hamster model for CL that infection by Leishmania generates the activation of the Nlrp3 inflammasome with the subsequent production of IL1β (Lima-Junior et al., 2013), and this cytokine may negatively regulate the expression of PDGF (Barrientos et al., 2008; Mundy, 2009). In the case of FGF and other growth factors, such as TGFα, KGF, and VEGF, it has been shown in the experimental CL hamster model that, in some types of ulcers induced by drugs or anti-tumor therapies, the decrease in proinflammatory cytokines such as IL1β and TNFα positively regulates the expression of these factors (Araújo et al., 2015). When the inflammatory process perpetuates, it has a negative regulatory effect on these two growth factors, PDGF and FGF, in some cases, halting expression and in other cases, reducing it. Another explanation for the reduction in the expression of these growth factors is that the macrophages, keratinocytes, endothelial cells, and fibroblasts involved in the production of growth factors in the infected and inflamed tissue are in a context where their functions of survival, proliferation, cell differentiation, and angiogenesis have been regulated by the need to eliminate the parasite.
Interestingly, TGFβ1 tripled its expression in 8/8 hamsters during infection. In addition, other studies have associated the early expression of this factor and its increased expression levels with the regulation of the immune response, allowing for the establishment of infection and development of ulcers (Balak et al., 1992; Barral-Netto and Barral, 1994; Barral et al., 1995; Mougneau et al., 2011; Hejazi et al., 2012; Nylén and Eidsmo, 2012; Rodrigues et al., 2014; Bhattacharya et al., 2016), since TGFβ1 has the capacity to inhibit the Th1 response affecting the production of IFNγ, IL12, and key cytokines in the control and elimination of the parasite (Reed, 1999). On the other hand, the expression of EGF decreased with respect to ID15, reducing by half in 8/8 animals. This growth factor is very important for the proliferation of keratinocytes, which are essential for the establishment of a Th1 immune response that favors the control of the infection (Steed, 1997; Eming et al., 2007; Kiwanuka et al., 2012; Pikuła et al., 2015). Its increase at ID15 is likely to be an attempt to favor the Th1 response. However, when the immune response is regulated by TGFβ1 and the infection is effectively established at ID30, its expression is negatively regulated, due to the fact that the macrophages and fibroblasts, as its main sources, are in a context in which the production of EGF is diminished.
When analyzing the early expression of growth factors as biomarkers for the clinical outcome of our experimental model, we initially found differences in the expression profile of these factors. Thus, in the 3 animals that relapsed after treatment, the EGF was expressed at higher levels that TGFβ1; nevertheless, since it is only a group of three hamsters, it limits the comparisons with the other groups. In turn, in hamsters in which the treatment failed, the expressed factor profile involved EGF, and TGFβ1. In turn, in the cured hamsters and those that showed improvement of their injuries, all growth factors, even FGF, were expressed.
When comparing the production profile of growth factors in fetal and adult fibroblasts, differences have been correlated with the healing and the re-epithelialization of injuries and a better appearance of the scars, which explains why fetal scarring leaves scarcely imperceptible scars (Broker et al., 1999). However, the presence of several factors is necessary in order to repair damaged tissue efficiently (Papanas and Maltezos, 2007; Buchberger et al., 2010). Some clinical trials have evaluated the use of one or several growth factors such as PDGF, EGF, and FGF for the treatment of chronic ulcers, showing good results when used individually but with better results when used in combination (Robson, 1991; Mast and Schultz, 1996; Singla et al., 2012).
On the other hand, the profile of the growth factors in our model allowed us to predict a positive clinical result of the treatment at an early stage, as well as the levels of induction of the different growth factors. In the case of FGF and PDGF, since their expression is not constant in all hamsters, it is difficult to conclude that the levels of induction of these factors are a good biomarker for early therapeutic response. However, the benefit of knowing the behavior of the expression of these growth factors and that their early induction depends on the treatment will allow for future evaluations in our animal model to identify candidates for medications that possess not only antileishmanial potential but also scarring potential.
In the case of TGFβ1, its role in pathogenesis is well described. There is an increase in the levels of TGFβ1 in peritoneal macrophages infected with the parasites (Balak et al., 1992). In human biopsies from active lesions in both the cutaneous form and the mucosal form of the disease, the presence of this factor is also detected (Barral et al., 1995), which is related to one of the functions of TGFβ1 in the regulation of the immune response allowing for the parasite to replicate (Abdoli et al., 2017). TGFβ1 appears to be a candidate biomarker for pathogenesis and for a negative therapeutic response to treatment (failure and relapse). Although the differences in the levels of expression were not statistically significant, the tendency is to increase in production when there is failure or relapse, and decrease in production when curing or improvement occur.
EGF showed significant differences in the levels of induction on TD7 with respect to TD0 and difference in the expression with respect to the clinical result among the cured animals and all the other clinical outcomes. EGF plays a very important role in the process of cell proliferation, activation, and re-epithelialization (Barrientos et al., 2008). It increases in acute wounds (Shen et al., 2017) and is found to decease in chronic wounds (Mast and Schultz, 1996). In the case of CL, we confirm low levels of EGF before treatment, because it is a chronic injury. However, by implementing a treatment that induces healing, we find that the level of EGF expression increases, in comparison to the treatments that produce improvement, failure, or relapse. Notoriously, propantheline bromide and MA, which were medications associated with healing, showed a similar profile. These results suggest that this profile can help predict a curative response during treatment follow-up.
This study focused on the search for biomarkers in leishmaniasis and allowed us to establish the role of multiple cytokines in the process of infection and in pathogenesis as well as in the candidates for biomarkers related to the immune response profile in visceral leishmaniasis (Portela et al., 2018). In the case of canine visceral leishmaniasis, a recent study identified some proteins as biomarkers of the therapeutic response in dogs. Nevertheless, none of these proteins were growth factors or were related to the activation of growth factors (Martinez-Subiela et al., 2017). This is most likely because the samples used for monitoring the therapeutic response biomarkers were taken 1 month after the end of the treatment, when the parasite is likely to have been eliminated. In this case, the samples would not indicate the effectiveness of the treatment early but would confirm whether the treatment was effective. Our results allow us to implement in our experimental model a rapid measurement of the therapeutic response to treatment and define the optimal dose or frequency, thus obtaining better therapeutic success with the new candidates that are being tested in vivo for the treatment of CL.
Conclusion
A differential profile of EGF, TGFβ1, PDGF, and FGF expression was observed during the establishment of L. (V) braziliensis infection and response to treatment in our experimental CL model. Higher induction of TGFβ1 are more associated with active disease (infection and failure or relapses after treatment) while the higher levels of EGF are associated with adequate response to treatment. Thus, the inversely EGF/TGFβ1 ratio may be an effective biomarker to identify establishment of Leishmania infection and early therapeutic response, respectively. However, further studies are needed to validate the utility of these growth factors as biomarkers in the pathogenesis of human CL and response to treatment under field conditions.
Author Contributions
AM participated in the design of the project, carried out the trials, analyzed the results, and participated in writing of the manuscript for publication. SR was in charge of the project and the analysis of results, and participated in writing of the manuscript. LY, AB, and RH helped in performing the experiments. GD and IV participated in the analysis of results and in the writing of the manuscript.
Conflict of Interest Statement
The authors declare that the research was conducted in the absence of any commercial or financial relationships that could be construed as a potential conflict of interest.
The reviewer AIM and handling editor declared their shared affiliation at time of review.
Acknowledgments
The authors would like to thank the Colombian Department of Science, Technology and Innovation - Colciencias (Grant CT-695-2014) for their financial support.
References
Abdoli, A., Maspi, N., and Ghaffarifar, F. (2017). Wound healing in cutaneous leishmaniasis: a double edged sword of IL-10 and TGF-β. Comp. Immunol. Microbiol. Infect. Dis. 51, 15–26. doi: 10.1016/j.cimid.2017.02.001
Alvar, J., Vélez, I. D., Bern, C., Herrero, M., Desjeux, P., Cano, J., et al. (2012). Leishmaniasis worldwide and global estimates of its incidence. PLoS ONE 7:e35671. doi: 10.1371/journal.pone.0035671
Andres, C., Hasenauer, J., Ahn, H. S., Joseph, E. K., Isensee, J., Theis, F. J., et al. (2013). Wound-healing growth factor, basic FGF, induces Erk1/2-dependent mechanical hyperalgesia. Pain 154, 2216–2226. doi: 10.1016/j.pain.2013.07.005
Araújo, A. A., De Varela, H., Addison, C., and Xavier, C. (2015). Azilsartan reduced TNF- α and IL-1 β levels, increased IL-10 levels and upregulated VEGF, FGF, KGF, and TGF- α in an oral mucositis model. PLoS ONE 10:e0116799. doi: 10.1371/journal.pone.0116799
Aronson, N., Herwaldt, B. L., Libman, M., Pearson, R., Lopez-Velez, R., Weina, P., et al. (2016). Diagnosis and treatment of Leishmaniasis: clinical practice guidelines by the infectious diseases society of america (IDSA) and the american society of tropical medicine and hygiene (ASTMH). Clin. Infect. Dis. 63, e202–e264. doi: 10.1093/cid/ciw670
Balak, K., Jacobson, M., Sunshine, J., Barral-netto, M., Barral, A., Brownell, C. E., et al. (1992). Transforming growth factor-y in leishmanial infection: a parasite escape mechanism. Science 257, 545–548.
Barral, A., Teixeira, M., Reis, P., Vinhas, V., Costa, J., Lessa, H., et al. (1995). Transforming growth factor-beta in human cutaneous leishmaniasis. Am. J. Pathol. 147, 947–954.
Barral-Netto, M., and Barral, A. (1994). Transforming growth factor-beta in tegumentary leishmaniasis. Braz. J. Med. Biol. Res. 27, 1–9.
Barrientos, S., Stojadinovic, O., Golinko, M. S., Brem, H., and Tomic-Canic, M. (2008). Perspective article: growth factors and cytokines in wound healing. Wound Repair Regen. 16, 585–601. doi: 10.1111/j.1524-475X.2008.00410.x
Bates, P. A. (2007). Transmission of Leishmania metacyclic promastigotes by phlebotomine sand flies. Int. J. Parasitol. 37, 1097–1106. doi: 10.1016/j.ijpara.2007.04.003
Bennett, N. T., and Schultz, G. S. (1993). Growth-factors and wound-healing - biochemical-properties of growth-factors and their receptors. Am. J. Surg. 165, 728–737. doi: 10.1016/S0002-9610(05)80797-4
Bhattacharya, P., Ghosh, S., Ejazi, S. A., Rahaman, M., Pandey, K., Ravi Das, V. N., et al. (2016). Induction of IL-10 and TGFβ1 from CD4+CD25+FoxP3+ T cells correlates with parasite load in Indian Kala-azar patients infected with Leishmania donovani. PLoS Negl. Trop. Dis. 10:e0004422. doi: 10.1371/journal.pntd.0004422
Bogdan, C., and Röllinghoff, M. (1998). The immune response to Leishmania: mechanisms of parasite control and evasion. Int. J. Parasitol. 28, 121–134. doi: 10.1016/S0020-7519(97)00169-0
Brewig, N., Kissenpfennig, A., Malissen, B., Veit, A., Bickert, T., Fleischer, B., et al. (2009). Priming of CD8+ and CD4+ T cells in experimental leishmaniasis is initiated by different dendritic cell subtypes. J. Immunol. 182, 774–783. doi: 10.4049/jimmunol.182.2.774
Broker, B. J., Chakrabarti, R., Blynman, T., Roesler, J., Wang, M. B., and Srivatsan, E. S. (1999). Comparison of growth factor expression in fetal and adult fibroblasts: a preliminary report. Arch. Otolaryngol. Head Neck Surg. 125, 676–680. doi: 10.1001/archotol.125.6.676
Buchberger, B., Follmann, M., Freyer, D., Huppertz, H., Ehm, A., and Wasem, J. (2010). The importance of growth factors for the treatment of chronic wounds in the case of diabetic foot ulcers. GMS Health Technol. Assess. 6:Doc12. doi: 10.3205/hta000090
Castellucci, L., Jamieson, S. E., Almeida, L., Oliveira, J., Guimaraes, L. H., Lessa, M., et al. (2012). Wound healing genes and susceptibility to cutaneous leishmaniasis in Brazil. Infect. Genet. Evol. 12, 1102–1110. doi: 10.1016/j.meegid.2012.03.017
Copeland, N. K., and Aronson, N. E. (2015). Leishmaniasis: treatment updates and clinical practice guidelines review. Curr. Opin. Infect. Dis. 28, 426–437. doi: 10.1097/QCO.0000000000000194
de Menezes, J. P., Guedes, C. E. S., Petersen, A. L. D. O.A., Fraga, D. B. M., and Veras, P. S. T. (2015). Advances in development of new treatment for Leishmaniasis. Biomed. Res. Int. 2015, 1–11. doi: 10.1155/2015/815023
Dörr, W., Spekl, K., and Farrell, C. L. (2002). The effect of keratinocyte growth factor on healing of manifest radiation ulcers in mouse tongue epithelium. Cell Prolif. 35(Suppl. 1), 86–92. doi: 10.1006/rwcy.2002.0811
Douglas, H. E. (2010). TGF-β in wound healing: a review. J. Wound Care 19, 403–406. doi: 10.12968/jowc.2010.19.9.78235
Elmekki, M. A., Elhassan, M. M., Ozbak, H. A., and Mukhtar, M. M. (2016). Elevated TGF-beta levels in drug-resistant visceral leishmaniasis. Ann. Saudi Med. 36, 73–7. doi: 10.5144/0256-4947.2016.73
Eming, S. A., Krieg, T., and Davidson, J. M. (2007). Inflammation in wound repair: molecular and cellular mechanisms. J. Invest. Dermatol. 127, 514–525. doi: 10.1038/sj.jid.5700701
Frank, R., and Hargreaves, R. (2003). Clinical biomarkers in drug discovery and development. Nat. Rev. Drug Discov. 2, 566–580. doi: 10.1038/nrd1130
Handler, M. Z., Patel, P. A., Kapila, R., Al-Qubati, Y., and Schwartz, R. A. (2015). Cutaneous and mucocutaneous leishmaniasis. J. Am. Acad. Dermatol. 73, 911–926. doi: 10.1016/j.jaad.2014.09.014
Hejazi, S., Hoseini, S., Javanmard, S., Zarkesh, S., and Khamesipour, A. (2012). Interleukin-10 and transforming growth factor-β in early and late lesions of patients with leishmania major induced cutaneous leishmaniasis. Iran J. Parasitol. 7, 16–23.
Hrdinka, M., Dráber, P., Štěpánek, O., Ormsby T, Otáhal, P., et al. (2011). PRR7 is a transmembrane adaptor protein expressed in activated T cells involved in regulation of T cell receptor signaling and apoptosis. J. Biol. Chem. 286, 19617–19629. doi: 10.1074/jbc.M110.175117
Johnson, K. E., and Wilgus, T. A. (2014). Vascular endothelial growth factor and angiogenesis in the regulation of cutaneous wound repair. Adv. Wound Care 3, 647–661. doi: 10.1089/wound.2013.0517
Judith, R., Nithya, M., Rose, C., and Mandal, A. B (2010). Application of a PDGF-containing novel gel for cutaneous wound healing. Life Sci. 87, 1–8. doi: 10.1016/j.lfs.2010.05.003
Kevric, I., Cappel, M. A., and Keeling, J. H. (2015). New world and old world Leishmania infections. Dermatol. Clin. 33, 579–593. doi: 10.1016/j.det.2015.03.018
Kiwanuka, E., Junker, J., and Eriksson, E. (2012). Harnessing growth factors to influence wound healing. Clin. Plast. Surg. 39:239–248. doi: 10.1016/j.cps.2012.04.003
Lakhal-Naouar, I., Slike, B. M., Aronson, N. E., and Marovich, M. A. (2015). The immunology of a healing response in cutaneous leishmaniasis treated with localized heat or systemic antimonial therapy. PLoS Negl. Trop. Dis. 9:e0004178. doi: 10.1371/journal.pntd.0004178
Lima-Junior, D. S., Costa, D. L., Carregaro, V., Cunha, L. D., Silva, A. L., Mineo, T. W., et al. (2013). Inflammasome-derived IL-1β production induces nitric oxide–mediated resistance to Leishmania. Nat. Med. 19, 909–915. doi: 10.1038/nm.3221
Martinez-Subiela, S., Horvatic, A., Escribano, D., Pardo-Marin, L., Kocaturk, M., Mrljak, V., et al. (2017). Identification of novel biomarkers for treatment monitoring in canine leishmaniosis by high-resolution quantitative proteomic analysis. Vet. Immunol. Immunopathol. 191, 60–67. doi: 10.1016/j.vetimm.2017.08.004
Mast, B. A., and Schultz, G. S. (1996). Interactions of cytokines, growth factors, and proteases in acute and chronic wounds. Wound Repair Regen. 4, 411–420. doi: 10.1046/j.1524-475X.1996.40404.x
McGwire, B. S., and Satoskar, A. R. (2014). Leishmaniasis: clinical syndromes and treatment. QJM 107, 7–14. doi: 10.1093/qjmed/hct116
Monge-Maillo, B., and López-Vélez, R. (2013). Therapeutic options for old world cutaneous leishmaniasis and new world cutaneous and mucocutaneous leishmaniasis. Drugs 73, 1889–1920. doi: 10.1007/s40265-013-0132-1
Mougneau, E., Bihl, F., and Glaichenhaus, N. (2011). Cell biology and immunology of Leishmania. Immunol. Rev. 240, 286–296. doi: 10.1111/j.1600-065X.2010.00983.x
Mundy, G. R. (2009). Cytokines and growth factors in the regulation of bone remodeling. J. Bone Miner. Res. 8, S505–S510. doi: 10.1002/jbmr.5650081315
Nieto, A., Domínguez-Bernal, G., Orden, J. A., De La Fuente, R., Madrid-Elena, N., and Carrión, J. (2011). Mechanisms of resistance and susceptibility to experimental visceral leishmaniosis: BALB/c mouse versus syrian hamster model. Vet. Res. 42:39. doi: 10.1186/1297-9716-42-39
Nylén, S., and Eidsmo, L. (2012). Tissue damage and immunity in cutaneous leishmaniasis. Parasite Immunol. 34, 551–561. doi: 10.1111/pim.12007
Okwor, I., and Uzonna, J. (2016). Social and economic burden of human Leishmaniasis. Am. J. Trop. Med. Hyg. 94, 489–493. doi: 10.4269/ajtmh.15-0408
Oliveira, L. F., Schubach, A. O., Martins, M. M., Passos, S. L., Oliveira, R. V., Marzochi, M. C., et al. (2011). Systematic review of the adverse effects of cutaneous leishmaniasis treatment in the New World. Acta Trop. 118, 87–96. doi: 10.1016/j.actatropica.2011.02.007
Papanas, N., and Maltezos, E. (2007). Growth factors in the treatment of diabetic foot ulcers: new technologies, any promises? Int. J. Low Extrem. Wounds 6, 37–53. doi: 10.1177/1534734606298416
Pikuła, M., Langa, P., Kosikowska, P., and Trzonkowski, P. (2015). Stem cells and growth factors in wound healing. Postepy Hig. Med. Dosw. 69, 874–885. doi: 10.5604/17322693.1162989
Ponticos, M. (2013). Connective tissue growth factor (CCN2) in blood vessels cardiovascular biomedicine cardiovascular biomedicine. Vascul. Pharmacol. 58, 189–193. doi: 10.1016/j.vph.2013.01.004
Portela, Á. S. B., Costa, L. E., Salles, B. C. S., Lima, M. P., Santos, T. T. O., Ramos, F. F., et al. (2018). Identification of immune biomarkers related to disease progression and treatment efficacy in human visceral leishmaniasis. Immunobiology 223, 303–309. doi: 10.1016/j.imbio.2017.10.043
Ramirez, H., Patel, S., and Pastar, I. (2013). The role of TGFβ1 signaling in wound epithelialization. Adv. Wound Care. 3, 482–491. doi: 10.1089/wound.2013.0466
Reed, S. G. (1999). TGF-β in infections and infectious diseases. Microbes Infect. 1, 1313–1325. doi: 10.1016/S1286-4579(99)00252-X
Robson, M. C. (1991). Growth factors as wound healing agents. Curr. Opin. Biotechnol. 2, 863–867. doi: 10.1016/S0958-1669(05)80122-3
Rodrigues, F. M., Coelho Neto, G. T., Menezes, J. G., Gama, M. E., Gonçalves, E. G., et al. (2014). Expression of Foxp3, TGF-β and IL-10 in American cutaneous leishmaniasis lesions. Arch. Dermatol. Res. 306, 163–171. doi: 10.1007/s00403-013-1396-8
Sharma, U., and Singh, S. (2008). Insect vectors of Leishmania: distribution, physiology and their control. J. Vector Borne Dis. 45, 255–272.
Shen, C., Sun, L., Zhu, N., and Qi, F. (2017). Kindlin-1 contributes to EGF-induced re-epithelialization in skin wound healing. Int. J. Mol. Med. 39, 949–959. doi: 10.3892/ijmm.2017.2911
Singh, B., and Coffey, R. J. (2014). From wavy hair to naked proteins: the role of transforming growth factor alpha in health and disease. Semin. Cell Dev. Biol. 28, 12–21. doi: 10.1016/j.semcdb.2014.03.003
Singh, N., Kumar, M., and Singh, R. K. (2012). Leishmaniasis : current status of available drugs and new potential drug targets. Asian Pac. J. Trop. Med. 5, 485–497. doi: 10.1016/S1995-7645(12)60084-4
Singla, S., Singla, S., Kumar, A., and Singla, M. (2012). Role of epidermal growth factor in healing of diabetic foot ulcers. Indian J. Surg. 74, 451–455. doi: 10.1007/s12262-012-0447-2
Sohrabi, Y., Havelkov, Á. H., Kobets, T., Šíma, M., Grekov, I., et al. (2013). Mapping the genes for susceptibility and response to leishmania tropica in mouse. PLoS Negl. Trop. Dis. 7:e2282. doi: 10.1371/journal.pntd.0002282
Soong, L., Henard, C. A., and Melby, P. C. (2012). Immunopathogenesis of non-healing American cutaneous leishmaniasis and progressive visceral leishmaniasis. Semin. Immunopathol. 34, 735–751. doi: 10.1007/s00281-012-0350-8
Steed, D. L. (1997). The role of growth factors in wound healing. Surg. Clin. North Am. 77, 575–586. doi: 10.1097/00005373-199607000-00029
Weiss, M. G. (2008). Stigma and the social burden of neglected tropical diseases. PLoS Negl. Trop. Dis. 2:e237. doi: 10.1371/journal.pntd.0000237
Wolf Nassif, P., De Mello, T. F. P., Demarchi, I. G., Aristides, S. M. A., et al. (2017). Safety and efficacy of current alternatives in the topical treatment of cutaneous leishmaniasis: a systematic review. Parasitology 144, 995–1004. doi: 10.1017/S0031182017000385
Keywords: growth factor, cutaneous leishmaniasis, L. braziliensis, EGF, TGFβI, FGF, PDGF, biomarkers
Citation: Montoya A, Yepes L, Bedoya A, Henao R, Delgado G, Vélez ID and Robledo SM (2018) Transforming Growth Factor Beta (TGFβ1) and Epidermal Growth Factor (EGF) as Biomarkers of Leishmania (V) braziliensis Infection and Early Therapeutic Response in Cutaneous Leishmaniasis: Studies in Hamsters. Front. Cell. Infect. Microbiol. 8:350. doi: 10.3389/fcimb.2018.00350
Received: 26 March 2018; Accepted: 13 September 2018;
Published: 02 October 2018.
Edited by:
Javier Moreno, Instituto de Salud Carlos III, SpainReviewed by:
Herbert Leonel de Matos Guedes, Universidade Federal do Rio de Janeiro, BrazilAna Victoria Ibarra-Meneses, Instituto de Salud Carlos III, Spain
Copyright © 2018 Montoya, Yepes, Bedoya, Henao, Delgado, Vélez and Robledo. This is an open-access article distributed under the terms of the Creative Commons Attribution License (CC BY). The use, distribution or reproduction in other forums is permitted, provided the original author(s) and the copyright owner(s) are credited and that the original publication in this journal is cited, in accordance with accepted academic practice. No use, distribution or reproduction is permitted which does not comply with these terms.
*Correspondence:Sara M. Robledo, sara.robledo@udea.edu.co