- 1School of Pharmaceutical Sciences, Shandong University, Jinan, China
- 2Department of Pharmacy, Feicheng Mining Central Hospital, Feicheng, China
- 3Department of Pharmacy, Qianfoshan Hospital Affiliated to Shandong University, Jinan, China
In recent decades, invasive fungal infections have been increasing significantly, contributing to high incidences and mortality in immunosuppressed patients. Candida albicans (C. albicans) is the most prevalent opportunistic fungal pathogen in humans that can cause severe and often fatal bloodstream infections. Current antifungal agents have several limitations, including that only a small number of classes of antifungals are available, certain of which have severe toxicity and high cost. Moreover, the emergence of drug resistance is a new limitation to successful patient outcomes. Therefore, the development of antifungals with novel targets is an essential strategy for the efficient management of C. albicans infections. It is widely recognized that ion homeostasis is crucial for all living cells. Many studies have identified that ion-signaling and transduction networks are central to fungal survival by regulating gene expression, morphological transition, host invasion, stress response, and drug resistance. Dysregulation of ion homeostasis rapidly mediates cell death, forming the mechanistic basis of a growing number of compounds that elicit antifungal activity. Most of the potent antifungals have been widely used in the clinic, and certain of them have low toxicity, meaning that they may be expected to be used as antifungal drugs in the future. Hence, we briefly summarize the homeostasis regulation of several important ions, potential antifungal targets based on these ion-signaling networks, and antifungal compounds based on the disruption of ion homeostasis. This summary will help in designing effective drugs and identifying new targets for combating fungal diseases.
Introduction
Invasive fungal infections are on the rise around the world, in parallel with increasing populations of immunosuppressed individuals; overprescription of chemotherapeutics, antifungal agents, and steroids; and extensive use of catheters, as well as other medical implants (Tobudic et al., 2012). C. albicans is the most widespread opportunistic fungal pathogen in the human body, causing mucosal, and systemic infections. More importantly, the mortality rate of bloodstream infections caused by Candida species was reported to be as high as 40–60% among immunosuppressed and hospitalized patients (Tobudic et al., 2012; Sun et al., 2015). C. albicans is polymorphic and capable of undergoing reversible morphological transitions between yeast, pseudohyphal, and hyphal growth forms. The hyphal form plays key roles in the infection process, and is coupled with many virulence factors including adherence and secretion of hydrolases (Vila et al., 2017). Biofilm production is related to a high level of antifungal resistance and easily occurs in host tissues, prostheses, and indwelling medical devices (Silva et al., 2017). Fungal-selective targets are insufficient due to the fact that most eukaryotes share similar metabolic pathways and essential cellular machinery with humans (Zhang et al., 2012). At present, antifungal agents are limited to three major classes: the polyenes, which bind fungal cell membrane ergosterol leading to cell lysis; azoles that inhibit ergosterol biosynthesis; and echinocandins that inhibit fungal (1,3)-β-D-glucan cell wall biosynthesis. Although echinocandins have a good safety profile, several of the traditional antifungals, such as itraconazole, voriconazole, and amphotericin B, possess severe toxicity (Zavrel and White, 2015). As a consequence of the wide use of antifungal agents, drug resistance of C. albicans is increasing, which poses a serious threat to antifungal therapy. Therefore, exploring effective antifungal agents with novel drug targets is urgently needed to cope with the challenges that the antifungal area faces (Guo X. et al., 2008).
Ions play a vital role in various living organisms. In C. albicans, ions could participate in membrane potential maintenance, cell volume regulation, cofactors formation of multiple enzymes, proliferation, and apoptosis (Yun and Lee, 2017). Additionally, ionic signal transduction network is a central cellular pathway that has received extensive attention in recent years. Moreover, studies identified that ion homeostasis was closely connected with oxidative stress response, morphogenesis, antifungal drug resistance, cell wall integrity, and invasive growth in C. albicans, which reveal the potential for defining novel drug targets (Yu et al., 2014c; Loboda and Rowinska-Zyrek, 2017; Yun and Lee, 2017). Several studies have illustrated that dysregulation of ion homeostasis could rapidly mediate fungal growth and virulence (Davis, 2009; Lew, 2011). Therefore, strategies that target or manipulate ion homeostasis regulation may pave the way for novel antifungal agents. In the following, we primarily review the genes, proteins, and enzymes involved in the regulation of ion homeostasis, including hydrogen (H+), calcium (Ca2+), iron (Fe3+), zinc (Zn2+), potassium (K+), and sodium (Na+) in C. albicans. In addition, the potential antifungal targets based on ion homeostasis are summarized. In this review, we provide recent advances in this field, and the summary is presented in the form of figures and tables. Figure 1 illustrates the ion homeostasis regulatory pathways and potential antifungal targets on the basis of ion signal networks. Figure 2 highlights the link between specific ion homeostasis and fungal survival. Table 1 illuminates the compounds that exhibit antifungal activity by disrupting ion homeostasis.
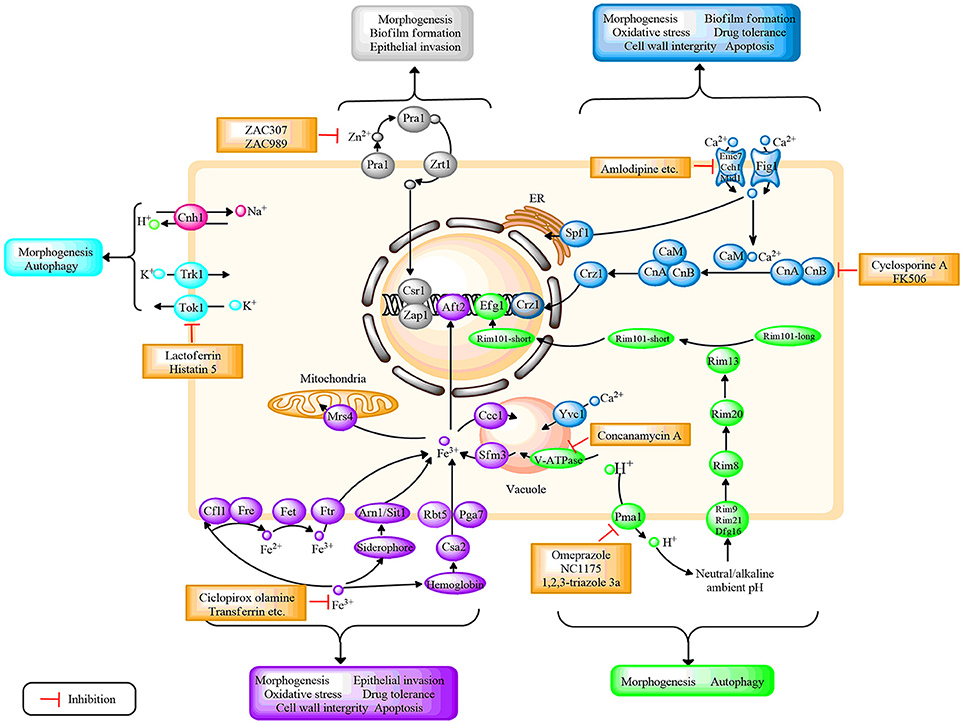
Figure 1. Schematic diagram depicting the regulation of different ion systems, their role in fungal growth, as well as the potential antifungal targets based on ion signaling pathways in C. albicans. Different ions correspond to different colors, H+(green); Ca2+(mazarine); Fe3+(purple); Zn2+(gray); K+(blue); and Na+ (pink). CaM, calmodulin; CnA, the a subunit of calcineurin; CnB, the b subunit of calcineurin. Further details are provided in the text.
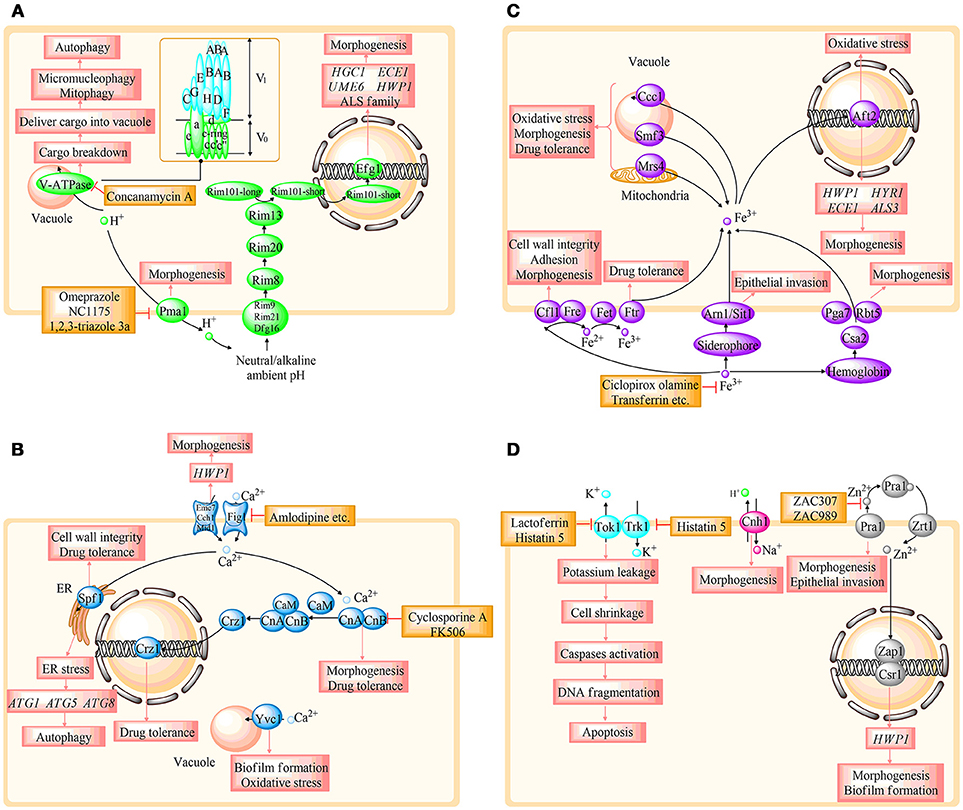
Figure 2. Overview of the relationship between the specific protein and fungal survival, tolerance, and virulence in different ion regulating systems. (A–C) represent H+, Ca2+, and Fe3+ homeostasis, respectively. (D) involves the homeostasis of Zn2+, K+, and Na+. HGC1, ECE1, UME6, HWP1, HYR1, and HYR1, hypha-specific genes; ALS, agglutinin-like sequence; CaM, calmodulin; CnA, the a subunit of calcineurin; CnB, the b subunit of calcineurin; ATG, autophagy-related genes. The details are shown in the text.
H+ Homeostasis and Potential Antifungal Targets
H+ Homeostasis Regulation
The pH of a solution refers to the negative logarithm of the H+ concentration.(Orij et al., 2011) It is widely recognized that the tight control of pH is a necessary requirement for the viability of all eukaryotic cells. Different organelles should maintain at distinct pH for multiple cellular events involving protein conformation, pH gradient establishment, and the regulation of enzymes activity (Kane, 2016). Fungi have evolved a number of strategies to accommodate its need of maintaining stable pH in response to rapid metabolism and a dramatically changing extracellular environment (Kane, 2016).
The pH homeostasis is primarily controlled by three types of components including pumps, exchangers, and buffers in all viable cells (Casey et al., 2010). As shown in Figure 2A, Pma1 (plasma membrane H+-ATPase) and V-ATPase are key regulators of intracellular pH that utilize the energy from ATP hydrolysis to transport proton out of the cytoplasm (Orij et al., 2011). Pma1 is thought to be the dominant proton pump of cytosolic pH, which belongs to the P-type H+-ATPase (Serrano et al., 1986). The proton pump is a 100-kD electrogenic, polytopic integral membrane protein with the N- and C- terminal transmembrane domains in the cytoplasm (Kane, 2016; Manzoor, 2016). Pma1 plays a crucial role in numerous ways such as creating the transmembrane electrochemical proton gradients that are necessary for nutrients and ions uptake and regulating cell growth and dimorphism in C. albicans (Khan et al., 2012; Kane, 2016). V-ATPase is another master regulator of pH that is highly conserved among all eukaryotic cells, and it is able to acidify intracellular organelles involving vacuole, endosomes, and Golgi apparatus (Kane, 2016). The substructure of V-ATPase is also illuminated in Figure 2A. This multisubunit enzyme is consisted of 14 subunits and divided into two domains. V1 complex, the peripheral membrane subunits responsible for hydrolyzing ATP, is making up of eight subunits from V1A to V1H (Olsen, 2014). V0 domain is the integral membrane proteins acting as a proton transporter, which contains six subunits known as a, c, c′, c”, d, e (Raines et al., 2013; Olsen, 2014; Kane, 2016). V0a is the only subunit composed by two homologs, with Vph1 located on the vacuole, whereas Stv1 is cycling between Golgi and endosomes (Finnigan et al., 2012). H+ gradients established by V-ATPase are essential for a diverse range of cellular functions such as in vacuole acidification, degradation of enzyme function, sequestration of toxic metal ions, receptor–ligand binding, and cargo sorting during endocytic and secretory pathways (Poltermann, 2005; Zhang and Rao, 2012; Olsen, 2014). Due to the prime regulation of Pma1 and V-ATPase cooperatively, intracellular pH could maintain the dynamic homeostasis, which ensures the normal development of cells.
Potential Antifungal Targets Related to H+ Homeostasis
In C. albicans, optimum pH has been implicated in various virulence pathways involving a dimorphic switch and proteinases and lipases secretion (Patenaude et al., 2013; Raines et al., 2013). As the primary regulator of cytosolic pH, Pma1 could affect the dimorphic transition, which is essential for the host invasion and tissue damage (Kaur and Mishra, 1991; Seto-Young et al., 1997). Simminder Kaur et al. reported that C. albicans failed to form germ tubes and hyphae in the presence of Pma1 inhibitor orthovanadate (Kaur et al., 1988; Stewart et al., 1989). In addition, Pma1 plays a pivotal role in excreting protons to acidify the external environment, thereby, activating acid-activated proteases and lipases, which facilitates the penetration of C. albicans into host cells (Perlin et al., 1997). Patricia Soteropoulos et al. demonstrated that antagonists of plasma membrane H+-ATPase might exhibit broad-spectrum activity for the high similarity of sequence among these enzymes in diverse pathogenic fungi (Soteropoulos et al., 2000). Therefore, we can conclude that Pma1 could be developed as a surface-mediated and broad-spectrum antifungal target with new mechanisms for its unique structure and function (Monk et al., 2005; Manzoor, 2016).
V-ATPase is a critical pH regulator that participates in multiple virulence-related processes, including stress response, biofilm formation, morphology transition, host tissue invasion, and host immune response in C. albicans as shown in Figure 2A (Zhang and Rao, 2012; Patenaude et al., 2013; Raines et al., 2013). The pH gradient created by V-ATPase is required for the secretion of numerous virulence-related proteins, such as aspartyl proteases, lipases, adhesions, and invasins, in the secretory pathway, which assists C. albicans in the invasion and colonization to host cells (Zhang and Rao, 2012). In addition, V-ATPase is needed for the autophagic degradation processes that require an acid lumen environment to stimulate the enzymes responsible for various cargos degradation. Dalibor Mijaljica et al. speculate that functional V-ATPase is indispensable for the final step of autophagic processes between cargo breakdown and delivery into the vacuolar in micronucleophagy and mitophagy autophagy pathways (Yu et al., 2008; Kanki et al., 2009). In addition to the virulence-related roles of V-ATPase mentioned earlier, further studies have been conducted to reveal the specific function of V-ATPase subunits that associate with C. albicans virulence. V0a subunit is composed of Vph1 and Stv1; disruption of Vph1 could result in reduced activity of aspartyl protease and lipase, as well as filamentation defects (Raines et al., 2013; Rane et al., 2014). Moreover, Cassandra Patenaude et al. identified that Stv1 was associated with the secretion of adhesion-related proteins (Patenaude et al., 2013). V1B subunit is encoded by VMA2, and inhibition of the expression of VMA2 leads to poor tolerance in oxidative response, temperature response, and stress response (Kane, 2006; Rane et al., 2014). VMA3 encodes V0c subunit, and it is required for hyphae formation (Rane et al., 2013; Olsen, 2014). No orthologs of V0c' subunit exist in mammals; thus, this fungal-specific subunit possesses great potential to make it a desirable target for antifungal drug discovery (Raines et al., 2013; Rane et al., 2013). Moreover, VMA5 is responsible for encoding the V1C subunit, which could not only affect hyphae formation and degradative enzymes secretion, but also be involved in the regulation of calcium concentration and maintenance of intracellular reactive oxygen species (ROS) (Zhang et al., 2017). Consequently, V-ATPase could serve as selective targets for antifungal drug development.
In addition to the intracellular pH, the ambient pH is another crucial factor that has a great impact on the morphological transition of C. albicans (Davis et al., 2000a). C. albicans is generally present in yeast form in acidic conditions, while in hyphae form under alkaline or neutral conditions, and this pH-response pathway is mediated by the transcription factor Rim101 (Davis, 2009; Cornet and Gaillardin, 2014). As depicted in Figure 2A, Dfg16, Rim9, and Rim21 are three receptor proteins located on the plasma membrane that act as environmental pH sensors. With the help of Rim8 and Rim20, the pH-changing signal could transmit to Rim13, which cleaves Rim101 precisely by identifying the enzymatic site on Rim101. Short-Rim101 is the active form that induces EFG1 expression, regulating hyphae-specific genes such as HGC1, ECE1, UME6, HWP1 as well as the agglutinin-like sequence (ALS) family, which is essential for adherence, biofilm formation, and host cell invasion (Fan et al., 2013; Cornet and Gaillardin, 2014). Dana Davis et al. confirmed that Rim101 pH response pathway could not only induce hyphae formation, but is also involved in virulence and pathogenicity in vivo (Davis et al., 2000a,b). Muriel Cornet et al. have demonstrated that all mutants in this pathway showed hypersensitivity to triazoles (fluconazole, voriconazole, and posaconazole) (Cornet and Gaillardin, 2014). Given that the Rim101 pH response pathway is essential for virulence, we can conclude that it is possible to exploit the Rim101 pH response pathway for new antifungal strategies.
Antifungal Agents Based on H+ Homeostasis
H+ homeostatic pathways are key regulators in C. albicans pathogenesis. Table 1 shows certain natural and synthetic compounds that exhibit an inhibitory effect against C. albicans by disrupting H+ homeostasis. Several studies demonstrated that the proton pump inhibitor, omeprazole, could inhibit C. albicans cell growth by restraining the activity of Pma1 (Monk et al., 1995; Perlin et al., 1997; Seto-Young et al., 1997). Activated lansoprazole is a novel benzimidazole proton pump inhibitor, which assists in the inhibition of hyphal growth (Monk et al., 1995; Perlin et al., 1997; Biswas et al., 2001). Furthermore, NC1175 and 1,2,3-triazole 3a are synthetic compounds that exhibit inhibitory activity against C. albicans by restraining the acidification of external medium with an minimum inhibitory concentration (MIC) of 25 μg/mL (Manavathu et al., 1999; Irfan et al., 2017). Additionally, concanamycin A, the most commonly used V-ATPase inhibitor, displays antifungal properties especially against C. albicans, and the MIC of concanamycin A is 25 μg/mL (Okoli et al., 2009). The accumulating knowledge may provide a novel avenue for antifungal drug discovery.
Ca2+ Homeostasis and Potential Antifungal Targets
Ca2+ Homeostasis Regulation
Calcium is one of the essential divalent ions, which is necessary for the growth of various eukaryotes (Cyert and Philpott, 2013). Furthermore, Ca2+ is a ubiquitous messenger that participates in the translation between various developmental signals and specific cellular responses. To serve its signaling function, a low cytosolic Ca2+ concentration must be maintained between 50 and 200 nM, which is achieved by the cooperation of various Ca2+ exchangers, pumps, and channels under specific conditions. Disruption of calcium homeostasis will lead to the growth defect and even cell death of all organisms (Liu et al., 2015).
Calcium cell survival (CCS) pathway (as described in Figure 2B) is the major calcium-signaling pathway that mediates cell survival under various environmental stimulates. The CCS pathway consists of four functional proteins, including the plasma membrane Ca2+ channel-containing Cch1 and Mid1, calmodulin (CaM), and calcineurin (CN). In the CCS pathway, environmental Ca2+ is first transferred into the cytoplasm through the calcium influx channel, the increased Ca2+ concentration results in the activation of CaM followed by the activation of calcium/CaM -dependent phosphatase CN. The transcription factor Crz1 is dephosphorylated by CN, leading to the translocation of Crz1 from the cytoplasm to the nucleus, which activates the expression of the various calcium-related genes (Thewes, 2014). Yeasts possess both high- and low-affinity systems for Ca2+ influx through the plasma membrane. The high-affinity calcium influx system (HACS) consists of three proteins containing Mid1, Cch1, and Ecm7. While the low-affinity calcium influx system (LACS) is the second and less well-defined Ca2+ influx system, displaying a 16-fold lower Ca2+ affinity than HACS, and FIG.1 is the core component of LACS (Harren and Tudzynski, 2013). In yeast, CaM is a ubiquitous and necessary protein that is required for the activation of CN. The CN is a highly conserved protein phosphatase, which consists of two subunits involving the catalytic subunit (encoded by CNA1, CNA2) and the regulatory subunit (encoded CNB1) (Cyert and Philpott, 2013).
In addition to the CCS pathway, there are certain components that are related to the Ca2+ release from cellular calcium pools. The vacuole is the primary Ca2+ storage organelle, which contains nearly 90% of the total intracellular Ca2+, and the effect of sequestrating intracellular Ca2+ mainly exerts through the H+/ Ca2+ exchanger Vcx1, as well as the P-type Ca2+-ATPase Pmc1. Vcx1 plays the dominant role in short-term Ca2+ dynamics,which utilizes the H+ gradient produced by V-ATPase to transport Ca2+ into the vacuole coupled with the H+ efflux into the cytoplasm. Furthermore, Yvc1 is a member of the transient receptor potential (TRP) channels with the primary function of mediating the release of Ca2+ stored in the vacuole (Cyert and Philpott, 2013; Yu et al., 2014c,d). Moreover, the endoplasmic reticulum (ER) is the major organelle responsible for protein folding and secretion; the Ca2+ homeostasis of the ER is primarily governed by the P-type ATPase Spf1, whose homolog is ScCod1/ScSpf1 in Saccharomyces cerevisiae (Yu et al., 2013b, 2015). In conclusion, diverse elements maintain the Ca2+ homeostasis cooperatively, aiming to regulate the growth and metabolism of cells.
Potential Targets Related to Ca2+ Homeostasis
Calcium, as a critical second messenger in eukaryotic cells, plays a direct role in the signal transduction and cellular responses. Moreover, calcium is closely related to the regulation of stress response, morphogenesis, drug tolerance, and cell wall integrity in C. albicans. Thus, calcium is of key importance to maintain the optimal level of intracellular Ca2+ (Yu et al., 2012a; Li and Sun, 2016). The CCS pathway is the central calcium transduction pathway, which is tightly connected with the virulence of C. albicans. Qilin Yu et al. confirmed that Cch1 and Mid1 were involved in the formation and maintenance of hyphae, oxidative stress response, and invasive growth in Candida albicans. Mutants deleted of CCH1 and MID1 showed reduced virulence in a murine model of infection. Moreover, mutants defected of Cch1 or Mid1 function were highly hypersensitive to azoles, indicating that CCS pathway is crucial for cell survival in azole therapies (Yu et al., 2012a). Xiaohui Ding et al. proved that Ecm7, a regulator of HACS, functions in the maintenance of intracellular redox homeostasis and hyphal formation in C. albicans. The mutants lacking ECM7 resulted in ROS accumulation in the presence of H2O2 and decreased expression of the hyphae-specific gene HWP1 (Ding et al., 2013). Calcineurin is highly associated with virulence and drug resistance in a diverse group of pathogenic fungi, such as Cryptococcus neoformans, C. albicans, and a number of plant fungal pathogens (Juvvadi et al., 2017). Many studies have shown that CN and its downstream target Crz1 are responsible for the transition between morphological states, stress responses, cell wall integrity, and tolerance to antifungal agents, such as azoles, terbinafine, and echinocandins (Sanglard et al., 2003). The CN plays a critical role in cell wall integrity pathways, which contributes to cell survival in the host environment (Sanglard et al., 2003; Juvvadi et al., 2017). Moreover, Thewes et al. confirmed that CN is essential in a murine model of infection, whereas Crz1 has a moderate effect on virulence (Karababa et al., 2006; Thewes, 2014). In conclusion, the CCS pathway provides attractive targets for the exploitation of potent antifungal agents.
In addition to the CCS pathway, regulation of calcium concentrations by intracellular organelles also has a profound effect on C. albicans survival. Spf1, the P-type ATPase located on the ER, is associated with autophagy, cell wall integrity, drug resistance, and biofilm formation in C. albicans (Yu et al., 2012b, 2013b, 2015). Qilin Yu et al. speculate that there is a possible correlation between autophagy and ER stress, and the deficiency of Spf1 function leads to the disorder of calcium homeostasis and ER stress, which leads to the increased autophagic activity and upregulation of autophagy-related (ATG) genes including ATG1, ATG5, and ATG8 (Yu et al., 2015). In addition, Qilin Yu et al. demonstrated that spf1Δ/Δ mutant showed hypersensitivity to cell wall stress and abnormal cell wall organization, suggesting that the mutant is deficient in cell wall integrity, which is essential for the virulence of C. albicans. Moreover, the spf1Δ/Δ mutant showed significant hyphae and biofilm formation defects in hyphae-inducing media (Yu et al., 2012b). Yvc1 is a member of vacuolar locating TRP channel family, which plays a significant role in fighting against oxidative stress (Yu et al., 2014d). Additionally, Qilin Yu et al. suggested that disruption of Yvc1 function resulted in attenuated virulence of mouse models and human epithelial cells. Yvc1 is necessary for hypha-associated gene expression during hyphal elongation and maintenance, while it is dispensable for hyphal formation (Yu et al., 2014c). In summary, disruption of Ca2+ homeostasis should improve the development of antifungal agents and provide alternatives for the treatment of C. albicans infection.
Antifungal Agents Based on Ca2+ Homeostasis
Studies revealed that disruption of Ca2+ homeostasis by specific compounds not only regulates cell survival, but also influences critical functions for the pathogenesis of C. albicans. Several reports have shown that certain agents could inhibit the growth of C. albicans by disrupting Ca2+ homeostasis, as shown in Table 1. Ethylenediaminetetraacetic acid (EDTA) and ethylene-bis(oxyethylenenitrilo)tetraacetic acid (EGTA) are two calcium chelators that possess antifungal activity contributing to their property of calcium chelating (Ates et al., 2005). Moreover, Yaojun Tong et al. identified that beauvericin had fungicidal activity in vitro by elevating intracellular calcium and ROS, and the MIC90 of beauvericin was 8 μg/mL (Tong et al., 2016). Particularly, mitochondrial Ca2+ overload plays a crucial role in the process of apoptosis. Silibinin could induce the activation of pro-apoptotic factors of C. albicans (Yun and Lee, 2016). Traditional antifungal agents could affect cell survival directly by interfering with ergosterol or the cell wall. Recent studies have shown that some drugs can produce antifungal activity by inhibiting virulence-related factors, such as adhesion, biofilm formation, and so on. It is worth noting that certain non-antifungal agents have significant synergetic effects when combined with traditional antifungal agents. Priya Uppuluri et al. reported that C. albicans biofilms are hypersensitive to CN inhibitor–fluconazole combinations. Cyclosporine A (CsA) and tacrolimus (FK506) are immunosuppressive drugs, which inhibit the activity of CN and possess dramatic synergistic effect against resistant C. albicans along with fluconazole treatment. The mechanisms of the synergistic effects are related to the inhibition of several virulence factors including cell adhesion, hyphal formation, and the downregulation of ALS3, HWP1, CDR1, MDR, ERG11 gene expressions (Juvvadi et al., 2017). Moreover, several studies have demonstrated that verapamil showed an inhibitory effect against C. albicans when used alone or in combination with fluconazole, and the synergistic effect was closely associated with biofilm development and adherence (Yu et al., 2013a, 2014a). Additionally, Shuyuan Liu et al. identified that several calcium channel blockers (amlodipine, nifedipine, benidipine, and flunarizine) showed a significant synergetic effect on resistant C. albicans when combined with fluconazole. The expressions of CNA1, CNB1, and YVC1 were downregulated when fluconazole was combined with amlodipine. Although amlodipine has certain side effects, it is currently used as an antihypertensive drug in clinical practice. Despite its limitation, the result of these data will provide new insights for the development of novel antifungal drugs with novel mechanisms (Liu et al., 2016). Studies have demonstrated that the antiarrhythmic drug amiodarone showed a synergistic effect against C. albicans, and the antifungal activity of amiodarone is mediated by the disruption of calcium homeostasis. Zhang et al. evaluated the clinical potential of combining fluconazole and amiodarone in treating fungal infections in a murine Candidiasis model. The microbial burden of C. albicans in kidneys was significantly reduced in the combination group (Gupta et al., 2003; Guo Q. et al., 2008; Zhang et al., 2010). In conclusion, blocking the calcium signaling pathway by specific inhibitors is tightly associated with cell survival, and further understanding of the calcium-related mechanisms of virulence and pathogenicity should provide novel ideas to the development of antifungal agents.
Fe3+ Homeostasis and Potential Antifungal Targets
Fe3+ Homeostasis Regulation
Iron is an essential nutrient as well as the most abundant trace metal in all eukaryotic cells. Iron is capable of converting between ferrous and ferric forms (Bairwa et al., 2017). The redox characteristic of iron plays a crucial role in carrying out vital cellular functions in many central metabolic pathways such as DNA synthesis, respiration, electron transport chains, oxygen transport/storage, and tricarboxylic acid cycle (Bairwa et al., 2017). However, iron can be toxic as it catalyzes the production of ROS that can severely damage biological molecules including nucleic acids, proteins, and lipids (Balhara et al., 2016; Malavia et al., 2017; Mamouei et al., 2017; Gerwien et al., 2018). Hence, all eukaryotes have developed exquisite iron-acquisition strategies to maintain the dynamic balance between cell growth and cytotoxicity.
The majority of iron in host cells is bound to iron-binding proteins such as hemoglobin, ferritin, and transferrin, with virtually no free iron in circulation. In the host environment, fungi such as C. albicans have evolved a sophisticated iron uptake regulatory network as described in Figure 2C (Malavia et al., 2017; Gerwien et al., 2018). There are three significant iron acquisition systems, including reductive iron uptake system, siderophore uptake system, and hemoglobin uptake system in C. albicans. Reductive iron uptake system is especially crucial in non-siderophore-producing C. albicans, which contributes to its growth and virulence (Gerwien et al., 2016). The bound Fe3+ that is extracted from ferric salts and ferric chelates is first reduced to Fe2+ via ferric reductases Cfl1 and Fre. Fe2+ is then oxidized by permease-coupled multicopper ferroxidases Fet, followed by Fe3+ transport into the intracellular space via the high-affinity permeases Ftr (Bairwa et al., 2017). Cfl1 was the first identified ferric reductase in C. albicans, which could rescue the growth defect in S. cerevisiae fre1 mutant (Xu et al., 2014b; Yu et al., 2014b). Moreover, the ferroxidase is encoded by five genes(FET3, FET31, FET33, FET34, FET99), and four permease genes are annotated as FTR1, FTR2, FTH1, and FTH2. Researchers have shown that C. albicans possesses multiple high-affinity iron transporters formed by a permease associated with a ferroxidase specifically (Muzzey et al., 2013; Mamouei et al., 2017). The second iron uptake system is the siderophore uptake system. Siderophore, as the central part of the siderophore uptake system, is a class of small molecules secreted by bacteria and fungi, which is capable of binding with extracellular Fe3+ with extremely high affinity (Gerwien et al., 2018). The iron transport process is followed by the combination of the Arn1/Sit1 siderophore transporter. C. albicans could utilize the siderophores secreted by other species although they are not siderophore producers (Bairwa et al., 2017; Gerwien et al., 2018). Hemoglobin uptake system is another critical iron uptake system, which is an essential iron source for C. albicans. Approximately 80% of iron inside the mammalian host is sequestered within host iron-binding proteins, and this is the reason why hemoglobin uptake system is necessary for iron acquisition in C. albicans. It has been speculated that the members of the C. albicans heme-receptor protein family possess the common in several fungal extracellular membranes (CFEM) domain involving Csa2, Pga7, and Rbt5, as illustrated in Figure 2C. The Csa2 functions as a secreted hemophore to deliver the hemoglobin to other CFEM proteins, such as Rbt5 and Pga7 (Nasser et al., 2016). Both Rbt5 and Pga7 are able to efficiently extract heme from hemoglobin, while Rbt5 shows a higher affinity and a higher amount (Malavia et al., 2017). Iron acquisition from the host environment is precisely regulated by the iron uptake systems, which ensures C. albicans cell growth.
In addition to the iron uptake systems, intracellular iron homeostasis also requires the regulation of several elements inside the cell. As shown in Figure 2C, the Mrs4–Ccc1–Smf3 pathway is composed of three iron transporters located on the intracellular organelles and plays a crucial role in the regulation of intracellular iron homeostasis (Xu et al., 2014a). Mrs4, the mitochondrial carrier proteins, facilitates the transport of iron across the mitochondrial inner membrane, as well as maintaining the mitochondrial morphology (Froschauer et al., 2009). Ccc1 is an iron transporter located at the vacuolar, and is involved in the sequestration of iron from the cytosol to the vacuole at the time when the media contains adequate amounts of iron (Li et al., 2001). Smf3 is the homolog of the Nramp family that helps to mobilize vacuolar stores of iron (Portnoy et al., 2000). Additionally, fungi have developed various iron-regulated systems to adjust cell requirements under diverse host environment. In S. cerevisiae, Aft1 and Aft2 are two iron-regulatory activators whose functions are involved in the transcription of iron uptake and metabolism-related genes (Goncalves et al., 2014). Ning Xu et al. have identified that C. albicans Aft2 is the ortholog of S. cerevisiae Aft1/Aft2 regulators, which is tightly associated with the expression of iron-related genes (Liang et al., 2010). We could summarize that the regulation of iron homeostasis is particularly sophisticated and plays a crucial role in C. albicans cell survival.
Potential Targets Related to Fe3+ Homeostasis
Several studies have implied that iron availability may play a significant role in signaling or facilitating the transition from commensal to pathogenic in C. albicans. The efficient iron acquisition composes necessary virulence factors and is involved in the invasion of the host epithelium and morphological transition (Spacek et al., 2005). The reductive iron uptake system in C. albicans is coordinated with adhesion, filamentation, antifungal drug resistance, cell wall integrity, and mitochondrial function (Jeeves et al., 2011; Yu et al., 2014b; Mamouei et al., 2017). The ferroxidases must cooperate with each other to support the full virulence. Fet shows an effect on cell growth, hyphal development, and virulence. Disruption of FET99 results in a clear growth deficiency. Moreover, loss of FET34 impedes the virulence of C. albicans in a mouse model of system infection, and the mutant exhibits a filamentous growth defect (Cheng et al., 2013; Mamouei et al., 2017). Cfl1 plays a significant role in the maintenance of the cell wall architecture, mitochondrial function, filamentous growth, and oxidative response in C. albicans. Disruption of CFL1 results in ROS accumulation. Furthermore, Cfl1 dysfunction leads to the defect in cell wall integrity (Xu et al., 2014b; Yu et al., 2014b). Siderophore uptake system is mediated by Sit1/Arn1 and is required for the epithelial invasion and penetration caused by C. albicans infection (Heymann et al., 2002). The hemoglobin uptake system is the third host-iron assimilation pathway, which is expressed not only during mouse model infections, but also in candidemia patients. Kazuko Okamoto-Shibayama et al. suggested that hemoglobin is a source of iron, which has an impact on the hyphal morphogenesis in C. albicans (Okamoto-Shibayama et al., 2014).
The Mrs4–Ccc1–Smf3 (MCS) pathway plays a vital role in maintaining intracellular iron homeostasis and is closely related to cell survival. Ning Xu et al. have demonstrated that the mitochondrial dysfunction caused by the MCS pathway dysregulation had a tremendous effect on various physiological processes, such as oxidative stress response, cell-wall stability, colony morphology, and filamentous growth (Xu et al., 2012, 2014a). Aft2 is the most important regulator of iron metabolism-related genes, and it also shows an impact on virulence, invasive growth, oxidative response, and morphology transition. Deletion of AFT2 leads to the accumulation of ROS and dramatically elevated superoxide dismutase activity under H2O2 treatment. Moreover, aft2Δ/Δ mutant also downregulates the transcript levels of hypha-specific genes involving HWP1, HYR1, ECE1, and ALS3, which have an effect on invasive growth in C. albicans. The information on iron homeostasis regulation sets the stage for a consideration of approaches to exploit novel antifungal targets.
Antifungal Agents Based on Fe3+ Homeostasis
The accumulating information provides opportunities to exploit iron acquisition for antifungal therapy, and new work highlights the development of iron chelators for therapeutic use alone or in conjunction with existing antifungal drugs. Table 1 lists the compounds that possess antifungal activity by disrupting iron homeostasis. Transferrin could restrict C. albicans growth in vitro due to its capacity of iron sequestration and membrane potential disruption, with an MIC80 of 2.0 μg/mL (Lin et al., 2014). Moreover, Markus Niewerth et al. identified that ciclopirox olamine could act as an iron chelator which in turn, influences iron metabolism and therefore reduces cell growth (Niewerth et al., 2003). Certain plant compounds and their derivatives also exhibit the properties of inhibiting the growth of C. albicans by disturbing iron homeostasis; these plant-derived compounds include eupolauridine and geraniol. Moreover, geraniol inhibits virulence factors that attribute to hyphal morphogenesis, biofilm formation, and cell adhesion (Meleddu et al., 2016; Singh et al., 2016; Tripathi et al., 2017). In addition, iron depletion in C. albicans with bathophenanthroline, disulfonic acid, and ferrozine as chelators enhanced its sensitivity to traditional antifungal agents (Prasad et al., 2006). In conclusion, compounds that block iron availability can effectively fight against C. albicans; this strategy represents a novel insight in the development of antifungal agents.
Zn2+ Homeostasis and Potential Antifungal Targets
Zn2+ Homeostasis Regulation
Zinc is the second-most abundant trace metal and is shown to be a second messenger that participates in various intracellular transduction signaling pathways. Zinc is indispensable in enzymes such as superoxide dismutases and metalloproteases that are crucial for the virulence and survival of fungal pathogens in host cells (Yike, 2011). Moreover, zinc-binding proteins are involved in transcription regulation via different zinc finger transcription factors in C. albicans (Loboda and Rowinska-Zyrek, 2017). However, because the concentration of free Zn2+ is as low as sub-nanomolar, excess zinc shows toxicity by binding to proteins inappropriately. Therefore, fungi have evolved sophisticated mechanisms to control intracellular zinc homeostasis tightly.
Zinc homeostasis regulation is shown in Figure 2D. The uptake of zinc from the host environment takes place mainly via two ZIP transporters in C. albicans. Zrt1 provides the high-affinity zinc uptake activity, while Zrt2 shows lower zinc affinity than Zrt1 (Kim et al., 2008). In addition to the Zrt membrane transporter, C. albicans is able to secrete an extracellular zinc-binding protein Pra1. This process is followed by the delivery to the Ztr1 transporter. The Pra1–Zrt1 zincophore transport system is more effective under zinc depletion and an alkaline environment (Gerwien et al., 2018). Moreover, zinc homeostasis is largely achieved by the expression of a zinc-related transcriptional factor. In yeast, Zap1 is the major zinc-sensing transcriptional factor that activates more than 80 genes' transcription including ZRT1, ZRT2 in response to zinc deficiency (Wu et al., 2008; Gerwien et al., 2018). Kim et al. have demonstrated that Csr1 is another functional zinc-responsive transcription factor, which shows a high similarity to Zap1 according to the amino acid sequence analysis (Kim et al., 2008).
Potential Targets Related to Zn2+ Homeostasis
It is well-known that zinc takes part in various intracellular physiological and metabolic processes. In contrast, far less is known concerning how zinc homeostasis influences eukaryotic pathogens virulence. Several studies have suggested that zinc homeostasis plays diverse roles in the morphological transition, biofilm formation, and virulence of C. albicans (Figure 2D) (Lehtovirta-Morley et al., 2017). Zrt1 is associated with cell growth and ZRT1 disruption results in growth defects in the neutral alkaline zinc-limited medium (Citiulo et al., 2012). Moreover, both Pra1 and Zrt1 were found to be upregulated in order to uptake adequate zinc in epithelial cells and liver infection models (Xu et al., 2015). Francesco Citiulo et al. suggested that the zincophore Pra1 mediates endothelial damage by scavenging host zinc because deletion of PRA1 showed significantly shorter hyphae than the wide type (Citiulo et al., 2012). Previous studies have shown that Zap1 could govern proliferation in a pathway that operates in the invasive infection environment. In addition, Zap1 has also been identified as a hypha-formation regulator that governs the balance of yeast and hyphal cells in biofilms. Csr1 is the ortholog of Zap1 in C. albicans, which not only controls zinc homeostasis, but also contributes to the filamentation and biofilm elaboration. Kim et al. have demonstrated that the transcriptional level of the hypha-related gene HWP1 was significantly inhibited in the CSR1 deletion mutant (Kim et al., 2008). Taken together, zinc homeostasis provides a promising step in the advancement of antifungal drug development.
Antifungal Agents Based on Zn2+ Homeostasis
In recent years, the importance of zinc homeostasis has been highlighted due to its vital contribution to fungal pathogenesis and virulence. Researchers identified two novel zinc-attenuating compounds (ZACs) ZAC307 and ZAC989, which showed antifungal activity against C. albicans by chelating zinc in vitro (O'Hanlon Cohrt et al., 2018). ZAC307 and ZAC989 have a high-binding affinity for zinc. As shown in Table 1, the MIC50 of ZAC307 and ZAC989 are 0.6 and 0.4 μg/mL, respectively. Moreover, Karen et al. reported that ZAC307 and ZAC989 exhibited in vivo efficacy in a murine fungal kidney burden candidiasis model. In summary, this series of ZACs represents a potentially new class of antifungal agents, and interfering with fungal zinc-dependent processes represents a promising new approach to antifungal therapy.
Others
K+ Homeostasis and Cell Survival
Potassium exists at higher concentrations (200–300 mmol/L) in Candida species, and the sufficient amount of potassium is essential for various cell functions, such as regulating cell volume and pH, compensating negative charge, and maintaining a membrane potential, and activation of key metabolic processes involving protein synthesis and stabilization (Ariño et al., 2010).
As shown in Figure 2D, Tok1 is the only certified K+-specific channel in Candida plasma membranes, which is activated by membrane depolarization and may be the principal voltage stabilization pathway in yeast cells. Although the sequence motifs of K+ channels are similar between fungi and mammals, Tok1 may also be regarded as attractive pharmacological targets because the fungal homologs of Tok1 possess the unique topological structure and gating mechanism (Loukin et al., 2002; Prole and Taylor, 2012).
Cell shrinkage is one of the most obvious features of apoptosis, and numerous studies have shown that the release of potassium is closely linked to the cell shrinkage for the reason that K+ is the major determinant of cell volume (Bortner and Cidlowski, 2007). Moreover, enhanced K+ efflux has been shown to mediate the downstream caspases activation and DNA fragmentation, which lead to apoptosis (Remillard and Yuan, 2004). As listed in Table 1, several studies have confirmed that some compounds can induce apoptosis of C. albicans by promoting the outflow of K+. Chlorogenic acid and Cassia spectabilis caused excessive potassium efflux, followed by apoptotic volume decrease, caspase activation, and DNA fragmentation (Torey et al., 2016; Yun and Lee, 2017). Andres et al. verified the role of potassium channels in the apoptosis of C. albicans. Cell death induced by human lactoferrin may be associated with a release of high concentrations of potassium through Tok1 channel (Andrés et al., 2008). Furthermore, studies have reported that Trk1 and Tok1 are critical transporters in the killing of Candida by the oral antimicrobial peptide, histatin 5 (Baev et al., 2003; Han et al., 2016).
Na+ Homeostasis and Cell Survival
K+ is essential in many important physiological roles as described earlier. In contrast, Na+, which shares similar atomic structures and properties with K+, shows toxicity at high concentration, and the toxicity is partly because of its substitute for K+ (Page and Di Cera, 2006). Therefore, the Na+ surplus must be effectively eliminated to maintain the minor concentration in the cytosol (Rodrígueznavarro, 2000). As described in Figure 2D, Cnh1 is the plasma membrane Na+/H+ antiporter, which utilizes the proton gradient to mediate the efflux of alkali metal cations and ensure the optimal intracellular level of them (Kinclova-Zimmermannova and Sychrova, 2007). Tuck-Wah Soong et al. verified that deletion of CNH1 led to the retarded growth in vitro and a considerable delay in the infected mouse model, and the pathogenicity-related features of CNH1 rendered the gene a potential target for developing anti-Candida agents (Soong et al., 2000).
Conclusion
Infections caused by C. albicans have been increasing in recent years and can result in local or systemic infections with high morbidity and mortality. The increase of drug resistance within C. albicans makes it crucial to investigate novel antifungal targets further. It should be considered that ions are crucial in every cellular system, since they are involved in a wide variety of important metabolic processes and pathogenesis. Moreover, fungi have evolved an accurate regulatory uptake and detoxification system for essential ions such as hydrogen (H+), calcium (Ca2+), iron (Fe3+), zinc (Zn2+), potassium (K+), and sodium (Na+). Interfering with ion-dependent processes in fungi may be an effective approach to defeat these microorganisms. Recent studies have shown that some agents are able to inhibit C. albicans essential biological processes, such as growth and proliferation, as well as relevant pathological events, such as adhesion to host structures, evasion of host immune response, and the regulation of virulence factors. Moreover, further studies have shown that compounds disrupting ion homeostasis could exert antifungal effects or even reverse the C. albicans drug resistance. Although certain limitations still exist in the development of antifungal drugs based on ion homeostasis due to the highly conserved structure in humans, we still believe that it is of great value. On the one hand, a series of in vivo studies have shown that compounds based on ion homeostasis could increase the survival rate of murine or Galleria mellonella Candidiasis models when used alone or in combination with antifungal agents. On the other hand, the new mechanisms based on ion homeostasis may provide clues for the search of fungal-specific targets. There is no doubt that more studies are needed to explore how to target ion signaling pathways in fungi for antifungal therapies.
Author Contributions
YL wrote the review. SS, LS, CL, YG, and ML also contributed to the writing of this article. The authors are grateful for the suggestions of SS regarding the language and structure of the review.
Funding
This work was supported by grants from the Department of Science and Technology of Shandong Province of China (2016GSF201187) and the Department of Health of Shandong Province of China (2007WH109).
Conflict of Interest Statement
The authors declare that the research was conducted in the absence of any commercial or financial relationships that could be construed as a potential conflict of interest.
References
Andrés, M. T., Viejo-Díaz, M., and Fierro, J. F. (2008). Human lactoferrin induces apoptosis-like cell death in Candida albicans: critical role of K+-channel-mediated K+ efflux. Antimicrob. Agents Chemother. 52, 4081–4088. doi: 10.1128/AAC.01597-07
Ariño, J., Ramos, J., and Sychrová, H. (2010). Alkali metal cation transport and homeostasis in yeasts. Microbiol. Mol. Biol. Rev. 74, 95–120. doi: 10.1128/MMBR.00042-09
Ates, M., Akdeniz, B. G., and Sen, B. H. (2005). The effect of calcium chelating or binding agents on Candida albicans. Oral Surg. Oral Med. Oral Pathol. Oral Radiol. Endod. 100, 626–630. doi: 10.1016/j.tripleo.2005.03.004
Baev, D., Rivetta, A., Li, X. S., Vylkova, S., Bashi, E., Slayman, C. L., et al. (2003). Killing of Candida albicans by human salivary histatin 5 is modulated, but not determined, by the potassium channel TOK1. Infect. Immun. 71, 3251–3260. doi: 10.1128/iai.71.6.3251-3260.2003
Bairwa, G., Hee Jung, W., and Kronstad, J. W. (2017). Iron acquisition in fungal pathogens of humans. Metallomics 9, 215–227. doi: 10.1039/c6mt00301j
Balhara, M., Chaudhary, R., Ruhil, S., Singh, B., Dahiya, N., Parmar, V. S., et al. (2016). Siderophores; iron scavengers: the novel & promising targets for pathogen specific antifungal therapy. Expert Opin. Ther. Targets 20, 1477–1489. doi: 10.1080/14728222.2016.1254196
Biswas, S. K., Yokoyama, K., Kamei, K., Nishimura, K., and Miyaji, M. (2001). Inhibition of hyphal growth of Candida albicans by activated lansoprazole, a novel benzimidazole proton pump inhibitor. Med. Mycol. 39, 283.
Bortner, C. D., and Cidlowski, J. A. (2007). Cell shrinkage and monovalent cation fluxes: role in apoptosis. Arch. Biochem. Biophys. 462, 176–188. doi: 10.1016/j.abb.2007.01.020
Casey, J. R., Grinstein, S., and Orlowski, J. (2010). Sensors and regulators of intracellular pH. Nat. Rev. Mol. Cell Biol. 11, 50–61. doi: 10.1038/nrm2820
Cheng, X., Xu, N., Yu, Q., Ding, X., Qian, K., Zhao, Q., et al. (2013). Novel insight into the expression and function of the multicopper oxidases in Candida albicans. Microbiology 159(Pt 6), 1044–1055. doi: 10.1099/mic.0.065268-0
Citiulo, F., Jacobsen, I. D., Miramon, P., Schild, L., Brunke, S., Zipfel, P., et al. (2012). Candida albicans scavenges host zinc via Pra1 during endothelial invasion. PLoS Pathog. 8:e1002777. doi: 10.1371/journal.ppat.1002777
Cornet, M., and Gaillardin, C. (2014). pH signaling in human fungal pathogens: a new target for antifungal strategies. Eukaryot. Cell 13, 342–352. doi: 10.1128/EC.00313-13
Cyert, M. S., and Philpott, C. C. (2013). Regulation of cation balance in Saccharomyces cerevisiae. Genetics 193, 677–713. doi: 10.1534/genetics.112.147207
Davis, D., John, E., Edwards, S. J., Mitchell, A. P., and Ibrahim, A. S. (2000a). Candida albicans RIM101 pH response pathway is required for host-pathogen interactions. Infect. Immun. 68, 5953–5959.
Davis, D., Wilson, R. B., and Mitchell, A. P. (2000b). RIM101-dependent and-independent pathways govern pH responses in Candida albicans. Mol. Cell. Biol. 20, 971–978.
Davis, D. A. (2009). How human pathogenic fungi sense and adapt to pH: the link to virulence. Curr. Opin. Microbiol. 12, 365–370. doi: 10.1016/j.mib.2009.05.006
Ding, X., Yu, Q., Xu, N., Wang, Y., Cheng, X., Qian, K., et al. (2013). Ecm7, a regulator of HACS, functions in calcium homeostasis maintenance, oxidative stress response and hyphal development in Candida albicans. Fungal Genet. Biol. 57, 23–32. doi: 10.1016/j.fgb.2013.05.010
Fan, Y., He, H., Dong, Y., and Pan, H. (2013). Hyphae-specific genes HGC1, ALS3, HWP1, and ECE1 and relevant signaling pathways in Candida albicans. Mycopathologia 176, 329–335. doi: 10.1007/s11046-013-9684-6
Finnigan, G. C., Cronan, G. E., Park, H. J., Srinivasan, S., Quiocho, F. A., and Stevens, T. H. (2012). Sorting of the yeast vacuolar-type, proton-translocating ATPase enzyme complex (V-ATPase): identification of a necessary and sufficient Golgi/endosomal retention signal in Stv1p. J. Biol. Chem. 287, 19487–19500. doi: 10.1074/jbc.M112.343814
Froschauer, E. M., Schweyen, R. J., and Wiesenberger, G. (2009). The yeast mitochondrial carrier proteins Mrs3p/Mrs4p mediate iron transport across the inner mitochondrial membrane. Biochim. Biophys. Acta 1788, 1044–1050. doi: 10.1016/j.bbamem.2009.03.004
Gerwien, F., Safyan, A., Wisgott, S., Hille, F., Kaemmer, P., Linde, J., et al. (2016). A novel hybrid iron regulation network combines features from pathogenic and nonpathogenic yeasts. MBio 7:e01782-16. doi: 10.1128/mBio.01782-16
Gerwien, F., Skrahina, V., Kasper, L., Hube, B., and Brunke, S. (2018). Metals in fungal virulence. FEMS Microbiol. Rev. 42:fux050. doi: 10.1093/femsre/fux050
Goncalves, I. R., Conde e Silva, N., Garay, C. L., Lesuisse, E., Camadro, J. M., and Blaiseau, P. L. (2014). The basis for evolution of DNA-binding specificity of the Aft1 transcription factor in yeasts. Genetics 196, 149–160. doi: 10.1534/genetics.113.157693
Guo, Q., Sun, S., Yu, J., Li, Y., and Cao, L. (2008). Synergistic activity of azoles with amiodarone against clinically resistant Candida albicans tested by chequerboard and time-kill methods. J. Med. Microbiol. 57(Pt 4), 457–462. doi: 10.1099/jmm.0.47651-0
Guo, X. L., Leng, P., Yang, Y., Yu, L. G., and Lou, H. X. (2008). Plagiochin E, a botanic-derived phenolic compound, reverses fungal resistance to fluconazole relating to the efflux pump. J. Appl. Microbiol. 104, 831–838. doi: 10.1111/j.1365-2672.2007.03617.x
Gupta, S. S., Ton, V.-K., Beaudry, V., Rulli, S., Cunningham, K., and Rao, R. (2003). Antifungal activity of amiodarone is mediated by disruption of calcium homeostasis. J. Biol. Chem. 278, 28831–28839. doi: 10.1074/jbc.M303300200
Han, J., Jyoti, M. A., Song, H. Y., Jang, W. S. (2016). Antifungal activity and action mechanism of histatin 5-halocidin hybrid peptides against Candida ssp. PLoS ONE 11:e0150196. doi: 10.1371/journal.pone.0150196
Harren, K., and Tudzynski, B. (2013). Cch1 and Mid1 are functionally required for vegetative growth under low-calcium conditions in the phytopathogenic ascomycete Botrytis cinerea. Eukaryot. Cell 12, 712. doi: 10.1128/EC.00338-12
Heymann, P., Gerads, M., Schaller, M., Dromer, F., Winkelmann, G., and Ernst, J. F. (2002). The siderophore iron transporter of Candida albicans (Sit1p/Arn1p) mediates uptake of ferrichrome-type siderophores and is required for epithelial invasion. Infect. Immun. 70, 5246–5255. doi: 10.1128/iai.70.9.5246-5255.2002
Irfan, M., Alam, S., Manzoor, N., and Abid, M. (2017). Effect of quinoline based 1,2,3-triazole and its structural analogues on growth and virulence attributes of Candida albicans. PLoS ONE 12:e0175710. doi: 10.1371/journal.pone.0175710
Jeeves, R. E., Mason, R. P., Woodacre, A., and Cashmore, A. M. (2011). Ferric reductase genes involved in high-affinity iron uptake are differentially regulated in yeast and hyphae of Candida albicans. Yeast 28, 629–644. doi: 10.1002/yea.1892
Juvvadi, P. R., Lee, S. C., Heitman, J., and Steinbach, W. J. (2017). Calcineurin in fungal virulence and drug resistance: prospects for harnessing targeted inhibition of calcineurin for an antifungal therapeutic approach. Virulence 8, 186–197. doi: 10.1080/21505594.2016.1201250
Kane, P. M. (2006). The where, when, and how of organelle acidification by the yeast vacuolar H+-ATPase. Microbiol. Mol. Biol. Rev. 70, 177–191. doi: 10.1128/MMBR.70.1.177-191.2006
Kane, P. M. (2016). Proton transport and pH control in fungi. Adv. Exp. Med. Biol. 892, 33–68. doi: 10.1007/978-3-319-25304-6_3
Kanki, T., Wang, K., Baba, M., and Bartholomew, C. R. (2009). A genomic screen for yeast mutants defective in selective mitochondria autophagy. Mol. Biol. Cell 20, 4730–4738. doi: 10.1091/mbc.E09-03-0225
Karababa, M., Valentino, E., Pardini, G., Coste, A. T., Bille, J., and Sanglard, D. (2006). CRZ1, a target of the calcineurin pathway in Candida albicans. Mol. Microbiol. 59, 1429–1451. doi: 10.1111/j.1365-2958.2005.05037.x
Kaur, S., and Mishra, P. (1991). Dimorphism-associated changes in plasma membrane H+-ATPase activity of Candida albicans. Arch. Microbiol. 156, 412–415.
Kaur, S., Mishra, P., and Prasad, R. (1988). Dimorphism-associated changes in intracellular pH of Candida albicans. Biochim. Biophys. Acta 972, 277–282.
Khan, N., Shreaz, S., Bhatia, R., Ahmad, S. I., Muralidhar, S., Manzoor, N., et al. (2012). Anticandidal activity of curcumin and methyl cinnamaldehyde. Fitoterapia 83, 434–440. doi: 10.1016/j.fitote.2011.12.003
Kim, M. J., Kil, M., Jung, J. H., and Kim, J. (2008). Roles of Zinc-responsive transcription factor Csr1 in filamentous growth of the pathogenic Yeast Candida albicans. J. Microbiol. Biotechnol. 18, 242–247.
Kinclova-Zimmermannova, O., and Sychrova, H. (2007). Plasma-membrane Cnh1 Na+/H+ antiporter regulates potassium homeostasis in Candida albicans. Microbiology 153(Pt 8), 2603–2612. doi: 10.1099/mic.0.2007/008011-0
Lehtovirta-Morley, L. E., Alsarraf, M., and Wilson, D. (2017). Pan-domain analysis of ZIP zinc transporters. Int. J. Mol. Sci. 18. doi: 10.3390/ijms18122631
Lew, R. R. (2011). How does a hypha grow? The biophysics of pressurized growth in fungi. Nat. Rev. Microbiol. 9, 509–518. doi: 10.1038/nrmicro2591
Li, L., Chen, O. S., McVey Ward, D., and Kaplan, J. (2001). CCC1 is a transporter that mediates vacuolar iron storage in yeast. J. Biol. Chem. 276, 29515–29519. doi: 10.1074/jbc.M103944200
Li, X., and Sun, S. (2016). Targeting the fungal calcium-calcineurin signaling network in overcoming drug resistance. Future Med. Chem. 8, 1379. doi: 10.4155/fmc-2016-0094
Liang, Y., Wei, D., Wang, H., Xu, N., Zhang, B., Xing, L., et al. (2010). Role of Candida albicans Aft2p transcription factor in ferric reductase activity, morphogenesis and virulence. Microbiology 156(Pt 10), 2912–2919. doi: 10.1099/mic.0.037978-0
Lin, L., Pantapalangkoor, P., Tan, B., Bruhn, K. W., Ho, T., Nielsen, T., et al. (2014). Transferrin iron starvation therapy for lethal bacterial and fungal infections. J. Infect. Dis. 210, 254–264. doi: 10.1093/infdis/jiu049
Liu, S., Hou, Y., Liu, W., Lu, C., Wang, W., and Sun, S. (2015). Components of the calcium-calcineurin signaling pathway in fungal cells and their potential as antifungal targets. Eukaryot. Cell 14, 324–334. doi: 10.1128/EC.00271-14
Liu, S., Yue, L., Gu, W., Li, X., Zhang, L., and Sun, S. (2016). Synergistic effect of fluconazole and calcium channel blockers against resistant Candida albicans. PLoS ONE 11:e0150859. doi: 10.1371/journal.pone.0150859
Loboda, D., and Rowinska-Zyrek, M. (2017). Zinc binding sites in Pra1, a zincophore from Candida albicans. Dalton Trans. 46, 13695–13703. doi: 10.1039/c7dt01675a
Loukin, S. H., Lin, J., Athar, U., Palmer, C., and Saimi, Y. (2002). The carboxyl tail forms a discrete functional domain that blocks closure of the yeast K+ channel. Proc. Natl. Acad. Sci. U.S.A. 99, 1926–1930. doi: 10.1073/pnas.042538599
Malavia, D., Crawford, A., and Wilson, D. (2017). Nutritional immunity and fungal pathogenesis: the struggle for micronutrients at the host-pathogen interface. Adv. Microb. Physiol. 70, 85–103. doi: 10.1016/bs.ampbs.2017.01.006
Mamouei, Z., Zeng, G., Wang, Y. M., and Wang, Y. (2017). Candida albicans possess a highly versatile and dynamic high-affinity iron transport system important for its commensal-pathogenic lifestyle. Mol. Microbiol. 106, 986–998. doi: 10.1111/mmi.13864
Manavathu, E. K., Dimmock, J. R., Vashishtha, S. C., and Chandrasekar, P. H. (1999). Proton-pumping-ATPase-targeted antifungal activity of a novel conjugated styryl ketone. Antimicrob. Agents Chemother. 43:2950.
Manzoor, N. (2016). “Plasma membrane ATPase: potential target for antifungal drug therapy,” in Regulation of Ca2+-ATPases,V-ATPases and F-ATPases, eds S. Chakraborti and N. Dhalla (Cham: Springer), 519–530.
Meleddu, R., Distinto, S., Corona, A., Maccioni, E., Arridu, A., Melis, C., et al. (2016). Exploring the thiazole scaffold for the identification of new agents for the treatment of fluconazole resistant Candida. J. Enzyme Inhib. Med. Chem. 31, 1672–1677. doi: 10.3109/14756366.2015.1113171
Monk, B. C., Mason, A. B., Abramochkin, G., Haber, J. E., Seto-Young, D., and Perlin, D. S. (1995). The yeast plasma membrane proton pumping ATPase is a viable antifungal target. I. Effects of the cysteine-modifying reagent omeprazole. Biochim. Biophys. Acta 1239, 81–90.
Monk, B. C., Niimi, K., Lin, S., Knight, A., Kardos, T. B., Cannon, R. D., et al. (2005). Surface-active fungicidal D-peptide inhibitors of the plasma membrane proton pump that block azole resistance. Antimicrob. Agents Chemother. 49, 57–70. doi: 10.1128/AAC.49.1.57-70.2005
Muzzey, D., Schwartz, K., Weissman, J. S., and Sherlock, G. (2013). Assembly of a phased diploid Candida albicans genome facilitates allele-specific measurements and provides a simple model for repeat and indel structure. Genome Biol. 14:R97. doi: 10.1186/gb-2013-14-9-r97
Nasser, L., Weissman, Z., Pinsky, M., Amartely, H., Dvir, H., and Kornitzer, D. (2016). Structural basis of heam-iron acquisition by fungal pathogens. Nat. Microbiol. 1:16156. doi: 10.1038/nmicrobiol.2016.156
Niewerth, M., Kunze, D., Seibold, M., Schaller, M., Korting, H. C., and Hube, B. (2003). Ciclopirox olamine treatment affects the expression pattern of Candida albicans genes encoding virulence factors, iron metabolism proteins, and drug resistance factors. Antimicrob. Agents Chemother. 47, 1805–1817. doi: 10.1128/aac.47.6.1805-1817.2003
O'Hanlon Cohrt, K. A., Marin, L., Kjellerup, L., Clausen, J. D., Dalby-Brown, W., Calera, J. A., et al. (2018). Novel zinc attenuating compounds as potent broad spectrum antifungal agents with in vitro and in vivo efficacy. Antimicrob. Agents Chemother. 62:e02024-17. doi: 10.1128/AAC.02024-17
Okamoto-Shibayama, K., Kikuchi, Y., Kokubu, E., Sato, Y., and Ishihara, K. (2014). Csa2, a member of the Rbt5 protein family, is involved in the utilization of iron from human hemoglobin during Candida albicans hyphal growth. FEMS Yeast Res. 14, 674–677. doi: 10.1111/1567-1364.12160
Okoli, I., Coleman, J. J., Tampakakis, E., An, W. F., Holson, E., Wagner, F., et al. (2009). Identification of antifungal compounds active against Candida albicans using an improved high-throughput Caenorhabditis elegans assay. PLoS ONE 4:e7025. doi: 10.1371/journal.pone.0007025
Olsen, I. (2014). Attenuation of Candida albicans virulence with focus on disruption of its vacuole functions. J. Oral Microbiol. 6:23898. doi: 10.3402/jom.v6.23898
Orij, R., Brul, S., and Smits, G. J. (2011). Intracellular pH is a tightly controlled signal in yeast. Biochim. Biophys. Acta 1810, 933–944. doi: 10.1016/j.bbagen.2011.03.011
Page, M. J., and Di Cera, E. (2006). Role of Na+ and K+ in enzyme function. Physiol. Rev. 86, 1049–1092. doi: 10.1152/physrev.00008.2006
Patenaude, C., Zhang, Y., Cormack, B., Kohler, J., and Rao, R. (2013). Essential role for vacuolar acidification in Candida albicans virulence. J. Biol. Chem. 288, 26256–26264. doi: 10.1074/jbc.M113.494815
Perlin, D. S., Seto-Young, D., and Monk, B. C. (1997). The plasma membrane H+-ATPase of fungi. A candidate drug target? Ann. N. Y. Acad. Sci. 834, 609–617.
Poltermann, S. (2005). The putative vacuolar ATPase subunit Vma7p of Candida albicans is involved in vacuole acidification, hyphal development and virulence. Microbiology 151, 1645–1655. doi: 10.1099/mic.0.27505-0
Portnoy, M. E., Liu, X. F., and Culotta, V. C. (2000). Saccharomyces cerevisiae expresses three functionally distinct homologues of the nramp family of metal transporters. Mol. Cell. Biol. 20, 7893–7902.
Prasad, T., Chandra, A., Mukhopadhyay, C. K., and Prasad, R. (2006). Unexpected link between iron and drug resistance of Candida spp.: iron depletion enhances membrane fluidity and drug diffusion, leading to drug-susceptible cells. Antimicrob. Agents Chemother. 50, 3597–3606. doi: 10.1128/AAC.00653-06
Prole, D. L., and Taylor, C. W. (2012). Identification and analysis of cation channel homologues in human pathogenic fungi. PLoS ONE 7:e42404. doi: 10.1371/journal.pone.0042404
Raines, S. M., Rane, H. S., Bernardo, S. M., Binder, J. L., Lee, S. A., and Parra, K. J. (2013). Deletion of vacuolar proton-translocating ATPase V0a isoforms clarifies the role of vacuolar pH as a determinant of virulence-associated traits in Candida albicans. J. Biol. Chem. 288, 6190–6201. doi: 10.1074/jbc.M112.426197
Rane, H. S., Bernardo, S. M., Hayek, S. R., Binder, J. L., Parra, K. J., and Lee, S. A. (2014). The contribution of Candida albicans vacuolar ATPase subunit V1B, encoded by VMA2, to stress response, autophagy, and virulence is independent of environmental pH. Eukaryot. Cell 13, 1207–1221. doi: 10.1128/EC.00135-14
Rane, H. S., Bernardo, S. M., Raines, S. M., Binder, J. L., Parra, K. J., and Lee, S. A. (2013). Candida albicans VMA3 is necessary for V-ATPase assembly and function and contributes to secretion and filamentation. Eukaryot. Cell 12, 1369–1382. doi: 10.1128/EC.00118-13
Remillard, C. V., and Yuan, J. X. (2004). Activation of K+ channels: an essential pathway in programmed cell death. Am. J. Physiol. Lung Cell. Mol. Physiol. 286, L49–L67. doi: 10.1152/ajplung.00041.2003
Rodrígueznavarro, A. (2000). Potassium transport in fungi and plants. Biochim. Biophys. Acta 1469, 1–30.
Sanglard, D. I. F., Marchetti, O., Entenza, J., and Bille, J. (2003). Calcineurin A of Candida albicans: involvement in antifungal tolerance, cell morphogenesis and virulence. Mol. Microbiol. 48, 959–976.
Serrano, R., Kiellandbrandt, M. C., and Fink, G. R. (1986). Yeast plasma membrane ATPase is essential for growth and has homology with (Na++K+), K+- and Ca2+-ATPases. Nature 319, 689–693. doi: 10.1038/319689a0
Seto-Young, D., Monk, B., Mason, A. B., and Perlin, D. S. (1997). Exploring an antifungal target in the plasma membrane H+-ATPase of fungi. Biochim. Biophys. Acta 1326, 249–256.
Silva, S., Rodrigues, C. F., Araujo, D., Rodrigues, M. E., and Henriques, M. (2017). Candida Species Biofilms' Antifungal Resistance. J. Fungi (Basel) 3:8. doi: 10.3390/jof3010008
Singh, S., Fatima, Z., and Hameed, S. (2016). Insights into the mode of action of anticandidal herbal monoterpenoid geraniol reveal disruption of multiple MDR mechanisms and virulence attributes in Candida albicans. Arch. Microbiol. 198, 459–472. doi: 10.1007/s00203-016-1205-9
Soong, T. W., Yong, T. F., Ramanan, N., and Wang, Y. (2000). The Candida albicans antiporter gene CNH1 has a role in Na+ and H+ transport, salt tolerance, and morphogenesis. Microbiology 146 (Pt 5), 1035–1044. doi: 10.1099/00221287-146-5-1035
Soteropoulos, P., Vaz, T., Santangelo, R., Paderu, P., Huang, D. Y., Tamás, M. J., et al. (2000). Molecular characterization of the plasma membrane H+-ATPase, an antifungal target in Cryptococcus neoformans. Antimicrob. Agents Chemother. 44, 2349–2355.
Spacek, J., Jilek, P., Buchta, V., F, M., Hronek, M., and Holeckova, M. (2005). The serum levels of calcium, magnesium, iron and zinc in patients with recurrent vulvovaginal candidosis during attack, remission and in healthy controls. Mycoses 48, 391–395. doi: 10.1111/j.1439-0507.2005.01164.x
Stewart, E., Hawser, S., and Gow, N. A. (1989). Changes in internal and external pH accompanying growth of Candida albicans: studies of non-dimorphic variants. Arch. Microbiol. 151, 149–153.
Sun, L., Liao, K., and Wang, D. (2015). Effects of magnolol and honokiol on adhesion, yeast-hyphal transition, and formation of biofilm by Candida albicans. PLoS ONE 10:e0117695. doi: 10.1371/journal.pone.0117695
Thewes, S. (2014). Calcineurin-Crz1 signaling in lower eukaryotes. Eukaryot. Cell 13, 694–705. doi: 10.1128/EC.00038-14
Tobudic, S., Kratzer, C., Lassnigg, A., and Presterl, E. (2012). Antifungal susceptibility of Candida albicans in biofilms. Mycoses 55, 199–204. doi: 10.1111/j.1439-0507.2011.02076.x
Tong, Y., Liu, M., Zhang, Y., Liu, X., Huang, R., Song, F., et al. (2016). Beauvericin counteracted multi-drug resistant Candida albicans by blocking ABC transporters. Synth. Syst. Biotechnol. 1, 158–168. doi: 10.1016/j.synbio.2016.10.001
Torey, A., Vijayarathna, S., Jothy, S. L., Gothai, S., Chen, Y., Latha, L. Y., et al. (2016). Exploration of the anticandidal mechanism of Cassia spectabilis in debilitating candidiasis. J. Tradit. Complement. Med. 6, 97–104. doi: 10.1016/j.jtcme.2014.11.017
Tripathi, S. K., Xu, T., Feng, Q., Avula, B., Shi, X., Pan, X., et al. (2017). Two plant-derived aporphinoid alkaloids exert their antifungal activity by disrupting mitochondrial iron-sulfur cluster biosynthesis. J. Biol. Chem. 292, 16578–16593. doi: 10.1074/jbc.M117.781773
Vila, T., Romo, J. A., Pierce, C. G., McHardy, S. F., Saville, S.P., and Lopez-Ribot, J. L. (2017). Targeting Candida albicans filamentation for antifungal drug development. Virulence 8, 150–158. doi: 10.1080/21505594.2016.1197444
Wu, C. Y., Bird, A. J., Chung, L. M., Newton, M. A., Winge, D. R., and Eide, D. J. (2008). Differential control of Zap1-regulated genes in response to zinc deficiency in Saccharomyces cerevisiae. BMC Genomics 9:370. doi: 10.1186/1471-2164-9-370
Xu, N., Cheng, X., Yu, Q., Zhang, B., Ding, X., Xing, L., et al. (2012). Identification and functional characterization of mitochondrial carrier Mrs4 in Candida albicans. FEMS Yeast Res. 12, 844–858. doi: 10.1111/j.1567-1364.2012.00835.x
Xu, N., Dong, Y., Cheng, X., Yu, Q., Qian, K., Mao, J., et al. (2014a). Cellular iron homeostasis mediated by the Mrs4-Ccc1-Smf3 pathway is essential for mitochondrial function, morphogenesis and virulence in Candida albicans. Biochim. Biophys. Acta 1843, 629–639. doi: 10.1016/j.bbamcr.2013.12.009
Xu, N., Qian, K., Dong, Y., Chen, Y., Yu, Q., Zhang, B., et al. (2014b). Novel role of the Candida albicans ferric reductase gene CFL1 in iron acquisition, oxidative stress tolerance, morphogenesis and virulence. Res. Microbiol. 165, 252–261. doi: 10.1016/j.resmic.2014.03.001
Xu, W., Solis, N. V., Ehrlich, R. L., Woolford, C. A., Filler, S. G., and Mitchell, A. P. (2015). Activation and alliance of regulatory pathways in C. albicans during mammalian infection. PLoS Biol. 13:e1002076. doi: 10.1371/journal.pbio.1002076
Yike, I. (2011). Fungal proteases and their pathophysiological effects. Mycopathologia 171, 299–323. doi: 10.1007/s11046-010-9386-2
Yu, L., Strandberg, L., and Lenardo, M. J. (2008). The selectivity of autophagy and its role in cell death and survival. Autophagy 4, 567–573.
Yu, Q., Ding, X., Xu, N., Cheng, X., Qian, K., Zhang, B., et al. (2013a). In vitro activity of verapamil alone and in combination with fluconazole or tunicamycin against Candida albicans biofilms. Int. J. Antimicrob. Agents 41, 179–182. doi: 10.1016/j.ijantimicag.2012.10.009
Yu, Q., Ding, X., Zhang, B., Xu, N., Cheng, X., Qian, K., et al. (2013b). The P-type ATPase Spf1 is required for endoplasmic reticulum functions and cell wall integrity in Candida albicans. Int. J. Med. Microbiol. 303, 257–266. doi: 10.1016/j.ijmm.2013.05.003
Yu, Q., Ding, X., Zhang, B., Xu, N., Jia, C., Mao, J., et al. (2014a). Inhibitory effect of verapamil on Candida albicans hyphal development, adhesion and gastrointestinal colonization. FEMS Yeast Res. 14, 633–641. doi: 10.1111/1567-1364.12150
Yu, Q., Dong, Y., Xu, N., Qian, K., Chen, Y., Zhang, B., et al. (2014b). A novel role of the ferric reductase Cfl1 in cell wall integrity, mitochondrial function, and invasion to host cells in Candida albicans. FEMS Yeast Res. 14, 1037–1047. doi: 10.1111/1567-1364.12194
Yu, Q., Jia, C., Dong, Y., Zhang, B., Xiao, C., Chen, Y., et al. (2015). Candida albicans autophagy, no longer a bystander: its role in tolerance to ER stress-related antifungal drugs. Fungal Genet. Biol. 81, 238–249. doi: 10.1016/j.fgb.2015.02.008
Yu, Q., Wang, F., Zhao, Q., Chen, J., Zhang, B., Ding, X., et al. (2014c). A novel role of the vacuolar calcium channel Yvc1 in stress response, morphogenesis and pathogenicity of Candida albicans. Int. J. Med. Microbiol. 304, 339–350. doi: 10.1016/j.ijmm.2013.11.022
Yu, Q., Wang, H., Cheng, X., Xu, N., Ding, X., Xing, L., et al. (2012a). Roles of Cch1 and Mid1 in morphogenesis, oxidative stress response and virulence in Candida albicans. Mycopathologia 174, 359–369. doi: 10.1007/s11046-012-9569-0
Yu, Q., Wang, H., Xu, N., Cheng, X., Wang, Y., Zhang, B., et al. (2012b). Spf1 strongly influences calcium homeostasis, hyphal development, biofilm formation and virulence in Candida albicans. Microbiology 158(Pt 9), 2272–2282. doi: 10.1099/mic.0.057232-0
Yu, Q., Zhang, B., Yang, B., Chen, J., Wang, H., Jia, C., et al. (2014d). Interaction among the vacuole, the mitochondria, and the oxidative stress response is governed by the transient receptor potential channel in Candida albicans. Free Radic. Biol. Med. 77, 152–167. doi: 10.1016/j.freeradbiomed.2014.09.011
Yun, D. G., and Lee, D. G. (2016). Silibinin triggers yeast apoptosis related to mitochondrial Ca2+ influx in Candida albicans. Int. J. Biochem. Cell Biol. 80, 1–9. doi: 10.1016/j.biocel.2016.09.008
Yun, J., and Lee, D. G. (2017). Role of potassium channels in chlorogenic acid-induced apoptotic volume decrease and cell cycle arrest in Candida albicans. Biochim. Biophys. Acta 1861, 585–592. doi: 10.1016/j.bbagen.2016.12.026
Zavrel, M., and White, T. C. (2015). Medically important fungi respond to azole drugs: an update. Future Microbiol. 10, 1355–1373. doi: 10.2217/FMB.15.47
Zhang, K., Jia, C., Yu, Q., Xiao, C., and Dong, Y. (2017). Contribution of VMA5 to vacuolar function, stress response, ion homeostasis and autophagy in Candida albicans. Future Microbiol. 12, 1147–1166. doi: 10.2217/fmb-2017-0029
Zhang, Y., Muend, S., and Rao, R. (2012). Dysregulation of ion homeostasis by antifungal agents. Front. Microbiol. 3:133. doi: 10.3389/fmicb.2012.00133
Zhang, Y., and Rao, R. (2012). The V-ATPase as a target for antifungal drugs. Current Protein and Peptide Science 13, 134–140. doi: 10.2174/138920312800493205
Keywords: Candida albicans, antifungal targets, ion homeostasis, virulence, ion signaling pathways
Citation: Li Y, Sun L, Lu C, Gong Y, Li M and Sun S (2018) Promising Antifungal Targets Against Candida albicans Based on Ion Homeostasis. Front. Cell. Infect. Microbiol. 8:286. doi: 10.3389/fcimb.2018.00286
Received: 01 June 2018; Accepted: 27 July 2018;
Published: 04 September 2018.
Edited by:
Andrew Alspaugh, Duke University, United StatesReviewed by:
Angie Gelli, University of California, Davis, United StatesJesus Antonio Romo, University of Texas at San Antonio, United States
Copyright © 2018 Li, Sun, Lu, Gong, Li and Sun. This is an open-access article distributed under the terms of the Creative Commons Attribution License (CC BY). The use, distribution or reproduction in other forums is permitted, provided the original author(s) and the copyright owner(s) are credited and that the original publication in this journal is cited, in accordance with accepted academic practice. No use, distribution or reproduction is permitted which does not comply with these terms.
*Correspondence: Shujuan Sun, c3Vuc2h1anVhbjg4OEAxNjMuY29t