- 1BIOEPAR, INRA, Oniris, Université Bretagne Loire, Nantes, France
- 2Institute of Parasitology, Biology Centre of the Czech Academy of Sciences, České Budějovice, Czechia
- 3Faculty of Science, University of South Bohemia, České Budějovice, Czechia
Although apicomplexan parasites of the group Piroplasmida represent commonly identified global risks to both animals and humans, detailed knowledge of their life cycles is surprisingly limited. Such a discrepancy results from incomplete literature reports, nomenclature disunity and recently, from large numbers of newly described species. This review intends to collate and summarize current knowledge with respect to piroplasm phylogeny. Moreover, it provides a comprehensive view of developmental events of Babesia, Theileria, and Cytauxzoon representative species, focusing on uniform consensus of three consecutive phases: (i) schizogony and merogony, asexual multiplication in blood cells of the vertebrate host; (ii) gamogony, sexual reproduction inside the tick midgut, later followed by invasion of kinetes into the tick internal tissues; and (iii) sporogony, asexual proliferation in tick salivary glands resulting in the formation of sporozoites. However, many fundamental differences in this general consensus occur and this review identifies variables that should be analyzed prior to further development of specific anti-piroplasm strategies, including the attractive targeting of life cycle stages of Babesia or Theileria tick vectors.
Introduction
The group Piroplasmida received its name after its pear-shaped (pyriform) intra-erythrocytic stages and refers to intracellular parasites transmitted exclusively by hard ticks (Ixodidae) (Mehlhorn and Schein, 1993; Votypka et al., 2017). Piroplasms belong to the most common group of mammalian blood parasites and their impact economically, as well as on veterinary and medical care, is significant. Due to the worldwide distribution of tick vectors, babesiosis is the most common blood disease of free living animals (Homer et al., 2000; Hunfeld et al., 2008) and is considered an emergent zoonosis of humans (Homer et al., 2000; Kjemtrup and Conrad, 2000; Zintl et al., 2003; Hunfeld et al., 2008; Leiby, 2011). From the veterinary point of view, great attention is paid to bovine babesiosis, which is associated with mortalities, abortions, decreased meat, and milk production, but despite permanent epidemiological surveillance, most of the 1–2 billion cattle worldwide are still exposed to babesiosis and outbreaks occur frequently (Bock et al., 2004; Gohil et al., 2013). Humans are not natural hosts for any species of Babesia but serve as accidental hosts (reviewed in e.g., Yabsley and Shock, 2013). Despite this fact, the incidence of human babesiosis is on the rise and clinical cases have been reported recently from many countries worldwide (reviewed in e.g., Yabsley and Shock, 2013; Vannier et al., 2015).
Taxonomic classification places Piroplasmida species in the phylum Apicomplexa, as close relatives of the malarial disease agents, Plasmodium parasites (e.g., Burki et al., 2009; Janouskovec et al., 2010; Arisue and Hashimoto, 2014; Schreeg et al., 2016). Based on multi-gene analyses, the order Piroplasmida includes three genera, Babesia, Theileria, and Cytauxzoon. Piroplasms share many morphological and developmental features such as apical complex organelles, merogony (asexual multiplication) within erythrocytes of vertebrate hosts and sexual multiplication followed by sporozoite formation in invertebrate vectors, ticks (Homer et al., 2000). There are five evolutionary lineages recognized in the order Piroplasmida (Schreeg et al., 2016). All these lineages differ in particular developmental features and possess unique adaptations (Table 1). The lifecycle of piroplasms is considered as only partially elucidated. There are many inconsistencies about crucial developmental events of piroplasms, and relevant information are spread throughout many publications. The recent nomenclature changes and redescription of many species has also contributed to these misconceptions (e.g., Mehlhorn and Schein, 1998; Malandrin et al., 2010; Baneth et al., 2015).
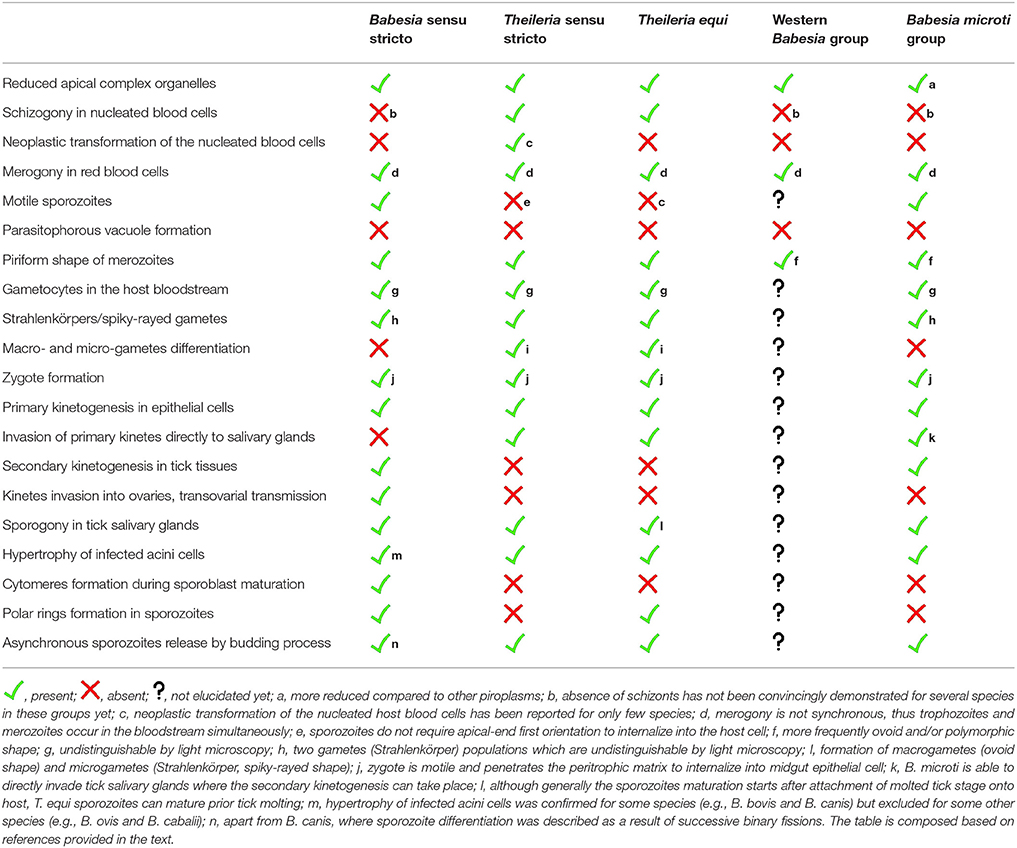
Table 1. Summarizing overview of characteristic life cycle events of five evolutionary lineages of the order Piroplasmida (based on Schreeg et al., 2016).
In this review we provide a comprehensive overview of piroplasm lifecycle events, proposing uniform consensus and stressing unique developmental adaptations with respect to evolutionary lineages.
Schizogony and Merogony: Asexual Multiplication in Blood Cells of the Vertebrate Host
All parasites of the group Piroplasmida reproduce asexually inside the blood cells of the vertebrate host (Figure 1, Table 1). The host infection is initiated by the invasion of sporozoites, transmitted through saliva secretion during the tick bite. The blood cells targeted by sporozoites differ according to the species of piroplasm (Shaw, 2003; Lobo et al., 2012). Theileria parasites are characterized by schizogony (Box 1) in nucleated blood cells—monocytes and lymphocytes—prior to red blood cell invasion (Schein et al., 1981; Moltmann et al., 1983a; Conrad et al., 1985; Webster et al., 1985; Dobbelaere and Heussler, 1999; Dobbelaere and Rottenberg, 2003; Shaw, 2003). Babesia parasites are believed to multiply exclusively in erythrocytes; so far a schizogony has never been convincingly confirmed (Mehlhorn and Schein, 1993; Lobo et al., 2012; Schreeg et al., 2016).
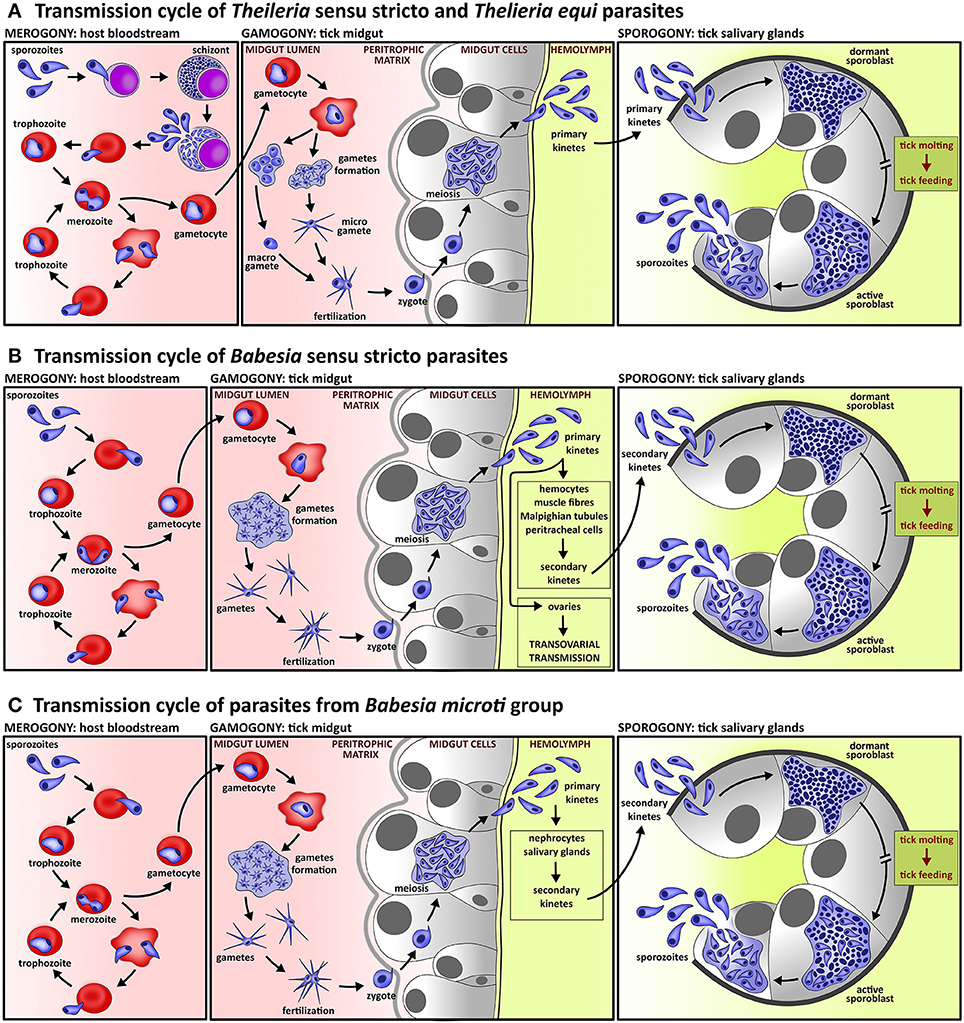
Figure 1. Lifecycle of piroplasms. (A) Lifecycle of species of Theileria sensu stricto lineage and Theileria equi includes intra-leucocytic schizogony prior to intra-erythrocytic merogony; schizogony is often accompanied by neoplastic transformation of host leucocytes. In contrast to Babesia species, the gametes of Theileria parasites form two morphologically distinguishable cell types, micro- and macro-gametes. Kinetes, which are produced in tick midgut cells, migrate directly to tick salivary glands where sporogony takes place. (B) Lifecycle of Babesia sensu stricto species comprise exclusively intra-erythrocytic asexual multiplication. Fertilization, which takes place in the tick midgut, is provoked by fusion of two morphologically indistinguishable gametes. The primary kinetes released from tick midgut cells invade various tick tissues, where secondary kinetes are produced. These then invade the tick salivary glands and undergo sporogony. All species of the lineage Babesia sensu stricto exploits transovarial transmission, a unique strategy of parasite invasion into ovarian cells by primary kinetes which results in Babesia-infected tick eggs and subsequent larvae. (C) The life cycle of Babesia microti group, the basal lineage of piroplasms, differs from species of the Babesia sensu stricto lineage by the lack of transovarial transmission.
Box 1. Subsequent phases of piroplasm development.
Schizogony. A process of asexual multiplication in nucleated blood cells (leukocytes) is typical only for two evolutionary lineages of piroplasms, Theileria sensu stricto and Theileria equi. Schizogony starts after sporozoite internalization into leukocytes and results in merozoite production, which further multiply by merogony. Schizogony can lead to neoplastic transformation of the nucleated host cells, which then proliferate indefinitely. Merogony. A process of asexual division in the red blood cell starts either with sporozoite (Babesia species) or merozoite (Theileria species) invasion of red blood cells. The internalized parasites develop into trophozoites, which further asexually divide into merozoites. Merozoites are then released by rupture of the host red blood cells and invade healthy erythrocytes. Gamogony. Sexual multiplication of the parasite starts by gametocytes appearing in the host red blood cells. During blood uptake by ticks, gametocytes develop into gametes that mature in the tick midgut lumen. Gamete fertilization then gives rise to a zygote that penetrates the tick peritrophic matrix to tick epithelial cells. Inside these, the zygote undergoes a meiotic division and results in the formation of kinetes, which are released to the haemolymph. The kinetes of Theileria species directly invade salivary glands (primary kinetes) but kinetes of Babesia parasites are subjected to two series of asexual multiplication in various tick tissues and subsequent secondary kinetes invade the tick salivary glands. Sporogony. Sporogony starts after kinete invasion of tick salivary glands, which form the sporont, a polymorphous syncytium. The sporont later evolves into a multinucleated meshwork referred as a sporoblast, which is dormant during tick ecdysis. Maturation of the parasite sporoblast starts after tick attachment to the host and results in sporozoites being released into tick saliva.
Schizogony
Intra-leukocytic asexual reproduction (Figure 1A) occurs in the lifecycle of two evolutionary lineages, Theileria sensu stricto (including Cytauxzoon spp.) and its sister clade, represented by Theileria equi (Table 1) (Kappmeyer et al., 2012; Schnittger et al., 2012; Schreeg et al., 2016). Schizogony serves to aid rapid parasite multiplication and gives rise to schizonts, referred to as Koch's bodies (Mehlhorn and Shein, 1984; Mehlhorn and Schein, 1993). These Theileria intra-leukocytic schizonts are able to modulate the host's immune response, e.g., to block host cell apoptosis (Blouin et al., 1987; Kawai et al., 1989; Sato et al., 1994; Hagiwara et al., 1997; Ahmed et al., 1999; Dobbelaere and Heussler, 1999; Susta et al., 2009). Moreover, leukocyte infection by Theileria parasites could lead to a fundamental change in the infected host cell's ability to proliferate indefinitely (Mehlhorn and Shein, 1984; Mehlhorn and Schein, 1993; Ahmed et al., 1999; Dobbelaere and Heussler, 1999; Dobbelaere and Rottenberg, 2003). Although schizogony in nucleated blood cells characterizes all Theileria parasites, the neoplastic transformation of the host cell was reported only for Theileria parva, Theileria annulata, Theileria lestoquardi, Theileria taurotragi, and Theileria sp. (buffalo) (Table 1; Ahmed et al., 1999; Dobbelaere and Heussler, 1999; Dobbelaere and Küenzi, 2004; Zweygarth et al., 2009; Sivakumar et al., 2014; Bishop et al., 2015). Changes in the host cell have not been described for T. equi (Schein et al., 1981; Moltmann et al., 1983a; Ramsay et al., 2013), presumably due to the absence of homologs of the putative Theileria host cell transforming genes (Kappmeyer et al., 2012; Schreeg et al., 2016).
The sporozoite invasion into nucleated blood cells is a complex process requiring numerous alterations in the metabolism of invading sporozoites (Shaw, 1995, 1996a,b), complicity of the host system (Shaw et al., 1991, 1993; Shaw, 1996a,b) and the involvement of tick saliva (Shaw et al., 1993). In contrast to other apicomplexan parasites, including Babesia, Theileria sporozoites are immotile (Table 1; Shaw, 1999, 2003). The initial contact of the parasite and host cell membrane thus occurs randomly (Shaw, 2003). Sporozoite attachment and internalization into the host cell does not require apical-end first orientation and the parasite enters in any orientation (Shaw, 1999, 2003). Thus, the proteins excreted by apical organelles are more involved in the establishment in the host cell cytoplasm rather than the entry process (Shaw et al., 1991). The invasion process comprises several consecutive stages and is completed within about 3 min: (i) recognition and attachment to the host cell membrane; (ii) formation of junctions between the parasite and the host cell membrane; (iii) a “zippering” process resulting in fully internalized parasite in the host cell, yet still surrounded by the host cell membrane; (iv–v) separation and progressive dissolution of the enclosing host cell membrane; and (vi) appearance of a microtubule network derived from the host cell and closely associated with the developing parasite (Fawcett et al., 1982c, 1984; Shaw et al., 1991; Shaw, 1997, 2003). During the invasion process the sporozoite sheds its coat (Dobbelaere et al., 1985; Webster et al., 1985; Shaw, 2003) and lies loosely in the host cell cytoplasm once it has escaped from the enclosing host membrane; no parasitophorous vacuole is formed (Shaw, 2003).
The internalized Theileria sporozoite undergoes a change into a multinucleate schizont (Shaw, 1997, 2003) and schizont-infected cells then circulate in the bloodstream (Dobbelaere and Heussler, 1999). The Theileria schizogony is accompanied by a series of ultrastructural changes affecting internal organelles as well as the outer surface of the parasite (Shaw and Tilney, 1992). The intra-leukocytic schizogony ends with the production of uninucleated merozoites released into the host bloodstream where they invade erythrocytes (Shaw and Tilney, 1992). The process of Theileria merozoite internalization into erythrocytes occurs in the same manner as sporozoite invasion of leukocytes (Shaw and Tilney, 1995). All Theileria parasites reproduce in erythrocytes but the process has been described in only a limited number of species (Conrad et al., 1986; Bishop et al., 2004).
Merogony
Exclusive intra-erythrocytic multiplication (Figures 1B,C) represents the cognitive feature of Babesia parasites but the absence of schizogony has not yet been demonstrated for several species of all three evolutionary distinct Babesia lineages, Babesia sensu stricto, Western Babesia group, and Babesia microti group (Table 1; Rudzinska and Trager, 1977; Kjemtrup et al., 2006; Lobo et al., 2012; Schreeg et al., 2016). The first contact between Babesia invasive stages and the host cell occurs through several random collisions. Unlike Theileria, Babesia's orientation of the apical (anterior) end establishes the junction between parasite and host cell membrane (Rudzinska et al., 1976; Montero et al., 2009; Asada et al., 2012; Lobo et al., 2012). Parasite orientation and penetration is mediated by proteins secreted from the apical secretory organelles (Dubremetz et al., 1998; Soldati et al., 2004), and thus is accompanied by apposition of apical organelles with the host cell membrane (Ward and Jack, 1981). Similarly with Theileria, Babesia internalizes within a few minutes without parasitophorous vacuole formation (Table 1), and thus the parasite lies freely within the host cell cytoplasm (Simpson et al., 1963; Rudzinska et al., 1976; Potgieter and Els, 1977b; Kawai et al., 1999a,b; Guimarães et al., 2003; Montero et al., 2009; Sun et al., 2011; Lobo et al., 2012). The invasion process does not differ for Babesia sensu stricto species (Montero et al., 2009; Asada et al., 2012) and B. microti (Rudzinska, 1976; Rudzinska et al., 1976). The internalized Babesia sporozoites develop into trophozoites (also described as ring stages), which further asexually divide and produce merozoites by a process referred to as merogony (Box 1, Figure 1) (Rudzinska and Trager, 1977; Fawcett et al., 1987; Montero et al., 2009; Lobo et al., 2012). Later, merozoites are released from ruptured cells and invade other intact and healthy erythrocytes. The merogony of piroplasms is asynchronous (Table 1), and thus trophozoites and merozoites occur in the bloodstream simultaneously (Jalovecka et al., 2016). The short-time residence of the parasite outside the host cell is characterized by the appearance of a fuzzy coat created from fibrillary material and hypervariable surface proteins. The coat occurs on the surface of both Theileria and Babesia free merozoites and is cut off during invasion of the host cell (Rudzinska et al., 1976; Shaw, 2003; Montero et al., 2009).
The size of merozoites varies according to the piroplasm species as well as the vertebrate host species. The merozoites of piroplasms are characterized by a piriform shape, forming pairs or tetrads (Potgieter and Els, 1977a,b; Lewis et al., 1980; Mehlhorn and Shein, 1984; Conrad et al., 1985, 1986; Kawai et al., 1986, 1999b; Gorenflot et al., 1991, 1992; Shaw and Tilney, 1995; Shaw, 2003; Wise et al., 2013; Del Carmen Terrón et al., 2016). However, divergently shaped merozoites were recorded for species of two early divergent lineages of piroplasms, the Western Babesia clade and the Babesia microti clade (Table 1) (Rudzinska, 1976; Kjemtrup et al., 2006; Clancey et al., 2010). They possess smaller merozoites of ovoid shape, which later become polymorphic and form numerous invaginations and pseudopods, twisting and coiling. Although all piroplasms exhibit a much reduced apical complex (e.g., absence of conoid) compared to the other apicomplexan parasites (Votypka et al., 2017), B. microti merozoites apical complex displays only a single large rhoptry and lack polar rings and a microtubular section (Rudzinska, 1976). The question of feeding mechanisms of piroplasms has not yet been answered. There is a general consensus that piroplasms phagocytose or pinocytose the host cytoplasm (Rudzinska and Trager, 1962; Conrad et al., 1985; Fawcett et al., 1987; Guimarães et al., 2003), but food vacuoles full of host cytoplasm were observed in both Theileria and Babesia merozoites, suggesting potential extracellular digestion of host cytoplasm (Rudzinska and Trager, 1962; Simpson et al., 1963; Rudzinska, 1976; Rudzinska et al., 1976; Simpson and Neal, 1980; Conrad et al., 1985; Fawcett et al., 1987; Guimarães et al., 2003). If piroplasms can directly digest host hemoglobin still remains a question; this phenomenon was so far suggested only for some Theileria species but potential host hemoglobin digestion is not accompanied by pigment or other visible residues formation (Votypka et al., 2017).
Gamogony: Sexual Reproduction in the Gut of the Tick Vector
The first sexual stages of piroplasms are referred to as gametocytes (misinterpreted as gamonts in older studies) and appear in the host red blood cells (Box 1, Figure 1, Table 1) (Rudzinska et al., 1979; Mehlhorn and Shein, 1984; Mackenstedt et al., 1990; MacKenstedt et al., 1995; Mehlhorn and Schein, 1993; Gauer et al., 1995; Becker et al., 2010; Bastos et al., 2013; Jalovecka et al., 2016). Gametocytes are predetermined to further differentiate into gametes in the lumen of the tick gut (Rudzinska et al., 1979; Bishop et al., 2004; Becker et al., 2010, 2013), and thus mediate the ability to infect the tick vector (Uilenberg, 2006; Lobo et al., 2012; Becker et al., 2013). Unlike the normally growing and asexually reproducing merozoites, the gametocytes do not reproduce (Rudzinska et al., 1979; Mackenstedt et al., 1990; MacKenstedt et al., 1995; Lobo et al., 2012). They are believed to be larger and unusually shaped compared to asexual stages, however, light microscopy does not allow their reliable recognition (Rudzinska et al., 1979; Lobo et al., 2012). As documented for B. microti by electron microscopy, intra-erythrocytic gametocytes are characterized by undifferentiated cytoplasm, large nuclei and unusually twisted, convoluted or folded shapes (Rudzinska et al., 1979). The gametocytes persistence in the host bloodstream was documented for many species of Babesia sensu stricto (Mackenstedt et al., 1990; MacKenstedt et al., 1995; Becker et al., 2010, 2013; Bastos et al., 2013; Jalovecka et al., 2016) and B. microti (Rudzinska et al., 1979, 1983b). It is generally assumed that sexual commitment of Theileria is identical to Babesia and gametocytes occur in the circulating blood (Mehlhorn and Shein, 1984; Mehlhorn and Schein, 1993; Zapf and Schein, 1994b; Gauer et al., 1995; Shaw, 2003; Bishop et al., 2004; Uilenberg, 2006). This is supported by recent descriptions of genes with expression specific to sexual commitment in intra-erythrocytic stages of Theileria sensu stricto species (Pieszko et al., 2015; Lempereur et al., 2017) and T. equi (Bastos et al., 2013). The gametocytes are taken up into the tick midgut together with the blood meal. Subsequently, ingested asexual intra-erythrocytic stages are rapidly destroyed in the gut lumen (Rudzinska et al., 1979; Mehlhorn and Shein, 1984; Bishop et al., 2004; Lobo et al., 2012). Still hidden inside the red blood cells, gametocytes of both Theileria and Babesia parasites start reorganizing the cytoplasm (Schein et al., 1977; Rudzinska et al., 1979; Mehlhorn et al., 1980; Zapf and Schein, 1994b). Gametocyte metamorphosis is asynchronous, presumably due to non-contemporary blood uptake (Rudzinska et al., 1984). The process is accompanied by microtubular reorganization and gametocytes became completely stretched out compared to previously folded intra-erythrocytic forms (Friedhoff and Büscher, 1976; Weber and Friedhoff, 1977; Rudzinska et al., 1979; Mehlhorn and Shein, 1984; Zapf and Schein, 1994b). As documented for B. microti, development of the gametocytes is completed outside of the already lysed erythrocytes in the lumen of the midgut (Rudzinska et al., 1979). Yet, in some cases the process can be completed inside the erythrocyte in the environment of the tick lumen (Rudzinska et al., 1984; Gough et al., 1998).
Metamorphosis of the gametocytes results in the formation of gametes (Figure 1), referred to as Strahlenkörper or spiky-rayed stages (Table 1) (Mehlhorn and Schein, 1993). It was suggested that gametes multiply to form large aggregates but once division is completed, haploid gametes are released to the tick midgut lumen (Warnecke et al., 1980; Mehlhorn and Schein, 1993; MacKenstedt et al., 1995; Gough et al., 1998; Bock et al., 2004). The appearance of piroplasm gametes is unique among apicomplexan parasites and characteristic structures—tail, arms, and arrowhead—begin to form in gametocytes (Rudzinska et al., 1984). Gametes of both Theileria and Babesia species are haploid (Mackenstedt et al., 1990; MacKenstedt et al., 1995; Gauer et al., 1995) and are considered to be anisogametes, although Babesia gametes appear as isogametes when examined by light microscopy (Mehlhorn and Shein, 1984; Gough et al., 1998). The gametes of Theileria sensu stricto and T. equi are clearly distinguishable by light microscopy as micro- and macro-gametes (Table 1). The characteristic ray bodies are considered to be micro-gametes and macro-gametes are spherically shaped without protrusions (Schein et al., 1977; Warnecke et al., 1980; Mehlhorn and Shein, 1984; Mehlhorn and Schein, 1993; Zapf and Schein, 1994b; Bishop et al., 2004; Uilenberg, 2006). Gametes of Babesia sensu stricto species as well as B. microti do not differentiate into macro- and micro-gametes but two gamete populations are formed (Table 1). These two types differ in the details of cytoplasm density and shape (Friedhoff and Büscher, 1976; Rudzinska et al., 1979, 1983b; Mehlhorn et al., 1981; Gough et al., 1998).
Fertilization of piroplasms is induced by close contact between two gametes of different types and may occur at very early stages of gamete formation (Rudzinska et al., 1983b). Filamentous structures are formed between membranes of closely adjacent gametes. Subsequently, a finger-like protrusions of one gamete penetrates the opposite one (Mehlhorn et al., 1981; Rudzinska et al., 1983b; Mehlhorn and Shein, 1984). Gamete fertilization results in the formation of a zygote (Schein et al., 1977; Mehlhorn et al., 1979; Warnecke et al., 1980; Rudzinska et al., 1983b, 1984; Mackenstedt et al., 1990; MacKenstedt et al., 1995; Higuchi et al., 1991a, 1999a,b; Zapf and Schein, 1994b; Gauer et al., 1995; Gough et al., 1998; Bishop et al., 2004). The zygote of Piroplasmida is a motile stage that is often referred to as an ookinete or kinete. However, such nomenclature is misleading since kinetes (often also called ookinetes or sporokinetes) represent haploid stages resulting from the meiotic division of a diploid zygote (Mehlhorn et al., 1978, 1979; Mehlhorn and Shein, 1984; Rudzinska et al., 1984). To further develop, the zygote penetrates the peritrophic matrix (Figure 1, Table 1) (Rudzinska et al., 1982), which appears temporarily during feeding at all tick stages and compartments the gut lumen into endo-peritrophic and ecto-peritrophic spaces (Sonenshine, 1991). Since the peritrophic matrix represents a strong mechanical barrier, zygote penetration is an active process accomplished by enzymes released from the arrowhead structure of the zygote (Rudzinska et al., 1982, 1984). Matrix penetration starts immediately after zygote formation (Rudzinska et al., 1982, 1983a,b). The arrowhead structure opens the way for the zygote body by release of enzymes. Subsequently, the zygote enters the ecto-peritrophic space and immediately invades gut epithelial cells. This cell invasion is triggered by the arrowhead structure of the zygote but the arrowhead does not pierce the cell membrane; the membrane remains intact. Once the zygote is internalized into the epithelial cell, the invagination membrane disappears. Thus, the zygote occurs loosely in the cytoplasm of the epithelial cell and is surrounded by cell organelles. Inside the epithelial cell, the zygote turns into a spherical shape. Simultaneously, the arrowhead structure loses its organized pattern and gradually disappears (Rudzinska et al., 1982, 1983a). Zygote penetration of the peritrophic matrix and internalization into epithelial cells has been described in detail only for B. microti species but is generally assumed to be consistent for all Piroplasmida (Mehlhorn and Schein, 1993). Once morphological changes are finalized, the zygote undergoes a meiotic division as evidenced by DNA measurements of Theileria and Babesia species (Gauer et al., 1995; MacKenstedt et al., 1995). Meiosis inside the epithelial cell results in the formation of kinetes as was documented for species of both Theileria sensu stricto and Babesia sensu stricto lineages, as well as for T. equi and B. microti (Table 1) (Potgieter et al., 1976; Potgieter and Els, 1977a; Mehlhorn et al., 1978; Warnecke et al., 1980; Rudzinska et al., 1984; Zapf and Schein, 1994b).
The kinetes are released from the gut epithelial cells into the tick haemolymph (Figure 1) (Potgieter et al., 1976; Potgieter and Els, 1977a; Mehlhorn et al., 1978, 1979; Schein and Friedhoff, 1978; Warnecke et al., 1980; Mehlhorn and Shein, 1984; Rudzinska et al., 1984; Karakashian et al., 1986; Higuchi et al., 1991b; Zapf and Schein, 1994b), where the motile kinetes are disseminated via the haemolymph throughout the whole tick body and invade internal tissues. Kinetes are primarily uni-nucleated but exceptionally, kinetes with more nuclei can occur in the haemolymph due to the early beginning of nuclear division (Mehlhorn and Schein, 1993). In the haemolymph, as other invasive stages of piroplasms, kinetes are covered with a fuzzy coat created from fibrillary material and hypervariable surface proteins (Karakashian et al., 1986). The kinetes of Babesia sensu stricto species are subjected to two cycles of asexual multiplication (Table 1) (Potgieter and Els, 1977a; Mehlhorn et al., 1980; Mehlhorn and Shein, 1984; Mehlhorn and Schein, 1998). In the first, the Babesia kinetes invade various tick tissues like haemocytes, muscle fibers, Malpighian tubules, peritracheal cells, and ovaries of adult females. Here, the kinetes undergo the second asexual multiplication (Potgieter and Els, 1977a; Moltmann et al., 1982b; Mehlhorn and Shein, 1984). Subsequently, the secondary kinetes invade the salivary glands where sporogony, the maturation of sporozoites, takes place (Christophers, 1907; Friedhoff et al., 1972; Potgieter and Els, 1976; Weber and Friedhoff, 1979; Moltmann et al., 1982a; Mosqueda et al., 2004). Species of the Babesia sensu stricto lineage possess a unique feature among all apicomplexan parasites; transovarial transmission (Figure 1, Table 1). This process is mediated by Babesia invasion into the ovarian cells and transmission via larval progeny to tick larvae (Joyner et al., 1963; Donnelly and Peirce, 1975; Lewis and Young, 1980; Moltmann et al., 1982b; Mehlhorn and Shein, 1984; Higuchi et al., 1993; Mehlhorn and Schein, 1993; Bonnet et al., 2007; Boldbaatar et al., 2008, 2010). No transovarial transmission occurs in the lifecycle of B. microti or Theileria species (Table 1). The kinetes of B. microti primarily invade fat body (nephrocytes) and salivary glands. Inside, the kinetes form the kinetoblast, which differentiates to produce secondary kinetes. Subsequently, secondary kinetes invade salivary glands to undergo sporogony (Karakashian et al., 1986). Kinetes of Theileria sensu stricto species and T. equi are believed to migrate directly to salivary glands (Table 1) as no kinete invasion of other tick tissues has been documented (Mehlhorn et al., 1979; Moltmann et al., 1983b; Mehlhorn and Shein, 1984; Mehlhorn and Schein, 1993, 1998; Zapf and Schein, 1994a; Uilenberg, 2006).
Remarkable differences have been documented in size and chronological order of piroplasm sexual development. Such divergence can be attributed to the variety of the piroplasm species and the wide spectrum of both vertebrate hosts and vectors. Gametes of Babesia parasites develop during tick feeding, appear before full tick engorgement and within ~3 days post tick repletion. Subsequently, the kinetes are found in the tick haemolymph from ~2 to ~6 days post repletion. Theileria sexual development seems to be a longer process; the first appearance of gametes was documented between ~1 and ~5 days post tick repletion and kinetes released to the haemolymph were first seen from ~13 to ~34 days post repletion. In general, the length of piroplasm sexual development correlates with the feeding duration of tick developmental stages (larvae vs. nymphs vs. adults), and tick developmental differences derived from the number of host species (one- vs. two- vs. three-host ticks) (Friedhoff and Büscher, 1976; Potgieter et al., 1976; Potgieter and Els, 1977a; Schein et al., 1977; Weber and Friedhoff, 1977; Mehlhorn et al., 1978, 1979, 1980; Warnecke et al., 1980; Rudzinska et al., 1982, 1983a,b, 1984; Mehlhorn and Shein, 1984; Mackenstedt et al., 1990; MacKenstedt et al., 1995; Higuchi et al., 1991a, 1992, 1999a,b; Mehlhorn and Schein, 1993; Zapf and Schein, 1994b; Gauer et al., 1995; Gough et al., 1998).
Sporogony: Asexual Reproduction in the Salivary Gland of the Tick Vector
The kinetes of Piroplasmida parasites further develop in tick salivary glands to produce invasive stages referred to as sporozoites (Figure 1). The sporozoites mediate parasite transmission from the tick vector to the vertebrate host. Piroplasms develop in acini of types II and III (Fawcett et al., 1982a,b), which represent the majority of acini in the typical grape-like structure of salivary glands (Coons and Roshdy, 1973; Binnington, 1978). Sporogony (Box 1) begins with invasion of tick salivary glands by piroplasm kinetes (Christophers, 1907; Holbrook et al., 1968; Purnell and Joyner, 1968; Friedhoff et al., 1972; Potgieter and Els, 1976; Schein et al., 1979; Weber and Friedhoff, 1979; Weber and Walter, 1980; Fawcett et al., 1982a,b; Moltmann et al., 1982a, 1983b; Karakashian et al., 1983; Blouin and van Rensburg, 1988; Blouin and De Waal, 1989; Higuchi et al., 1994; Zapf and Schein, 1994a; Guimarães et al., 1998a,b). Invading kinetes rapidly enlarge and transform into the polymorphous single-membrane syncytium referred to as a sporont. Later, the sporont evolves into a sporoblast, a multinucleated and relatively undifferentiated three-dimensional branching meshwork which has already developed before the beginning of tick ecdysis and molting. The formation of a sporoblast is associated with hypertrophy of the infected acini cells (Table 1), which is a common feature of sporogony in Theileria sensu stricto species (Purnell and Joyner, 1968; Schein and Friedhoff, 1978; Mehlhorn et al., 1979; Fawcett et al., 1982a,b), T. equi (Zapf and Schein, 1994a) and B. microti (Weber and Walter, 1980; Karakashian et al., 1983; Piesman et al., 1986; Yano et al., 2005). The same phenomena were documented for some Babesia sensu stricto species like B. bovis (Potgieter and Els, 1976) and B. canis (Schein et al., 1979) but not for B. ovis (Friedhoff et al., 1972; Moltmann et al., 1982a) or B. caballi (Blouin and De Waal, 1989). During tick ecdysis, the sporoblast appears to be dormant and its maturation starts when the molted tick attaches to the host. However, a unique formation of fully matured sporozoites prior to tick molting was documented for T. equi (Table 1). Sporozoites developed during parasite acquisition and were competent to transmit and expand the infection to the naïve host. Later, the secondary sporozoites developed after attachment of the molted tick stages (Zapf and Schein, 1994a).
Sporoblast maturation of Babesia sensu stricto species begins with the appearance of numerous cytomeres (Table 1) (Friedhoff et al., 1972; Potgieter and Els, 1977a; Schein et al., 1979; Moltmann et al., 1982a) but cytomere formation was absent in Theileria species (Fawcett et al., 1982a,b; Hazen-Karr et al., 1987) and B. microti (Karakashian et al., 1983). The structures of the apical complex appear subsequently, but prior to the sporozoites budding off the sporoblast (Karakashian et al., 1983). Some skeletal components of the apical complex—the conoid and microtubules—are absent in sporozoites of all piroplasms (Friedhoff et al., 1972; Schein and Friedhoff, 1978; Mehlhorn et al., 1979; Schein et al., 1979; Fawcett et al., 1982a,b; Karakashian et al., 1983; Moltmann et al., 1983b; Piesman et al., 1986; Zapf and Schein, 1994a). Polar rings are formed in sporozoites of Babesia sensu stricto species (Table 1) (Friedhoff et al., 1972; Schein et al., 1979; Weber and Friedhoff, 1979; Moltmann et al., 1982a; Blouin and van Rensburg, 1988; Blouin and De Waal, 1989) and T. equi (Moltmann et al., 1983b) but do not appear in sporozoites of B. microti (Karakashian et al., 1983; Yano et al., 2005) and Theileria sensu stricto species (Fawcett et al., 1982a,b). Thus, B. microti sporogony is more reminiscent of Theileria sensu stricto species with respect to sporoblast structure. The formation of sporozoites is attributed to the process of multiple fissions, referred to as budding (Table 1). Apart from B. canis, where sporozoite differentiation was described as a result of successive binary fissions (Schein et al., 1979), the budding process was documented for both Theileria and Babesia species (Holbrook et al., 1968; Friedhoff et al., 1972; Potgieter and Els, 1976; Schein and Friedhoff, 1978; Fawcett et al., 1982a; Moltmann et al., 1982a, 1983b; Hazen-Karr et al., 1987; Blouin and van Rensburg, 1988; Blouin and De Waal, 1989). Since the parasitophorous vacuole is not formed, the sporogony stages are in immediate contact with the host-cell cytoplasm (Friedhoff et al., 1972; Fawcett et al., 1982a; Moltmann et al., 1982a, 1983b). Piroplasm sporogony is asynchronous and the various developmental stages occur within individual acinar cells (Karakashian et al., 1983; Blouin and van Rensburg, 1988; Yano et al., 2005). This is attributed to the continuous release of sporozoites into the tick saliva and to the bloodstream of the vertebrate host during the several days of tick feeding (Yano et al., 2005). Sporogony ends with longish piriform sporozoites equipped with apical organelles that later mediate internalization to the host blood cell.
Sporogenic events and progress are assumed to be consistent for both Babesia and Theileria species (Christophers, 1907; Purnell and Joyner, 1968; Friedhoff et al., 1972; Potgieter and Els, 1976; Weber and Friedhoff, 1979; Fawcett et al., 1982a; Moltmann et al., 1982a, 1983b; Karakashian et al., 1983; Higuchi et al., 1994; Zapf and Schein, 1994a; Guimarães et al., 1998a,b; Mehlhorn and Schein, 1998; Mosqueda et al., 2004) but alteration in the process length and sporozoite size occurs. Such a discrepancy is attributed to the variety of tick species and their natural developmental characteristics such as the number of host species (one- vs. two- vs. three-host ticks). Generally, sporozoite maturation after tick attachment to the host lasts at least 48 h, as documented for representatives of lineages Babesia sensu stricto, Theileria sensu stricto, T. equi and B. microti (Mehlhorn et al., 1979; Karakashian et al., 1983; Takahashi et al., 1993; MacKenstedt et al., 1995; Guimarães et al., 1998a,b).
Conclusion and Future Perspectives: The Role of the Life Cycle in Anti-piroplasm Strategies
Species of the group Piroplasmida possess a characteristic lifecycle that significantly differs from other apicomplexan parasites. A uniform consensus describes three consecutive phases (Figure 1): (i) schizogony and merogony, asexual multiplication in blood cells of the vertebrate host; (ii) gamogony, sexual reproduction inside the tick midgut, later followed by kinete invasion of the tick internal tissues; and (iii) sporogony, asexual proliferation in tick salivary glands resulting in the formation of sporozoites (Box 1). However, the order Piroplasmida includes many species spread into five evolutionary distinct lineages. Thus, in the lifecycles of piroplasms many fundamental variations occur from the general consensus and these discrepancies need to be taken into account in the development of anti-piroplasm strategies.
To date, the majority of inventions in anti-piroplasm vaccine development exploits the vertebrate host stages: schizonts and/or merozoites (Florin-Christensen et al., 2014; Nene and Morrison, 2016). Trends in anti-babesial strategies operate particularly with subunit vaccines based on merozoite surface antigens since recently, many of these have been characterized (recently reviewed in Florin-Christensen et al., 2014). In general, these surface antigens exhibit high immunogenicity and antibodies against them are able to mediate inhibition of parasite intra-erythrocytic invasion and development (Florin-Christensen et al., 2014). Analogously, surface antigens of schizont-infected cells represent hot candidates for anti-theilerial vaccines (Nene and Morrison, 2016). Sporozoites, crucial piroplasm stages responsible for parasite transmission from the tick vector to the vertebrate host, are currently the center of interest for anti-piroplasm strategies (Florin-Christensen et al., 2014; Nene and Morrison, 2016; Nene et al., 2016) but this research is restricted by the absence of effective laboratory transmission models, particularly for species in lineages of T. equi and B. microti. So far, a few sporozoite surface antigens have been defined but they generally displayed a low level of immunogenicity (Florin-Christensen et al., 2014; Nene and Morrison, 2016; Nene et al., 2016). However, antibodies neutralizing sporozoite infectivity have been demonstrated in animals exposed to repeated sporozoite challenges (Nene and Morrison, 2016). On the contrary, very limited knowledge of intra-tick developmental stages restricts research on tick-pathogen interactions. Up to now, only few vaccine candidates were defined for species, particularly of the Babesia sensu stricto lineage, and partially also of the B. microti group (Hajdušek et al., 2013; de la Fuente et al., 2017) but there is lack of knowledge about Theileria and tick interactions. Although intra-tick development differs among all piroplasm evolutionary lineages, comparative bioinformatics analysis implies a high level of conservation of crucial regulatory domains responsible for piroplasm life cycle transitions (Alzan et al., 2016). Targeting key Babesia or Theileria developmental stages in tick tissues represents an attractive way toward transmission-blocking vaccines. However, this research requires in depth knowledge of parasite intra-tick development with a strong focus on conserved or divergent developmental features.
Author Contributions
MJ, OH, and LM conceived and designed the review. DS and PK contributed ideas and concepts. MJ did the literature search, wrote the manuscript, and designed the figure. MJ, OH, DS, PK, and LM finalized the paper and figure.
Funding
This work was primarily supported by the grants of Czech Science Foundation (GA CR) No. 17-27386S to OH and No. 17-14631S to DS. OH, DS, and PK were also supported by the Centre for research of pathogenicity and virulence of parasites (No. CZ.02.1.01/0.0/0.0/16_019/0000759) funded by European Regional Development Fund (ERDF) and Ministry of Education, Youth and Sport, Czech Republic (MEYS). MJ was additionally supported by GACR grant No. 17-27393S and by the Dual Czech-French PhD. program (Doctorat en co-tutelle) provided by the French Institute in Prague, Czech Republic. LM was supported by her lab's own resources.
Conflict of Interest Statement
The authors declare that the research was conducted in the absence of any commercial or financial relationships that could be construed as a potential conflict of interest.
The reviewer JL declared a shared affiliation, with no collaboration, with several of the authors, MJ, OH, DS, and PF, to the handling Editor.
References
Ahmed, J. S., Schnittger, L., and Mehlhorn, H. (1999). Review: Theileria schizonts induce fundamental alterations in their host cells. Parasitol. Res. 85, 527–538. doi: 10.1007/s004360050592
Alzan, H. F., Knowles, D. P., and Suarez, C. E. (2016). Comparative bioinformatics analysis of transcription factor genes indicates conservation of key regulatory domains among Babesia bovis, Babesia microti, and Theileria equi. PLoS Negl. Trop. Dis. 10:e0004983. doi: 10.1371/journal.pntd.0004983
Arisue, N., and Hashimoto, T. (2014). Phylogeny and evolution of apicoplasts and apicomplexan parasites. Parasitol. Int. 64, 254–259. doi: 10.1016/j.parint.2014.10.005
Asada, M., Goto, Y., Yahata, K., Yokoyama, N., Kawai, S., Inoue, N., et al. (2012). Gliding motility of Babesia bovis merozoites visualized by time-lapse video microscopy. PLoS ONE 7:e35227. doi: 10.1371/journal.pone.0035227
Baneth, G., Florin-Christensen, M., Cardoso, L., and Schnittger, L. (2015). Reclassification of Theileria annae as Babesia vulpes sp. nov. Parasit. Vectors 8:207. doi: 10.1186/s13071-015-0830-5
Bastos, R. G., Suarez, C. E., Laughery, J. M., Johnson, W. C., Ueti, M. W., and Knowles, D. P. (2013). Differential expression of three members of the multidomain adhesion CCp family in Babesia bigemina, Babesia bovis and Theileria equi. PLoS ONE 8:e67765. doi: 10.1371/journal.pone.0067765
Becker, C. A., Malandrin, L., Depoix, D., Larcher, T., David, P. H., Chauvin, A., et al. (2010). Identification of three CCp genes in Babesia divergens: novel markers for sexual stages parasites. Mol. Biochem. Parasitol. 174, 36–43. doi: 10.1016/j.molbiopara.2010.06.011
Becker, C. A., Malandrin, L., Larcher, T., Chauvin, A., Bischoff, E., and Bonnet, S. I. (2013). Validation of BdCCp2 as a marker for Babesia divergens sexual stages in ticks. Exp. Parasitol. 133, 51–56. doi: 10.1016/j.exppara.2012.10.007
Binnington, K. C. (1978). Sequential changes in salivary gland structure during attachment and feeding of the cattle tick, Boophilus microplus. Int. J. Parasitol. 8, 97–115. doi: 10.1016/0020-7519(78)90004-8
Bishop, R., Musoke, A., Morzaria, S., Gardner, M., and Nene, V. (2004). Theileria: intracellular protozoan parasites of wild and domestic ruminants transmitted by ixodid ticks. Parasitology 129, 271–283. doi: 10.1017/S0031182003004748
Bishop, R. P., Hemmink, J. D., Morrison, W. I., Weir, W., Toye, P. G., Sitt, T., et al. (2015). The African buffalo parasite Theileria. sp. (buffalo) can infect and immortalize cattle leukocytes and encodes divergent orthologues of Theileria parva antigen genes. Int. J. Parasitol. Parasites Wildl. 4, 333–342. doi: 10.1016/j.ijppaw.2015.08.006
Blouin, E. F., and De Waal, D. T. (1989). The fine structure of developmental stages of Babesia caballiin the salivary glands of Hyalomma truncatum. Onderstepoort J. Vet. Res. 56, 189–193.
Blouin, E. F., Kocan, A. A., Kocan, K. M., and Hair, J. (1987). Evidence of a limited schizogonous cycle for Cytauxzoon felis in bobcats following exposure to infected ticks. J. Wildl. Dis. 23, 499–501. doi: 10.7589/0090-3558-23.3.499
Blouin, E. F., and van Rensburg, L. (1988). An ultrastructural study of the development of Babesia occultans in the salivary glands of adult Hyalomma marginatum rufipes. Onderstepoort J. Vet. Res. 55, 93–100.
Bock, R., Jackson, L., de Vos, A., and Jorgensen, W. (2004). Babesiosis of cattle. Parasitol. 129(Suppl.) S247–S269. doi: 10.1017/S0031182004005190
Boldbaatar, D., Battsetseg, B., Matsuo, T., Hatta, T., Umemiya-Shirafuji, R., Xuan, X., et al. (2008). Tick vitellogenin receptor reveals critical role in oocyte development and transovarial transmission of Babesia parasite. Biochem. Cell Biol. 86, 331–344. doi: 10.1139/O08-071
Boldbaatar, D., Umemiya-Shirafuji, R., Liao, M., Tanaka, T., Xuan, X., and Fujisaki, K. (2010). Multiple vitellogenins from the Haemaphysalis longicornis tick are crucial for ovarian development. J. Insect Physiol. 56, 1587–1598. doi: 10.1016/j.jinsphys.2010.05.019
Bonnet, S., Jouglin, M., Malandrin, L., Becker, C., Agoulon, A., L'hostis, M., et al. (2007). Transstadial and transovarial persistence of Babesia divergens DNA in Ixodes ricinus ticks fed on infected blood in a new skin-feeding technique. Parasitology 134, 197–207. doi: 10.1017/S0031182006001545
Burki, F., Inagaki, Y., Bråte, J., Archibald, J. M., Keeling, P. J., Cavalier-Smith, T., et al. (2009). Large-scale phylogenomic analyses reveal that two enigmatic protist lineages, telonemia and centroheliozoa, are related to photosynthetic chromalveolates. Genome Biol. Evol. 1, 231–238. doi: 10.1093/gbe/evp022
Christophers, S. R. (1907). Preliminary note on the development of Piroplasma canis in the tick. Br. Med. J. 1, 76–78. doi: 10.1136/bmj.1.2402.76
Clancey, N., Horney, B., Burton, S., Birkenheuer, A., McBurney, S., and Tefft, K. (2010). Babesia (Theileria) annae in a red fox (Vulpes vulpes) from Prince Edward Island, Canada. J. Wildl. Dis. 46, 615–621. doi: 10.7589/0090-3558-46.2.615
Conrad, P. A., Denham, D., and Brown, C. G. (1986). Intraerythrocytic multiplication of Theileria parva in vitro: an ultrastructural study. Int. J. Parasitol. 16, 223–229. doi: 10.1016/0020-7519(86)90047-0
Conrad, P. A., Kelly, B. G., and Brown, C. G. (1985). Intraerythrocytic schizogony of Theileria annulata. Parasitology 91, 67–82. doi: 10.1017/S0031182000056523
Coons, L. B., and Roshdy, M. A. (1973). Fine structure of the salivary glands of unfed male Dermacentor variabilis (Say) (Ixodoidea: Ixodidae). J. Parasitol. 59, 900–912. doi: 10.2307/3278433
de la Fuente, J., Antunes, S., Bonnet, S., Cabezas-Cruz, A., Domingos, A. G., Estrada-Peña, A., et al. (2017). Tick-pathogen interactions and vector competence: identification of molecular drivers for tick-borne diseases. Front. Cell. Infect. Microbiol. 7:114. doi: 10.3389/fcimb.2017.00114
Del Carmen Terrón, M., González-Camacho, F., González, L. M., Luque, D., and Montero, E. (2016). Ultrastructure of the Babesia divergens free merozoite. Ticks Tick Borne Dis. 7, 1274–1279. doi: 10.1016/j.ttbdis.2016.07.001
Dobbelaere, D., and Heussler, V. (1999). Transformation of leukocytes by Theileria parva and T. annulata. Annu. Rev. Microbiol. 53, 1–42. doi: 10.1146/annurev.micro.53.1.1
Dobbelaere, D. A., and Küenzi, P. (2004). The strategies of the Theileria parasite: a new twist in host-pathogen interactions. Curr. Opin. Immunol. 16, 524–530. doi: 10.1016/j.coi.2004.05.009
Dobbelaere, D. A., and Rottenberg, S. (2003). Theileria-induced leukocyte transformation. Curr. Opin. Microbiol. 6, 377–382. doi: 10.1016/S1369-5274(03)00085-7
Dobbelaere, D. A., Webster, P., Leitch, B. L., Voigt, W. P., and Irvin, A. D. (1985). Theileria parva: expression of a sporozoite surface coat antigen. Exp. Parasitol. 60, 90–100. doi: 10.1016/S0014-4894(85)80026-6
Donnelly, J., and Peirce, M. A. (1975). Experiments on the transmission of Babesia divergens to cattle by the tick Ixodes ricinus. Int. J. Parasitol. 5, 363–367. doi: 10.1016/0020-7519(75)90085-5
Dubremetz, J. F., Garcia-Réguet, N., Conseil, V., and Fourmaux, M. N. (1998). Apical organelles and host-cell invasion by Apicomplexa. Int. J. Parasitol. 28, 1007–1013. doi: 10.1016/S0020-7519(98)00076-9
Fawcett, D., Musoke, A., and Voigt, W. (1984). Interaction of sporozoites of Theileria parva with bovine lymphocytes in vitro. I. Early events after invasion. Tissue Cell 16, 873–884. doi: 10.1016/0040-8166(84)90068-5
Fawcett, D. W., Büscher, G., and Doxsey, S. (1982a). Salivary gland of the tick vector of East Coast fever. III. The ultrastructure of sporogony in Theileria parva. Tissue Cell 14, 183–206. doi: 10.1016/0040-8166(82)90017-9
Fawcett, D. W., Büscher, G., and Doxsey, S. (1982b). Salivary gland of the tick vector of East Coast fever. IV. Cell type selectivity and host cell responses to Theileria parva. Tissue Cell 14, 397–414. doi: 10.1016/0040-8166(82)90035-0
Fawcett, D. W., Conrad, P. A., Grootenhuis, J. G., and Morzaria, S. P. (1987). Ultrastructure of the intra-erythrocytic stage of Theileria species from cattle and waterbuck. Tissue Cell 19, 643–655. doi: 10.1016/0040-8166(87)90071-1
Fawcett, D. W., Doxsey, S., Stagg, D. A., and Young, A. S. (1982c). The entry of sporozoites of Theileria parva into bovine lymphocytes in vitro. Electron microscopic observations. Eur. J. Cell Biol. 27, 10–21.
Florin-Christensen, M., Suarez, C. E., Rodriguez, A. E., Flores, D. A., and Schnittger, L. (2014). Vaccines against bovine babesiosis: where we are now and possible roads ahead. Parasitology 28, 1–30. doi: 10.1017/S0031182014000961
Friedhoff, K., Scholtyseck, E., and Weber, G. (1972). Fine structure of the merozoites of Babesia ovis in the salivary glands of female ticks. Z. Parasitenkd. 38, 132–140. doi: 10.1007/BF00329024
Friedhoff, K. T., and Büscher, G. (1976). Rediscovery of Koch's “strahlenörper” of Babesia bigemina. Z. Parasitenkd. 50, 345–347. doi: 10.1007/BF02462979
Gauer, M., Mackenstedt, U., Mehlhorn, H., Schein, E., Zapf, F., Njenga, E., et al. (1995). DNA measurements and ploidy determination of developmental stages in the life cycles of Theileria annulata and T. parva. Parasitol. Res. 81, 565–574. doi: 10.1007/BF00932023
Gohil, S., Herrmann, S., Günther, S., and Cooke, B. M. (2013). Bovine babesiosis in the 21st century: advances in biology and functional genomics. Int. J. Parasitol. 43, 125–132. doi: 10.1016/j.ijpara.2012.09.008
Gorenflot, A., Brasseur, P., Precigout, E., L'Hostis, M., Marchand, A., and Schrevel, J. (1991). Cytological and immunological responses to Babesia divergens in different hosts: ox, gerbil, man. Parasitol. Res. 77, 3–12. doi: 10.1007/BF00934377
Gorenflot, A., Precigout, E., Valentin, A., Bissuel, G., Carcy, B., Brasseur, P., et al. (1992). Babesia divergens vaccine. Mem. Inst. Oswaldo Cruz. 87, 279–281. doi: 10.1590/S0074-02761992000700047
Gough, J. M., Jorgensen, W. K., and Kemp, D. H. (1998). Development of tick gut forms of Babesia bigemina in vitro. J. Eukaryot. Microbiol. 45, 298–306. doi: 10.1111/j.1550-7408.1998.tb04540.x
Guimarães, A. M., Lima, J. D., and Ribeiro, M. F. B. (1998a). Sporogony and experimental transmission of Babesia equi by Boophilus microplus. Parasitol. Res. 84, 323–327. doi: 10.1007/s004360050404
Guimarães, A. M., Lima, J. D., Ribeiro, M. F. B., Camargos, E. R. S., and Bozzi, I. A. (1998b). Ultrastructure of sporogony in Babesia equi in salivary glands of adult female Boophilus microplus ticks. Parasitol. Res. 84, 69–74.
Guimarães, A. M., Lima, J. D., and Ribeiro, M. F. B. (2003). Ultrastructure of Babesia equi trophozoites isolated in Minas Gerais, Brazil. Pesq. Vet. Bras. 23, 101–104. doi: 10.1590/S0100-736X2003000300002
Hagiwara, K., Takahashi, K., Taniyama, H., Kawamoto, S., Kurosawa, T., Ikuta, K., et al. (1997). Detection of Theileria sergenti schizonts in bovine lymph node. Int. J. Parasitol. 27, 1375–1378. doi: 10.1016/S0020-7519(97)00092-1
Hajdušek, O., Síma, R., Ayllón, N., Jalovecká, M., Perner, J., de la Fuente, J., et al. (2013). Interaction of the tick immune system with transmitted pathogens. Front. Cell. Infect. Microbiol. 3:26. doi: 10.3389/fcimb.2013.00026
Hazen-Karr, C. G., Kocan, A. A., Kocan, K. M., and Hair, J. A. (1987). The ultrastructure of sporogony in Theileria cervi (Bettencourt et al., 1907) in salivary glands of female Amblyomma americanum (L.) ticks. J. Parasitol. 73, 1182–1188. doi: 10.2307/3282304
Higuchi, S., Izumitani, M., Hoshi, H., Kawamura, S., and Yasuda, Y. (1999a). Development of Babesia gibsoni in the midgut of larval tick, Rhipicephalus sanguineus. J. Vet. Med. Sci. 61, 689–691. doi: 10.1292/jvms.61.689
Higuchi, S., Konno, H., Hoshi, F., Kawamura, S., and Yasuda, Y. (1993). Observations of Babesia gibsoni in the ovary of the tick, Haemaphysalis longicornis. Kitasato. Arch. Exp. Med. 65, 153–158.
Higuchi, S., Kuroda, H., Hoshi, H., Kawamura, S., and Yasuda, Y. (1999b). Development of Babesia gibsoni in the midgut of the nymphal stage of the tick, Rhipicephalus sanguineus. J. Vet. Med. Sci. 61, 697–699. doi: 10.1292/jvms.61.697
Higuchi, S., Oya, H., Hoshi, F., Kawamura, S., and Yasuda, Y. (1992). Observations of Babesia gibsoni in midgut epithelial cells of the tick, Haemaphysalis longicornis. Kitasato. Arch. Exp. Med. 65, 143–147.
Higuchi, S., Oya, H., Hoshi, F., Kawamura, S., and Yasuda, Y. (1994). Development of Babesia ovata in the salivary glands of the nymphal tick, Haemaphysalis longicornis. J. Vet. Med. Sci. 56, 207–209. doi: 10.1292/jvms.56.207
Higuchi, S., Simomura, S., Yoshida, H., Hoshi, F., Kawamura, S., and Yasuda, Y. (1991a). Development of Babesia gibsoni in the gut epithelium of the tick, Haemaphysalis longicornis. J. Vet. Med. Sci. 53, 129–131. doi: 10.1292/jvms.53.129
Higuchi, S., Simomura, S., Yoshida, H., Hoshi, F., Kawamura, S., and Yasuda, Y. (1991b). Development of Babesia gibsoni in the hemolymph of the vector tick, Haemaphysalis longicornis. J. Vet. Med. Sci. 53, 491–493. doi: 10.1292/jvms.53.491
Holbrook, A. A., Anthony, D. W., and Johnson, A. J. (1968). Observations on the development of Babesia caballi(Nuttall) in the tropical horse tick Dermacentor nitens Neumann. J. Protozool. 15, 391–396. doi: 10.1111/j.1550-7408.1968.tb02143.x
Homer, M. J., Aguilar-Delfin, I., Telford, S. R., Krause, P. J., and Persing, D. H. (2000). Babesiosis. Clin. Microbiol. Rev. 13, 451–469. doi: 10.1128/CMR.13.3.451-469.2000
Hunfeld, K. P., Hildebrandt, A., and Gray, J. S. (2008). Babesiosis: recent insights into an ancient disease. Int. J. Parasitol. 38, 1219–1237. doi: 10.1016/j.ijpara.2008.03.001
Jalovecka, M., Bonsergent, C., Hajdusek, O., Kopacek, P., and Malandrin, L. (2016). Stimulation and quantification of Babesia divergens gametocytogenesis. Parasit. Vectors 9:439. doi: 10.1186/s13071-016-1731-y
Janouskovec, J., Horák, A., Oborník, M., Lukes, J., and Keeling, P. J. (2010). A common red algal origin of the apicomplexan, dinoflagellate, and heterokont plastids. Proc. Natl. Acad. Sci. U.S.A. 107, 10949–10954. doi: 10.1073/pnas.1003335107
Joyner, L. P., Davies, S. F., and Kendall, S. B. (1963). The experimental transmission of Babesia divergens by Ixodes ricinus. Exp. Parasitol. 14, 367–373. doi: 10.1016/0014-4894(63)90044-4
Kappmeyer, L. S., Thiagarajan, M., Herndon, D. R., Ramsay, J. D., Caler, E., Djikeng, A., et al. (2012). Comparative genomic analysis and phylogenetic position of Theileria equi. BMC Genomics. 13:603. doi: 10.1186/1471-2164-13-603
Karakashian, S. J., Rudzinska, M. A., Spielman, A., Lewengrub, S., and Campbell, J. (1986). Primary and secondary ookinetes of Babesia microti in the larval and nymphal stages of the tick Ixodes dammini. Can. J. Zool. 64, 328–339. doi: 10.1139/z86-053
Karakashian, S. J., Rudzinska, M. A., Spielman, A., Lewengrub, S., Piesman, J., and Shoukrey, N. (1983). Ultrastructural studies on sporogony of Babesia microti in salivary gland cells of the tick Ixodes dammini. Cell Tissue Res. 231, 275–287. doi: 10.1007/BF00222180
Kawai, S., Igarashi, I., Abgaandorjiin, A., Ikadai, H., Omata, Y., Saito, A., et al. (1999a). Tubular structures associated with Babesia caballi in equine erythrocytes in vitro. Parasitol. Res. 85, 171–175. doi: 10.1007/s004360050530
Kawai, S., Igarashi, I., Abgaandorjiin, A., Miyazawa, K., Ikadai, H., Nagasawa, et al. (1999b). Ultrastructural characteristics of Babesia caballi in equine erythrocytes in vitro. Parasitol. Res. 85, 794–799. doi: 10.1007/s004360050635
Kawai, S., Takahashi, K., Kawamoto, S., Nagahara, A., Sonoda, M., Kurosawa, T., et al. (1989). Bar-structure in bovine erythrocytes infected with Theileria sergenti. Nippon Juigaku Zasshi 51, 1219–1225. doi: 10.1292/jvms1939.51.1219
Kawai, S., Takahashi, K., Sonoda, M., and Kurosawa, T. (1986). Ultrastructure of intra-erythrocytic stages of Babesia ovata. Nippon Juigaku Zasshi 48, 943–949. doi: 10.1292/jvms1939.48.943
Kjemtrup, A. M., and Conrad, P. A. (2000). Human babesiosis: an emerging tick-borne disease. Int. J. Parasitol. 30, 1323–1337. doi: 10.1016/S0020-7519(00)00137-5
Kjemtrup, A. M., Wainwright, K., Miller, M., Penzhorn, B. L., and Carreno, R. A. (2006). Babesia conradae, sp. Nov., a small canine Babesia identified in California. Vet. Parasitol. 138, 103–111. doi: 10.1016/j.vetpar.2006.01.044
Leiby, D. A. (2011). Transfusion-transmitted Babesia spp.: bull's-eye on Babesia microti. Clin. Microbiol. Rev. 24, 14–28. doi: 10.1128/CMR.00022-10
Lempereur, L., Larcombe, S. D., Durrani, Z., Karagenc, T., Bilgic, H. B., Bakirci, S., et al. (2017). Identification of candidate transmission-blocking antigen genes in Theileria annulata and related vector-borne apicomplexan parasites. BMC Genomics 18:438. doi: 10.1186/s12864-017-3788-1
Lewis, D., Purnell, R. E., and Shaw, S. R. (1980). The isolation and characterization of human and bovine strains of Babesia divergens from Drumnadrochit, Scotland. Parasitology 81, 145–155. doi: 10.1017/S0031182000055116
Lewis, D., and Young, E. R. (1980). The transmission of a human strain of Babesia divergens by Ixodes ricinus ticks. J. Parasitol. 66, 359–360. doi: 10.2307/3280841
Lobo, C. A., Rodriguez, M., and Cursino-Santos, J. R. (2012). Babesia and red cell invasion. Curr. Opin. Hematol. 19, 170–175. doi: 10.1097/MOH.0b013e328352245a
MacKenstedt, U., Gauer, M., Fuchs, P., Zapf, F., Schein, E., and Mehlhorn, H. (1995). DNA measurements reveal differences in the life cycles of Babesia bigemina and B. canis, two typical members of the genus Babesia. Parasitol. Res. 81, 595–604. doi: 10.1007/BF00932027
Mackenstedt, U., Gauer, M., Mehlhorn, H., Schein, E., and Hauschild, S. (1990). Sexual cycle of Babesia divergens confirmed by DNA measurements. Parasitol. Res. 76, 199–206. doi: 10.1007/BF00930815
Malandrin, L., Jouglin, M., Sun, Y., Brisseau, N., and Chauvin, A. (2010). Redescription of Babesia capreoli (Enigk and Friedhoff, 1962) from roe deer (Capreolus capreolus): isolation, cultivation, host specificity, molecular characterisation and differentiation from Babesia divergens. Int. J. Parasitol. 40, 277–284. doi: 10.1016/j.ijpara.2009.08.008
Mehlhorn, H., Moltmann, U., Schein, E., and Voigt, W. P. (1981). Fine structure of supposed gametes and syngamy of Babesia canis (Piroplasmea) after in-vitro development. Zentralbl. Bakteriol. Mikrobiol. Hyg. A. 250, 248–255.
Mehlhorn, H., and Schein, E. (1993). The piroplasms: “A long story in short” or “Robert Koch has seen it”. Eur. J. Protistol. 29, 279–293. doi: 10.1016/S0932-4739(11)80371-8
Mehlhorn, H., and Schein, E. (1998). Redescription of Babesia equi Laveran, 1901 as Theileria equi Mehlhorn, Schein 1998. Parasitol. Res. 84, 467–475. doi: 10.1007/s004360050431
Mehlhorn, H., Schein, E., and Voigt, W. P. (1980). Light and electron microscopic study on developmental stages of Babesia canis within the gut of the tick Dermacentor reticulatus. J. Parasitol. 66, 220–228. doi: 10.2307/3280808
Mehlhorn, H., Schein, E., and Warnecke, M. (1978). Electron microscopic studies on the development of kinetes of Theileria parva Theiler, 1904 in the gut of the vector ticks Rhipicephalus appendiculatus Neumann, 1901. Acta Trop. 35, 123–136.
Mehlhorn, H., Schein, E., and Warnecke, M. (1979). Electron-microscopic studies on Theileria ovis Rodhain, 1916: development of kinetics in the gut of the vector tick, Rhipicephalus evertsi evertsi Neumann, 1897, and their transformation within cells of the salivary glands. J. Protozool. 26, 377–385. doi: 10.1111/j.1550-7408.1979.tb04640.x
Mehlhorn, H., and Shein, E. (1984). The piroplasms: life cycle and sexual stages. Adv. Parasitol. 23, 37–103. doi: 10.1016/S0065-308X(08)60285-7
Moltmann, U. G., Mehlhorn, H., and Friedhoff, K. T. (1982a). Electron microscopic study on the development of Babesia ovis (Piroplasmia) in the salivary glands of the vector tick Rhipicephalus bursa. Acta Trop. 39, 29–40.
Moltmann, U. G., Mehlhorn, H., and Friedhoff, K. T. (1982b). Ultrastructural study of the development of Babesia ovis (Piroplasmia) in the ovary of the vector tick Rhipicephalus bursa. J. Protozool. 29, 30–38. doi: 10.1111/j.1550-7408.1982.tb02877.x
Moltmann, U. G., Mehlhorn, H., Schein, E., Rehbein, G., Voigt, W. P., and Zweygarth, E. (1983a). Fine structure of Babesia equi Laveran, 1901 within lymphocytes and erythrocytes of horses: an in vivo and in vitro study. J. Parasitol. 69, 111–120. doi: 10.2307/3281285
Moltmann, U. G., Mehlhorn, H., Schein, E., Voigt, W. P., and Friedhoff, K. T. (1983b). Ultrastructural study on the development of Babesia equi (Coccidia: Piroplasmia) in the salivary glands of its vector ticks. J. Protozool. 30, 218–225. doi: 10.1111/j.1550-7408.1983.tb02907.x
Montero, E., Rodriguez, M., Oksov, Y., and Lobo, C. A. (2009). Babesia divergens apical membrane antigen 1 and its interaction with the human red blood cell. Infect. Immun. 77, 4783–4793. doi: 10.1128/IAI.00969-08
Mosqueda, J., Ramos, J. A., Falcon, A., Alvarez, J. A., Aragon, V., and Figueroa, J. V. (2004). Babesia bigemina: sporozoite isolation from Boophilus microplus nymphs and initial immunomolecular characterization. Ann. N.Y. Acad. Sci. 1026, 222–231. doi: 10.1196/annals.1307.034
Nene, V., Kiara, H., Lacasta, A., Pelle, R., Svitek, N., and Steinaa, L. (2016). The biology of Theileria parva and control of East Coast fever - Current status and future trends. Ticks Tick Borne Dis. 7, 549–564. doi: 10.1016/j.ttbdis.2016.02.001
Nene, V., and Morrison, W. I. (2016). Approaches to vaccination against Theileria parva and Theileria annulata. Parasite Immunol. 38, 724–734. doi: 10.1111/pim.12388
Piesman, J., Karakashian, S. J., Lewengrub, S., Rudzinska, M. A., and Spielmank, A. (1986). Development of Babesia microti sporozoites in adult Ixodes dammini. Int. J. Parasitol. 16, 381–385. doi: 10.1016/0020-7519(86)90118-9
Pieszko, M., Weir, W., Goodhead, I., Kinnaird, J., and Shiels, B. (2015). ApiAP2 Factors as Candidate regulators of stochastic commitment to merozoite production in Theileria annulata. PLoS Negl. Trop. Dis. 9:e0003933. doi: 10.1371/journal.pntd.0003933
Potgieter, F. T., and Els, H. J. (1976). Light and electron microscopic observations on the development of small merozoites of Babesia bovis in Boophilus microplus larvae. Onderstepoort. J. Vet. Res. 43, 123–128.
Potgieter, F. T., and Els, H. J. (1977a). Light and electron microscopic observations on the development of Babesia bigemina in larvae, nymphae and non-replete females of Boophilus decoloratus. Onderstepoort. J. Vet. Res. 44, 213–231.
Potgieter, F. T., and Els, H. J. (1977b). The fine structure of intra-erythrocytic stages of Babesia bigemina. Onderstepoort. J. Vet. Res. 44, 157–168.
Potgieter, F. T., Els, H. J., and Vuuren, A. S. (1976). The fine structure of merozoites of Babesia bovis in the gut epithelium of Boophilus microplus. Onderstepoort. J. Vet. Res. 43, 1–9.
Purnell, R. E., and Joyner, L. P. (1968). The development of Theileria parva in the salivary glands of the tick, Rhipicephalus appendiculatus. Parasitology. 58, 725–732. doi: 10.1017/S0031182000029036
Ramsay, J. D., Ueti, M. W., Johnson, W. C., Scoles, G. A., Knowles, D. P., and Mealey, R. H. (2013). Lymphocytes and macrophages are infected by Theileria equi, but T cells and B cells are not required to establish infection in vivo. PLoS ONE 8:e76996. doi: 10.1371/journal.pone.0076996
Rudzinska, M. A. (1976). Ultrastructure of intraerythrocytic Babesia microti with emphasis on the feeding mechanism. J. Protozool. 23, 224–233. doi: 10.1111/j.1550-7408.1976.tb03759.x
Rudzinska, M. A., Lewengrub, S., Spielman, A., and Piesman, J. (1983a). Invasion of Babesia microti into epithelial cells of the tick gut. J. Protozool. 30, 338–346. doi: 10.1111/j.1550-7408.1983.tb02927.x
Rudzinska, M. A., Spielman, A., Lewengrub, S., Piesman, J., and Karakashian, S. (1982). Penetration of the peritrophic membrane of the tick by Babesia microti. Cell Tissue Res. 221, 471–481. doi: 10.1007/BF00215696
Rudzinska, M. A., Spielman, A., Lewengrub, S., Piesman, J., and Karakashian, S. (1984). The sequence of developmental events of Babesia microti in the gut of Ixodes dammini. Protistologica 4, 649–663.
Rudzinska, M. A., Spielman, A., Lewengrub, S., Trager, W., and Piesman, J. (1983b). Sexuality in piroplasms as revealed by electron microscopy in Babesia microti. Proc. Natl. Acad. Sci. U.S.A. 80, 2966–2970. doi: 10.1073/pnas.80.10.2966
Rudzinska, M. A., Spielman, A., Riek, R. F., Lewengrub, S. J., and Piesman, J. (1979). Intraerythrocytic ’gametocytes’ of Babesia microti and their maturation in ticks. Can. J. Zool. 57, 424–434. doi: 10.1139/z79-050
Rudzinska, M. A., and Trager, W. (1962). Intracellular phagotrophy in Babesia rodhaini as revealed by electron microscopy. J. Protozool. 9, 279–288. doi: 10.1111/j.1550-7408.1962.tb02621.x
Rudzinska, M. A., and Trager, W. (1977). Formation of merozoites in intraerythrocytic Babesia microti: an ultrastructural study. Can. J. Zool. 55, 928–938. doi: 10.1139/z77-121
Rudzinska, M. A., Trager, W., Lewengrub, S. J., and Gubert, E. (1976). An electron microscopic study of Babesia microti invading erythrocytes. Cell Tissue Res. 169, 323–334. doi: 10.1007/BF00219605
Sato, M., Kamio, T., Tanaka, S., Taniguchi, T., and Fujisaki, K. (1994). Development of Theileria sergenti schizonts in the lymph node of experimentally infected cattle. J. Vet. Med. Sci. 56, 715–722. doi: 10.1292/jvms.56.715
Schein, E., and Friedhoff, K. T. (1978). Light microscopic studies on the development of Theileria annulata (Dschunkowsky and Luhs, 1904) in Hyalomma anatolicum excavatum (Koch, 1844): the development in haemolymph and salivary glands. Z. Parasitenkd. 56, 287–303. doi: 10.1007/BF00931721
Schein, E., Mehlhorn, H., and Voigt, W. P. (1979). Electron microscopical studies on the development of Babesia canis (Sporozoa) in the salivary glands of the vector tick Dermacentor reticulatus. Acta Trop. 36, 229–241.
Schein, E., Rehbein, G., Voigt, W. P., and Zweygarth, E. (1981). Babesia equi (Laveran 1901) development in horses and in lymphocyte culture. Tropenmed. Parasitology 32, 223–227.
Schein, E., Warnecke, M., and Kirmse, P. (1977). Development of Theileria parva (Theiler, 1904) in the gut of Rhipicephalus appendiculatus (Neumann, 1901). Parasitology 75, 309–316. doi: 10.1017/S0031182000051854
Schnittger, L., Rodriguez, A. E., Florin-Christensen, M., and Morrison, D. A. (2012). Babesia: a world emerging. Infect. Genet. Evol. 12, 1788–1809. doi: 10.1016/j.meegid.2012.07.004
Schreeg, M. E., Marr, H. S., Tarigo, J. L., Cohn, L. A., Bird, D. M., Scholl, E. H., et al. (2016). Mitochondrial genome sequences and structures aid in the resolution of piroplasmida phylogeny. PLoS ONE 11:e0165702. doi: 10.1371/journal.pone.0165702
Shaw, M. K. (1995). Mobilization of intrasporozoite Ca2+ is essential for Theileria parva sporozoite invasion of bovine lymphocytes. Eur. J. Cell Biol. 68, 78–87.
Shaw, M. K. (1996a). Characterization of the parasite-host cell interactions involved in Theileria parva sporozoite invasion of bovine lymphocytes. Parasitology 113, 267–277.
Shaw, M. K. (1996b). Theileria parva sporozoite entry into bovine lymphocytes involves both parasite and host cell signal transduction processes. Exp. Parasitol. 84, 344–354. doi: 10.1006/expr.1996.0123
Shaw, M. K. (1997). The same but different: the biology of Theileria sporozoite entry into bovine cells. Int. J. Parasitol. 27, 457–474. doi: 10.1016/S0020-7519(97)00015-5
Shaw, M. K. (1999). Theileria parva: sporozoite entry into bovine lymphocytes is not dependent on the parasite cytoskeleton. Exp. Parasitol. 92, 24–31. doi: 10.1006/expr.1998.4393
Shaw, M. K. (2003). Cell invasion by Theileria sporozoites. Trends Parasitol. 19, 2–6. doi: 10.1016/S1471-4922(02)00015-6
Shaw, M. K., and Tilney, L. G. (1992). How individual cells develop from a syncytium: merogony in Theileria parva (Apicomplexa). J. Cell. Sci. 101, 109–123.
Shaw, M. K., and Tilney, L. G. (1995). The entry of Theileria parva merozoites into bovine erythrocytes occurs by a process similar to sporozoite invasion of lymphocytes. Parasitology 11, 455–461. doi: 10.1017/S0031182000065951
Shaw, M. K., Tilney, L. G., and McKeever, D. J. (1993). Tick salivary gland extract and interleukin-2 stimulation enhance susceptibility of lymphocytes to infection by Theileria parva sporozoites. Infect. Immun. 61, 1486–1495.
Shaw, M. K., Tilney, L. G., and Musoke, A. J. (1991). The entry of Theileria parva sporozoites into bovine lymphocytes: evidence for MHC class I involvement. J. Cell Biol. 113, 87–101. doi: 10.1083/jcb.113.1.87
Simpson, C. F., Bild, C. E., and Stolkier, H. E. (1963). Electron microscopy of canine and equine Babesia. Am. J. Vet. Res. 24, 408–414.
Simpson, C. F., and Neal, F. C. (1980). Ultrastructure of Babesia equi in ponies treated with imidocarb. Am. J. Vet. Res. 41, 267–271.
Sivakumar, T., Hayashida, K., Sugimoto, C., and Yokoyama, N. (2014). Evolution and genetic diversity of Theileria. Infect. Genet. Evol. 27, 250–263. doi: 10.1016/j.meegid.2014.07.013
Soldati, D., Foth, B. J., and Cowman, A. F. (2004). Molecular and functional aspects of parasite invasion. Trends Parasitol. 20, 567–574. doi: 10.1016/j.pt.2004.09.009
Sun, Y., Moreau, E., Chauvin, A., and Malandrin, L. (2011). The invasion process of bovine erythrocyte by Babesia divergens: knowledge from an in vitro assay. Vet. Res. 42:62. doi: 10.1186/1297-9716-42-62
Susta, L., Torres-Velez, F., Zhang, J., and Brown, C. (2009). An in situ hybridization and immunohistochemical study of cytauxzoonosis in domestic cats. Vet. Pathol. 46, 1197–1204. doi: 10.1354/vp.08-VP-0132-B-FL
Takahashi, K., Kawai, S., Yaehata, K., Kawamoto, S., Hagiwara, K., Kurosawa, T., et al. (1993). Sporogony of Theileria sergenti in the salivary glands of the tick vector Haemaphysalis longicornis. Parasitol. Res. 79, 1–7. doi: 10.1007/BF00931210
Uilenberg, G. (2006). Babesia–a historical overview. Vet. Parasitol. 138, 3–10. doi: 10.1016/j.vetpar.2006.01.035
Vannier, E. G., Diuk-Wasser, M. A., Ben Mamoun, C., and Krause, P. J. (2015). Babesiosis. Infect. Dis. Clin. North Am. 29, 357–370. doi: 10.1016/j.idc.2015.02.008
Votypka, J., Modry, D., Obornik, M., Slapeta, J., and Lukes, J. (2017). “Apicomplexa,” in Handbook of the Protists, eds J. M. Archibald, A. G. B. Simpson, and C. H. Slamovits (Cham: Springer International Publishing AG), 1–58. doi: 10.1007/978-3-319-32669-6_20-1
Ward, P. A., and Jack, R. M. (1981). The entry process of Babesia merozoites into red cells. Am. J. Pathol. 102, 109–113.
Warnecke, M., Schein, E., Voigt, W. P., Uilenberg, G., and Young, A. S. (1980). Development of Theileria mutans (Theiler, 1906) in the gut and the haemolymph of the tick Amblyomma variegatum (Fabricius, 1794). Z. Parasitenkd. 62, 119–125. doi: 10.1007/BF00927858
Weber, G., and Friedhoff, K. (1979). Electron microscopic detection of initial and some subsequent developmental stages of Babesia bigemina in salivary glands of ticks. Z. Parasitenkd. 58, 191–194. doi: 10.1007/BF01951346
Weber, G., and Friedhoff, K. T. (1977). Preliminary observations on the ultrastructure of suppossed sexual stages of Babesia bigemina (Piroplasmea). Z. Parasitenkd. 53, 83–92. doi: 10.1007/BF00383118
Weber, G., and Walter, G. (1980). Babesia microti (Apicomplexa, Piroplasmida) - electron-microscope detection in salivary glands of the tick vector Ixodes ricinus (Ixodoidea, Ixodidae). Z. Parasitenkd. 64, 113–115. doi: 10.1007/BF00927061
Webster, P., Dobbelaere, D. A., and Fawcett, D. W. (1985). The entry of sporozoites of Theileria parva into bovine lymphocytes in vitro. Immunoelectron microscopic observations. Eur. J. Cell Biol. 36, 157–162.
Wise, L. N., Kappmeyer, L. S., Mealey, R. H., and Knowles, D. P. (2013). Review of equine piroplasmosis. J. Vet. Intern. Med. 27, 1334–1346. doi: 10.1111/jvim.12168
Yabsley, M. J., and Shock, B. C. (2013). Natural history of Zoonotic Babesia: role of wildlife reservoirs. Int. J. Parasitol. Parasites Wildl. 2, 18–31. doi: 10.1016/j.ijppaw.2012.11.003
Yano, Y., Saito-Ito, A., Anchalee, D., and Takada, N. (2005). Japanese Babesia microti cytologically detected in salivary glands of naturally infected tick Ixodes ovatus. Microbiol. Immunol. 49, 891–897. doi: 10.1111/j.1348-0421.2005.tb03680.x
Zapf, F., and Schein, E. (1994a). New findings in the development of Babesia (Theileria) equi (Laveran, 1901) in the salivary glands of the vector ticks, Hyalomma species. Parasitol. Res. 80, 543–548. doi: 10.1007/BF00933000
Zapf, F., and Schein, E. (1994b). The development of Babesia (Theileria) equi (Laveran, 1901) in the gut and the haemolymph of the vector ticks, Hyalomma species. Parasitol. Res. 80, 297–302. doi: 10.1007/BF02351869
Zintl, A., Mulcahy, G., Skerrett, H. E., Taylor, S. M., and Gray, J. S. (2003). Babesia divergens, a bovine blood parasite of veterinary and zoonotic importance. Clin. Microbiol. Rev. 16, 622–636. doi: 10.1128/CMR.16.4.622-636.2003
Keywords: piroplasms, Babesia, Theileria, developmental cycle, merogony, gamogony, sporogony
Citation: Jalovecka M, Hajdusek O, Sojka D, Kopacek P and Malandrin L (2018) The Complexity of Piroplasms Life Cycles. Front. Cell. Infect. Microbiol. 8:248. doi: 10.3389/fcimb.2018.00248
Received: 03 May 2018; Accepted: 29 June 2018;
Published: 23 July 2018.
Edited by:
Lilach Sheiner, Wellcome Trust Centre for Molecular Parasitology (WT), University of Glasgow, United KingdomReviewed by:
Julius Lukes, University of South Bohemia, CzechiaMattie Christine Pawlowic, University of Dundee, United Kingdom
Copyright © 2018 Jalovecka, Hajdusek, Sojka, Kopacek and Malandrin. This is an open-access article distributed under the terms of the Creative Commons Attribution License (CC BY). The use, distribution or reproduction in other forums is permitted, provided the original author(s) and the copyright owner(s) are credited and that the original publication in this journal is cited, in accordance with accepted academic practice. No use, distribution or reproduction is permitted which does not comply with these terms.
*Correspondence: Marie Jalovecka, jalovecka@paru.cas.cz