- Key Laboratory of Rubber-Plastics Ministry of Education/Shandong Provincial Key Laboratory of Rubber-plastics, College of Polymer Science and Engineering, Qingdao University of Science and Technology, Qingdao, China
Recent studies indicated that the toxicity of heavy metal ions caused a series of environmental, food, and human health problems. Chemical ionochromic sensors are crucial for detecting these toxicity ions. Incorporating organic ligands into π-conjugated polymers made them receptors for metal ions, resulting in an ionochromism phenomenon, which is promising to develop chemosensors for metal ions. This review highlights the recent advances in π-conjugated polymers with ionochromism to metal ions, which may guide rational structural design and evaluation of chemosensors.
Introduction
π-Conjugated polymers refer to polymers in which double bonds (or triple bonds) and single bonds are alternately arranged, including π-π conjugated system, p-π conjugated system, and σ-π-conjugated system (Moon et al., 2007; Tan et al., 2009). It is a research hotspot in the multidisciplinary research field (Bhupathiraju et al., 2018; Biswas et al., 2020; Lee et al., 2020; Zhao et al., 2020) since Shirakawa and coworkers discovered that polyacetylene (PA) doped with I2 or AsF5 exhibited high conductivity more than 103 S/cm in 1997 (Chiang et al., 1977; Shirakawa et al., 1977). Some parent structures of π-conjugated polymers in the backbone are presented in Figure 1A (Hoeben et al., 2005). By far π-conjugated polymers are the most promising functional polymers in the field of cheap and portable electronic devices such as photovoltaic cells (Brabec et al., 2003; Chen et al., 2019), light-emitting diodes (Tang et al., 2013; Saito et al., 2018), prototype field-effect transistors (Dimitrakopoulos and Malenfant, 2002; Melkonyan et al., 2016; Zhang et al., 2017, 2018; Zhang H. et al., 2020) and coatings (Zeng et al., 2019; Zhang H. C. et al., 2020).
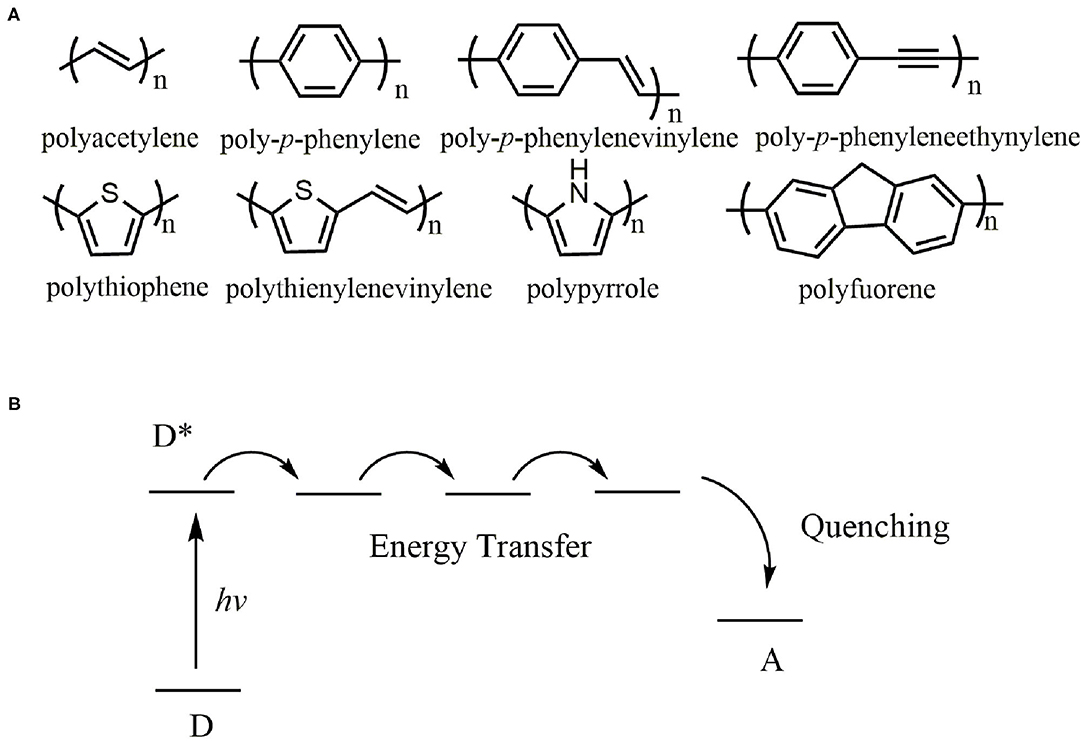
Figure 1. (A) Chemical structures of π-conjugated polymers, (B) Illustration of energy-transfer quenching through a conjugated polymer. *Excited state.
In recent years, researches on the π-conjugated system are booming in the fields of organic optoelectronics and sensors based on chromogenic effect. Chromogenic systems are required to be responsive to external inputs, such as metal ions (ionochromism), (Cheng and Tieke, 2014) electrons (electrochromism), (Thakur et al., 2012), or light (photochromism) (Bisoyi and Li, 2016) that could be used for image production. Among these chromogenic systems, ionochromism is realized through the coordination between metal and ligand, resulting in sensitivity to metal ions, which could be used as metal sensors. Trace metal detection plays a vital role in the environment, the human body, and equipment safety. According to the Environmental Protection Agency (EPA), thirteen heavy metal ions are listed as “priority pollutants” because the toxicity of heavy metal ions caused a series of environmental problems. It is of significance to detect the content of alkali metals ions for human condition monitoring.
This review aims to bring together the areas of metal-ligand coordination and π-conjugated systems. Materials based on metal-ligand coordination that show a polymerlike structure in solid-state refers to “metal-organic frameworks” (MOFs) (Kaneti et al., 2017; Ding et al., 2019), and in solution refers to “metallo-supramolecular polymers” (Winter and Schubert, 2016). MOFs and metallo-supramolecular polymers will not be considered in this review because they have been reviewed a lot in recent years. Therefore, this review focuses on the ionochromic properties of π-conjugated polymers with organic ligand as receptors for metal ions.
Ionochromism
Ionochromic effect based on π-conjugated polymers generates from induced conductivity fluctuations either by destroying the conjugation of polymers (conformational effect) or by lowering charge carrier mobility (electrostatic effect). The conductivity of π-conjugated polymers is highly sensitive to the nature and regiospecificity of the side chains, resulting in sensory signal amplification through energy-transfer along polymer chains. Figure 1B illustrated the energy-transfer process, in which upon the excitations, the energy may migrate along the polymer backbone due to the conjugation. As a result, the π-conjugated polymers act as a molecular wire, and the conjugated system generates a response more significant than that afforded by a small interaction in an analogous small mono-receptor system (Zhou and Swager, 1995).
Organic ligand containing conjugated polymers are receptors for metal ions. The coordinating interaction between metal ions and ligand causes electrostatic or conformational changes, resulting in an ionochromic effect. Ionochromic performance in π-conjugated systems is expected to find use in portable optical devices for the detection of metal ions and some organic cations. The electron effect and steric hindrance effect of the ligand are selective for the type of metal. The ionochromic phenomenon in the π-conjugated polymer will be introduced according to the type of ligands.
Crown Ether
Crown ethers were discovered by the Nobel Prize winner Charles Pedersen (Pedersen, 1967) more than 50 years ago. Recent progress in the design and applications of chemosensors based on crown ethers for small molecules has been reviewed (Li et al., 2017). In contrast to small molecules, π-conjugated polymers have enormous advantages for sensing applications in terms of energy migration and facile exciton transport, which improve the electronic communication between receptors. Additionally, polymers could be processed into films exhibiting semi-permeability to ions. Herein, we focus attention on the design of crown ethers containing π-conjugated polymers and their applications in chemosensors.
Ionochromism was initially reported in the 1990s. Upon coordination with alkali-metal ions (K+, Na+, and Li+), polythiophenes with crown ether side chains (1, Figure 2) (Bäuerle and Scheib, 1993; Marsella and Swager, 1993) underwent interesting sensory effect because of dramatic conformational changes of polymer chains. Casanovas et al. (Casanovas et al., 2009) studied the affinity of crown ether functionalized polythiophenes for Na+, K+, and Li+ by quantum mechanical calculations. The results showed that although the association of Li+ to the polythiophenes derivatives is entropically unfavored, the binding energies increased in the order of K+ < Na+ < Li+. The authors explain that the alkali ions with small dimensions underwent large fluctuations when the dimensions of the cavities changed, leading to an increase in thermal energy.
In addition to polythiophene, chemosensors based on other conjugated systems containing benzocrown or azacrown ethers were also developed. A multiple signal responsive chemosensor was realized by a poly(phenylene-ethynylene) polymer with pendent dibenzocrown groups (2), which was responsive to multi-excitation (K+, Cl−, pH, or temperature change) (Ji et al., 2013). Additionally, exposure of a 2-based film to ammonia increased fluorescence, making it a good candidate for gas sensing. Morgado et al. studied the ionochromic properties of poly(p-phenylenevinylene) (PPV) with benzocrown ether (Morgado et al., 2000). Single-layer devices with Al cathodes showed higher electroluminescence efficiencies than those with Ca cathodes due to the existence of aggregates, induced by the crown ether side groups.
Due to the easy chemical access to modification, the functionalization of polypyrrole is widely studied by incorporating various active groups on the nitrogen atom. However, the modification of polypyrrole generated a loss of conjugation, resulting in extremely low conductivity for poly(N-substituted pyrroles), of the order of 10−4 S/cm or less (Eaves et al., 1985; Bettelheim et al., 1987). Youssoufi et al. found that the equivalent 3-substituted pyrroles (3) gave rise to highly conducting polymer films, and they developed azacrown ether substituted polypyrroles with selective cation binding on voltammetric cycling in organic media (Youssoufi et al., 1993). In contrast to benzocrown ethers, a drawback of the azacrown ethers is that it exhibits low thermodynamic stability upon alkali and alkaline-earth metal ions (Ushakov et al., 2008). The main reason is that the planar structure of the junction section of the azacrown ether and benzene moieties. The increasing electron-withdrawing ability of the moiety conjugated to the crown ether is helpful for improving the thermodynamic stability. However, this method does not always lead to the expected enhancement of optical signal induced by metal ions but may conversely attenuate the signal (Izatt et al., 1996; Ushakov et al., 1997). Gromov et al. (Gromov et al., 2013) synthesized 4-pyridine-, 2-benzothiazole-, and 2-, and 4-quinoline-based styryl dyes with an n-methylbenzoazacrown ether as a ligand. Electron spectroscopy studies showed that these compounds had a high sensibility for alkali metal and alkaline earth metal cations. In terms of electrochromism and cation binding capacity, they proved to be far superior to those based on phenylazacrown ether. After complexing with Ba2+, the fluorescence enhancement factor reached 61. The discovery of high levels of macrocyclic pre-organization is one of the factors that determine the high cation binding capacity of sensor molecules based on N-methylbenzoaza-crown ether.
Alkali-metal ions, especially K+ and Na+, are the messengers of living cells, controlling a series of physiological processes through the action of ion channels. Crown ether containing π-conjugated polymers are highly sensitive to alkali-metal ions and could be designed to medical detectors. Nevertheless, most researches focused on the ionochromism of sensors operating in organic solvents rather than in aqueous solutions (Xiang et al., 2014), which is impractical for applications. Additionally, most reported ion-selective films require long incubation times to generate a detectable response, precluding their practical use (Giovannitti et al., 2016). Single-component π-conjugated polymers (4 and 5) were synthesized that respond selectively and rapidly to varying concentrations of Na+ and K+ in aqueous media, respectively (Wustoni et al., 2019). Using a miniaturized organic electrochemical transistor chip, variations in the concentration of these two metal ions in a blood serum sample could be measured in real-time. The devices based on these crown ether containing polymers are valuable for analyzing cellular machinery and detecting human body conditions that result in electrolyte imbalance.
Pyridine
The studies on crown ether substituted π-conjugated polymers have clearly demonstrated the ionochromism in alkali chemosensors. It is well-known that oligopyridine, such as bipyridine (bpy), terpyridine (tpy), and its derivatives exhibit super abilities to coordinate a large number of metal ions. If selecting oligopyridine as ligands for π-conjugated polymers, the range of metal ion sensors could be extended from alkali metal ion selective systems to transition metal ion selective systems. Additionally, pyridine and its derivatives not only have the electron-accepting ability to coordinate with metal ions, but also are reactive for metal complex-forming reactions, such as N-oxidation, N-protonation, and quaternization with RX, which can adjust their optical and electrical properties (Yamamoto et al., 1994).
In 1997, Wang et al. reported a transition metal-induced ionochromic polymer with bpy in the backbone (6) (Wang and Wasielewski, 1997). According to theoretical calculations, there is a 20° dihedral angle between two adjacent pyridyl rings in bpy when it is in its transoid-like conformation (Cumper et al., 1962). As a result, bpy-based polymers are pseudoconjugated. With the addition of metal ions, the chelating effect of bpy ligand with the metal ions forces the pseudoconjugated conformation into a planar one, and thus makes the polymers fully conjugated, leading to the redshift in absorption spectra. Besides, incorporating bpy as a ligand directly into the backbone results in a more sensitive response with the addition of metal ions. Different linkers between bipyridine and conjugated polymer in the backbones cause differences in flexibility and rigidity of the resulting polymers. Bin Liu et al. studied the effect of linkages, including C-C single, vinylene, and ethynylene bonds, on the electronic properties and response sensitivities to metal ions (Liu et al., 2001). During the chelation with metal ions, the C-C single bond linkage provided better flexibility to the coplanarity of the pyridine unit. Therefore, C-C single bond possessed the highest sensitivity, and it was followed by vinylene bond, while ethynylene bond exhibited the lowest sensitivity. A conjugated polymer containing 2,6-substituted pyridine derivative (7) was synthesized for Pb2+ sensing (Liu et al., 2011). With the addition of Pb2+, the color changed from yellow-green to brown, and this can be easily observed by the naked eye. The detection limit of the polymer is less than 1 ppm, while the threshold of Pb2+ in drugs is 5–10 ppm. Therefore, 7 could be adopted to design an excellent sensor for Pb2+ detection.
Increasing the association constant of a molecular recognition event could improve the sensitivity of a sensor. Terpyridine (tpy) ligand possesses an excellent ability to coordinate various of metal ions with higher sensitivity than bipyridine. Zhang et al. prepared poly[p-(phenyleneethynylene)-alt-(thienyleneethynylene)] (PPETE) with bpy (8) and tpy (9) as receptors, respectively (Zhang et al., 2002). With the addition of Ni2+, 9 was quenched to 10.9% of its original emission intensity, while 8 was only quenched to 38.2%, illustrating that tpy was more sensitive to Ni2+ ion. Rabindranath et al. reported tpy substituted polyiminofluorenes (10) (Rabindranath et al., 2009). Fe2+, Co2+, Ni2+, Cu2+, Pd2+, Fe3+, Gd3+, and Zr4+ led to complete quenching of the green emission for 10, while Zn2+, Cd2+, and Eu3+ caused a weak red emission, which was redshifted by 10-30 nm compared with pure 10. Additionally, a biscomplex formed upon the addition of Zn2+, leading to red luminescent precipitation. This effect can be used for the detection of Zn2+.
Although tpy exhibits high coordinating ability with a large number of metal ions, the preparation of tpy ligands is expensive and very time-consuming. In contrast to bpy, 2,6-bis(10-methylbenzimidazolyl)pyridine (bip) ligands can be more easily synthesized on a large scale (Beck et al., 2005). Kalie Cheng et al. reported a polyimide fluorene (11) with bip ligand (Cheng and Tieke, 2014) and studied the optical properties with Zn2+ and Cu2+. Due to charge transfer from ion-specific metal to ligand, the 11/Zn2+ film is orange, while the 11/Cu2+ film is purple. Due to the oxidation of the polymer backbone, the 11/Zn2+ and 11/Cu2+ films will turn blue if anodically oxidized to 750 mV vs. FOC., and the color change is reversible. The conjugated polymer with bip ligand exhibited high contrast and short switching times in color change upon 12 dipping cycles. However, the long-term stability of bip with metal ions is lower than that of tpy based systems.
Some biomacromolecules such as DNA, RNA, and proteins are easy to be inhibited by Pd2+ in vivo and in vitro (Kielhorn et al., 2002). Additionally, Pd2+ is able to elicit a series of cytotoxic effects, resulting in severe primary skin and eye irritations. It is essential to investigate a sensor for highly selective and sensitive detection of Pd2+. Xiang et al. reported three conjugated polymers (12–14) via Sonogashira reaction (Xiang et al., 2014). The conjugated backbones of 13 and 14 are twisted, which were proved to be selective for Ni+. In contrast to 13 and 14, 12 exhibited high selectivity for Ag+ because of its linear conjugated backbone. Theoretically, the same functional group should have the same metal ion recognition capability. According to the ionochromic effect of 12–14, changes of linkage site for recognition groups resulted in different metal ion selectivity. Cyclic voltammetry measurement for 12–14 was carried out to analyze the cation selectivity by LUMO and HOMO energy levels. The LUMO levels of 12 are slightly lower than that of 13 and 14, indicating that their electron affinity is in the order of 12 > 14 ≈ 13. Additionally, the HOMO levels of 13 and 14 are slightly raised relative to 12, illustrating that the energy barrier of hole injection from the anode is in the order of 13 = 14<12. As a result, both electron and hole affinities of 12 are improved, resulting in enhanced carrier injection and transport. Moreover, smaller coordination cavity in 13 and 14 fits well with the size of Ni+ because of their twisted conjugated backbones and smaller radius of Ni+. This work provided guidelines to tune the structure of conjugated polymers for the design and preparation of the selective metal ion sensors.
Despite the successful development of chemosensors in conjugated polymers, most of the examples are in the solution state, and seldom chemosensors in neat π-conjugated polymer films have been reported. This is because of strong interpolymer π-π interactions resulting in the self-quenching of luminescence in such a condensed solid-state phase (Sahoo et al., 2014). It is a non-negligible challenge to control such random and strong interactions in the solid-state. Hosomi et al. reported π-conjugated polymer with bipyridine moieties as ligand and permethylated α-cyclodextrin (PM α-CD) as the main chain (15). The PM α-CD suppresses the interactions between π-conjugated and enabled the polymers to show efficient emission even in the solid-state (Hosomi et al., 2016). Additionally, the metal-ion recognition ability of 15 is maintained in the solid-state, leading to reversible changes in the luminescent color in response to cations. The prepared π-polymer is expected to be applicable for recyclable luminescent sensors to detect different metal ions.
1,10-Phenanthroline
1,10-Phenanthroline (phen) is an electron-poor, rigid planar, hydrophobic, and heteroaromatic ligand that has played an important role in the development of coordination chemistry (Cockrell et al., 2008; Bencini and Lippolis, 2010; Iqbal et al., 2016). Phen is a bidentate ligand for transition metal ions whose nitrogen atoms are beautifully placed to act cooperatively in cation binding. In contrast to the parent bpy and tpy systems, phen is characterized by two inwardly pointing nitrogen donor atoms, which is held juxtaposed. As a result, phen is pre-organized for strong and entropically favored metal binding. A luminescent phen-containing π-conjugated copolymer (16) responsive with Zn2+, Ir3+, and Eu3+ was reported (Yasuda et al., 2003). The λmax of 16 is shifted from 385 to 404 nm on the addition of NiCl2. Photoluminescence intensity of 1 steeply decreases in the presence of Ni2+ because of the chelating effect of the phen unit to Ni2+. Other metal ions also caused similar shifts of λmax. Mainly, Li+, Mg2+, Al3+, Zn2+, Ag+, and La3+ caused a redshift of λmax to a smaller degree of about 10 ± 35 nm; Fe3+, Co2+, Ni2+, Cu2+, and Pd2+ gave rise to a larger redshift by about 20 ± 50 nm and complete quenching of photoluminescence. This quenching phenomenon is related to an energy transfer from the π-conjugated polymer to the metal complexes. Yasuda group further synthesized a copolymer composed of alternating phen/9,9-dioctylfluorene (17) (Yasuda and Yamamoto, 2003). The color of emitted light from the polymer complex could be tuned from blue to red by transition metal ions (Co2+, Ni2+, Cu2+, and Pd2+) upon absorption spectra. Additionally, Satapathy et al. reported conjugated polymers containing phenanthroline that show remarkable sensing capabilities toward Fe2+ (Satapathy et al., 2012).
Calixarene
Calixarenes have unique hole structures, which can be functionalized to recognize metal ions. Moreover, the hydrophobic cavity of the calixarene scaffold can accommodate various gases and organic molecules (Rudkevich, 2007). With the addition of metal ions or small molecules, calixarenes undergo dramatic geometric changes, including phenol ring flips between cone, partial cone, and 1,3-alternate conformational isomers (Gutsche, 1998). The small molecular of calixarene-based sensors for recognition of transition metal cations have been recently reviewed (Kumar et al., 2019), and here only list some calixarene-based conjugated polymer sensors. Calixarene-functionalized polymers (18 and 19) were first reported in the 1990s (Marsella et al., 1995). Binding constant measurements of the calixarene-bithiophene generated a Ka (7.6 × 107) for Na+, which is approximately100 times stronger than K+ and 40 times stronger than Li+. A stronger binding constant means higher sensitivity. Ion recognition behavior of 18 and 19 toward Li+, Na+, and K+ was analyzed by UV-vis absorption and fluorescence emission spectroscopy. The resulting polymers exhibit good selectivity toward Na+, with a 24 nm blue shift for 66 and 32 nm red shift for 67.
Other calixarene-based receptors in π-conjugated polymers were reported (Wosnick and Swager, 2004; Costa et al., 2008), in which the calixarene groups were mainly as pendant groups. The direct attachment of the calixarene unit (at the upper rim) to a conjugated polymer (20) has also been reported (Yu et al., 2003). The conical configuration of calixarene makes the polymer chain segment a zigzag orientation. The segmental structure in 20 imposes great localization of the carriers, and the rapid self-exchange between discrete units causes the conductivity of such a segmented system. Protonation promoted the electron exchange resulted in high conductivity for 20. Hence, electroactive calixarene polymer that requires protonation to be highly conductive was prepared, which is useful for the design of actuating materials. A fluorescent polymer (21) in which calixarene scaffolds are the part of uninterrupted linear polymeric backbone was first reported (Molad et al., 2012). Short conjugated fragments combined with the nonlinear geometry gave rise to rather moderate sensitivity with selected stimuli. The coordination of the calixarenes in the π-conjugated polymers allows for the recognition of small molecules, such as NO.
Imidazole
Imidazole-based ligands are widely used due to their reversible fluorescence. This reversibility is realized by protonation/deprotonation upon an acid/base or metallation/demetallation with metal ions/suitable counter ligands (Jiang et al., 2010). As an important functional conjugated polymer material, polydiacetylene (PDA) has received more and more attention since the first report in 1969 (Wegner, 1969). PDA has significant color conversion and fluorescence enhancement under various environmental stimuli, including heat (Takeuchi et al., 2017), organic solvent (Yoon et al., 2007), bioanalyte (Zhou et al., 2013), ion (Wang et al., 2016), and so on. In response to different stimuli, PDA can be changed to different colors, such as purple, yellow, orange, or red, of which the transition from blue to red is the most common type. Due to their spontaneous color change and fluorescence emission development under stimulation, many PDA liposomes with specific receptor groups have been designed and widely used to detect metal ions such as Hg2+ and Cu2+ (Lee et al., 2009; Xu et al., 2011).
An imidazole-functionalized disubstituted polyacetylene (22) was prepared (Zeng et al., 2008). 22 was not sensitive to alkali and alkaline earth metal ions, and transition metals Cd2+, Mn2+, Ag+, and Zn2+, because of the poor coordination ability of the imidazole receptor with these ions. Nevertheless, Pb2+, Al3+, and Cr3+ could quench the fluorescence of 22 not completely, while Cu2+, Co2+, Fe2+, Fe3+, and Ni2+ could quench its fluorescence more efficiently. Particularly, Cu2+ quenched the fluorescence entirely at a very low concentration (1.48 ppm). Satapathy et al. reported imidazole-based polymers (23-25) that present significant ion recognition ability toward Fe2+ in semi-aqueous solutions (Satapathy et al., 2012). The fluorescence lifetime of polymer 25 (11.4-fold) decreased larger than that of 23 (4.6-fold) and 24 (6.2-fold) further, illustrating that 25 showed superior sensing capability by virtue of its stronger molecular wire effect. The fluorescence of these three polymers recovered by adding phenanthroline or Na2-EDTA. Additionally, the selectivity of 23-25 for Fe2+ interaction was not interfered by other competing metal ions.
Conclusions and Outlook
π-Conjugated polymers represent useful chemical platforms for the design of chemosensors for metal ions. In this review, we have summarized the types and characteristics of functional groups that chelating with different metal ions as well as the ionochromic effect of the π-conjugated polymers based on these functional groups. In the past few decades, significant progress has been made in the development of novel chemosensors in environmental protection, food and drug testing, and human health monitoring. Although there has been a lot of research on these materials, preparing chemical sensors with high sensitivity, long-term stability, and selectivity is still a critical challenge. The chemical and physical relationship between ligand and metal coordination also needs to be further studied to improve the theoretical guidance for the preparation of metal sensors.
Author Contributions
JL and JH: conceptualization and design. LH and ZW: acquisition of data. JG: software. JL and LH: analysis of data. JL: drafting of article. JL, LH, JG, JH, and ZW: final approval of manuscript. All authors: contributed to the article and approved the submitted version.
Conflict of Interest
The authors declare that the research was conducted in the absence of any commercial or financial relationships that could be construed as a potential conflict of interest.
References
Bäuerle, P., and Scheib, S. (1993). Molecular recognition of alkali-ions by crown-ether-functionalized poly(alkylthiophenes). Adv. Mater. 5, 848–853. doi: 10.1002/adma.19930051113
Beck, J. B., Ineman, J. M., and Rowan, S. J. (2005). Metal/ligand-induced formation of metallo-supramolecular polymers. Macromolecules 38, 5060–5068. doi: 10.1021/ma050369e
Bencini, A., and Lippolis, V. (2010). 1,10-phenanthroline: a versatile building block for the construction of ligands for various purposes. Coord. Chem. Rev. 254, 2096–2180. doi: 10.1016/j.ccr.2010.04.008
Bettelheim, A., White, B. A., Raybuck, S. A., and Murray, R. W. (1987). Cheminform abstract: electrochemical polymerization of amino-, pyrrole-, and hydroxy-substituted tetraphenylporphyrins. ChemInform 18:18. doi: 10.1002/chin.198733064
Bhupathiraju, N., Sayeedi, M., Rizvi, W., Singh, S., Batteas, J. D., and Drain, C. M. (2018). Practical, high-yield synthesis of thiol-terminated diacetylenes for formation of conductive monolayers. Tetrahedron Lett. 59, 3629–3631. doi: 10.1016/j.tetlet.2018.08.057
Bisoyi, H. K., and Li, Q. (2016). Light -driven liquid crystalline materials: from photo -induced phase transitions and property modulations to applications. Chem. Rev. 116, 15089–15166. doi: 10.1021/acs.chemrev.6b00415
Biswas, S., Kim, K., Nam, I. W., Choi, M., Bae, J. H., and Kim, H. (2020). Highly conductive and thermally stable nanoparticle-conjugated polymer compounds through environmentally friendly in situ synthesis. Prog. Org. Coat. 142:105606. doi: 10.1016/j.porgcoat.2020.105606
Brabec, C. J., Dyakonov, V., Parisi, J., and Sariciftci, N. S. (2003). Organic Photovoltaics: Concepts and Realization. London: Springer Series in Materials Science. doi: 10.1007/978-3-662-05187-0
Casanovas, J., Preat, J., Zanuy, D., and Aleman, C. (2009). Sensing abilities of crown ether functionalized polythiophenes. Chemistry 15, 4676–4684. doi: 10.1002/chem.200802006
Chen, H. J., Lyu, G. Y., Yue, Y. F., Wang, T. W., Li, D. P., Shi, H., et al. (2019). Improving the photovoltaic performance by employing alkyl chains perpendicular to the pi-conjugated plane of an organic dye in dye-sensitized solar cells. J. Mater. Chem. C 7, 7249–7258. doi: 10.1039/c9tc01520e
Cheng, K. L., and Tieke, B. (2014). Polyiminofluorene with conjugated benzimidazolylpyridine substituent groups: Optical properties, ionochromism and coordinative self-assembly into electrochromic films. RSC Adv. 4, 25079–25088. doi: 10.1039/c4ra03969f
Chiang, C. K., Ficher, C. R., Park, Y. W., Heeger, A. J., Shirakawa, H., Louis, E. J., et al. (1977). Electrical conductivity in doped polyacetylene. Phys. Rev. Lett. 39:1098. doi: 10.1103/PhysRevLett.40.1472
Cockrell, G. M., Zhang, G., VanDerveer, D. G., Thummel, R. P., and Hancock, R. D. (2008). Enhanced metal ion selectivity of 2,9-di-(pyrid-2-yl)-1,10-phenanthroline and its use as a fluorescent sensor for cadmium(II). J. Am. Chem. Soc. 130, 1420–1430. doi: 10.1021/ja077141m
Costa, A. I., Ferreira, L. F., and Prata, J. V. (2008). Novel fluorescent (p-phenylene ethynylene)-calix [4] arene-based polymer: design, synthesis, and properties. J. Polym. Sci. Part A Polym. Chem. 46, 6477–6488. doi: 10.1002/pola.22957
Cumper, C. W. N., Ginman, R. F. A., and Vogel, A. I. (1962). 226. Physical properties and chemical constitution. Part xxxv. The electric dipole moments of some phenanthrolines and bipyridyls. J. Chem. Soc. 4:1188–1192. doi: 10.1039/jr9620001188
Dimitrakopoulos, C. D., and Malenfant, P. R. L. (2002). Organic thin film transistors for large area electronics. Adv. Mater. 14:99–117. doi: 10.1002/1521-4095(20020116)14:2<99::AID-ADMA99>3.0.CO;2-9
Ding, M. L., Flaig, R. W., Jiang, H. L., and Yaghi, O. M. (2019). Carbon capture and conversion using metal-organic frameworks and mof-based materials. Chem. Soc. Rev. 48, 2783–2828. doi: 10.1039/c8cs00829a
Eaves, J. G., Munro, H. S., and Parker, D. (1985). Electroactive thin films from the anodic electropolymerisation of iron(ii) and ruthenium(ii) tris(n-bipyridylpyrrole) complexes. J. Chem. Soc. Chem. Commun. 11, 684–685. doi: 10.1039/C39850000684
Giovannitti, A., Nielsen, C. B., Rivnay, J., Kirkus, M., Harkin, D. J., White, A. J. P., et al. (2016). Sodium and potassium ion selective conjugated polymers for optical ion detection in solution and solid state. Adv. Funct. Mater. 26, 514–523. doi: 10.1002/adfm.201503791
Gromov, S. P., Dmitrieva, S. N., Vedernikov, A. I., Kurchavov, N. A., Kuz'mina, L. G., Sazonov, S. K., et al. (2013). Synthesis, structure, and characterization of chromo(fluoro)ionophores with cation-triggered emission based on n-methylaza-crown-ether styryl dyes. J. Org. Chem. 78, 9834–9847. doi: 10.1021/jo401555a
Hoeben, F. J., Jonkheijm, P., Meijer, E. W., and Schenning, A. P. (2005). About supramolecular assemblies of pi-conjugated systems. Chem. Rev. 105, 1491–1546. doi: 10.1021/cr030070z
Hosomi, T., Masai, H., Fujihara, T., Tsuji, Y., and Terao, J. (2016). A typical metal-ion-responsive color-tunable emitting insulated π-conjugated polymer film. Angew. Chem. Int. Ed. 55, 13427–13431. doi: 10.1002/anie.201603160
Iqbal, A., Tian, Y. J., Wang, X. D., Gong, D. Y., Guo, Y. L., Iqbal, K., et al. (2016). Carbon dots prepared by solid state method via citric acid and 1,10-phenanthroline for selective and sensing detection of fe2+ and fe3+. Sensor. Actuat. B Chem. 237, 408–415. doi: 10.1016/j.snb.2016.06.126
Izatt, R. M., Pawlak, K., and Bradshaw, J. S. (1996). Thermodynamic and kinetic data for macrocycle interaction with cations, anions, and neutral molecules. Cheminform 27:322. doi: 10.1002/chin.199611336
Ji, X. F., Yao, Y., Li, J. Y., Yan, X. Z., and Huang, F. H. (2013). A supramolecular cross-linked conjugated polymer network for multiple fluorescent sensing. J. Am. Chem. Soc. 135, 74–77. doi: 10.1021/ja3108559
Jiang, J., Xiao, X., Ping, Z., and He, T. (2010). Colorimetric naked-eye recognizable anion sensors synthesized via raft polymerization. J. Polym. Sci. A Polym. Chem. 48, 1551–1556. doi: 10.1002/pola.23922
Kaneti, Y. V., Tang, J., Salunkhe, R. R., Jiang, X. C., Yu, A. B., Wu, K. C. W., et al. (2017). Nanoarchitectured design of porous materials and nanocomposites from metal-organic frameworks. Adv. Mater. 29:1604898. doi: 10.1002/adma.201604898
Kielhorn, J., Melber, C., Keller, D., and Mangelsdorf, I. (2002). Palladium – a review of exposure and effects to human health. Int. J. Hyg. Environ. Health 205, 417–432. doi: 10.1078/1438-4639-00180
Kumar, R., Sharma, A., Singh, H., Suating, P., Kim, H. S., Sunwoo, K., et al. (2019). Revisiting fluorescent calixarenes: from molecular sensors to smart materials. Chem. Rev. 119, 9657–9721. doi: 10.1021/acs.chemrev.8b00605
Lee, D. C., Sellers, D. L., Liu, F., Boydston, A. J., and Pun, S. H. (2020). Synthesis and characterization of anionic poly(cyclopentadienylene vinylene) and its use in conductive hydrogels. Angew. Chem. Int. Ed. Engl. 132, 13532–13538. doi: 10.1002/anie.202004098
Lee, J., Jun, H., and Kim, J. (2009). Polydiacetylene-liposome microarrays for selective and sensitive mercury(II) detection. Adv. Mater. 21:3674–3677. doi: 10.1002/adma.200900639
Li, J., Yim, D., Jang, W. D., and Yoon, J. (2017). Recent progress in the design and applications of fluorescence probes containing crown ethers. Chem. Soc. Rev. 46, 2437–2458. doi: 10.1039/c6cs00619a
Liu, B., Dai, H. G., Bao, Y. Y., Du, F. F., Tian, J., and Bai, R. K. (2011). 2,6-substituted pyridine derivative-containing conjugated polymers: synthesis, photoluminescence and ion-sensing properties. Polym. Chem. 2, 1699–1705. doi: 10.1039/c1py00149c
Liu, B., Yu, W.-L., Pei, J., Liu, S.-Y., Lai, Y.-H., and Huang, W. (2001). Design and synthesis of bipyridyl-containing conjugated polymers: effects of polymer rigidity on metal ion sensing. Macromolecules. 34, 7932–7940. doi: 10.1021/ma0106651
Marsella, M. J., Newland, R. J., Carroll, P. J., and Swager, T. M. (1995). Ionoresistivity as a highly sensitive sensory probe: investigations of polythiophenes functionalized with calix[4]arene-based ion receptors. J. Am. Chem. Soc. 117, 9842–9848. doi: 10.1021/ja00144a009
Marsella, M. J., and Swager, T. M. (1993). Designing conducting polymer-based sensors - selective ionochromic response in crown-ether containing polythiophenes. J. Am. Chem. Soc. 115, 12214–12215. doi: 10.1021/ja00078a090
Melkonyan, F. S., Zhao, W., Drees, M., Eastham, N. D., Leonardi, M. J., Butler, M. R., et al. (2016). Bithiophenesulfonamide building block for pi-conjugated donor-acceptor semiconductors. J. Am. Chem. Soc. 138, 6944–6947. doi: 10.1021/jacs.6b03498
Molad, A., Goldberg, I., and Vigalok, A. (2012). Tubular conjugated polymer for chemosensory applications. J. Am. Chem. Soc. 134, 7290–7292. doi: 10.1021/ja301918f
Moon, J. H., McDaniel, W., MacLean, P., and Hancock, L. E. (2007). Live-cell-permeable poly (p-phenylene ethynylene). Angew. Chem. Int. Ed. 46, 8223–8225. doi: 10.1002/anie.200701991
Morgado, J., Cacialli, F., Friend, R. H., Chuah, B. S., Moratti, S. C., and Holmes, A. B. (2000). Luminescence properties of ppv-based copolymers with crown ether substituents. Synth. Met. 111–112, 449–452. doi: 10.1016/S0379-6779(99)00397-5
Pedersen, C. J. (1967). Cyclic polyethers and their complexes with metal salts. J. Am. Chem. Soc. 89, 7017–7036. doi: 10.1021/ja00986a052
Rabindranath, A. R., Maier, A., Schafer, M., and Tieke, B. (2009). Luminescent and ionochromic polyiminofluorene with conjugated terpyridine substituent groups. Macromol. Chem. Phys. 210, 659–668. doi: 10.1002/macp.200800542
Rudkevich, D. M. (2007). Progress in supramolecular chemistry of gases. Eur. J. Org. Chem. 2007, 3255–3270. doi: 10.1002/ejoc.200700165
Sahoo, D., Sugiyasu, K., Tian, Y., Takeuchi, M., and Scheblykin, I. G. (2014). Effect of conjugated backbone protection on intrinsic and light-induced fluorescence quenching in polythiophenes. Chem. Mater. 26, 4867–4875. doi: 10.1021/cm5021959
Saito, H., Kuwabara, J., Yasuda, T., and Kanbara, T. (2018). Synthesis of polyfluoro arylene-based poly(arylenevinylene)s via pd-catalyzed dehydrogenative direct alkenylation. Macromol. Rapid Commun. 39:1800414. doi: 10.1002/marc.201800414
Satapathy, R., Padhy, H., Wu, Y. H., and Lin, H. C. (2012). Synthesis and characterization of reversible chemosensory polymers: modulation of sensitivity through the attachment of novel imidazole pendants. Chem. Eur. J. 18, 16061–16072. doi: 10.1002/chem.201201437
Shirakawa, H., Louis, E. J., MacDiarmid, A. G., Chiang, C. K., and Heeger, A. H. (1977). Synthesis of electrically conducting organic polymers: halogen derivatives of polyacetylene, (ch)x. J. Chem. Soc. Chem. Commum. 1, 578–580. doi: 10.1039/C39770000578
Takeuchi, M., Imai, H., and Oaki, Y. (2017). Effects of the intercalation rate on the layered crystal structures and stimuli-responsive color-change properties of polydiacetylene. J. Mater. Chem. C 5, 8250–8255. doi: 10.1039/c7tc02218b
Tan, H., Liu, N. S., He, B. P., Wong, S. Y., Chen, Z. K., Li, X., et al. (2009). Facile synthesis of hybrid silica nanocapsules by interfacial templating condensation and their application in fluorescence imaging. Chem. Commun. 41, 6240–6242. doi: 10.1039/b914366a
Tang, C., Liu, X. D., Liu, F., Wang, X. L., Xu, H., and Huang, W. (2013). Recent progress in polymer white light-emitting materials and devices. Macromol. Chem. Phys. 214, 314–342. doi: 10.1002/macp.201200305
Thakur, V. K., Ding, G. Q., Ma, J., Lee, P. S., and Lu, X. H. (2012). Hybrid materials and polymer electrolytes for electrochromic device applications. Adv. Mater. 24, 4071–4096. doi: 10.1002/adma.201200213
Ushakov, E. N., Alfimov, M. V., and Gromov, S. P. (2008). Design principles for optical molecular sensors and photocontrolled receptors based on crown ethers. Russ. Chem. Rev. 77, 39–58. doi: 10.1070/RC2008v077n01ABEH003757
Ushakov, E. N., Gromov, S. P., Fedorova, O. A., and Alfimov, M. V. (1997). Crown-containing styryl dyes. 19. complexation and cation-induced aggregation of chromogenic aza-15-crown-5-ethers. Russ. Chem. Bull. 46, 463–471. doi: 10.1007/BF02495396
Wang, B., and Wasielewski, M. R. (1997). Design and synthesis of metal ion-recognition-induced conjugated polymers: an approach to metal ion sensory materials. J. Am. Chem. Soc. 119, 12–21. doi: 10.1021/ja962229d
Wang, D. E., Zhao, L., Yuan, M. S., Chen, S. W., Li, T. B., and Wang, J. Y. (2016). Fabrication of polydiacetylene liposome chemosensor with enhanced fluorescent self-amplification and its application for selective detection of cationic surfactants. ACS Appl. Mater. Interfaces. 8, 28231–28240. doi: 10.1021/acsami.6b10794
Wegner, G. (1969). Topochemische reaktionen von monomeren mit konjugierten dreifachbindungen / tochemical reactions of monomers with conjugated triple bonds. Z. Naturforsch. B. 24, 824–832. doi: 10.1515/znb-1969-0708
Winter, A., and Schubert, U. S. (2016). Synthesis and characterization of metallo-supramolecular polymers. Chem. Soc. Rev. 45, 5311–5357. doi: 10.1039/c6cs00182c
Wosnick, J. H., and Swager, T. M. (2004). Enhanced fluorescence quenching in receptor-containing conjugated polymers: a calix[4]arene-containing poly(phenylene ethynylene). Chem Commun. 23, 2744–2745. doi: 10.1039/b411489b
Wustoni, S., Combe, C., Ohayon, D., Akhtar, M. H., McCulloch, I., and Inal, S. (2019). Membrane-free detection of metal cations with an organic electrochemical transistor. Adv. Funct. Mater. 29:1904403. doi: 10.1002/adfm.201904403
Xiang, G., Wang, L. Y., Cui, W., An, X. N., Zhou, L. X., Li, L., et al. (2014). 2-pyridine-1h-benzo d imidazole based conjugated polymers: a selective fluorescent chemosensor for ni2+ or ag+ depending on the molecular linkage sites. Sensor. Actuat. B Chem. 196, 495–503. doi: 10.1016/j.snb.2014.02.047
Xu, Q. L., Lee, K. M., Wang, F., and Yoon, J. (2011). Visual detection of copper ions based on azide- and alkyne-functionalized polydiacetylene vesicles. J. Mater. Chem. 21, 15214–15217. doi: 10.1039/c1jm11660f
Yamamoto, T., Maruyama, T., Zhou, Z.-H., Ito, T., Fukuda, T., Yoneda, Y., et al. (1994). Π-conjugated poly(pyridine-2,5-diyl), poly(2,2'-bipyridine-5,5'-diyl), and their alkyl derivatives. Preparation, linear structure, function as a ligand to form their transition metal complexes, catalytic reactions, n-type electrically conducting properties, optical properties, and alignment on substrates. J. Am. Chem. Soc. 116, 4832–4845. doi: 10.1021/ja00090a031
Yasuda, T., Yamaguchi, I., and Yamamoto, T. (2003). A new soluble 1,10-phenanthroline-containing π-conjugated polymer: synthesis and effect of metal complexation on optical properties. Adv. Mater. 15, 293–296. doi: 10.1002/adma.200390070
Yasuda, T., and Yamamoto, T. (2003). Synthesis and characterization of new luminescent 1,10-phenanthroline- and pyridine-containing π-conjugated polymers. their optical response to protic acid, mn+, and solvents. Macromolecules 36, 7513–7519. doi: 10.1021/ma030280d
Yoon, J., Chae, S. K., and Kim, J. M. (2007). Colorimetric sensors for volatile organic compounds (vocs) based on conjugated polymer-embedded electrospun fibers. J. Am. Chem. Soc. 129, 3038–3039. doi: 10.1021/ja067856+
Youssoufi, H. K., Hmyene, M., Garnier, F., and Delabouglise, D. (1993). Cation recognition properties of polypyrrole 3-substituted by azacrown ethers J. Chem. Soc. Chem. Commun. 20, 1550–15552. doi: 10.1002/chin.199410200
Yu, H. H., Xu, B., and Swager, T. M. (2003). A proton-doped calix 4 arene-based conducting polymer. J. Am. Chem. Soc. 125, 1142–1143. doi: 10.1021/ja028545b
Zeng, Q., Cai, P., Li, Z., Qin, J. G., and Tang, B. Z. (2008). An imidazole-functionalized polyacetylene: convenient synthesis and selective chemosensor for metal ions and cyanide. Chem. Commun. 9, 1094–1096. doi: 10.1039/b717764j
Zeng, W. X., Deng, Z. F., Wang, H. R., Zhang, H. C., and Zhou, Q. X. (2019). Benzodifuranone based color-changing epoxy-polyamine coating. Dyes Pigm. 164, 198–205. doi: 10.1016/j.dyepig.2019.01.016
Zhang, H., Deng, R., Wang, J., Li, X., Chen, Y. M., Liu, K., et al. (2017). Crystalline organic pigment-based field-effect transistors. ACS Appl. Mater. Interfaces. 9, 21891–21899. doi: 10.1021/acsami.7b03170
Zhang, H., Li, R., Deng, Z., Cui, S., Wang, Y., Zheng, M., et al. (2020). Π-conjugated oligomers based on aminobenzodifuranone and diketopyrrolopyrrole. Dyes Pigm. 181:108552. doi: 10.1016/j.dyepig.2020.108552
Zhang, H. C., Yang, K., Zhang, K., Zhang, Z. Z., Sun, Q. K., and Yang, W. J. (2018). Thionating iso-diketopyrrolopyrrole-based polymers: from p-type to ambipolar field effect transistors with enhanced charge mobility. Polym. Chem. 9, 1807–1814. doi: 10.1039/c8py00292d
Zhang, H. C., Zeng, W. X., Du, H. L., Ma, Y. T., Ji, Z. T., Deng, Z. F., et al. (2020). Comparison for color change between benzodifuranone and benzodipyrrolidone based epoxy coating. Dyes Pigm. 175:108171. doi: 10.16/j.dyepig.2019.108171
Zhang, Y., Murphy, C. B., Wayne, E, and Jones, J. (2002). Poly[p-(phenyleneethynylene)-alt-(thienyleneethynylene)] polymers with oligopyridine pendant groups: highly sensitive chemosensors for transition metal ions. Macromolecules 35, 630–636. doi: 10.1021/ma011479y
Zhao, X. H., Liang, X. G., and Sun, Q. J. (2020). Spray printed conjugated polymer on tissue paper for highly sensitive pressure sensors. Polym. Int. doi: 10.1002/pi.6022
Zhou, G. D., Wang, F., Wang, H. L., Kambam, S., Chen, X. Q., and Yoon, J. (2013). Colorimetric and fluorometric assays based on conjugated polydiacetylene supramolecules for screening acetylcholinesterase and its inhibitors. ACS Appl. Mater. Interfaces 5, 3275–3280. doi: 10.1021/am400260y
Keywords: sensors, metal ions, π-conjugated polymers, chemosensors, ionochromism
Citation: Liu J, Han L, Geng J, Hua J and Wang Z (2020) Metal-Ligand Coordination Induced Ionochromism for π-Conjugated Materials. Front. Chem. 8:589106. doi: 10.3389/fchem.2020.589106
Received: 30 July 2020; Accepted: 01 September 2020;
Published: 02 October 2020.
Edited by:
Qixin Zhou, University of Akron, United StatesReviewed by:
Jun Ling, Zhejiang University, ChinaZhou Qiong, China University of Petroleum, China
Kun Yang, Southern University of Science and Technology, China
Copyright © 2020 Liu, Han, Geng, Hua and Wang. This is an open-access article distributed under the terms of the Creative Commons Attribution License (CC BY). The use, distribution or reproduction in other forums is permitted, provided the original author(s) and the copyright owner(s) are credited and that the original publication in this journal is cited, in accordance with accepted academic practice. No use, distribution or reproduction is permitted which does not comply with these terms.
*Correspondence: Jing Hua, aHVhamluZzcyQHF1c3QuZWR1LmNu; Zhaobo Wang, d2FuZ3poYkBxdXN0LmVkdS5jbg==