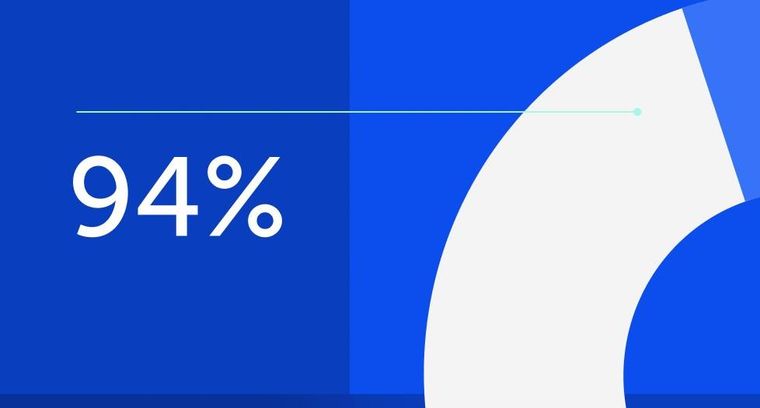
94% of researchers rate our articles as excellent or good
Learn more about the work of our research integrity team to safeguard the quality of each article we publish.
Find out more
MINI REVIEW article
Front. Chem., 12 August 2020
Sec. Chemical Physics and Physical Chemistry
Volume 8 - 2020 | https://doi.org/10.3389/fchem.2020.00692
This article is part of the Research TopicBioinspired Functional Surfaces with Superwettability: From Fabrication to ApplicationsView all 8 articles
The surface wettability of plants exhibits many unique advantages, which enhances the environmental adaptability of plants. In view of the rapid development of responsive materials, smart surfaces have been explored extensively to regulate surface wettability through external stimuli. Herein, we summarized recent advancements in bioinspired surfaces with switchable wettability. Typical bioinspired surfaces with switchable wettability and their emerging applications have been reviewed. In the end, we have discussed the remaining challenges and provided perspective on future development.
The surface wettability of plants exhibits unique advantages (Wu et al., 2009, 2010, 2011a,b; Jiang et al., 2011), which enhances the environmental adaptability and improves survival chances. (Zhang et al., 2012a, 2019b, 2020; Yong et al., 2017) For example, water droplets roll freely on the surface of lotus leaves, which shows self-cleaning characteristics (Figure 1a; Zhang et al., 2012c). Rose petals demonstrate water droplets pinning effect, which is helpful for keeping rose petals hydrated (Figure 1b; Zhang et al., 2012b) Water droplets on the surface of reed leaves prefer to flow along the direction of the parallel leaf veins (Figure 1c; Wang et al., 2015) This anisotropic rolling characteristic plays an important role in collecting dewdrops on the roots and improves the environmental adaptability in dry and hot climates (Jiang et al., 2016). Insects easily slide from the edge of pitcher plants to the inner bottom and provide nourishment for the pitcher plants (Figure 1d; Huang et al., 2017; Zhang et al., 2017). Similar to insects, water droplets are also easy to slide on the liquid-infused surface (Yong et al., 2018). In addition, there are plenty of stimulated-responsive creatures on our planet. For example, organisms show reversible deformable body postures under environment stimulate (Cui et al., 2019). Chameleon owns excellent camouflage capabilities (Jiang et al., 2019b). Venus Flytrap generates closure motions under external forces (Le et al., 2019). Pinecone opens in dry environment and closes in wet environment (Mulakkal et al., 2018). Currently, motivated by such examples with extreme wettability and stimulated-responsive creatures, bioinspired surfaces with switchable wettability have been proposed and prepared (Xin et al., 2018; Jiang et al., 2019a; Han et al., 2020; Li et al., 2020).
Figure 1. Bioinspired Smart Surfaces with Switchable Wettability. (a) Photograph of a lotus leaf. Reproduced from Wang et al. (2018b) with permission of WILEY-VCH. (b) Photograph of a rose. Reproduced from Wang et al. (2018b) with permission of WILEY-VCH. (c) Photograph of reed leaves. Reproduced from Jiang et al. (2018) with permission of American Chemical Society. (d) Photograph of Nepenthes pitcher plants. Reproduced from Huang et al. (2017) with permission of WILEY-VCH. The schemes for typical surfaces with switchable wettability based on (e) surface chemistry changing, (f) surface roughness changing, and (g) a combination changing of surface chemistry and surface roughness. (h) Photothermal response. Reproduced from Geng et al. (2018) with permission of WILEY-VCH. (i) Electric response. Reproduced from Oh et al. (2018) with permission of WILEY-VCH. (j) Magnetic response. Reproduced from Huang et al. (2017) with permission of WILEY-VCH. (k) Pneumatic response. Reproduced under the terms of the CC-BY Creative Commons Attribution 4.0 International License (http://creativecommons.org/licenses/by/4.0/) (Wang et al., 2018a). Copyright 2018, the authors, published by Springer Nature. (l) PH response. Reproduced from Zhu et al. (2017) with permission of American Chemical Society.
Smart surfaces have attracted considerable interests because the surface chemistry and surface roughness play an important role in controlling surface wettability (Fang et al., 2010; Xu et al., 2013; Huang et al., 2017; Wei et al., 2017). Nowadays, the rapid development of responsive materials has enabled surface chemistry and surface roughness change to switch surface wettability through external stimuli (Xin et al., 2016; Wu et al., 2017; You et al., 2018; Salter and Booth, 2019; Zhang et al., 2019c; Fu et al., 2020; Zou et al., 2020). Due to the reversible dynamic control capability, tremendous effects have been devoted to developing driving techniques (Yin et al., 2018; Liu et al., 2020; You et al., 2020) such as temperature, light, electric/magnetic fields, chemicals, and mechanical motion (Han et al., 2015a,b, 2016; Yong et al., 2015). Importantly, the stimulated-responsive bioinspired surface has great prospects in diverse applications, such as droplet manipulations, oil-water separation, cell culture, smart skin (Yang et al., 2018; Liu et al., 2019; Lu et al., 2019).
In this minireview, we focus on the recent advancements in bioinspired stimulated-responsive surfaces with switchable wettability. Typical examples, such as thermal/photothermal, electric, magnetic, mechanical motion and chemical response surfaces, have been summarized. Finally, the challenges and future perspective for smart surfaces with switchable wettability are also discussed.
Typically, surface chemistry and surface roughness play important roles in the surface wettability. According to the Cassie equation (Li et al., 2019; Zhang et al., 2019a; Namdari et al., 2020): cos θ* = f s cos θs - f a; f s + f a =1, θ* and θs are the apparent contact angle (CA) and intrinsic CA of the substrate. f s and f a are apparent area fractions of the substrate and air troughs. Therefore, the surface wettability becomes switchable when the surface chemistry and surface roughness change under different environment stimuli. For example, as shown in the Figure 1e, the surface is initially hydrophobic without stimuli because of the structured substrate. Under stimuli, the surface chemical composition changes, leading to the changing of θs. Therefore, the surface water CA changes. Similarly, as shown in the Figure 1f, the surface water CA changes when the apparent area fractions of the substrate and air troughs change (f s and f a) under environment stimuli. In addition, a combination change of surface chemistry and surface roughness can be used to design surfaces with switchable wettability (Figure 1g) because of the combination change of θs, f s, and f a.
Benefiting from temperature responsive shape memory polymer (SMP) materials, Cheng et al. have successfully developed thermal response surfaces that tune superhydrophobic characters between isotropic and anisotropic state (Cheng et al., 2018). The groove structure is prepared by heat-pressing a template on the micro/nanostructured pillars surface. The collapsed pillars would recover to the initial structure when the surrounding temperature is above the Tg. In particular, this thermal response surface shows outstanding rewritable capability. Besides, Geng et al. have made intensive efforts to develop a photothermal responsive tube based on PDMS/rGO-PNIPAm (Figure 1h; Geng et al., 2018). rGO converts light into heat. PNIPAm shows the reversible hydrophilic/hydrophobic switch. Therefore, the PDMS/rGO-PNIPAm tube can be used as an amazing sunlight-driven water transporter by gradient in the surface wettability.
In 2017, Wei et al. fabricated electric-responsive polypyrrole (Ppy) arrays (Wei et al., 2017). The Ppy array shows reversible morphological transition between hydrophobic nanotubes and hydrophilic nanotips. The morphological transition is because of the volume change of Ppy under different voltage. As a result, the water CAs of the Ppy array are 105 ± 15° under −0.8 V and 44 ± 10° under 0.5 V, respectively. Besides, Oh et al. demonstrated dielectric elastomer-actuated liquid-infused poroelastic film (Figure 1i; Oh et al., 2018) The elastomeric film contracts in the thickness direction and expands in-plane under voltage. Therefore, the liquid-infused poroelastic film can be used for droplet manipulations including droplet oscillation, jetting, mixing.
Magnetically transformable surface was constructed by conformally infusing a liquid lubricant into magnetically responsive hierarchical micropillars (Figure 1j; Huang et al., 2017) The surface shows superhydrophobic property when micropillars are perpendicular to the surface. Whereas, the surface shows slippery property when micropillars are parallel to the surface. This liquid-infused magnetism responsive surface shows adaptive liquid repellency. Besides, due to the switching wetting state, the magnetic response surface can be used for fog harvesting and liquid transport. Similarly, various magnetic response switching wetting surfaces have been successfully designed and fabricated based on PDMS@cobalt microparticles and PDMS@Fe3O4 (Cao et al., 2017; Li et al., 2018). Recently, Jiang et al. have developed magnetic response Janus microplates arrays (Jiang et al., 2019b). The microplates were prepared by casting a mixture of PDMS and magnetic particles into a polystyrene mold. Then, the one side of microplates was modified by superhydrophobic spray to obtain superhydrophobic property. Another side of microplates was scanned by femtosecond laser to expose the hydrophilic carbonyl irons particles.
Wang et al. developed a superhydrophobic PDMS skin that switches between lotus leaf and rose petal modes (Wang et al., 2018b). The superhydrophobic PDMS skins with monolithic and hierarchical structures were fabricated by direct laser writing technique. The water droplet rolled off with a slight tilt under ε <50% (rolling state). Whereas, the water droplet firmly stuck on the surface under ε >70% (pinning state). This smart surface shows potential in droplet manipulations by movable joints. Similarly, this group developed pneumatic surfaces by embedding micro-air-sac network in an elastomer (Figure 1k; Wang et al., 2018a). The surface exposes one surface and hiding the other by deflation and inflation.
Zhu et al. prepared polyphenylsulfone-pyridine (PPSU-Pyx)-based nanoporous membrane with switchable wettability in response to pH (Figure 1l; Zhu et al., 2017) Due to the conformational switch of pyridine pendants, the porous membrane shows reversibly switch wettability. The CAs of PPSU-Pyx are 63.3° in acid solution (pH = 3) and 106.5° in alkaline solution (pH = 11). Besides, Liu et al. developed chemical response structured copper surfaces by exchanging counterion from PFO− to Cl− (Liu et al., 2018). After the PFO− treatment, structured copper surfaces was filled with fluorine-containing groups, leading to hydrophobic characters. Whereas, the hydrophobic surface loses fluorine-containing groups after the Cl− treatment. As a result, the hydrophobic surface become hydrophilic.
In this minireview, we have summarized the typical stimulated-responsive surfaces with switchable wettability including thermal/photothermal, electric, magnetic, mechanical motion and chemical response surfaces. Taking advantage of the stimulated-responsive characters, the smart surfaces can be used as droplet manipulators, fog collection, smart skin, stem cell differentiation, and others. The further trend of smart surfaces with switchable wettability may be developed from new driving mechanism, fabrication methods, and broaden the application areas. We believe that continued efforts to smart surfaces with switchable wettability would have potential applications in the fields of bionic manufacturing, electronic information, biomedicine, etc.
All authors listed have made a substantial, direct and intellectual contribution to the work, and approved it for publication.
This work was supported by the National Natural Science Foundation of China (NSFC) (Nos. 61590930, 61905087, 61805098, and 61827826) and Postdoctoral Science Foundation of China (2020M670850).
The authors declare that the research was conducted in the absence of any commercial or financial relationships that could be construed as a potential conflict of interest.
Cao, M. Y., Jin, X., Peng, Y., Yu, C. M., Li, K., Liu, K. S., et al. (2017). Unidirectional wetting properties on multi-bioinspired magnetocontrollable slippery microcilia. Adv. Mater. Weinheim. 29:1606869. doi: 10.1002/adma.201606869
Cheng, Z. J., Zhang, D. J., Lv, T., Lai, H., Zhang, E. S., Kang, H. J., et al. (2018). Superhydrophobic shape memory polymer arrays with switchable isotropic/anisotropic wetting. Adv. Funct. Mater. 28:1705002. doi: 10.1002/adfm.201705002
Cui, H. Q., Zhao, Q. L., Wang, Y. L., and Du, X. M. (2019). Bioinspired actuators based on stimuli-responsive polymers. Chem. Asian J. 14, 2369–2387. doi: 10.1002/asia.201900292
Fang, H. H., Yang, J., Ding, R., Chen, Q. D., Wang, L., Xia, H., et al. (2010). Polarization dependent two-photon properties in an organic crystal. Appl. Phys. Lett 97:101101. doi: 10.1063/1.3486683
Fu, X. Y., Chen, Z. D., Han, D. D., Zhang, Y. L., Xia, H., and Sun, H. B. (2020). Laser fabrication of graphene-based supercapacitors. Photonics Res. 8, 577–588. doi: 10.1364/PRJ.382401
Geng, H. Y., Zhou, K., Zhou, J. J., Ma, H. Y., Lv, C. J., Li, C., et al. (2018). Sunlight-driven water transport via a reconfigurable pump. Angew. Chem. Intern. Edition 57, 15435–15440. doi: 10.1002/anie.201808835
Han, D. D., Zhang, Y. L., Jiang, H. B., Xia, H., Feng, J., Chen, Q. D., et al. (2015a). Moisture-responsive graphene paper prepared by self-controlled photoreduction. Adv. Materials 27, 332–338. doi: 10.1002/adma.201403587
Han, D. D., Zhang, Y. L., Liu, Y., Liu, Y. Q., Jiang, H. B., Han, B., et al. (2015b). Bioinspired graphene actuators prepared by unilateral UV irradiation of graphene oxide papers. Adv. Funct. Mater 25, 4548–4557. doi: 10.1002/adfm.201501511
Han, D. D., Zhang, Y. L., Ma, J. N., Liu, Y. Q., Han, B., and Sun, H. B. (2016). Light-mediated manufacture and manipulation of actuators. Adv. Mater. 28, 8328–8343. doi: 10.1002/adma.201602211
Han, D. D., Chen, Z. D., Li, J. C., Mao, J. W., Jiao, Z. Z., Wang, W., et al. (2020). Airflow enhanced solar evaporation based on Janus graphene membranes with stable interfacial floatability. ACS Appl. Mater. Interfaces. 12, 25435–25443. doi: 10.1021/acsami.0c05401
Huang, Y., Stogin, B. B., Sun, N., Wang, J., Yang, S. K., and Wong, T. S. (2017). A switchable cross-species liquid repellent surface. Adv. Mater. 29:1604641. doi: 10.1002/adma.201604641
Jiang, H. B., Liu, Y., Liu, J., Li, S. Y., Song, Y. Y., Han, D. D., et al. (2019a). Moisture-responsive graphene actuators prepared by two-beam laser interference of graphene oxide paper. Front. Chem. 7:464. doi: 10.3389/fchem.2019.00464
Jiang, H. B., Liu, Y. Q., Zhang, Y. L., Liu, Y., Fu, X. Y., Han, D. D., et al. (2018). Reed leaf-inspired graphene films with anisotropic superhydrophobicity. ACS Appl. Mater. Interfaces 10, 18416–18425. doi: 10.1021/acsami.8b03738
Jiang, H. B., Zhang, Y. L., Liu, Y., Fu, X. Y., Li, Y. F., Liu, Y. Q., et al. (2016). Bioinspired few-layer graphene prepared by chemical vapor deposition on femtosecond laser-structured Cu foil. Laser Photon. Rev. 10, 441–450. doi: 10.1002/lpor.201500256
Jiang, S. J., Hu, Y. L., Wu, H., Zhang, Y. C., Zhang, Y. Y., Wang, Y. L., et al. (2019b). Multifunctional Janus microplates arrays actuated by magnetic fields for water/light switches and bio-inspired assimilatory coloration. Adv. Mater. 31:1807507. doi: 10.1002/adma.201807507
Jiang, Y., Wang, H. Y., Wang, H., Gao, B. R., Hao, Y. W., Jin, Y., et al. (2011). Surface plasmon enhanced fluorescence of dye molecules on metal grating films. J. Phys. Chem. C 115, 12636–12642. doi: 10.1021/jp203530e
Le, X. X., Lu, W., Zhang, J. W., and Chen, T. (2019). Recent progress in biomimetic anisotropic hydrogel actuators. Adv. Sci 6:1801584. doi: 10.1002/advs.201801584
Li, D. K., Huang, J. X., Han, G. C., and Guo, Z. G. (2018). A facile approach to achieve bioinspired PDMS@Fe3O4 fabric with switchable wettability for liquid transport and water collection. J. Mater. Chem. A 6, 22741–22748. doi: 10.1039/C8TA08993K
Li, J., Li, J. Q., Sun, J., Feng, S. L., and Wang, Z. K. (2019). Biological and engineered topological droplet rectifiers. Adv. Mater. 31:1806501. doi: 10.1002/adma.201806501
Li, Z. Z., Wang, L., Fan, H., Yu, Y. H., Chen, Q. D., Juodkazis, S., et al. (2020). O-FIB: far-field-induced near-field breakdown for direct nanowriting in an atmospheric environment. Light Sci. Applicat. 9:41. doi: 10.1038/s41377-020-0275-2
Liu, Y., Gao, H. P., Li, S. Y., Han, Z. W., and Ren, L. Q. (2018). Bioinspired platform with reversibly switchable wettability for transfer and storage of droplets. Chem. Eng. J. 337, 697–708. doi: 10.1016/j.cej.2017.12.139
Liu, Y. Q., Chen, Z. D., Mao, J. W., Han, D. D., and Sun, X. Y. (2019). Laser fabrication of graphene-based electronic skin. Front. Chem. 7:461. doi: 10.3389/fchem.2019.00461
Liu, Y. Q., Mao, J. W., Chen, Z. D., Han, D. D., Jiao, Z. Z., Ma, J. N., et al. (2020). Three-dimensional micropatterning of graphene by femtosecond laser direct writing technology. Opt. Lett. 45, 113–116. doi: 10.1364/OL.45.000113
Lu, Z., He, F., Hsieh, C. Y., Wu, X., Song, M., Liu, X., et al. (2019). Magnetic hierarchical photocatalytic nanoreactors: toward highly selective Cd2+ removal with secondary pollution free tetracycline degradation. ACS Appl. Nano Mater. 2, 1664–1674. doi: 10.1021/acsanm.9b00113
Mulakkal, M. C., Trask, R. S., Ting, V. P., and Seddon, A. M. (2018). Responsive cellulose-hydrogel composite ink for 4D printing. Mater. Des. 160, 108–118. doi: 10.1016/j.matdes.2018.09.009
Namdari, N., Mohammadian, B., Jafari, P., Mohammadi, R., Sojoudi, H., Ghasemi, H., et al. (2020). Advanced functional surfaces through controlled damage and instabilities. Mat. Horizons 7, 366–396. doi: 10.1039/C9MH01516G
Oh, I., Keplinger, C., Cui, J. X., Chen, J. H., Whitesides, G. M., Aizenberg, J., et al. (2018). Dynamically actuated liquid-infused poroelastic film with precise control over droplet dynamics. Adv. Funct. Mater 28:1802632. doi: 10.1002/adfm.201802632
Salter, P. S., and Booth, M. J. (2019). Adaptive optics in laser processing. Light-Sci. Appl. 8:110. doi: 10.1038/s41377-019-0215-1
Wang, J. N., Liu, Y. Q., Zhang, Y. L., Feng, J., and Sun, H. B. (2018a). Pneumatic smart surfaces with rapidly switchable dominant and latent superhydrophobicity. NPG Asia Mater. 10:e470. doi: 10.1038/am.2017.218
Wang, J. N., Liu, Y. Q., Zhang, Y. L., Feng, J., Wang, H., Yu, Y. H., et al. (2018b). Wearable superhydrophobic elastomer skin with switchable wettability. Adv. Funct. Mater. 28:1800625. doi: 10.1002/adfm.201800625
Wang, J. N., Zhang, Y. L., Liu, Y., Zheng, W. H., Lee, L. P., and Sun, H. B. (2015). Recent developments in superhydrophobic graphene and graphene-related materials: from preparation to potential applications. Nanoscale 7, 7101–7114. doi: 10.1039/C5NR00719D
Wei, Y., Mo, X. J., Zhang, P. C., Li, Y. Y., Liao, J. W., Li, Y. J., et al. (2017). Directing stem cell differentiation via electrochemical reversible switching between nanotubes and nanotips of polypyrrole array. ACS Nano 11, 5915–5924. doi: 10.1021/acsnano.7b01661
Wu, D., Chen, Q. D., Niu, L. G., Wang, J. N., Wang, J., Wang, R., et al. (2009). Femtosecond laser rapid prototyping of nanoshells and suspending components towards microfluidic devices. Lab. Chip. 9, 2391–2394. doi: 10.1039/b902159k
Wu, D., Wang, J. N., Wu, S. Z., Chen, Q. D., Zhao, S. A., Zhang, H., et al. (2011a). Three-level biomimetic rice-leaf surfaces with controllable anisotropic sliding. Adv. Funct. Mater 21, 2927–2932. doi: 10.1002/adfm.201002733
Wu, D., Wu, S. Z., Chen, Q. D., Zhao, S., Zhang, H., Jiao, J., et al. (2011b). Facile creation of hierarchical PDMS microstructures with extreme underwater superoleophobicity for anti-oil application in microfluidic channels. Lab Chip 11, 3873–3879. doi: 10.1039/c1lc20226j
Wu, D., Wu, S. Z., Niu, L. G., Chen, Q. D., Wang, R., Song, J. F., et al. (2010). High numerical aperture microlens arrays of close packing. Appl. Phys. Lett. 97:031109. doi: 10.1063/1.3464979
Wu, J., Wang, H., Su, Z., Zhang, M., Hu, X., Wang, Y., et al. (2017). Highly flexible and sensitive wearable e-skin based on graphite nanoplatelet and polyurethane nanocomposite films in mass industry production available. ACS Appl. Mater. Interf. 9, 38745–38754. doi: 10.1021/acsami.7b10316
Xin, W., Chen, X. D., Liu, Z. B., Jiang, W. S., Gao, X. G., Jiang, X. Q., et al. (2016). Photovoltage enhancement in twisted-bilayer graphene using surface plasmon resonance. Adv. Opt. Mater. 4, 1703–1710. doi: 10.1002/adom.201600278
Xin, W., Li, X. K., He, X. L., Su, B. W., Jiang, X. Q., Huang, K. X., et al. (2018). Black-phosphorus-based orientation-induced diodes. Adv. Mater. 30:1704653. doi: 10.1002/adma.201704653
Xu, B. B., Zhang, Y. L., Xia, H., Dong, W. F., Ding, H., and Sun, H. B. (2013). Fabrication and multifunction integration of microfluidic chips by femtosecond laser direct writing. Lab. Chip. 13, 1677–1690. doi: 10.1039/c3lc50160d
Yang, S., Yin, K., Chu, D. K., He, J., and Duan, J. A. (2018). Femtosecond laser structuring of Janus foam: Water spontaneous antigravity unidirectional penetration and pumping. Appl. Phys. Lett 113:203701. doi: 10.1063/1.5061723
Yin, K., Yang, S., Dong, X. R., Chu, D. K., Duan, J. A., and He, J. (2018). Ultrafast achievement of a superhydrophilic/hydrophobic Janus foam by femtosecond laser ablation for directional water transport and efficient fog harvesting. ACS Appl. Mater. Interfaces 10, 31433–31440. doi: 10.1021/acsami.8b11894
Yong, J. L., Chen, F., Yang, Q., Farooq, U., and Hou, X. (2015). Photoinduced switchable underwater superoleophobicity–superoleophilicity on laser modified titanium surfaces. J. Mater. Chem. A 3, 10703–10709. doi: 10.1039/C5TA01782C
Yong, J. L., Chen, F., Yang, Q., Huo, J. L., and Hou, X. (2017). Superoleophobic surfaces. Chem. Soc. Rev. 46, 4168–4217. doi: 10.1039/C6CS00751A
Yong, J. L., Huo, J. L., Yang, Q., Chen, F., Fang, Y., Wu, X. J., et al. (2018). Femtosecond laser direct writing of porous network microstructures for fabricating super-slippery surfaces with excellent liquid repellence and anti-cell proliferation. Adv. Mater. Interfaces 5:1701479. doi: 10.1002/admi.201701479
You, R., Han, D. D., Liu, F. M., Zhang, Y. L., and Lu, G. Y. (2018). Fabrication of flexible room-temperature NO2 sensors by direct laser writing of In2O3 and graphene oxide composites. Sensors Actuators B Chem. 277, 114–120. doi: 10.1016/j.snb.2018.07.179
You, R., Liu, Y. Q., Hao, Y. L., Han, D. D., Zhang, Y. L., and You, Z. (2020). Laser fabrication of graphene-based flexible electronics. Adv. Mater. 32:1901981. doi: 10.1002/adma.201901981
Zhang, P. C., Zhao, C. Q., Zhao, T. Y., Liu, M. J., and Jiang, L. (2019a). Recent advances in bioinspired gel surfaces with superwettability and special sdhesion. Adv. Sci. 6:1900996. doi: 10.1002/advs.201900996
Zhang, P. F., Zhang, L. W., Chen, H. W., Dong, Z. C., and Zhang, D. Y. (2017). Surfaces inspired by the nepenthes peristome for unidirectional liquid transport. Adv. Mater. 29:995. doi: 10.1002/adma.201702995
Zhang, X. L., Song, J. F., Li, X. B., Feng, J., and Sun, H. B. (2012a). Optical tamm states enhanced broad-band absorption of organic solar cells. Appl. Phys. Lett 101:243901. doi: 10.1063/1.4770316
Zhang, Y. L., Chen, Q. D., Jin, Z., Kim, E., and Sun, H. B. (2012b). Biomimetic graphene films and their properties. Nanoscale 4, 4858–4869. doi: 10.1039/c2nr30813d
Zhang, Y. L., Liu, Y. Q., Han, D. D., Ma, J. N., Wang, D., Li, X. B., et al. (2019b). Quantum-confined-superfluidics-enabled moisture actuation based on unilaterally structured graphene oxide papers. Adv. Mater. 31:1901585. doi: 10.1002/adma.201901585
Zhang, Y. L., Ma, J. N., Liu, S., Han, D. D., Liu, Y. Q., Chen, Z. D., et al. (2020). A “Yin” - “Yang” complementarity strategy for design and fabrication of dual-responsive bimorph actuators. Nano Energy 68:104302. doi: 10.1016/j.nanoen.2019.104302
Zhang, Y. L., Xia, H., Kim, E., and Sun, H. B. (2012c). Recent developments in superhydrophobic surfaces with unique structural and functional properties. Soft Matter. 8, 11217–11231. doi: 10.1039/c2sm26517f
Zhang, Y. Y., Jiao, Y. L., Chen, C., Zhu, S. W., Li, C. Z., Li, J. W., et al. (2019c). Reversible tuning between isotropic and anisotropic sliding by one-direction mechanical stretching on microgrooved slippery surfaces. Langmuir 35, 10625–10630. doi: 10.1021/acs.langmuir.9b01035
Zhu, X. B., Zhou, Y. H., Hao, J. R., Bao, B., Bian, X. J., Jiang, X. Y., et al. (2017). A charge-density-tunable three/two-dimensional polymer/graphene oxide heterogeneous nanoporous membrane for ion transport. ACS Nano 11, 10816–10824. doi: 10.1021/acsnano.7b03576
Keywords: bioinspired surfaces, smart surfaces, switchable wettability, fabrication, applications
Citation: Han D-D, Cai Q, Chen Z-D, Li J-C, Mao J-W, Lv P and Gao B-R (2020) Bioinspired Surfaces With Switchable Wettability. Front. Chem. 8:692. doi: 10.3389/fchem.2020.00692
Received: 25 May 2020; Accepted: 03 July 2020;
Published: 12 August 2020.
Edited by:
Jiale Yong, Xi'an Jiaotong University, ChinaReviewed by:
Moyuan Cao, Tianjin University, ChinaCopyright © 2020 Han, Cai, Chen, Li, Mao, Lv and Gao. This is an open-access article distributed under the terms of the Creative Commons Attribution License (CC BY). The use, distribution or reproduction in other forums is permitted, provided the original author(s) and the copyright owner(s) are credited and that the original publication in this journal is cited, in accordance with accepted academic practice. No use, distribution or reproduction is permitted which does not comply with these terms.
*Correspondence: Bing-Rong Gao, YnJnYW9Aamx1LmVkdS5jbg==
†These authors have contributed equally to this work
Disclaimer: All claims expressed in this article are solely those of the authors and do not necessarily represent those of their affiliated organizations, or those of the publisher, the editors and the reviewers. Any product that may be evaluated in this article or claim that may be made by its manufacturer is not guaranteed or endorsed by the publisher.
Research integrity at Frontiers
Learn more about the work of our research integrity team to safeguard the quality of each article we publish.