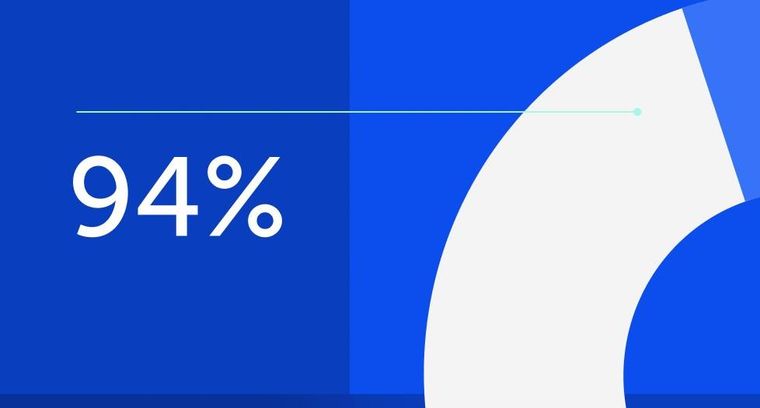
94% of researchers rate our articles as excellent or good
Learn more about the work of our research integrity team to safeguard the quality of each article we publish.
Find out more
ORIGINAL RESEARCH article
Front. Chem., 19 June 2020
Sec. Organic Chemistry
Volume 8 - 2020 | https://doi.org/10.3389/fchem.2020.00429
This article is part of the Research TopicSynthetic Methodologies for Privileged Natural Product ScaffoldsView all 8 articles
Benzimidazoles are important N-heteroaromatic compounds with various biological activities and pharmacological applications. Herein, we present the first iron-catalyzed selective synthesis of 1,2-disubstituted benzimidazoles via acceptorless dehydrogenative coupling of primary alcohols with aromatic diamines. The tricarbonyl (η4-cyclopentadienone) iron complex catalyzed dehydrogenative cyclization, releasing water and hydrogen gas as by-products. The earth abundance and low toxicity of iron metal enable the provision of an eco-friendly and efficient catalytic method for the synthesis of benzimidazoles.
Benzimidazoles, which have been found in pharmaceuticals and natural products, are important N-heteroaromatic structural motifs because of their biological activities (Bansal and Silakari, 2012; Chandrika et al., 2016; Suk et al., 2019). Of these, the 1,2-disubstituted benzimidazole is considered a privileged scaffold in drug discovery. As shown in Figure 1, many drugs contain this moiety in their structures, for example, maribavir (antiviral), mizolastine (antihistamine), and telmisartan and candesartan (antihypertensive). Furthermore, 1,2-disubstituted benzimidazoles show various biological activities, such as anticancer (Zimmermann et al., 2013, 2014) antibacterial (Bandyopadhyay et al., 2011; Göker et al., 2016), antiallergic (Nakano et al., 2000), and anti-HIV (Morningstar et al., 2007) traits along with cannabinoid-1 (CB1) and cannabinoid-2 (CB2) receptors (Watson et al., 2011; Nanda et al., 2014). Based on their attractive biological profiles, the synthesis of 1,2-disubstituted benzimidazoles has gained the interest of synthetic chemists.
Diverse synthetic approaches have been reported for the synthesis of 1,2-disubstituted benzimidazoles (Scheme 1). The first is the respective substitution on the C-1 or N-2 position of the preformed benzimidazoles, (i) N-alkylation of 2-substituted benzimidazoles (Ramla et al., 2007; Martin et al., 2015) and (ii) Suzuki coupling of aryl boronic acids with 1-halo-2-alkylbenzimidazoles (Wang and Smith, 2003; Martin et al., 2015). Another approach is the classic cyclocondensation of (iii) N-alkyl-N-acyl-1,2-diaminobenzene (Smith and Krchnák, 1999; Takeuchi et al., 2000) or (iv) N-alkyl-1,2-diaminobenzene with aldehyde (Smith and Krchnák, 1999; Özden et al., 2005). In addition, a large number of (v) direct one-pot cyclocondensations of 1,2-diaminobenzene with aldehydes have been reported (Chebolu et al., 2012; Girish et al., 2015; Sharma et al., 2015). This appears to be a straightforward approach; however, selectivity control between 2-substituted and 1,2-disubstituted benzimidazoles is often problematic.
During the past decade, the borrowing hydrogen (BH) strategy has become a powerful tool for the benign and sustainable construction of C–N bonds using abundant alcohols as coupling reagents (Mutti et al., 2015; Yang et al., 2015). C–N bond couplings through BH usually proceed in the following sequence (Figure 2, blue): dehydrogenation of an alcohol to the corresponding carbonyl compound, followed by condensation and reduction of imine using the borrowed H2 from the alcohol. If the imine participates in the aromatic system, the last hydrogenation step is suppressed, and the hydrogen gas is liberated (Figure 2, red), so it is called acceptorless dehydrogenative coupling (ADC). ADCs are highly efficient and environmentally benign methods to construct N-heteroaromatic structures since water and hydrogen gas are the only valuable by-products (Gunanathan and Milstein, 2013; Michlik and Kempe, 2013; Nandakumar et al., 2015). In recent years, considerable progress has been directed toward the synthesis of benzimidazole involving dehydrogenative coupling (Scheme 2); however, most of these methods use precious noble metals, such as Ru- (Blacker et al., 2009; Li et al., 2018), Ir- (Hille et al., 2014; Sharma et al., 2017), and Pd-based catalysts (Mori et al., 2019). The replacement of noble-metal catalysts by inexpensive and environmentally friendly earth-abundant base metals is an important task for organic chemists. Among the base metals, Cu- (Xu et al., 2017, 2018), Co- (Daw et al., 2017), Ni- (Bera et al., 2019), and Mn-based catalysts (Das et al., 2018; Zhang et al., 2019) have been well-applied in the condensation of alcohols with 1,2-diaminobenzene to benzimidazoles. However, many of these metal complexes utilize quite expensive or labile ligands to achieve higher product yields, which is a major concern in comparison to the advantages of base metals. Iron is the second most earth-abundant and highly desirable metal catalyst in the synthesis of pharmaceuticals due to its low toxicity (Bauer and Knölker, 2015; Fürstner, 2016). The tricarbonyl (η4-cyclopentadienone) iron complexes were initially described by Knölker (Knölker et al., 1999), and they have a core bifunctional structure to mediate the BH process consisting of both a proton-donor site (ligand) and a hydride-donor site (metal center) (Ikariya and Blacker, 2007). Since it was first developed, Knölker's complex has been widely applied in C–N or C–C bond formation through a BH strategy (Yan et al., 2014; Brown et al., 2017; Reed-Berendt et al., 2019). Based on the previous results, we envisioned the possibility of iron-catalyzed direct benzimidazole formation starting from 1,2-diaminobenzene and alcohol via the ADC strategy. To the best of our knowledge, the synthesis of benzimidazoles directly from 1,2-diaminobenzene and alcohol catalyzed by iron has not been reported. Herein, we describe a selective method to synthesize 1,2-disubstituted benzimidazoles using Knölker-type iron complexes as a catalyst.
Scheme 2. Transition metal catalyzed 1,2-disubstituted benzimidazole synthesis via dehydrogenative coupling of alcohol.
All catalytic reactions were carried out under nitrogen atmosphere using a Schlenk flask. Fe complexes cat. I–V (Moulin et al., 2013) and cat. VI (Dambatta et al., 2019) were prepared according to the literature. All commercially available reagents and solvents (purchased from Sigma-Aldrich, TCI, Alfa-Aesar and Acros) were used without further purification unless otherwise noted. Reactions were monitored by thin-layer chromatography on silica gel 60 F254 plate using UV illumination at 254 nm. Column chromatography was performed on silica gel (230–400 mesh), using a mixture of hexane and ethyl acetate as eluents. Nuclear magnetic resonance (1H-NMR and 13C-NMR) spectra were measured on JEOL JNM-ECZ400s [400 MHz (1H), 100 MHz (13C)], using CDCl3 as solvent. It was reported in ppm relative to CDCl3 (δ 7.26) for 1H-NMR and relative to the central CDCl3 (δ 77.16) for 13C-NMR. Coupling constants (J) in 1H-NMR and 13C-NMR are in hertz. All high-resolution mass spectra (HR-MS) were acquired under fast atom bombardment (FAB) condition on a JMS-700 MStation mass spectrometer. Melting points were measured on a Büchi B-540 melting point apparatus and were not corrected. X-ray diffraction studies were carried out in a Super Nova, Dual, Mo at home/near, Atlas S2 diffractometer.
In a 15-ml Schlenk flask, a mixture of 1,2-diaminobenzene (1a, 54.05 mg, 0.5 mmol), alcohol (2, 1.5 mmol), tBuOK (84.16 mg, 0.75 mmol), cat. I (8.36 mg, 0.02 mmol), and TMAO (3.0 mg, 0.04 mmol) was stirred at 150°C in xylene (2 ml) for 24 h under a nitrogen atmosphere. Then, the reaction mixture was cooled to room temperature and diluted with dichloromethane. After removing the solvent, the resulting residue was further purified by column chromatography on silica gel using 10–30% ethyl acetate in hexane as an eluent to obtain the desired benzimidazoles.
Following the general procedure with 1a and 2a, 3a was obtained as white solid (121 mg, 85% yield). m.p. 128–130°C. 1H-NMR (400 MHz, CDCl3) δ 7.88 (d, J = 7.8 Hz, 1H), 7.69 (dd, J = 7.5, 1.6 Hz, 2H), 7.46 (dd, J = 13.0, 5.7 Hz, 3H), 7.29–7.33 (m, 4H), 7.23 (q, J = 7.5 Hz, 2H), 7.11 (d, J = 6.9 Hz, 2H), 5.46 (s, 2H). 13C-NMR (100 MHz, CDCl3) δ 154.24, 143.27, 136.46, 136.14, 130.15, 129.99, 129.31, 129.12, 128.83, 127.84, 126.02, 123.10, 122.74, 120.06, 110.61, 48.43. HRMS (FAB+) m/z calcd for C20H17N2 [M+H]+: 285.1392, found: 285.1382.
In a preliminary study, we explored the feasibility of benzimidazole formation between 1,2-diaminobenzene 1a and benzyl alcohol 2a using standard Knölker complex cat. I (Table 1). The reaction was carried out in toluene, and trimethylamine N-oxide (TMAO) was used to activate cat. I and liberate a vacant site in situ. In the first trial, no benzimidazole products were formed in the absence of a base (entry 1). Based on previous reports (Xu et al., 2017; Das et al., 2018), we expected that a stoichiometric amount of base is required for benzimidazole formation. Various kinds of bases were screened in the reaction system, and tBuOK was found to be a more effective base than KOH and K2CO3 for the formation of 1,2-disubstituted benzimidazoles 3a (entries 2–4). Surprisingly, we could not detect any 2-mono-substituted benzimidazole product in the reaction. Higher conversion was achieved when the reaction temperature was increased from 130 to 150°C (entries 4 and 5). Next, we examined the efficacy of different solvents and neat conditions, and the best yield of 3a (85%) was obtained in xylene (entries 5–8). The control experiment was also performed, and it was revealed that no desired product was obtained in the absence of a catalyst, demonstrating a crucial role of iron complex in the dehydrogenative coupling (entry 9). Additionally, we tried to reduce the amount of alcohol 2a and base in the reaction; however, slightly lower yields were observed (entries 10 and 11). To investigate a feasibility for the selective synthesis of 2-mono-substituted benzimidazole, 1.0 equivalent of 2a was reacted with 1a. Unfortunately, 1,2-disubstituted and 2-substituted benzimidazoles were obtained in 17 and 6% yields, respectively (entry 12).
As we were optimizing the reaction conditions, the effect of the amount of catalysts was also investigated (Table 2). Decreasing the loading of catalyst from 4 to 3 mol% resulted in 80% yield of desired product 3a, and a small amount of diamine substrate 1a remained. Interestingly, when we increased the catalyst loading to 5 mol%, a significantly decreased yield of 3a and increased formation of N, N-dibenzylbenzene-1,2-diamine 4 were observed. We supposed that a large amount of catalyst accelerated imine reduction competitively with the annulation process. Various Knölker-type complexes were also explored to estimate their activity in the reaction, and the results are shown in Table 2. The cat. VI gave desired product 3a in good yield (80%), similar to that of cat. I. However, the cat. II and IV showed moderate efficiency and cat. III and V resulted in low efficiency. Based on the above results, we choose the optimal dehydrogenative coupling conditions as diamine 1 (1.0 equiv.), alcohol 2 (3.0 equiv.), cat. I (4 mol%), TMAO (8 mol%), and tBuOK (1.5 equiv.) in xylene (2 ml) at 150°C under N2 for 24 h.
We applied the optimized conditions on a variety of diamines 1 and alcohols 2 to explore the reaction scope. First, a wide range of alcohol 2 was employed for annulation with 1a (Table 3). Benzyl alcohols containing electron-donating groups in the phenyl ring showed good yields (3b–f, 75–83%). The steric effect slightly influenced the formation of the desired product, depending on the position of the substituent. Substrates with substituents at the ortho position showed slightly lower yields than those of meta- and para-substituted analogs (yield sequence order: para > meta > ortho). 4-Chlorobenzyl alcohol afforded excellent yield for the corresponding product (3g, 92%), whereas low yield was obtained in the case of bromo- and iodo-substituted analogs with loss of one halogen atom (3h and 3i, 52–59%). This partial dehalogenation might be involved in the hydrogenative activity of the hydrogenated iron complex, which could be formed in situ. The molecular structure of 3i was confirmed by X-ray crystal structure as shown in Table 3. XRD data showed that the N-substituted benzyl group has iodine and C-2-substituted phenyl ring loose iodine. A series of alcohols containing heterocycles, such as furan, thiophene, and pyridine, were well-applied and afforded the desired products in good yields (3j–l, 72–82%). In the case of 1,3-benzodioxole-5-methanol and 4-trifluoromethyl benzyl alcohol, the desired products were obtained in moderate yield even if a longer reaction time is needed for full conversion (3m and 3n, 67–68%). Additionally, 1-naphthalene methanol was also applied in the reaction system and gave the corresponding product in high yield (3o, 85%). For further expansion of the alcohol scope, aliphatic alcohols such as 1-hexanol and 3-phenyl propanol were also investigated. Aliphatic alcohols could participate in dehydrogenative coupling; however, desired products were obtained in low yields (3p–q, 38–40%). After the screening of alcohols, the scope of diamine 1 was also investigated (Table 4). Under the same conditions, the reaction of 4,5-dimethyl-1,2-diaminobenzene with 2a proceeded smoothly and afforded the product inwas also investigated good yield (5a, 81%). On the other hand, 3,4-diaminotoluene and 4-chloro-1,2-diaminobenzene gave a mixture of 1-benzyl-2-phenyl-benzimidazole products (5b and 5c). To explore the possibility of imidazole formation, we employed 1,2-diphenyl-1,2-ethylenediamine as a substrate. Unfortunately, the corresponding imidazole product was obtained in very low yield (5d, 20%).
The above successful results led us to further investigate the reaction generality. N-Benzyl-1,2-diaminobenzene 6 was designed for the selective introduction of a substituent on the N-1 or C-2 position of benzimidazole. As shown in Table 5, benzylic alcohol 2 usually participates in the annulation process and is located on C-2 and its substituents on the benzimidazole product (7a–e). In contrast, five-membered heteroaromatic methyl alcohols gave N-1-heteroarylmethyl-C-2-phenyl-benzoimidazole products (7f and 7g). This opposite selectivity is expected to depend on the electron density of the aromatic group. Furthermore, we applied the iron complex to achieve the direct N-alkylation of benzimidazole 8 with 2a (Scheme 3). Unfortunately, no desired product was observed; however, this result suggests that the reaction mechanism did not proceed through benzimidazole as an intermediate. Besides benzimidazole, 2-phenyl benzothiazole 10 was also successfully synthesized in high yield (87%) using 2-aminobenzenethiol 9 under optimized reaction conditions (Scheme 4).
Scheme 4. Synthesis of 2-phenylbenzo[d]thiazole. Reaction conditions: 9 (0.5 mmol), 2a (1.5 mmol), tBuOK (0.75 mmol), cat. I (0.02 mmol), TMAO (0.04 mmol), and xylene (2 ml) in Schlenk flasks under N2, 24 h, 150°C.
Based on the above observations and previous reports (Xu et al., 2017, 2018; Das et al., 2018), we proposed a plausible mechanism as shown in Scheme 5. Initially, aldehyde A was generated from alcohol via iron-catalyzed dehydrogenation. Then, the formation of bisimine intermediate B took place through the condensation of diamine 1 with aldehyde A. Bisimine B underwent intramolecular cyclization, followed by rearrangement to give 1,2-disubstituted benzimidazole 3 (Path a) (Chebolu et al., 2012). As mentioned in Table 2, we also identified diamine 4 as a side product, which might be generated from bisimine B through Fe-H2-mediated hydrogenation. On the other hand, N-benzyl-1,2-diaminobenzene 6 also reacted with the aldehyde A and generated imine intermediate D (Path b). Cyclic intermediate E was formed by an intramolecular nucleophilic attack, followed by iron-catalyzed dehydrogenation and aromatization, leading to the formation of 1,2-disubstituted benzimidazoles (7a–e). In the case of alcohols substituted with electron-rich heteroaromatic groups, such as furan and thiophene, an intramolecular nucleophilic attack of D might be less favored. Thus, it is rearranged quickly to intermediate F, and a regioisomer (7f and 7g) was produced following a similar process.
In conclusion, we have reported the first iron-catalyzed synthesis of 1,2-disubstituted benzimidazoles using alcohol oxidation-level substrates via the ADC strategy. The Knölker-type catalysts, tricarbonyl (η4-cyclopentadienone) iron complexes, were successfully employed in the dehydrogenative coupling of alcohol with 1,2-diaminobenzene, followed by annulation to give the 1,2-disubstituted benzimidazole products in good yields. Under the developed conditions, the reaction of N-benzyl-1,2-diaminobenzene with alcohols also provided 1,2-disubstituted benzimidazoles, and the regioselectivity of the substituents depends on the electron density of the alcohol substrate. In addition to benzoimidazole, benzothiazole was also synthesized well using the developed method. Iron is an earth-abundant and low-toxicity metal, and water and hydrogen gas are liberated as by-products in the reaction. Therefore, this methodology provides an eco-friendly alternative for the selective synthesis of 1,2-disubstituted benzimidazoles. Further extension using the Knölker-type complex to access other types of N-heterocycles is under investigation in our research group.
All datasets generated for this study are included in the article/Supplementary Material.
RP conducted the most experiments and wrote the manuscript. SC prepared iron complex. SL conducted the initial experiments. D-CO reviewed and edited the manuscript. SH directed the project and co-wrote the manuscript.
This work was supported by the Research Resettlement Fund for the new faculty of Seoul National University. This work was also supported by the National Research Foundation of Korea (NRF) grant funded by the Korean government (2018R1C1B6005607 and 2018R1A4A1021703).
The authors declare that the research was conducted in the absence of any commercial or financial relationships that could be construed as a potential conflict of interest.
The supporting information and X-ray data for this article can be found online at: https://www.frontiersin.org/articles/10.3389/fchem.2020.00429/full#supplementary-material
Bandyopadhyay, P., Sathe, M., Ponmariappan, S., Sharma, A., Sharma, P., Srivastava, A. K., et al. (2011). Exploration of in vitro time point quantitative evaluation of newly synthesized benzimidazole and benzothiazole derivatives as potential antibacterial agents. Bioorg. Med. Chem. Lett. 21, 7306–7309. doi: 10.1016/j.bmcl.2011.10.034
Bansal, Y., and Silakari, O. (2012). The therapeutic journey of benzimidazoles: a review. Bioorg. Med. Chem. 20, 6208–6236. doi: 10.1016/j.bmc.2012.09.013
Bauer, I., and Knölker, H. J. (2015). Iron catalysis in organic synthesis. Chem. Rev. 115, 3170–3387. doi: 10.1021/cr500425u
Bera, A., Sk, M., Singh, K., and Banerjee, D. (2019). Nickel-catalysed dehydrogenative coupling of aromatic diamines with alcohols: selective synthesis of substituted benzimidazoles and quinoxalines. Chem. Commun. 55, 5958–5961. doi: 10.1039/C9CC02319D
Blacker, A. J., Farah, M. M., Hall, M. I., Marsden, S. P., Saidi, O., and Williams, J. M. (2009). Synthesis of benzazoles by hydrogen-transfer catalysis. Org. Lett. 11, 2039–2042. doi: 10.1021/ol900557u
Brown, T. J., Cumbes, M., Diorazio, L. J., Clarkson, G. J., and Wills, M. (2017). Use of (cyclopentadienone) iron tricarbonyl complexes for C-N bond formation reactions between amines and alcohols. J. Org Chem. 82, 10489–10503. doi: 10.1021/acs.joc.7b01990
Chandrika, N. T., Shrestha, S. K., Ngo, H. X., and Garneau-Tsodikova, S. (2016). Synthesis and investigation of novel benzimidazole derivatives as antifungal agents. Bioorg. Med. Chem. 24, 3680–3686. doi: 10.1016/j.bmc.2016.06.010
Chebolu, R., Kommi, D. N., Kumar, D., Bollineni, N., and Chakraborti, A. K. (2012). Hydrogen-bond-driven electrophilic activation for selectivity control: scope and limitations of fluorous alcohol-promoted selective formation of 1, 2-disubstituted benzimidazoles and mechanistic insight for rationale of selectivity. J. Org. Chem. 77, 10158–10167. doi: 10.1021/jo301793z
Dambatta, M. B., Polidano, K., Northey, A. D., Williams, J. M., and Morrill, L. C. (2019). Iron-catalyzed borrowing hydrogen C-alkylation of oxindoles with alcohols. ChemSusChem 12, 2345–2349. doi: 10.1002/cssc.201900799
Das, K., Mondal, A., and Srimani, D. (2018). Selective synthesis of 2-substituted and 1, 2-disubstituted benzimidazoles directly from aromatic diamines and alcohols catalyzed by molecularly defined nonphosphine manganese (I) complex. J. Org. Chem. 83, 9553–9560. doi: 10.1021/acs.joc.8b01316
Daw, P., Ben-David, Y., and Milstein, D. (2017). Direct synthesis of benzimidazoles by dehydrogenative coupling of aromatic diamines and alcohols catalyzed by cobalt. ACS Catal. 7, 7456–7460. doi: 10.1021/acscatal.7b02777
Fürstner, A. (2016). Iron catalysis in organic synthesis: a critical assessment of what it takes to make this base metal a multitasking champion. ACS Cent. Sci. 2, 778–789. doi: 10.1021/acscentsci.6b00272
Girish, Y. R., Kumar, K. S. S., Thimmaiah, K. N., Rangappa, K. S., and Shashikanth, S. (2015). ZrO 2-β-cyclodextrin catalyzed synthesis of 2, 4, 5-trisubstituted imidazoles and 1, 2-disubstituted benzimidazoles under solvent free conditions and evaluation of their antibacterial study. RSC Adv. 5, 75533–75546. doi: 10.1039/C5RA13891D
Göker, H., Karaaslan, C., Püsküllü, M. O., Yildiz, S., Duydu, Y., Üstündag, A., et al. (2016). Synthesis and in vitro activity of polyhalogenated 2-phenylbenzimidazoles as a new class of anti-MRSA and anti-VRE agents. Chem. Biol. Drug Des. 87, 57–68. doi: 10.1111/cbdd.12623
Gunanathan, C., and Milstein, D. (2013). Applications of acceptorless dehydrogenation and related transformations in chemical synthesis. Science 341:1229712. doi: 10.1126/science.1229712
Hille, T., Irrgang, T., and Kempe, R. (2014). The synthesis of benzimidazoles and quinoxalines from aromatic diamines and alcohols by iridium-catalyzed acceptorless dehydrogenative alkylation. Chem. A Eur. J. 20, 5569–5572. doi: 10.1002/chem.201400400
Ikariya, T., and Blacker, A. J. (2007). Asymmetric transfer hydrogenation of ketones with bifunctional transition metal-based molecular catalysts. Acc. Chem. Res. 40, 1300–1308. doi: 10.1021/ar700134q
Knölker, H. J., Baum, E., Goesmann, H., and Klauss, R. (1999). Demetalation of tricarbonyl (cyclopentadienone) iron complexes initiated by a ligand exchange reaction with NaOH-X-Ray analysis of a complex with nearly square-planar coordinated sodium. Angew. Chem. Int. Ed. 38, 2064–2066. doi: 10.1002/(SICI)1521-3773(19990712)38:13/14<2064::AID-ANIE2064>3.0.CO2-W
Li, L., Luo, Q., Cui, H., Li, R., Zhang, J., and Peng, T. (2018). Air-stable ruthenium (II)-NNN pincer complexes for the efficient coupling of aromatic diamines and alcohols to 1H-benzo[d] imidazoles with the liberation of H2. ChemCatChem 10, 1607–1613. doi: 10.1002/cctc.201800017
Martin, A. D., Siamaki, A. R., Belecki, K., and Gupton, B. F. (2015). A convergent approach to the total synthesis of telmisartan via a suzuki cross-coupling reaction between two functionalized benzimidazoles. J. Org. Chem. 80, 1915–1919. doi: 10.1021/jo5025333
Michlik, S., and Kempe, R. (2013). A sustainable catalytic pyrrole synthesis. Nat. Chem. 5, 140–144. doi: 10.1038/nchem.1547
Mori, T., Ishii, C., and Kimura, M. (2019). Pd-Catalyzed dehydrogenative oxidation of alcohols to functionalized molecules. Org. Process Res. Dev. 23, 1709–1717. doi: 10.1021/acs.oprd.9b00207
Morningstar, M. L., Roth, T., Farnsworth, D. W., Kroeger Smith, M., Watson, K., Buckheit, R. W., et al. (2007). Synthesis, biological activity, and crystal structure of potent nonnucleoside inhibitors of HIV-1 reverse transcriptase that retain activity against mutant forms of the enzyme. J. Med. Chem. 50, 4003–4015. doi: 10.1021/jm060103d
Moulin, S., Dentel, H., Pagnoux-Ozherelyeva, A., Gaillard, S., Poater, A., Cavallo, L., et al. (2013). Bifunctional (cyclopentadienone)iron-tricarbonyl complexes: synthesis, computational studies and application in reductive amination. Chem. A Eur. J. 19, 17881–17890. doi: 10.1002/chem.201302432
Mutti, F. G., Knaus, T., Scrutton, N. S., Breuer, M., and Turner, N. J. (2015). Conversion of alcohols to enantiopure amines through dual-enzyme hydrogen-borrowing cascades. Science 349, 1525–1529. doi: 10.1126/science.aac9283
Nakano, H., Inoue, T., Kawasaki, N., Miyataka, H., Matsumoto, H., Taguchi, T., et al. (2000). Synthesis and biological activities of novel anti-allergic agents with 5-lipoxygenase inhibiting action. Bioorg. Med. Chem. 8, 373–380. doi: 10.1016/S0968-0896(99)00291-6
Nanda, K. K., Henze, D. A., Della Penna, K., Desai, R., Leitl, M., Lemaire, W., et al. (2014). Benzimidazole CB2 agonists: design, synthesis and SAR. Bioorg. Med. Chem. Lett. 24, 1218–1221. doi: 10.1016/j.bmcl.2013.12.068
Nandakumar, A., Midya, S. P., Landge, V. G., and Balaraman, E. (2015). Transition-metal-catalyzed hydrogen-transfer annulations: access to heterocyclic scaffolds. Angew. Chem. Int. Ed. 54, 11022–11034. doi: 10.1002/anie.201503247
Özden, S., Atabey, D., Yildiz, S., and Göker, H. (2005). Synthesis and potent antimicrobial activity of some novel methyl or ethyl 1H-benzimidazole-5-carboxylates derivatives carrying amide or amidine groups. Bioorg. Med. Chem. 13, 1587–1597. doi: 10.1016/j.bmc.2004.12.025
Ramla, M. M., Omar, M. A., Tokuda, H., and El-Diwani, H. I. (2007). Synthesis and inhibitory activity of new benzimidazole derivatives against burkitt's lymphoma promotion. Bioorg. Med. Chem. 15, 6489–6496. doi: 10.1016/j.bmc.2007.04.010
Reed-Berendt, B. G., Polidano, K., and Morrill, L. C. (2019). Recent advances in homogeneous borrowing hydrogen catalysis using earth-abundant first row transition metals. Org. Biomol. Chem. 17, 1595–1607. doi: 10.1039/C8OB01895B
Sharma, A. K., Joshi, H., Bhaskar, R., and Singh, A. K. (2017). Complexes of (η 5-Cp*) Ir (iii) with 1-benzyl-3-phenylthio/selenomethyl-1, 3-dihydrobenzoimidazole-2-thione/selenone: catalyst for oxidation and 1, 2-substituted benzimidazole synthesis. Dalton Trans. 46, 2228–2237. doi: 10.1039/C6DT04271F
Sharma, H., Kaur, N., Singh, N., and Jang, D. O. (2015). Synergetic catalytic effect of ionic liquids and ZnO nanoparticles on the selective synthesis of 1, 2-disubstituted benzimidazoles using a ball-milling technique. Green Chem. 17, 4263–4270. doi: 10.1039/C5GC00536A
Smith, J. M., and Krchnák, V. (1999). A solid phase traceless synthesis of benzimidazoles with three combinatorial steps. Tetrahedron Lett. 40, 7633–7636. doi: 10.1016/S0040-4039(99)01560-9
Suk, F. M., Liu, C. L., Hsu, M. H., Chuang, Y. T., Wang, J. P., and Liao, Y. J. (2019). Treatment with a new benzimidazole derivative bearing a pyrrolidine side chain overcomes sorafenib resistance in hepatocellular carcinoma. Sci. Rep. 9:17259. doi: 10.1038/s41598-019-53863-2
Takeuchi, K., Bastian, J. A., Gifford-Moore, D. S., Harper, R. W., Miller, S. C., Mullaney, J. T., et al. (2000). 1,2-disubstituted indole, azaindole and benzimidazole derivatives possessing amine moiety: a novel series of thrombin inhibitors. Bioorg. Med. Chem. Lett. 10, 2347–2351. doi: 10.1016/S0960-894X(00)00454-6
Wang, B. B., and Smith, P. J. (2003). Synthesis of a terbenzimidazole topoisomerase I poison via iterative borinate ester couplings. Tetrahedron Lett. 44, 8967–8969. doi: 10.1016/j.tetlet.2003.10.007
Watson, C., Owen, D. R., Harding, D., Kon, K., Lewis, M. L., Mason, H. J., et al. (2011). Optimisation of a novel series of selective CNS penetrant CB2 agonists. Bioorg. Med. Chem. Lett. 21, 4284–4287. doi: 10.1016/j.bmcl.2011.05.063
Xu, Z., Wang, D. S., Yu, X., Yang, Y., and Wang, D. (2017). Tunable triazole-phosphine-copper catalysts for the synthesis of 2-Aryl-1H-benzo[d]imidazoles from benzyl alcohols and diamines by acceptorless dehydrogenation and borrowing hydrogen reactions. Adv. Synth. Catal. 359, 3332–3340. doi: 10.1002/adsc.201700179
Xu, Z., Yu, X., Sang, X., and Wang, D. (2018). BINAP-copper supported by hydrotalcite as an efficient catalyst for the borrowing hydrogen reaction and dehydrogenation cyclization under water or solvent-free conditions. Green Chem. 20, 2571–2577. doi: 10.1039/C8GC00557E
Yan, T., Feringa, B. L., and Barta, K. (2014). Iron catalysed direct alkylation of amines with alcohols. Nat. Commun. 5:5602. doi: 10.1038/ncomms6602
Yang, Q., Wang, Q., and Yu, Z. (2015). Substitution of alcohols by N-nucleophiles via transition metal-catalyzed dehydrogenation. Chem. Soc. Rev. 44, 2305–2329. doi: 10.1039/C4CS00496E
Zhang, C., Hu, B., Chen, D., and Xia, H. (2019). Manganese (I)-catalyzed transfer hydrogenation and acceptorless dehydrogenative condensation: promotional influence of the uncoordinated N-heterocycle. Organometallics 38, 3218–3226. doi: 10.1021/acs.organomet.9b00475
Zimmermann, G., Papke, B., Ismail, S., Vartak, N., Chandra, A., Hoffmann, M., et al. (2013). Small molecule inhibition of the KRAS-PDEδ interaction impairs oncogenic KRAS signalling. Nature 497, 638–642. doi: 10.1038/nature12205
Keywords: iron catalysis, dehydrogenative coupling, borrowing hydrogen, alcohol, benzimidazoles
Citation: Putta RR, Chun S, Lee SB, Oh D-C and Hong S (2020) Iron-Catalyzed Acceptorless Dehydrogenative Coupling of Alcohols With Aromatic Diamines: Selective Synthesis of 1,2-Disubstituted Benzimidazoles. Front. Chem. 8:429. doi: 10.3389/fchem.2020.00429
Received: 20 March 2020; Accepted: 23 April 2020;
Published: 19 June 2020.
Edited by:
Nam-Jung Kim, Kyung Hee University, South KoreaReviewed by:
In Su Kim, Sungkyunkwan University, South KoreaCopyright © 2020 Putta, Chun, Lee, Oh and Hong. This is an open-access article distributed under the terms of the Creative Commons Attribution License (CC BY). The use, distribution or reproduction in other forums is permitted, provided the original author(s) and the copyright owner(s) are credited and that the original publication in this journal is cited, in accordance with accepted academic practice. No use, distribution or reproduction is permitted which does not comply with these terms.
*Correspondence: Suckchang Hong, c2Nob25nMTdAc251LmFjLmty
Disclaimer: All claims expressed in this article are solely those of the authors and do not necessarily represent those of their affiliated organizations, or those of the publisher, the editors and the reviewers. Any product that may be evaluated in this article or claim that may be made by its manufacturer is not guaranteed or endorsed by the publisher.
Research integrity at Frontiers
Learn more about the work of our research integrity team to safeguard the quality of each article we publish.