- 1Key Laboratory of New Processing Technology for Nonferrous Metal & Materials, Guilin University of Technology, Ministry of Education, Guilin, China
- 2Institute of Industrial Science, The University of Tokyo, Tokyo, Japan
A novel fluorescence chemosensor array composed of pyrenylboronic acid-based probes for multi- anion detection has been developed. The pyrenylboronic acid derivatives showed fluorescence quenching or enhancement due to photoinduced electron transfer originating from anion binding. The recognition ability was assessed by fluorescence titrations and electrospray ionization mass spectrometry. Because the array is constructed with cross-reactive probes, the combination of differential binding affinities for anions (i.e., fluoride, acetate, oxalate, malonate, citrate, dihydrogen phosphate, and pyrophosphate) and pattern recognitions, such as linear discriminant analysis, offered a successful simultaneous anion detection with a classification rate of 100%. Furthermore, the chemosensor array allowed for quantitative prediction of oxalate, malonate, and citrate in mixtures using a support vector machine. Importantly, the array system employs low-cost and commercially available reagents as probes. Thus, this study could lead to the development of user-friendly and high-throughput methods to detect a variety of analytes in complicated systems.
Introduction
During the past decades, great efforts have been devoted to anion detection, because anions are essential to life and play important roles in industrial, environmental, and chemical processes (Schmidtchen and Berger, 1997; Gunnlaugsson et al., 2005; Gale and Dehaen, 2010). Although the methods for anion detection, such as mass spectrometry, have already been established (Berthod et al., 2018), use of large equipment is a potential drawback for on-site analysis. Furthermore, the diverse chemical and physical properties of anions limit their easy detection (Gómez et al., 2005; Mahoney et al., 2005; Sessler et al., 2006; Li et al., 2012; Chang et al., 2014; Edwards et al., 2015). Of all the methods for anion detection, we believe that the most powerful technique is chemosensing due to its advantages such as high sensitivity and applicability for rapid and high-throughput detection. Hence, a significant number of chemosensors have been developed for anion recognition via various capturing mechanisms (Amendola et al., 2010; Cavallo et al., 2010; Xu et al., 2010; Nishimuta et al., 2013; You et al., 2015; Mako et al., 2019). Among them, boronic acid derivatives have been widely utilized based on their reversible nature of binding with anions (Reetz et al., 1991; Cooper et al., 1998; Yamaguchi et al., 2001; Kubo et al., 2003, 2006; Koskela et al., 2005; Liu et al., 2005; Galbraith and James, 2010; Rout et al., 2012; Minami et al., 2016). In particular, the Lewis acidity allows boron-based chemosensors to act as a receptor for Lewis basic anions such as fluoride and phosphate (Wade et al., 2010; Wu X. et al., 2017). However, to the best of our knowledge, general methods to distinguish the multiple anions simultaneously using simple boronic acid-based chemosensors have not been established owing to the complex interference among analytes.
Chemosensor arrays constructed by multiple sensing units offer multi-target detection by utilizing crosstalk between sensors and analytes. The cross-reactivity in the array system provides a lot of advantages for practical applications such as reduction of cost and time of analysis. Therefore, chemosenor arrays were actively considered as those with the best methods to solve the above-mentioned challenge (Lavigne and Anslyn, 2001; Liu and Bonizzoni, 2014; Li et al., 2019). For example, Yamaguchi et al. synthesized a series of Zn(II)-dipicolylamine complexes covalently linked to anthracene for the detection of phosphate anions (Yamaguchi et al., 2005). Although these chemosensors were well designed according to the target analytes, their complicated structures may limit the practical applications of chemosensor arrays. Thus, it is of great importance and interest to develop chemosensor arrays using only simple materials. We previously reported indicator displacement assays utilizing only commercially available compounds, which realized the efficient discrimination of metal ions (Sasaki et al., 2017), saccharides (Sasaki et al., 2019a,b) and oxyanions (Hamedpour et al., 2019). Herein, we have employed a new combination of commercially available reagents to construct the chemosensor array in which the components are more simplified (Figure 1). Pyrenylboronic acid derivatives [1-Pyrenylboronic acid (1) and 4-(1-pyrenyl)phenylboronic acid (2)] were employed as probes because of their very simple chemical structures for fluorescence response (Wu D. et al., 2017). The simultaneous discrimination of seven anions was achieved by monitoring the fluorescence changes due to photoinduced electron transfer (PeT) (de Silva et al., 1997; Duke et al., 2010) originating from anion recognition. In addition, a quantitative analysis resulted in successful prediction of the concentrations of oxyanions (oxalate, citrate, and malonate) in the mixture.
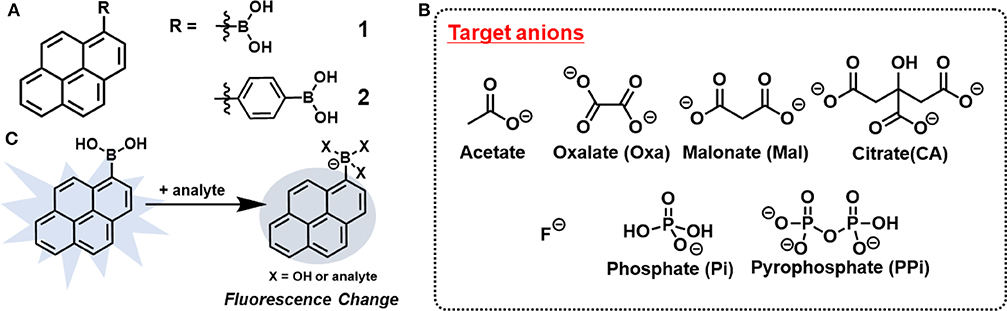
Figure 1. Chemical structures of (A) probes and (B) target anions in this study. (C) Schematic illustration for mechanism of anion recognition by 1.
Materials and Methods
Materials
The materials were purchased from commercial suppliers and used without further purification. The probes 1, 2, citric acid, and aqueous solution of tetra-n-butylammonium hydroxide (40% w/w) were purchased from Tokyo Chemical Industry Co. Ltd (Tokyo, Japan). Tetra-n-butylammonium salts of fluoride (F−), chloride (Cl−), bromide (Br−), iodide (I−), acetate, dihydrogen phosphate (Pi), and pyrophosphate (PPi) were purchased from Sigma-Aldrich (St. Louis, MO, USA). Oxalic acid and malonic acid were purchased from FUJIFILM WAKO Chemicals Corp (Tokyo, Japan). The general procedure for the synthesis of tetra-n-butylammonium salts of oxalate, citrate and malonate was as follows: 5 mL of an aqueous solution of tetra-n-butylammonium hydroxide (40% w/w) was mixed with oxalic acid, citric acid or malonic acid in a 20 mL glass bottle. The pH value of the solution was neutralized by adding the acids, followed by vacuum drying at 50°C.
Measurements
Fluorescence spectra were recorded on a HITACHI F-7100 spectrophotometer. Fluorescence titrations with anions were performed in DMSO, and the titration isotherms were obtained from changes in the fluorescence maxima (378 and 401 nm for 1 and 2, respectively). The fluorescence titration was carried out under ambient conditions at 25°C. The binding constants were determined by fitting the fluorescence titration curves according to previous studies (Hargrove et al., 2010). Mass spectrometry was performed using a SHIMADZU LCMS-2020 spectrometer.
The array experiment for qualitative and quantitative analyses was performed by a Biotek SYNERGY H4 microplate reader. The details of the processes are as follows: the analyte was added to the probe solution at a certain concentration, and then the resulting mixing solution was shaken and allowed to stand for 30 min. After this period, the solution was transferred to the 96-well microplates and fluorescence spectra were recorded from 350 to 550 nm. The fluorescence measurements were repeated 24 times. A class of 20 repetitions with a variability coefficient lower than 9% was extracted by the Student's t-test. Then, the qualitative and semi-quantitative analyses were carried out using a linear discriminant analysis (LDA) without any further pretreatment (Brereton, 2007; Anzenbacher et al., 2010). A support vector machine with a principal component analysis preprocessing (PCs = 3) was used for the analysis of quantitative assay (Hamel, 2009).
Results and Discussion
To examine the binding of the boronic acid-based probes (1 and 2) with various anions, the fluorescence titrations were carried out at 25°C. The spectra are shown in Figures 2A,B and Supplementary Materials. The fluorescence spectra of probe 2 were broader than those of probe 1. 1-Aryl substituted pyrene derivatives reported a loss of vibrational structure in UV-vis absorption and fluorescence properties (Weigel et al., 2003), meaning that separation of S2 ← S0 and S1 ← S0 excitations is almost impossible. All orbitals including the above excitations possess nonzero contributions at the 1-position, resulting in the significant influence on both transitions (Crawford et al., 2011). The probe 1 exhibited fluorescence increase and decrease depending on the types of analytes tested. The fluorescence increase was observed in the presence of acetate, Pi, and PPi, while the other types of anions (oxalate, citrate, malonate, and fluoride) resulted in fluorescence decrease. In case of probe 2, the addition of any type of anions increased the fluorescence intensity. The fluorescence ON-OFF and OFF-ON features are presumably due to the PeT between fluorophore and the boron atom (Yoon and Czarnik, 1992; Sandanayake et al., 1995; Sun et al., 2019), which may be affected by the difference of the electronic delocalization. To address the mechanism of the observed PeT phenomenon, we measured UV-Vis spectra of the probes in the presence or absence of citrate as an example of the target anions. The probe 2 showed no UV-Vis spectral change, which supports the PeT mechanism (de Silva et al., 1997). On the other hand, slight UV-Vis and fluorescence spectral shifts were observed for the probe 1, indicating that a weak intramolecular charge transfer would co-occur with the PeT (see Supplementary Material) (Hagopian and Singer, 1985; de Silva et al., 1997; Schneider et al., 2001; Oesch and Luedtke, 2015; Sasaki et al., 2016). Among the targets, the highest response to anions was citrate. Probes 1 and 2 showed 76% of an on-off response (i.e., Isat./I0) and 162% of an off-on response, respectively. On the other hand, the probes showed very weak or no response to fructose which can potentially interfere the boronic acid-based anion detection (see Supplementary Material). In addition, the formation of the boronate species was also proven by electrospray ionization mass spectrometry (ESI-MS) (Figures 2C,D and Supplementary Material).
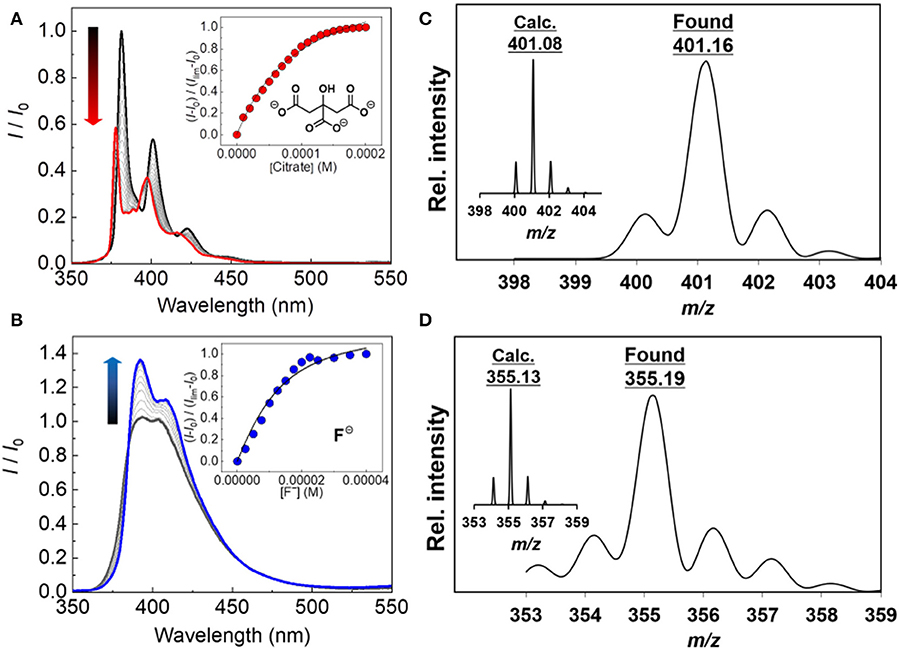
Figure 2. Fluorescence spectra of (A) 1 in DMSO upon addition of citrate at 25°C (0–200 μM) and (B) 2 in DMSO upon addition of fluoride at 25°C (0–40 μM). [1] = [2] =10 μM, λex = 340 nm for 1 and 2. ESI-MS spectrum (negative) of (C) the 1–citrate complex (inset: calculated isotope pattern for C22H14), and (D) the 2–fluoride complex (inset: calculated isotope pattern for C23H17).
The stoichiometries for binding (i.e., anion-to-probe ratio) were estimated to be 1:1 except for Pi and PPi. From the fluorescence titration isotherms, the binding constants (Ks) of the probes for anions were determined (Table 1). It is worth noting that the probes displayed different affinities for the anions, which resulted in a unique response of the probes to various guests. It is well known that the cross-reactivity between the probe and analyte is crucial for the classification rate of an array. Therefore, we envisioned that the fluorescent properties of the probe 1 and 2 contribute to the development of a new chemosensor array to discriminate multiple anions qualitatively and quantitatively.
Among the pattern recognition algorithms, a linear discriminant analysis (LDA) (Brereton, 2007) is one of the suitable supervised methods to test our hypothesis. We employed the LDA to reduce the dimensionality and to realize multivariate data classification. Additionally, the leave-one-out cross-validation protocol (namely the Jackknife method) was employed to evaluate the level of correct classification within the clusters (see Supplementary Material). To ensure the accuracy, the trials were repeated 24 times for each set of analytes, and then four outliers were eliminated by the Student's t-test. As shown in Figure 3, seven types of analytes and controls (160 data points) were clearly discriminated with a successful rate of 100%. This result proves our expectation that the new chemosensor array constructed of probe 1 and 2 possesses excellent capability for multiple anions detection.
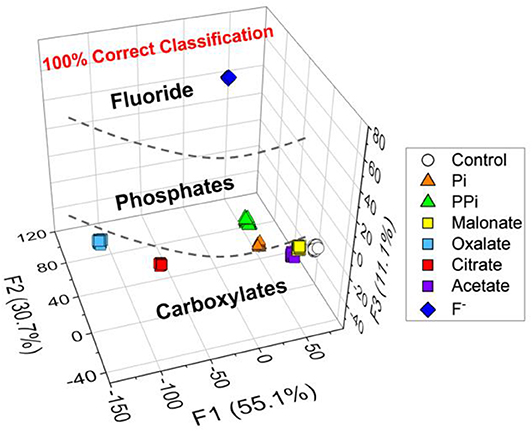
Figure 3. LDA plots for the response of 8 types of clusters (including a control sample). [Anion] = 200 μM. Twenty.repetitions were measured for each and the cross-validation routine shows 100% successful classification. The data were obtained by using the probes 1 and 2.
Taking advantage of LDA, the semi-quantitative assay was carried out to evaluate the capability of the array system for quantitative anion recognition. The analysis of the oxyanions (oxalate, citrate, and malonate) is shown in Figure 4. As can be seen, the LDA plots were clearly dependent not only on the concentration of anions but also the type, which is completely consistent with the results of the fluorescence titrations. This result encouraged us to employ the array for regression analysis of the anions in mixtures.
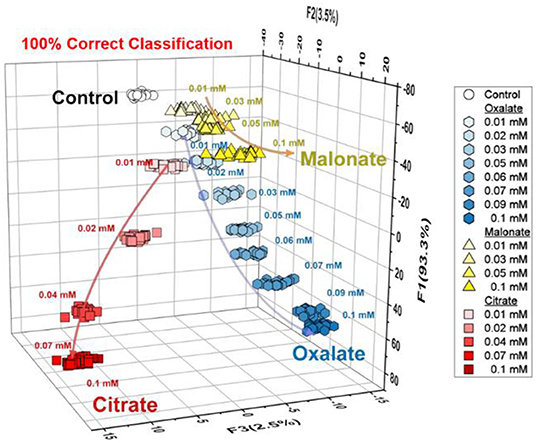
Figure 4. Semi-quantitative LDA plot for three types of anions (citrate, oxalate and malonate) at a concentration range of 0–100 μM. For each concentration, the trial was repeated 24 times. The data were obtained by using the probes 1 and 2.
Figure 5 shows the results of the quantitative array for a mixture of the same concentrations of oxalate, citrate, and malonate. For the regression analysis, a support vector machine (SVM) algorithm, which is suitable for complex nonlinear responses, was employed. Here, we used nine analyte concentrations for model construction and two different analyte concentrations for prediction. Consequently, the unknown concentrations were successfully predicted by the constructed model with excellent root-mean-square errors (RMSE values). This indicated that the developed array can predate the concentration of oxalate, citrate, and malonate even in mixtures.
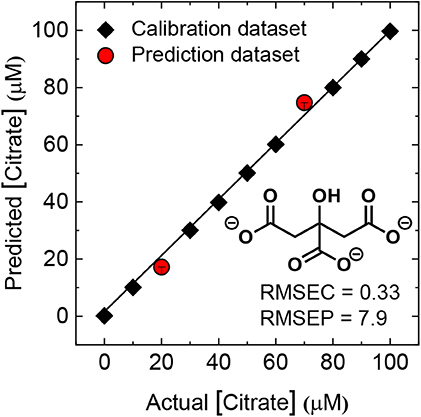
Figure 5. The results of the regression analysis for quantitative analysis of citrate in mixtures of oxyanions (oxalate, citrate, and malonate). The data were obtained by using the probes 1 and 2. The detailed concentration conditions are summarized in Supplementary Material. Plots of actual vs. predicted concentrations show high accuracy of prediction for multiple concentrations of each analyte. The values of root-mean-square error (RMSE) of calibration (C) and prediction (P) prove the high quality of the model and prediction.
Conclusion
In summary, a pyrenylboronic acid-based chemosensor array was constructed through a combination of simple commercially available reagents. The proposed array exhibited an excellent capability for discriminating between multiple anions in a qualitative as well as quantitative manner. In the qualitative analysis, seven types of anions were successfully discriminated by employing LDA with 100% correct classification. Moreover, the semi-quantitative assay for oxalate, citrate, and malonate showed species-dependent and concentration-dependent changes in the LDA plots. Thus, the regression analysis was performed and the concentration of individual components was successfully predicted in the mixture. To the best of our knowledge, fabrication of a boronic acid-based chemosensor array with the ability to discriminate between several anions has not previously been reported. Thus, the simple chemosensor array proposed in this study will provide a new platform for the application of chemosensors in the field of molecular recognition chemistry.
Data Availability Statement
All datasets generated for this study are included in the article/Supplementary Material.
Author Contributions
ZC, YC, RK, YS, KA, and XL performed the fluorescence and mass spectrometry experiments, and wrote the manuscript. ZZ, QZ, XZ, XX, and SW contributed equally in this work. TM and YL conceived the entire project.
Funding
This research was funded by the Natural Science Foundation of Guangxi (Grant No. 2018GXNSFAA138131), Key Laboratory of New Processing Technology for Nonferrous Metal & Materials, Guilin University of Technology, Ministry of Education (Grant No. 19AA-6), and Foundation of Guilin University of Technology (Grant No. GUTQDJJ2017108). TM thanks Japan Society for the Promotion of Science (JSPS), Grant-in-Aid for Scientific Research, No. 17H04882. YS also thanks the JSPS, Research Fellow for Young Scientists (DC1), No. 18J21190.
Conflict of Interest
The authors declare that the research was conducted in the absence of any commercial or financial relationships that could be construed as a potential conflict of interest.
Supplementary Material
The Supplementary Material for this article can be found online at: https://www.frontiersin.org/articles/10.3389/fchem.2020.00414/full#supplementary-material
References
Amendola, V., Fabbrizzi, L., and Mosca, L. (2010). Anion recognition by hydrogen bonding: urea-based receptors. Chem. Soc. Rev. 39, 3889–3915. doi: 10.1039/B822552B
Anzenbacher, P. Jr., Lubal, P., Bucek, P., Palacios, M. A., and Kozelkova, M. E. (2010). A practical approach to optical cross-reactive sensor arrays. Chem. Soc. Rev. 39, 3954–3979. doi: 10.1039/B926220M
Berthod, A., Ruiz-Ángel, M. J., and Carda-Broch, S. (2018). Recent advances on ionic liquid uses in separation techniques. J. Chromatogr. A. 1559, 2–16. doi: 10.1016/j.chroma.2017.09.044
Cavallo, G., Metrangolo, P., Pilati, T., Resnati, G., Sansotera, M., and Terraneo, G. (2010). Halogen bonding: a general route in anion recognition and coordination. Chem. Soc. Rev. 2010, 39, 3772–3783. doi: 10.1039/B926232F
Chang, K.-C., Minami, T., Koutnik, P, Savechenkov, P. Y., Liu, Y., and Anzenbacher, P. Jr. (2014). Anion binding modes in meso-substituted hexapyrrolic Calix[4]pyrrole isomers. J. Am. Chem. Soc. 136, 1520–1525. doi: 10.1021/ja411391c
Cooper, C. R., Spencer, N., and James, T. D. (1998). Selective fluorescence detection of fluoride using boronic acids. Chem. Commun. 1365–1366. doi: 10.1039/A801693C
Crawford, A. G., Dwyer, A. D., Liu, Z., Stephen, A., Beeby, A., Pålsson, L.-O., et al. (2011). Experimental and theoretical studies of the photophysical properties of 2- and 2,7-functionalized pyrene derivatives. J. Am. Chem. Soc. 133, 13349–13362. doi: 10.1021/ja2006862
de Silva, A. P., Gunaratne, H. Q. N., Gunnlaugsson, T., Huxley, A. J. M., McCoyy, C. P., Rademacher, J. T., et al. (1997). Signaling recognition events with fluorescent sensors and switches. Chem. Rev. 97, 1515–1566. doi: 10.1021/cr960386p
Duke, R. M., Veale, E. B., Pfeffer, F. M., Kruger, P. E., and Gunnlaugsson, T. (2010). Colorimetric and fluorescent anion sensors: an overview of recent developments in the use of 1,8-naphthalimide-based chemosensors. Chem. Soc. Rev. 39, 3936–3953. doi: 10.1039/B910560N
Edwards, S. J., Valkenier, H., Busschaert, N., Gale, P. A., and Davis, A. P. (2015). High-affinity anion binding by steroidal squaramide receptors. Angew. Chem. Int. Ed. 54, 4592–4596. doi: 10.1002/anie.201411805
Galbraith, E., and James, T. D. (2010). Boron based anion receptors as sensors. Chem. Soc. Rev. 39, 3831–3842. doi: 10.1039/b926165f
Gale, P. A., and Dehaen, W. (2010). Anion Recognition in Supramolecular Chemistry (Topics in Heterocyclic Chemistry). Springer.
Gómez, D. E., Fabbrizzi, L., Licchelli, M., and Monzani, E. (2005). Urea vs. thiourea in anion recognition. Org. Biomol. Chem. 3, 1495–1500. doi: 10.1039/B500123D
Gunnlaugsson, T., Ali, H. D. P., Glynn, M., Kruger, P. E., Hussey, G. M., Pfeffer, F. M., et al. (2005). Fluorescent photoinduced electron transfer (PET) sensors for anions; from design to potential application. J. Fluoresc. 15, 287–299. doi: 10.1007/s10895-005-2627-y
Hagopian, S., and Singer, L. A. (1985). Photophysical studies on 1-(p-aminophenyl)pyrene. characterization of an intramolecular charge-transfer state with application to proton-transfer dynamics. J. Am. Chem. Soc. 107, 1874–1880. doi: 10.1021/ja00293a012
Hamedpour, V., Sasaki, Y., Zhang, Z., Kubota, R., and Minami, T. (2019). Simple colorimetric chemosensor array for oxyanions: quantitative assay for herbicide glyphosate. Anal. Chem. 91, 13627–13632. doi: 10.1021/acs.analchem.9b02822
Hamel, L. H. (2009). Knowledge Discovery with Support Vector Machines. (Hoboken, NJ: John Wiley and Sons, Ltd).
Hargrove, A. E., Zhong, Z., Sessler, J. L., and Anslyn, E. V. (2010). Algorithms for the determination of binding constants and enantiomeric excess in complex host: guest equilibria using optical measurements. New J. Chem. 34, 348–354. doi: 10.1039/b9nj00498j
Koskela, S. J. M., Fyles, T. M., and James, T. D. (2005). A ditopic fluorescent sensor for potassium fluoride. Chem. Commun. 945–947. doi: 10.1039/b415522j
Kubo, Y., Ishida, T., Minami, T., and James, T. D. (2006). Highly selective fluoride ion detection based on a fluorescent alizarin-o-aminomethylphenylboronic acid ensemble in aqueous MeOH solution. Chem. Lett. 35, 996–997. doi: 10.1246/cl.2006.996
Kubo, Y., Yamamoto, M., Ikeda, M., Takeuchi, M., Shinkai, S., Yamaguchi, S., et al. (2003). A colorimetric and ratiometric fluorescent chemosensor with three emission changes: fluoride ion sensing by a triarylborane– porphyrin conjugate. Angew. Chem. Int. Ed. Engl. 42, 2036–2040. doi: 10.1002/anie.200250788
Lavigne, J. J., and Anslyn, E. V. (2001). Sensing a paradigm shift in the field of molecular recognition: from selective to differential receptors. Angew. Chem. Int. Ed. 40, 3118–3130. doi: 10.1002/1521-3773(20010903)40:17<3118::AID-ANIE3118>3.0.CO;2-Y
Li, Y.-J., Xu, L., Yang, W.-L., Liu, H.-B., Lai, S.-W., Che, C.-M., et al. (2012). Amidetriazole: a versatile building block for construction of oxyanion anion receptors. Chem. Eur. J. 18, 4782–4790. doi: 10.1002/chem.201102760
Li, Z., Askim, J. R., and Suslick, K. S. (2019). The optoelectronic nose: colorimetric and fluorometric sensor arrays. Chem. Rev. 119, 231–292. doi: 10.1021/acs.chemrev.8b00226
Liu, Y., and Bonizzoni, M. (2014). A supramolecular sensing array for qualitative and quantitative analysis of organophosphates in water. J. Am. Chem. Soc. 136, 14223–14229. doi: 10.1021/ja507905r
Liu, Z.-Q., Shi, M., Li, F.-Y., Fang, Q., Chen, Z.-H., Yi, T., et al. (2005). Highly selective two-photon chemosensors for fluoride derived from organic boranes. Org. Lett. 7, 5481–5484. doi: 10.1021/ol052323b
Mahoney, J. M., Stucker, K. A., Jiang, H., Carmichael, I., Brinkmann, N. R., Beatty, A. M., et al. (2005). Molecular recognition of trigonal oxyanions using a ditopic salt receptor: evidence for anisotropic shielding surface around nitrate anion. J. Am. Chem. Soc. 127, 2922–2928. doi: 10.1021/ja0440295
Mako, T. N., Racicot, J. M., and Levine, M. (2019). Supramolecular luminescent sensors. Chem. Rev. 119, 322–477. doi: 10.1021/acs.chemrev.8b00260
Minami, T., Emami, F., Nishiyabu, R., Kubo, Y., and Anzenbacher, P. Jr. (2016). Quantitative analysis of modeled ATP hydrolysis in water by a colorimetric sensor array. Chem. Commun. 52, 7838–7841. doi: 10.1039/C6CC02923J
Nishimuta, T., Xu, S.-Y., Jiang, Y.-B., Fossey, J. S., Sakurai, K., Bull, S. D., et al. (2013). A simple visual sensor with the potential for determining the concentration of fluoride in water at environmentally significant levels. Chem. Commun. 49, 478–480. doi: 10.1039/C2CC36107H
Oesch, D., and Luedtke, N. W. (2015). Fluorescent chemosensors of carbohydrate triols exhibiting TICT emissions. Chem. Commun., 51, 12641–12644. doi: 10.1039/C5CC03857J
Reetz, M. T., Miemeyer, C. M., and Harms, K. (1991). Crown ethers with a lewis acidic center: a new class of heterotopic host molecules. Angew. Chem. Int. Ed. Engl. 30, 1472–1474. doi: 10.1002/anie.199114721
Rout, B., Unger, L., Armony, G., Iron, M. A., and Margulies, D. (2012). Medication detection by a combinatorial fluorescent molecular sensor. Angew. Chem. Int. Ed. 124, 12645–12649. doi: 10.1002/ange.201206374
Sandanayake, K. R. A. S., James, T. D., and Shinkai, S. (1995). Two dimensional photoinduced electron transfer (PET) fluorescence sensor for saccharides. Chem. Lett. 7, 503–504. doi: 10.1246/cl.1995.503
Sasaki, S., Drummen, G. P. C., and Konishi, G.-I. (2016). Recent advances in twisted intramolecular charge transfer (TICT) fluorescence and related phenomena in materials chemistry. J. Mater. Chem. C 4, 2731–2743. doi: 10.1039/C5TC03933A
Sasaki, Y., Hamedpour, V., Kubota, R., He, Y., Torii, Y., and Minami, T. (2019a). Facile indicator displacement assay-based supramolecular chemosensor: quantitative colorimetric determination of xylose and glucose in the presence of ascorbic acid. Chem. Lett. 48, 1368–1370. doi: 10.1246/cl.190570
Sasaki, Y., Minamiki, T., Tokito, S., and Minami, T. (2017). A molecular self-assembled colourimetric chemosensor array for simultaneous detection of metal ions in water. Chem. Commun. 53, 6561–6564. doi: 10.1039/c7cc03218h
Sasaki, Y., Zhang, Z., and Minami, T. (2019b). A saccharide chemosensor array developed based on an indicator displacement assay using a combination of commercially available reagents. Front. Chem. 7:49. doi: 10.3389/fchem.2019.00049
Schmidtchen, F. P., and Berger, M. (1997). Artificial organic host molecules for anions. Chem. Rev. 97, 1609–1646. doi: 10.1021/cr9603845
Schneider, S., Kurzawa, J., Stockmann, A., Engl, R., Daub, J., Matousek, P., et al. (2001). Photoinduced electron transfer in phenothiazine and pyrene based dyades studied by picosecond time-gated Raman spectroscopy. Chem. Phys. Lett. 348, 277–284. doi: 10.1016/S0009-2614(01)01134-4
Sessler, J. L., Gale, P. A., and Cho, W.-S. (2006). “Monographs in supramolecular Chemistry,” in Anion Receptor Chemistry (Cambridge: Royal Society of Chemistry).
Sun, X., Chapin, B. M., Metola, P., Collins, B., Wang, B., James, T. D., et al. (2019). The mechanisms of boronate ester formation and fluorescent turn-on in ortho-aminomethylphenylboronic acids. Nat. Chem. 11, 768–778. doi: 10.1038/s41557-019-0314-x
Wade, C. R., Broomsgrove, A. E. J., Aldridge, S., and Gabbai, F. P. (2010). Fluoride ion complexation and sensing using organoboron compounds. Chem. Rev. 110, 3958–3984. doi: 10.1021/cr900401a
Weigel, W., Rettig, W., Dekhtyar, M., Modrakowski, C., Beinhoff, M., and Schlüter, A. D. (2003). Dual fluorescence of phenyl and biphenyl substituted pyrene derivatives. J. Phys. Chem. A 107, 5941–5947. doi: 10.1021/jp026116u
Wu, D., Sedgwick, A. C., Gunnlaugsson, T., Akkaya, E. U., and Yoon, J.;, James, T. D. (2017). Fluorescent chemosensors: the past, present and future. Chem. Soc. Rev. 46, 7105–7123. doi: 10.1039/C7CS00240H
Wu, X., Chen, X., and Jiang, Y. (2017). Recent advances in boronic acid-based optical chemosensors. Analyst. 142, 1403–1414. doi: 10.1039/c7an00439g
Xu, Z., Kim, S. K., and Yoon, J. (2010). Revisit to imidazolium receptors for the recognition of anions: highlighted research during 2006–2009. Chem. Soc. Rev. 39, 1457–1466. doi: 10.1039/B918937H
Yamaguchi, S., Akiyama, S., and Tamao, K. (2001). Colorimetric fluoride ion sensing by boron-containing π-electron systems. J. Am. Chem. Soc. 123, 11372–11375. doi: 10.1021/ja015957w
Yamaguchi, S., Yoshimura, I., Kohira, T., Tamaru, S., and Hamachi, I. (2005). Cooperation between artificial receptors and supramolecular hydrogels for sensing and discriminating phosphate derivatives. J. Am. Chem. Soc. 127, 11835–11841. doi: 10.1021/ja052838y
Yoon, J., and Crarnik, A. W. (1992). Fluorescent chemosensors of carbohydrates. A means of chemically communicating the binding of polyols in water based on chelation-enhanced quenching. J. Am. Chem. Soc. 114, 5874–5875. doi: 10.1021/ja00040a067
Keywords: anion, chemosensor array, boronic acid, fluorescence, regression analysis
Citation: Cao Z, Cao Y, Kubota R, Sasaki Y, Asano K, Lyu X, Zhang Z, Zhou Q, Zhao X, Xu X, Wu S, Minami T and Liu Y (2020) Fluorescence Anion Chemosensor Array Based on Pyrenylboronic Acid. Front. Chem. 8:414. doi: 10.3389/fchem.2020.00414
Received: 27 February 2020; Accepted: 21 April 2020;
Published: 28 May 2020.
Edited by:
Tony D. James, University of Bath, United KingdomReviewed by:
Shaomin Ji, Guangdong University of Technology, ChinaDavid C. Magri, University of Malta, Malta
Copyright © 2020 Cao, Cao, Kubota, Sasaki, Asano, Lyu, Zhang, Zhou, Zhao, Xu, Wu, Minami and Liu. This is an open-access article distributed under the terms of the Creative Commons Attribution License (CC BY). The use, distribution or reproduction in other forums is permitted, provided the original author(s) and the copyright owner(s) are credited and that the original publication in this journal is cited, in accordance with accepted academic practice. No use, distribution or reproduction is permitted which does not comply with these terms.
*Correspondence: Tsuyoshi Minami, tminami@iis.u-tokyo.ac.jp; Yuanli Liu, lyuanli@glut.edu.cn
†These authors have contributed equally to this work