- 1Key Laboratory of Pesticide and Chemical Biology of Ministry of Education, Hubei International Scientific and Technological Cooperation Base of Pesticide and Green Synthesis, International Joint Research Center for Intelligent Biosensing Technology and Health, College of Chemistry, Central China Normal University, Wuhan, China
- 2Jiangxi Key Laboratory for Mass Spectrometry and Instrumentation, East China University of Technology, Nanchang, China
- 3Hubei Key Laboratory of Cell Homeostasis, Hubei Province Key Laboratory of Allergy and Immunology, Key Laboratory of Combinatorial Biosynthesis and Drug Discovery, College of Life Sciences, Ministry of Education, Wuhan University, Wuhan, China
Chemical synthesis is an attractive approach allows for the assembly of homogeneous complex N-linked glycopeptides and glycoproteins, but the limited coupling efficiency between glycans and peptides hampered the synthesis and research in the related field. Herein we developed an alternative glycosylation to construct N-linked glycopeptide via efficient selenoester-assisted aminolysis, which employs the peptidyl ω-asparagine selenoester and unprotected glycosylamine to perform rapid amide-bond ligation. This glycosylation strategy is highly compatible with the free carboxylic acids and hydroxyl groups of peptides and carbohydrates, and readily available for the assembly of structure-defined homogeneous N-linked glycopeptides, such as segments derived from glycoprotein EPO and IL-5.
Introduction
Many proteins undergo co- or post-translational modifications, including phosphorylation, acetylation, and glycosylation to fulfill their functions (Walsh and Jefferis, 2006; Carubbi et al., 2019). It is estimated that glycosylation modifications are associated with approximately 50% of human proteins (Clerc et al., 2016; Oliveira-Ferrer et al., 2017) and 30% of approved biopharmaceutical proteins (Zou et al., 2020), which are critical for important biological processes in living systems, such as cell's adhesion, recognition, targeting, and differentiation (Varki, 2017; Bhat et al., 2019). Despite the importance of glycosylations, rigorous evaluation of the relationship between the precise structure and biological function of glycoproteins is complicated by the structural heterogeneity of the oligosaccharides in biological organisms, and the difficulty to obtain sufficient amounts of structure-defined glycoproteins with single glycoform from natural sources (Park et al., 2009).
In order to develop viable and efficient strategies to chemically construct homogeneous complex N-linked glycopeptides and glycoproteins, extensive efforts and advances have been made in the field (Payne and Wong, 2010; Wilson et al., 2013; Okamoto et al., 2014a; Wang and Amin, 2014; Fairbanks, 2019; Li et al., 2019), such as the resin-bound glycosylation (Kunz and Unverzagt, 1988; Vetter et al., 1995; Offer et al., 1996; Mezzato et al., 2005; Kajihara et al., 2006; Yamamoto et al., 2008; Piontek et al., 2009a,b; Chen and Tolbert, 2010; Conroy et al., 2010; Ullmann et al., 2012; Okamoto et al., 2014b; Reif et al., 2014; Lee et al., 2016; Schöwe et al., 2019) and solution glycosylation (Anisfeld and Lansbury, 1990; Cohen-Anisfeld and Lansbury, 1993; Kaneshiro and Michael, 2006; Wang et al., 2011, 2012, 2013; Aussedat et al., 2012; Nagorny et al., 2012; Sakamoto et al., 2012; Joseph et al., 2013; Chai et al., 2016; Schöwe et al., 2019). However, unneglectable limitations still remain in these strategies. Consumption of large amount of precious materials and low coupling yields usually occurred for the glycopeptide assembly on-resin via either the stepwise (Scheme 1A) or the convergent (Scheme 1B) strategy. Based on the aspartylation technology pioneered by Lansbury and co-workers (Scheme 1C) (Anisfeld and Lansbury, 1990; Cohen-Anisfeld and Lansbury, 1993), Danishefsky group and Unverzagt group developed the synthetic methods and optimized the pseudoproline dipeptide building block to construct the peptide fragement at the site of Asn-Xaa-Ser/Thr, and this approach significantly suppressed the formation of aspartimide byproducts during glycosylation (Ullmann et al., 2012; Wang et al., 2012). Although useful, requirement for additional metal catalysts or protected C-terminal carboxylic acid derivatives may limit the application of this strategy in glycopeptide assembly.
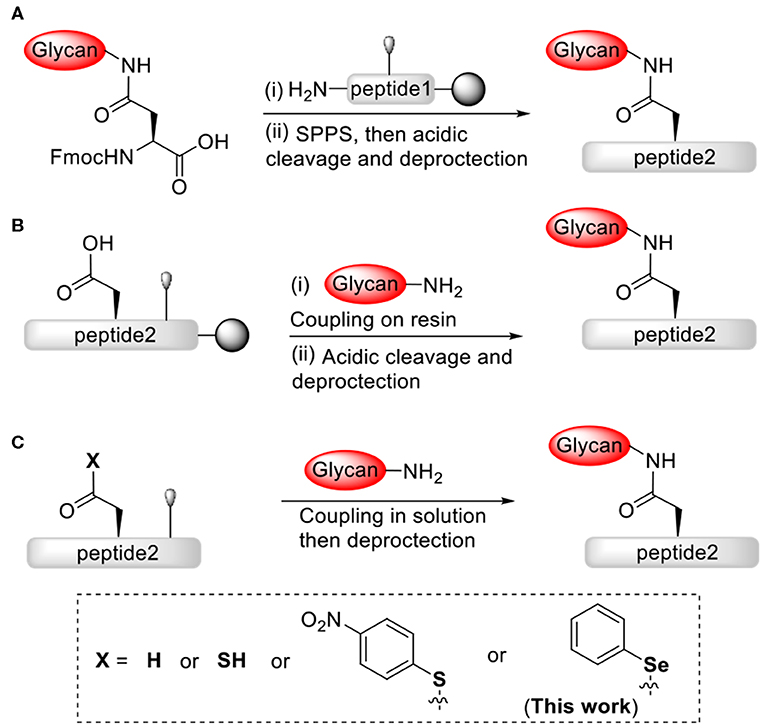
Scheme 1. Effective strategies for chemical construction of N-linked glycopeptides: (A) stepwise strategy via solid-phase; (B) convergent strategy via solid-phase; (C) convergent strategy via solution phase.
Notwithstanding substantial advances have been made in N-linked glycopeptides and glycoproteins synthesis, it is still a great challenge to efficiently achieve large N-linked gylcoproteins bearing complex glycan forms. The desired synthetic methods will have fewer protecting groups and modifications on the peptide and glycan fragments, and promote efficient and selective ligation reactions between fragments. Previously, our research group has developed a strategy for the convergent synthesis of N-linked glycopeptides via peptidyl ω-Asp p-nitrophenyl thioester-assisted glycosylation (Scheme 1C) (Du et al., 2016). This convergent strategy with direct aminolysis provides an access to complex N-linked glycopeptides, usually with good yields and simple operation, and is worthy of further investigating more reactions and applications.
Many investigators have proved that coupling of peptide fragments via direct aminolysis is a feasible method for preparation of peptides and glycopeptides. This method employs direct coupling reaction between peptide fragments bearing N-terminal free amines and peptide fragments bearing C-terminal active esters, such as oxoesters (Kemp and Vellaccio, 1975; Wan et al., 2008; Li et al., 2010), thioesters (Payne et al., 2008; Agrigento et al., 2014; LingáTung and Clarence, 2015; Gui et al., 2016) or selenoester derivatives (Grieco et al., 1981; Mitchell et al., 2015; Raj et al., 2015; Takei et al., 2017; Temperini et al., 2017; Du et al., 2018; Sayers et al., 2018a,b; Chisholm et al., 2020; Wang et al., 2020), eliminates the need for N-terminal cysteine residues or thiol ligation auxiliaries, which are generally required for the sequential native chemical ligation (Dawson et al., 1994; Kent, 2009). Notably, the active selenoesters or derivatives always offer enhanced reactivity compared to the thio- or oxoesters (Mitchell et al., 2015; Raj et al., 2015; Takei et al., 2017). Our previous studies have shown that the aminolysis of peptidyl selenoester is an efficient strategy for peptide and glycopeptide assembly (Yin et al., 2016; Du et al., 2018). Herein we are interested in pursuing a highly reactive peptidyl ω-Asp selenoester-assisted glycosylation methodology for constructing N-linked glycopeptides without coupling reagents (Scheme 1C). This methodology is assumed to be compatible with free carboxylic groups and hydroxyl groups of peptides and glycans.
Results and Discussion
Evaluation of the Reactivity of the Active Esters for Glycosidic Amide Bond Formation
To evaluate the methods for synthesizing N-linked glycopeptide synthesis via active ester-assisted aminolysis (Du et al., 2016), the activity and efficiency of different active esters were compared and investigated using model reactions, in which Fmoc-Gly ester 2 and glycosylamine 1a (Likhosherstov et al., 1986; Cohen-Anisfeld and Lansbury, 1993) were condensed in DMSO to form β-anomer product 3 and monitored by HPLC (Table 1, Figure 1).
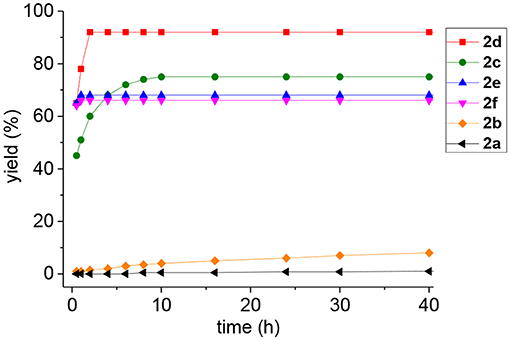
Figure 1. Reaction curves (yields of 3 vs. time) of the glycosidic linkage formation between 2a−2f and 1a.
For oxoester 2a, it has the lowest activity and almost no product was observed (Table 1, entry 1). For thioesters (Table 1, entries 2-3), phenyl thioester 2b underwent glycosidic bond formation slightly faster than the oxoester 2a, but it is not efficient enough to be applied in the N-linked glycopeptide synthesis; p-nitrophenyl thioester 2c with a strong electron-withdrawing group reacts more efficiently, providing the target product in a yield of 75% within 10 h, which is consistent with previous studies (Hondal et al., 2001; Du et al., 2016). Therefore, the peptidyl p-nitrophenyl thioester has been successfully utilized to prepare N-linked glycopeptide in our lab (Du et al., 2016).
To improve the efficiency of glycosylation reaction, various selenoesters were assessed under the same conditions (Table 1, entries 4–6). For seleno-phenyl ester 2d, it underwent complete conversion within 2 h, and afforded the target product 3 in 92% yield; for seleno-benzaldehyde esters 2e with the o-benzaldehyde group and 2f with the p-benzaldehyde group (Raj et al., 2015), they underwent complete conversion in <1 h, and gave the products in yield of 69 and 67%, respectively. We postulate that the participation of o-benzaldehyde (neighbor-participating group) and p-benzaldehyde, which both have electron-withdrawing groups can increase the phenyl selenoester's electrophile reaction rate, but also facilitate the hydrolysis reaction and reduce the yield of aminolysis product. Therefore, the seleno-phenyl ester 2d affords an optimal balance between high reactivity and sufficient stability, will be appropriate for the selenoester-assisted aminolysis in glycosylation reactions.
As shown in Table 2, we compared the reaction kinetic data p-nitrophenyl thioester 2c and seleno-phenyl ester 2d. As expected, the glycosylation reaction for the product 3 between glycine-derived ester and glycosylamine follows a second-order kinetics, with a rate constant 0.0071 ± 0.0004 M−1 s−1 for 2c and 0.0420 ± 0.0012 M−1 s−1 for 2d, respectively. The seleno-phenyl ester is roughly 6-times faster than the p-nitrophenyl thioester to form the glycosidic amide bond.
Condition Optimization
As depicted in Table 3, various glycosylation reaction conditions were evaluated for further optimization. From the results of optimizing the solvent (Table 3, entries 1–4), the efficiency of the glycosylation reaction was shown to be greatly boosted in DMSO, but the aqueous solution of NMP/PB is prone to decompose the seleno-phenyl ester 2d. The amounts of DIPEA from 0.1 to 3.0 equivalents didn't significantly influence the yields (Table 3, entries 4–7). Additionally, we found that the product 3 was achieved in optimal yield when seleno-phenyl ester 2d was treated with 2.0 equivalents of glycosylamine 1a (Table 3, entries 4, 8–9). In order to maximize the glycosylation and minimize the hydrolysis, we selected the optimum conditions, i.e., 2.0 equivalents of DIPEA and glycosylamine 1a, and 1.0 equivalent of seleno-phenyl ester 2d were dissolved in DMSO.
Substrate Scope
To explore the universal applicability of selenoester-assisted glycosylation, we embarked on the attachment of seleno-phenyl esters to a series of peptides to assemble peptidyl ω-Asp selenoester substrates, and examined substrates that incorporating the free C-terminal carboxylic groups and unprotected glycosylamines. A series of partially protected peptides bearing selenoesters at the ω-aspartyl terminus (including pseudoproline dipeptides that suppress aspartimide formation) were successfully prepared for evaluation (Ullmann et al., 2012; Wang et al., 2012). These peptide substrates were conducted via stepwise solid-phase peptide synthesis (SPPS), the general synthetic procedures for 4b-12b are outlined in Figure 2 (more details are shown in the Supporting Information). The installation of phenyl selenoester group at the ω-aspartyl terminus is straightforward on the resin: firstly, the allyl esters were removed; subsequently, the ω-aspartyl carboxyl groups were converted to selenoesters (4a-12a); finally, these peptidyl selenoesters were cleaved from the resin. The ω-aspartyl selenoester peptide substrates (4b-12b) were isolated via reverse-phase HPLC purification in 58–83% yields. In addition, the glycosylamines (Figure 3) for the study are monosaccharide 1a, chitobiose 1b and undecasaccharide 1c (extracted from fresh egg yolks) (Seko et al., 1997; Sun et al., 2014).
With peptidyl selenoesters and glycosylamines in hand, the glycosylation reactions at the site of natural ω-asparagine linkage were evaluated. On the one hand, the coupling of monosaccharide 1a and peptides 4b-6b gave glycosylated peptides 4c-6c in approximately 69%-83% isolated yields (Table 4, entries 1–3), proving the feasibility of utilizing unprotected glycosylamines together with peptidyl selenoesters bearing free C-terminal carboxylic groups in glycosylation reactions. To our delight, peptide 7b containing two ω-asparagine selenoesters, still gave an isolated yield of 80% of product 7c derived from multiply glycosylated protein erythropoietin (EPO; fragment 22–43) (Park et al., 2009; Wang et al., 2013; Wilson et al., 2013) with two glycosylation modifications (Table 4, entry 4). On the other hand, this strategy also afforded good results for glycosylation of disaccharides. As shown in entries 5–7, coupling of chitobiose 1b and peptidyl selenoesters 5b, 8b, and 9b formed glycosidic bond at ω-asparagine residue with excellent yields.
For this methodology, it is noteworthy that the desired N-linked glycopeptides are synthesized rapidly only through mixing two substrates, without using a condensation reagent, and the workup procedure is simple. Excitingly, the free carboxylic groups of ω-aspartyl peptide segments were readily converted into peptidyl selenoesters for further condensation with various glycosylamines. Additionally, each amino acid protecting group in glycopeptide can be easily removed in an acidic environment.
Syntheses of N-Linked Glycopeptides With Complex-Type Oligosaccharide
As shown in Table 5, the protocol of selenoester-mediated glycopeptide synthesis is extended to complex-type oligosaccharide amines. Given the structural complexity of the precious undecasaccharide amine 1c, an excessive amount of peptidyl selenoester (1.5:1) was used, and the final products (10e, 11e, 12e) of the peptides modified with undecasaccharides were achieved in good yields of 59–65% (Table 5, entries 1–3). Specially, product 12e corresponds to the truncated segment of the glycoprotein found in human interleukin-5 (IL-5, an eosinophil chemotactic factor, fragment 26–43) (Coffman et al., 1989; Liu and Dong, 2018).
Conclusion
In this work we have developed a convergent and facile synthetic methodology to construct homogeneous N-linked glycopeptides from the peptides with ω-Asp phenyl selenoester, the use of peptidyl selenoesters has the merits of simple operation and obtained excellent yields of N-linked glycopeptides, such as truncated segments derived from glycoprotein EPO or IL-5. This selenoester-mediated glycosylation provides several advantages: the reactivity of the peptide ester is improved, the complex sialyloligosaccharide in its native form without protection, it is not only compatible with free C-terminal carboxylic acid groups, but also rapidly forms glycosidic bond without additional coupling reagents or catalysts. This method will be further applied to the formation of homogenous N-linked glycopeptides and glycoproteins with therapeutic potential.
Data Availability Statement
The raw data supporting the conclusions of this article will be made available by the authors, without undue reservation, to any qualified researcher.
Author Contributions
JG conceived the project. J-JD, LZ, and X-FG designed and performed the experiments. All authors discussed the results and commented on the manuscript.
Funding
The project was funded by the National Natural Science Foundation of China (Nos. 21772056 and 81670531), the National Key Research and Development Program of China (No. 2017YFA0505200), Program of Introducing Talents of Discipline to Universities of China (111 program, B17019), and the Research Fund of East China University of Technology (No. DHBK2017114).
Conflict of Interest
The authors declare that the research was conducted in the absence of any commercial or financial relationships that could be construed as a potential conflict of interest.
Supplementary Material
The Supplementary Material for this article can be found online at: https://www.frontiersin.org/articles/10.3389/fchem.2020.00396/full#supplementary-material
References
Agrigento, P., Albericio, F., Chamoin, S., Dacquignies, I., Koc, H., and Eberle, M. (2014). Facile and mild synthesis of linear and cyclic peptides via thioesters. Org. Lett. 16, 3922–3925. doi: 10.1021/ol501669n
Anisfeld, S. T., and Lansbury, P. T. (1990). A convergent approach to the chemical synthesis of asparagine-linked glycopeptides. J. Org. Chem. 55, 5560–5562. doi: 10.1021/jo00308a009
Aussedat, B., Fasching, B., Johnston, E., Sane, N., Nagorny, P., and Danishefsky, S. J. (2012). Total synthesis of the α-subunit of human glycoprotein hormones: toward fully synthetic homogeneous human follicle-stimulating hormone. J. Am. Chem. Soc. 134, 3532–3541. doi: 10.1021/ja2111459
Bhat, A. H., Maity, S., Giri, K., and Ambatipudi, K. (2019). Protein glycosylation: sweet or bitter for bacterial pathogens? Crit. Rev. Microbiol. 45, 82–102. doi: 10.1080/1040841x.2018.1547681
Carubbi, F., Alunno, A., Gerli, R., and Giacomelli, R. (2019). Post-translational modifications of proteins: novel insights in the autoimmune response in rheumatoid arthritis. Cells 8:657. doi: 10.3390/cells8070657
Chai, H., Le Mai Hoang, K., Vu, M. D., Pasunooti, K., Liu, C.-F., and Liu, X.-W. (2016). N-linked glycosyl auxiliary-mediated native chemical ligation on aspartic acid: application towards N-glycopeptide synthesis. Angew. Chem. Int. Ed. 55, 10363–10367. doi: 10.1002/anie.201605597
Chen, R., and Tolbert, T. J. (2010). Study of on-resin convergent synthesis of N-linked glycopeptides containing a large high mannose N-linked oligosaccharide. J. Am. Chem. Soc. 132, 3211–3216. doi: 10.1021/ja9104073
Chisholm, T. S., Kulkarni, S. S., Hossain, K. R., Cornelius, F., Clarke, R. J., and Payne, R. J. (2020). Peptide ligation at high dilution via reductive diselenide-selenoester ligation. J. Am. Chem. Soc. 142, 1090–1100. doi: 10.1021/jacs.9b12558
Clerc, F., Reiding, K. R., Jansen, B. C., Kammeijer, G. S., Bondt, A., and Wuhrer, M. (2016). Human plasma protein N-glycosylation. Glycoconj. J. 33, 309–343. doi: 10.1007/s10719-015-9626-2
Coffman, R. L., Seymour, B. W., Hudak, S., Jackson, J., and Rennick, D. (1989). Antibody to interleukin-5 inhibits helminth-induced eosinophilia in mice. Science 245, 308–310. doi: 10.1126/science.2787531
Cohen-Anisfeld, S. T., and Lansbury, P. T. (1993). A practical, convergent method for glycopeptide synthesis. J. Am. Chem. Soc. 115, 10531–10537. doi: 10.1021/ja00076a010
Conroy, T., Jolliffe, K. A., and Payne, R. J. (2010). Synthesis of N-linked glycopeptides via solid-phase aspartylation. Org. Biomol. Chem. 8, 3723–3733. doi: 10.1039/c003673k
Dawson, P. E., Muir, T. W., Clark-Lewis, I., and Kent, S. B. H. (1994). Synthesis of proteins by native chemical ligation. Science 266, 776–779. doi: 10.1126/science.7973629
Du, J.-J., Gao, X.-F., Xin, L.-M., Lei, Z., Liu, Z., and Guo, J. (2016). Convergent synthesis of N-linked glycopeptides via aminolysis of ω-Asp p-nitrophenyl thioesters in solution. Org. Lett. 18, 4828–4831. doi: 10.1021/acs.orglett.6b02288
Du, J.-J., Xin, L.-M., Lei, Z., Zou, S.-Y., Xu, W.-B., Wang, C.-W., et al. (2018). Glycopeptide ligation via direct aminolysis of selenoester. Chin. Chem. Lett. 29, 1127–1130. doi: 10.1016/j.cclet.2018.04.016
Fairbanks, A. J. (2019). Chemoenzymatic synthesis of glycoproteins. Curr. Opin. Chem. Biol. 53, 9–15. doi: 10.1016/j.cbpa.2019.05.015
Grieco, P. A., Jaw, J. Y., Claremon, D., and Nicolaou, K. (1981). N-Phenylselenophthalimide. A useful reagent for the facile transformation of (1) carboxylic acids into either selenol esters or amides and (2) alcohols into alkyl phenyl selenides. J Org Chem. 46, 1215–1217. doi: 10.1021/jo00319a037
Gui, Y., Qiu, L., Li, Y., Li, H., and Dong, S. (2016). Internal activation of peptidyl prolyl thioesters in native chemical ligation. J. Am. Chem. Soc. 138, 4890–4899. doi: 10.1021/jacs.6b01202
Hondal, R. J., Nilsson, B. L., and Raines, R. T. (2001). Selenocysteine in native chemical ligation and expressed protein ligation. J. Am Chem. Soc. 123, 5140–5141. doi: 10.1021/ja005885t
Joseph, R., Dyer, F. B., and Garner, P. (2013). Rapid formation of N-glycopeptides via cu(II)-promoted glycosylative ligation. Org. Lett. 15, 732–735. doi: 10.1021/ol302961s
Kajihara, Y., Yoshihara, A., Hirano, K., and Yamamoto, N. (2006). Convenient synthesis of a sialylglycopeptide-thioester having an intact and homogeneous complex-type disialyl-oligosaccharide. Carbohydr. Res. 341, 1333–1340. doi: 10.1016/j.carres.2006.04.037
Kaneshiro, C. M., and Michael, K. (2006). A convergent synthesis of N-glycopeptides. Angew. Chem. Int. Ed. 45, 1077–1081. doi: 10.1002/anie.200502687
Kemp, D., and Vellaccio, F. Jr, (1975). Rapid intramolecular acyl transfer from phenol to carbinolamine. Progress toward a new class of peptide coupling reagent. Org. Chem. 40, 3003–3004. doi: 10.1021/jp3083858
Kent, S. B. H. (2009). Total chemical synthesis of proteins. Chem. Soc. Rev. 38, 338–351. doi: 10.1039/b700141j
Kunz, H., and Unverzagt, C. (1988). Protecting-group-dependent stability of intersaccharide bonds-synthesis of a fucosyl-chitobiose glycopeptide. Angew. Chem. Int. Ed. 27, 1697–1699. doi: 10.1002/anie.198816971
Lee, C. L., Liu, H., Wong, C. T. T., Chow, H. Y., and Li, X. (2016). Enabling N-to-C Ser/Thr ligation for convergent protein synthesis via combining chemical ligation approaches. J. Am. Chem. Soc. 138, 10477–10484. doi: 10.1021/jacs.6b04238
Li, X., Lam, H. Y., Zhang, Y., and Chan, C. K. (2010). Salicylaldehyde ester-induced chemoselective peptide ligations: enabling generation of natural peptidic linkages at the serine/threonine sites. Org. Lett. 12, 1724–1727. doi: 10.1021/ol1003109
Li, Y., Tran, A. H., Danishefsky, S. J., and Tan, Z. (2019). Chemical biology of glycoproteins: From chemical synthesis to biological impact. Methods Enzymol. 621, 213–229. doi: 10.1016/bs.mie.2019.02.030
Likhosherstov, L. M., Novikova, O. S., Derevitskaja, V. A., and Kochetkov, N. K. (1986). A new simple synthesis of amino sugar β-D-glycosylamines. Carbohydr. Res. 146, C1–C5. doi: 10.1016/0008-6215(86)85037-6
LingáTung, C., and Clarence, T. (2015). Peptide 2-formylthiophenol esters do not proceed through a Ser/Thr ligation pathway, but participate in a peptide aminolysis to enable peptide condensation and cyclization. Org. Biomol. Chem. 13, 6922–6926. doi: 10.1039/C5OB00825E
Liu, J., and Dong, S. (2018). Synthetic studies toward human interleukin-5. Chin. Chem. Lett. 29, 1131–1134. doi: 10.1016/j.cclet.2018.05.014
Mezzato, S., Schaffrath, M., and Unverzagt, C. (2005). An orthogonal double-linker resin facilitates the efficient solid-phase synthesis of complex-type N-glycopeptide thioesters suitable for native chemical ligation. Angew. Chem. Int. Ed. 44, 1650–1654. doi: 10.1002/anie.200461125
Mitchell, N. J., Malins, L. R., Liu, X., Thompson, R. E., Chan, B., Radom, L., et al. (2015). Rapid additive-free selenocystine–selenoester peptide ligation. J. Am. Chem. Soc. 137, 14011–14014. doi: 10.1021/jacs.5b07237
Nagorny, P., Sane, N., Fasching, B., Aussedat, B., and Danishefsky, S. J. (2012). Probing the frontiers of glycoprotein synthesis: the fully elaborated β-subunit of the human follicle-stimulating hormone. Angew. Chem. Int. Ed. 51, 975–979. doi: 10.1002/anie.201107482
Offer, J., Quibell, M., and Johnson, T. (1996). On-resin solid-phase synthesis of asparagine N-linked glycopeptides: use of N-(2-acetoxy-4-methoxybenzyl) (AcHmb) aspartyl amide-bond protection to prevent unwanted aspartimide formation. J. Chem. Soc. Perkin Trans. 1, 175–182. doi: 10.1039/P19960000175
Okamoto, R., Izumi, M., and Kajihara, Y. (2014a). Decoration of proteins with sugar chains: recent advances in glycoprotein synthesis. Curr. Opin. Chem. Biol. 22, 92–99. doi: 10.1016/j.cbpa.2014.09.029
Okamoto, R., Mandal, K., Ling, M., Luster, A. D., Kajihara, Y., and Kent, S. B. H. (2014b). Total chemical synthesis and biological activities of glycosylated and non-glycosylated forms of the chemokines CCL1 and Ser-CCL1. Angew. Chem. Int. Ed. 53, 5188–5193. doi: 10.1002/anie.201310574
Oliveira-Ferrer, L., Legler, K., and Milde-Langosch, K. (2017). Role of protein glycosylation in cancer metastasis. Semin. Cancer Biol. 44, 141–152. doi: 10.1016/j.semcancer.2017.03.002
Park, S. S., Park, J., Ko, J., Chen, L., Meriage, D., Crouse-Zeineddini, J., et al. (2009). Biochemical assessment of erythropoietin products from Asia versus US Epoetin alfa manufactured by Amgen. J. Pharm. Sci. 98, 1688–1699. doi: 10.1002/jps.21546
Payne, R. J., Ficht, S., Greenberg, W. A., and Wong, C. H. (2008). Cysteine-free peptide and glycopeptide ligation by direct aminolysis. Angew. Chem. Int. Ed. 47, 4411–4415. doi: 10.1002/anie.200705298
Payne, R. J., and Wong, C.-H. (2010). Advances in chemical ligation strategies for the synthesis of glycopeptides and glycoproteins. Chem. Commun. 46, 21–43. doi: 10.1039/B913845E
Piontek, C., Ring, P., Harjes, O., Heinlein, C., Mezzato, S., Lombana, N., et al. (2009a). Semisynthesis of a homogeneous glycoprotein enzyme: ribonuclease C: Part 1. Angew. Chem. Int. Ed. 48, 1936–1940. doi: 10.1002/anie.200804734
Piontek, C., Varón Silva, D., Heinlein, C., Pöhner, C., Mezzato, S., Ring, P., et al. (2009b). Semisynthesis of a homogeneous glycoprotein enzyme: ribonuclease C: Part 2. Angew. Chem. Int. Ed. 48, 1941–1945. doi: 10.1002/anie.200804735
Raj, M., Wu, H., Blosser, S. L., Vittoria, M. A., and Arora, P. S. (2015). Aldehyde capture ligation for synthesis of native peptide bonds. J. Am. Chem. Soc. 137, 6932–6940. doi: 10.1021/jacs.5b03538
Reif, A., Siebenhaar, S., Tröster, A., Schmälzlein, M., Lechner, C., Velisetty, P., et al. (2014). Semisynthesis of biologically active glycoforms of the human cytokine interleukin 6. Angew. Chem. Int. Ed. 53, 12125–12131. doi: 10.1002/anie.201407160
Sakamoto, I., Tezuka, K., Fukae, K., Ishii, K., Taduru, K., Maeda, M., et al. (2012). Chemical synthesis of homogeneous human glycosyl-interferon-β that exhibits potent antitumor activity in vivo. J. Am. Chem. Soc. 134, 5428–5431. doi: 10.1021/ja2109079
Sayers, J., Karpati, P. M., Mitchell, N. J., Goldys, A. M., Kwong, S. M., Firth, N., et al. (2018a). Construction of challenging proline–proline junctions via diselenide–selenoester ligation chemistry. J. Am. Chem. Soc. 140, 13327–13334. doi: 10.1021/jacs.8b07877
Sayers, J., Payne, R. J., and Winssinger, N. (2018b). Peptide nucleic acid-templated selenocystine-selenoester ligation enables rapid miRNA detection. Chem. Sci. 9, 896–903. doi: 10.1039/C7SC02736B
Schöwe, M. J., Keiper, O., Unverzagt, C., and Wittmann, V. (2019). A Tripeptide approach to the solid-phase synthesis of peptide thioacids and N-glycopeptides. Chem. Eur. J. 25, 15759–15764. doi: 10.1002/chem.201904688
Seko, A., Koketsu, M., Nishizono, M., Enoki, Y., Ibrahim, H. R., Juneja, L. R., et al. (1997). Occurrence of a sialylglycopeptide and free sialylglycans in hen's egg yolk. Biochim. Biophys. Acta Gen. Subj. 1335, 23–32. doi: 10.1016/S0304-4165(96)00118-3
Sun, B., Bao, W., Tian, X., Li, M., Liu, H., Dong, J., et al. (2014). A simplified procedure for gram-scale production of sialylglycopeptide (SGP) from egg yolks and subsequent semi-synthesis of Man3GlcNAc oxazoline. Carbohydr. Res. 396, 62–69. doi: 10.1016/j.carres.2014.07.013
Takei, T., Andoh, T., Takao, T., and Hojo, H. (2017). One-pot four-segment ligation using seleno- and thioesters: synthesis of superoxide dismutase. Angew. Chem. Int. Ed. 56, 15708–15711. doi: 10.1002/anie.201709418
Temperini, A., Piazzolla, F., Minuti, L., Curini, M., and Siciliano, C. (2017). General, mild, and metal-free synthesis of phenyl selenoesters from anhydrides and their use in peptide synthesis. J. Org. Chem. 82, 4588–4603. doi: 10.1021/acs.joc.7b00173
Ullmann, V., Rädisch, M., Boos, I., Freund, J., Pöhner, C., Schwarzinger, S., et al. (2012). Convergent solid-phase synthesis of N-glycopeptides facilitated by pseudoprolines at consensus-sequence Ser/Thr residues. Angew. Chem. Int. Ed. 51, 11566–11570. doi: 10.1002/anie.201204272
Vetter, D., Tumelty, D., Singh, S. K., and Gallop, M. A. (1995). A versatile solid-phase synthesis of N-linked glycopeptides. Angew. Chem. Int. Ed. 34, 60–63. doi: 10.1002/anie.199500601
Walsh, G., and Jefferis, R. (2006). Post-translational modifications in the context of therapeutic proteins. Nat. Biotechnol. 24, 1241–1252. doi: 10.1038/nbt1252
Wan, Q., Chen, J., Yuan, Y., and Danishefsky, S. J. (2008). Oxo-ester mediated native chemical ligation: concept and applications. J. Am Chem. Soc. 130, 15814–15816. doi: 10.1021/ja804993y
Wang, L.-X., and Amin, M. N. (2014). Chemical and chemoenzymatic synthesis of glycoproteins for deciphering functions. Chem. Biol. 21, 51–66. doi: 10.1016/j.chembiol.2014.01.001
Wang, P., Aussedat, B., Vohra, Y., and Danishefsky, S. J. (2012). An advance in the chemical synthesis of homogeneous N-linked glycopolypeptides by convergent aspartylation. Angew. Chem. Int. Ed. 51, 11571–11575. doi: 10.1002/anie.201205038
Wang, P., Dong, S., Shieh, J.-H., Peguero, E., Hendrickson, R., Moore, M. A. S., et al. (2013). Erythropoietin derived by chemical synthesis. Science 342, 1357–1360. doi: 10.1126/science.1245095
Wang, P., Li, X., Zhu, J., Chen, J., Yuan, Y., Wu, X., et al. (2011). Encouraging progress in the ω-aspartylation of complex oligosaccharides as a general route to β-N-linked glycopolypeptides. J. Am. Chem. Soc. 133, 1597–1602. doi: 10.1021/ja110115a
Wang, X., Corcilius, L., Premdjee, B., and Payne, R. J. (2020). Synthesis and utility of β-selenophenylalanine and β-selenoleucine in diselenide–selenoester ligation. J Org. Chem. 85, 1567–1578. doi: 10.1021/acs.joc.9b02665
Wilson, R. M., Dong, S., Wang, P., and Danishefsky, S. J. (2013). The winding pathway to erythropoietin along the chemistry–biology frontier: a success at last. Angew. Chem. Int. Ed. 52, 7646–7665. doi: 10.1002/anie.201301666
Yamamoto, N., Tanabe, Y., Okamoto, R., Dawson, P. E., and Kajihara, Y. (2008). Chemical synthesis of a glycoprotein having an intact human complex-type sialyloligosaccharide under the boc and fmoc synthetic strategies. J. Am. Chem. Soc. 130, 501–510. doi: 10.1021/ja072543f
Yin, X.-G., Gao, X.-F., Du, J.-J., Zhang, X.-K., Chen, X.-Z., Wang, J., et al. (2016). Preparation of protein conjugates via homobifunctional diselenoester cross-linker. Org. Lett. 18, 5796–5799. doi: 10.1021/acs.orglett.6b02568
Keywords: N-linked glycopeptide, glycosylation, selenoester, aminolysis, chemical synthesis
Citation: Du J-J, Zhang L, Gao X-F, Sun H and Guo J (2020) Peptidyl ω-Asp Selenoesters Enable Efficient Synthesis of N-Linked Glycopeptides. Front. Chem. 8:396. doi: 10.3389/fchem.2020.00396
Received: 25 January 2020; Accepted: 15 April 2020;
Published: 05 May 2020.
Edited by:
Zhongping Tan, Chinese Academy of Medical Sciences and Peking Union Medical College, ChinaCopyright © 2020 Du, Zhang, Gao, Sun and Guo. This is an open-access article distributed under the terms of the Creative Commons Attribution License (CC BY). The use, distribution or reproduction in other forums is permitted, provided the original author(s) and the copyright owner(s) are credited and that the original publication in this journal is cited, in accordance with accepted academic practice. No use, distribution or reproduction is permitted which does not comply with these terms.
*Correspondence: Jun Guo, amd1byYjeDAwMDQwO21haWwuY2NudS5lZHUuY24=
†These authors have contributed equally to this work