- 1The State Key Laboratory of Refractories and Metallurgy and Institute of Advanced Materials and Nanotechnology, Wuhan University of Science and Technology, Wuhan, China
- 2The College of Resources and Environment Engineering, Wuhan University of Science and Technology, Wuhan, China
- 3Departments of Physics, Materials Science and Engineering, and Biomedical Engineering, City University of Hong Kong, Hong Kong, China
Development of efficient electrocatalysts combining the features of low cost and high performance for both the hydrogen evolution reaction (HER) and oxygen evolution reaction (OER) still remains a critical challenge. Here, we proposed a facile strategy to construct in situ a novel hierarchical heterostructure composed of 0D−2D CoSe2/MoSe2 by the selenization of CoMoO4 nanosheets grafted on a carbon cloth (CC). In such integrated structure, CoSe2 nanoparticles dispersed well and tightly bonded with MoSe2 nanosheets, which can not only enhance kinetics due to the synergetic effects, thus promoting the electrocatalytic activity, but also effectively improve the structural stability. Benefiting from its unique architecture, the designed CoSe2/MoSe2 catalyst exhibits superior OER and HER performance. Specifically, a small overpotential of 280 mV is acquired at a current density of 10 mA·cm−2 for OER with a small Tafel slope of 86.8 mV·dec−1, and the overpotential is 90 mV at a current density of 10 mA·cm−2 for HER with a Tafel slope of 84.8 mV·dec−1 in 1 M KOH. Furthermore, the symmetrical electrolyzer assembled with the CoSe2/MoSe2 catalysts depicts a small cell voltage of 1.63 V at 10 mA·cm−2 toward overall water splitting.
Introduction
Hydrogen is a promising energy source that boasts a high power density and environmental friendliness; therefore, electrolysis of water is hotly pursued as a renewable, efficient, and pollution-free technique (Amiinu et al., 2017; Luo et al., 2018; Zhu et al., 2018). Electrocatalytic water splitting consists of the hydrogen evolution reaction (HER) and oxygen evolution reaction (OER), and electrocatalysts as the chemical reaction centers play a critical role in the water splitting electrolyzer. Although some noble metal oxide catalysts (RuO2 and IrO2) have high electrocatalytic performance for the OER and some noble metal catalysts (Pt and Ir) deliver good electrochemical property in the HER, the high cost and scarcity restrict their wide industrial application (Trasatti, 1972, 1984). Therefore, noble-metal-free catalysts with high stability and efficiency are crucial to large-scale hydrogen production from water splitting. Currently, the OER activity in alkaline solution is the bottleneck in overall water splitting due to the sluggish kinetics arising from the multiproton-coupled electron transfer steps (Jamesh and Sun, 2018). In practice, the HER catalyst in the electrolyzer should be compatible with the OER catalyst and functions in the same medium. Hence, development of suitable bifunctional noble-metal-free electrocatalyts with both high HER and OER performance in alkaline media is of great significance.
In recent years, transition metal dichalcogenides (TMDs) have attracted significant research interests owing to their earth-abundant reserves and acceptable activity for electrocatalytic HER (Xie et al., 2013; Zhang et al., 2017a; Xue et al., 2018; Wang et al., 2019). Particularly, layered MoSe2 has been considered as a promising HER electrocatalyst because of its unique structure features and high electrochemical activity (Shi et al., 2015; Chen et al., 2018a; Zhang et al., 2018). Theoretical research has demonstrated that the Gibbs free energy for H atom absorption on the edge of MoSe2 is lower than that of MoS2 due to the more metallic nature of MoSe2, revealing the higher HER performance (Tang et al., 2014; Lai et al., 2017; Yang et al., 2018). In addition, it also has been experimentally confirmed that the unsaturated Se edges in MoSe2 nanosheets are extremely active as the S edges in MoS2, which is responsible for the high HER activity (Jaramillo et al., 2007; Tang and Jiang, 2016). However, similar to MoS2, the HER activity of layered MoSe2 is largely limited by its poor conductivity and serious aggregation or restacking during the synthesis procedure (Mao et al., 2015; Qu et al., 2015), inhibiting the practical application of MoSe2 catalyst. Therefore, it is significant to improve the electrochemical activity of MoSe2-based catalyst. Recent works have shown that coupling MoSe2 with other transition metal selenides and constructing heterostrucurted materials could be an effective approach to further enhance the electrochemical performance of MoSe2. For instance, Wang et al. found that the MoSe2@Ni0.85Se nanowire delivered enhanced kinetics and performance for HER in alkaline conditions due to the high density of active edges of MoSe2 and the good conductivity of Ni0.85Se (Wang et al., 2017a). Zhang et al. synthesized 3D MoSe2/NiSe2 nanowires, which significantly enhanced HER activity with a low Tafel slope and overpotential in 0.5 M H2SO4, because the 3D structure affords more active sites (Zhang et al., 2017a). Liu et al. fabricated MoSe2-NiSe@carbon heteronanostructures and achieved glorious HER catalytic properties and excellent durability in both acidic and base conditions (Liu et al., 2018). In addition, the hierarchical mesoporous MoSe2@CoSe/N–C composite also exhibits outstanding HER activity (Chen et al., 2019b). Despite significant success, most of previous reports mainly focused on the improvement of HER performance, while the OER activity of MoSe2 catalyst in alkaline media has been ignored. Hence, the rational design and fabrication of MoSe2-based bifunctional electrocatalysts with satisfactory activity and stability toward overall water splitting in alkaline solution still remain a big challenge.
In this work, we developed a facile in situ phase separation strategy to construct a novel hierarchical heterostructure consisting of 0D−2D CoSe2/MoSe2 via the selenization of CoMoO4 nanosheets supported on a carbon cloth (CC) (Figure 1). Due to the in situ phase transformation, CoSe2 nanoparticles are uniformly anchored on MoSe2 nanosheets in the integrated structure, which can not only enhance reaction kinetics because of the synergetic effects, thus boosting the electrocatalytic activity, but also effectively suppress the aggregation/restacking of MoSe2 nanosheets, thereby improving the structural stability. Moreover, the hierarchical structure assembled by 0D−2D CoSe2/MoSe2 could provide abundant active sites for the electrochemical reactions. As a result, the designed CoSe2/MoSe2 architecture exhibits outstanding OER and HER performance in alkaline media. More specifically, a small overpotential of 280 mV is achieved at a current density of 10 mA·cm−2 for OER with a small Tafel slope of 86.8 mV·dec−1, and the overpotential is 90 mV at a current density of 10 mA·cm−2 for HER with a Tafel slope of 84.8 mV·dec−1 in 1 M KOH. Moreover, the symmetrical electrolyzer assembled with the CoSe2/MoSe2 catalysts delivers a small cell voltage of 1.63 V at 10 mA·cm−2 toward overall water splitting.
Experimental Section
Synthesis of CoMoO4 Nanosheet
Firstly, a pristine carbon cloth (CC) was treated with nitric acid solution overnight, subsequently ultrasonicated in deionized (DI) water and dried in an oven at 80°C for 2 h. After that, 1 mmol cobalt acetate, 1 mmol ammonium molybdate, 2 mmol urea, and 5 mmol ammonium fluoride were dissolved in 30 mL DI water, followed by ultrasonication for 30 min. Then, the homogeneous solution was poured into a 50-mL Teflon-lined stainless autoclave with the CC kept at 150°C for 6 h. After cooling to room temperature, the CC was washed with DI water for several times and dried in a vacuum freeze-dryer overnight. Finally, the obtained sample was treated at 400°C for 2 h with a ramp rate of 5°C min−1 in an argon atmosphere.
Synthesis of CoSe2/MoSe2
The as-prepared CoMoO4 precursor was reacted with 0.5 g selenium powder at 450°C for 1 h under an Ar/H2 (90%/10%) atmosphere to form the CoSe2/MoSe2.
Synthesis of CoSe2
The CoSe2 was prepared through two steps. Firstly, the treated CC was immersed in a 0.1 M Co(NO3)2 solution for the electrodeposition of Co (Yang et al., 2015). Then, the collected sample was reacted with 0.5 g selenium powder under an Ar/H2 (90%/10%) atmosphere at 450°C for 1 h.
Synthesis of MoSe2
Firstly, MoS2 was prepared via hydrothermal reaction with the CC at 200°C for 12 h, followed by heating at 400°C for 2 h to form MoO3 (Wu et al., 2018). Then, the obtained MoO3 was reacted with 0.5 g selenium powder at 450°C for 1 h under an Ar/H2 (90%/10%) atmosphere.
Preparation of Pt/C
Four milligrams of 20% Pt/C and 20 μL 5% Nafion solution were added into 1 mL solution of isopropanol and DI water (9:1) and then sonicated to form a uniform solution. Finally, the 1*1 cm2 CC was soaked in the homogeneous solution and dried in air at atmospheric temperature.
Preparation of RuO2
Four milligrams of RuO2 and 20 μL 5% Nafion solution were added into 1 mL solution of isopropanol and DI water (9:1), and then the sample was sonicated to form a uniform solution. Finally, the 1*1 cm2 CC was soaked in the homogeneous solution and dried in air at atmospheric temperature.
Characterization
The phase composition of the samples were characterized by X-ray diffraction (XRD, Bruker D8A A25), and the chemical states were determined through X-ray photoelectron spectroscopy (XPS, ESCALB 250Xi). The morphology and microstructure were recorded via field emission scanning electron microscopy (FE-SEM, FEI Nova NANOSEM 400) and high-resolution transmission electron microscopy (HR-TEM, JEM-2100 UHR STEM).
Electrochemical Measurements
All samples made use of a three-electrode system performed by a biologic VSP300 type electrochemical workstation (Biologic Science Instruments, France). The sample of CoSe2/MoSe2 was put on the electrode holder as the working electrode with a mass loading of 4 mg/cm2, the saturated calomel electrode (SCE) was the reference electrode, and a carbon rod served as the counter electrode. The electrolyte was 1 M KOH solution with saturated N2. Linear sweep voltammetry (LSV) was characterized by polarization curves of OER with a scanning rate of 5 mV s−1 from 0 to 0.8 V vs. SCE. Similarly, the polarization curves of HER were determined under the same condition from 0 to −0.8 V vs. SCE. The potentials were standardized by a reversible hydrogen electrode (RHE) as shown in the following: E (RHE) = E (SCE) + 0.059 × pH with instrument automatic 85% iR compensation. The electrochemically active surface area (ECSA) was calculated by cyclic voltammetry (CV) performed from −0.3 to −0.2 V vs. SCE with different scanning rates of 40, 60, 80, 100, and 120 mV s−1. Electrochemical impedance spectroscopy (EIS) measurements were conducted by biologic VMP3 (Biologic Science Instruments, France) from 100 KHz to 0.1 Hz. The overall water-splitting electrolyzer was performed with CoSe2/MoSe2 as electrodes and 1 M KOH as the electrolyte.
Results and Discussion
Figure 2A presents the FE-SEM image of the as-prepared CoMoO4 precursor, which presents uniform nanosheets (with a lateral size of 2 μm) perpendicularly grown on the CC substrate with high coverage. After a selenization process, the obtained CoSe2/MoSe2 sample well maintains the pristine morphology of the CoMoO4 precursor (Figure 2B). Moreover, the high-magnification SEM image further reveals that lots of nanoparticles are well dispersed on the surface of the nanosheet (Figure 2C), implying the structure and phase evolution during the selenization treatment. The elemental maps in (Figure 2D) show that Mo, Co, and Se are uniformly distributed throughout the nanosheets. In addition, the low-resolution TEM images in (Figures 2E,F) display that nanoparticles are uniformly distributed on the nanosheet during the thermal reduction procedure, forming the 0D/2D structure. Furthermore, the high-resolution TEM (Figure 2G) shows the lattice fringes of 0.26 nm and 0.65 nm corresponding to the (111) and (002) planes of CoSe2 and MoSe2, respectively (Qu et al., 2016; Liu et al., 2017), demonstrating the successful formation of the CoSe2/MoSe2 after the selenization reaction.
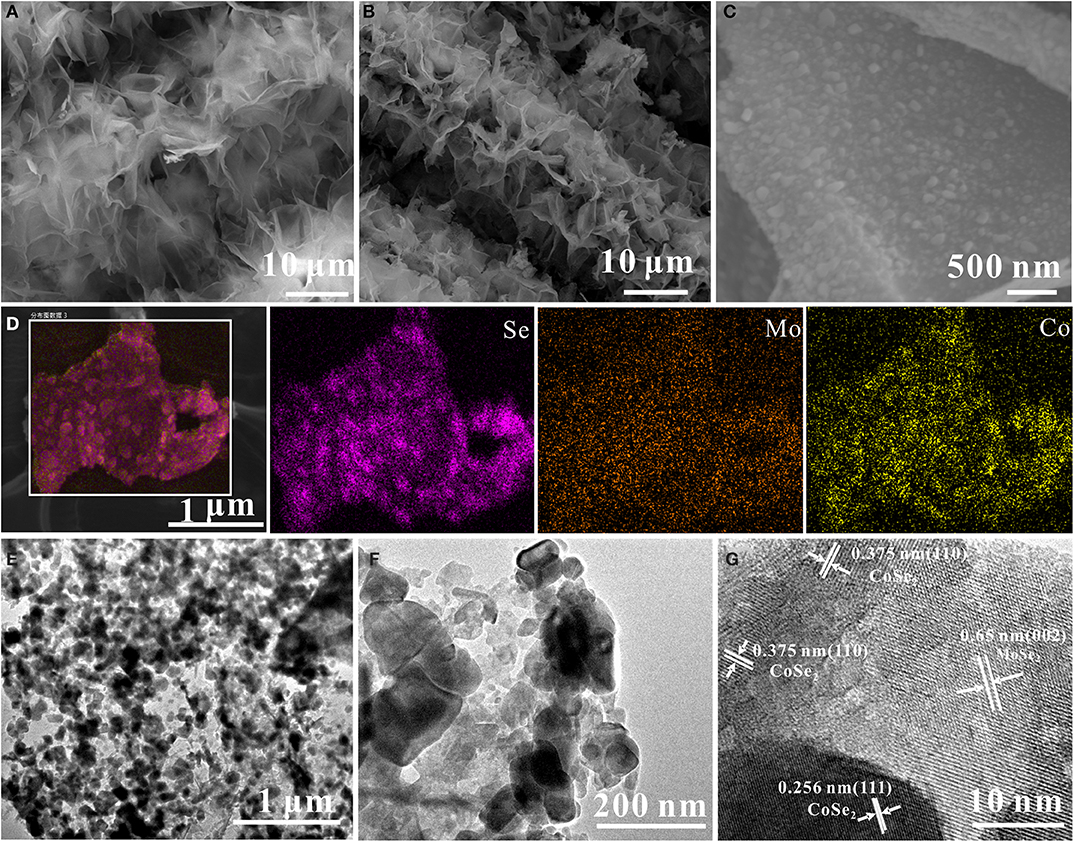
Figure 2. FE-SEM images of (A) CoMoO4 and (B,C) CoSe2/MoSe2; (D) elemental maps of CoSe2/MoSe2; (E,F) TEM of CoSe2/MoSe2; (G) HR-TEM images of CoSe2/MoSe2.
To investigate phase evolution during the selenization process, the crystal structure and phase composition of the obtained samples were characterized by X-ray diffraction (XRD) analysis (Figure 3A). The diffraction peaks of CoMoO4 precursor (in the black line) can be well indexed to the CoMoO4 phase (JCPDS No: 21-0868) (Wang et al., 2016). After the thermal reduction, some new diffraction peaks can be observed. The diffraction peaks at around 13.7 °, 27.6 °, 31.4 °, and 37.8 ° can be assigned to the MoSe2 phase (JCPDS No: 77-1715) (Qu et al., 2016), while the other peaks could be attributed to the phase of CoSe2 (JCPDS No:53-0449) (Liu et al., 2017). The XRD result clearly manifests the successful phase separation of the CoSe2 and MoSe2 from the CoMoO4 precursor via the selenization process.
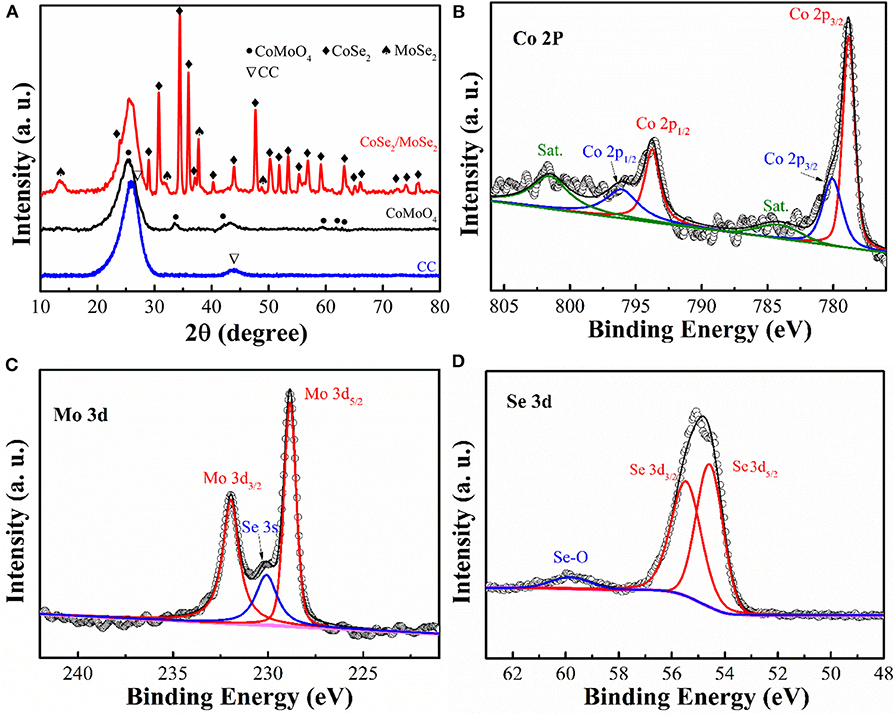
Figure 3. (A) XRD patterns of CC, CoMoO4, and CoSe2/MoSe2; high-resolution XPS spectra of (B) Mo 3d, (C) Co 2p, and (D) Se 3d of CoSe2/MoSe2.
X-ray photoelectron spectroscopy (XPS) measurement was carried out to analyze the composition and chemical state of as-prepared samples. (Figure 3B) illustrates the high-resolution Co 2p peaks at 778.8 eV (Co 2p3/2), 793.7 eV (Co 2p1/2), 780 eV (Co 2p3/2), and 796 eV (Co 2p1/2), corresponding to CoSe2 and cobalt–oxide bond, while those peaks at 784.1 eV and 801.5 eV are the satellite peaks (Mu et al., 2016; Wang et al., 2017b; Gao et al., 2018). Furthermore, the fine Mo 3d XPS spectrum (Figure 3C) shows the main peaks at 228.8 eV and 231.9 eV, which represent the Mo 3d5/2 and Mo 3d3/2 of MoSe2 (Wang et al., 2018a,b). Additionally, the peak located at 230 eV can be ascribed to the Se 3s of MoSe2 (Zhao et al., 2018). The Se 3d XPS spectrum (Figure 3D) displays the characteristic of CoSe2 and MoSe2 at 54.5 eV and 55.4 eV in agreement with the Se 3d5/2 and Se 3d3/2, respectively (Gao et al., 2018). Moreover, the peak at around 59.8 eV is confirmed to correspond to the selenium–oxygen bond (Kong et al., 2014). According to these results, the selenization process induced the phase separation from CoMoO4 into the nanoscale CoSe2 and MoSe2.
It is generally recognized that highly efficient electrocatalysts worked in alkaline solution is the bottleneck for large-scale application of overall water splitting. Linear sweep voltammetry (LSV) at a scanning rate of 5 mV s−1 was characterized by the electrocatalytic HER and OER capacities of the samples by a three-electrode system in 1 M KOH solution with saturated N2. By contrast, CoSe2, MoSe2 (Figure S1), RuO2, and Pt/C catalysts were performed in the same condition. The HER polarization curves and corresponding Tafel slops are depicted in (Figures 4A,B). The overpotential (η10) and Tafel slope of CoSe2/MoSe2 are 90 mV and 84.8 mV·dec−1, which are better than those of CoMoO4 (277 mV, 123.6 mV·dec−1), CoSe2 (205 mV, 195.2 mV·dec−1), and MoSe2 (199 mV, 152.4 mV·dec−1). CoSe2 has a metallic character, which can promote the dissociation of water and provide protons under alkaline conditions, thus improving the HER performance of MoSe2 (Kwak et al., 2016). In addition, the hierarchical nanosheet array assembled by the CoSe2/MoSe2 provides abundant active sites for the electrochemical reaction at the phase interface, which can further enhance the HER performance (Zhang et al., 2017a). Therefore, the CoSe2/MoSe2 catalyst exhibits improved HER performance benefiting from the synergistic effect. The catalyst of Pt/C illustrates an overpotential (η10) (59 mV) and Tafel slope (36.9 mV·dec−1) in 1 M KOH that are similar to those in other literatures (Chen et al., 2018b; Wan et al., 2018). Moreover, the overpotential of CoSe2/MoSe2 is superior to those of recently reported selenide catalysts such as NiSe NWs/Ni Foam (96 mV) (Tang et al., 2015), EG/cobalt selenide/NiFe–LDH (260 mV) (Hou et al., 2016), o-CoSe2/P (104 mV) (Zheng et al., 2018), CoSe2 NCs (520 mV) (Kwak et al., 2016), Co0.75Ni0.25Se/NF (106 mV) (Liu et al., 2019), 1T MoSe2/NiSe (120 mV) (Zhang et al., 2019), and SWCNTs/MoSe2 (219 mV) (Najafi et al., 2019) (Table S1).
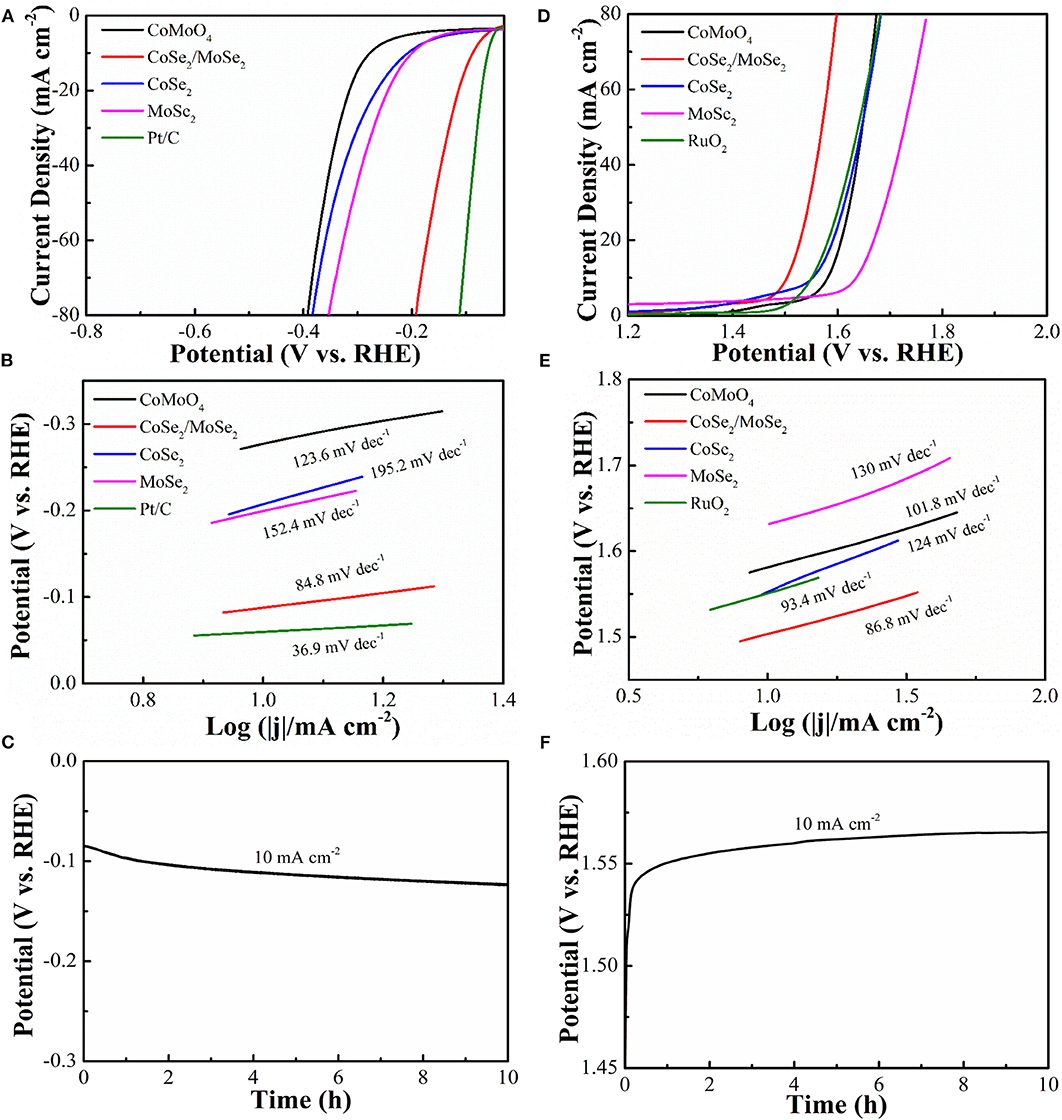
Figure 4. (A) HER polarization curves of CoMoO4, CoSe2/MoSe2, CoSe2, MoSe2, and Pt/C; (B) Tafel slopes; (C) galvanostatic of CoSe2/MoSe2 for HER; (D) OER polarization curves of CoMoO4, CoSe2/MoSe2, CoSe2, MoSe2, and RuO2; (E) Tafel slopes in OER; (F) galvanostatic of CoSe2/MoSe2 for OER.
The electrocatalytic OER properties are determined by LSV and polarization measurements as shown in (Figures 4D,E). The CoSe2/MoSe2 catalyst shows a lower overpotential (η10 of 280 mV) than those of the CoMoO4 (352 mV), CoSe2 (322 mV), MoSe2 (404 mV), and RuO2 (318 mV), respectively. More importantly, the OER performance of the designed CoSe2/MoSe2 sample exceeds those of recently reported selenide catalysts in OER, for instance, the Ag–CoSe2 (320 mV) (Zhao et al., 2017), CoSe2 NCs (430 mV) (Kwak et al., 2016), CoSe2/DETA (392 mV) (Guo et al., 2017), NiCo2Se4 holey nanosheets (295 mV) (Fang et al., 2017), NiSe–Ni0.85Se/CP (300 mV) (Chen et al., 2018a), SWCNTs/MoSe2 (295 mV) (Najafi et al., 2019), 1T/2H MoSe2 (397 mV) (Li et al., 2019), and CoSe2@MoSe2 (309 mV) (Chen et al., 2019c) (Table S2). Furthermore, the corresponding Tafel slope of CoSe2/MoSe2 is 86.8 mV·dec−1, which is smaller than those of the CoMoO4 (101.8 mV·dec−1), CoSe2 (124 mV·dec−1), MoSe2 (130 mV·dec−1), and RuO2 (93.4 mV·dec−1). The CoSe2/MoSe2 has lower overpotential and smaller Tafel, which can be attributed to its unique hierarchical heterostructure, facilitating electron transfer and accelerating OER kinetics. In this heterostructure, the transfer of electrons from CoSe2 phase to MoSe2 phase in the CoSe2/MoSe2 interface can result in electron-poor Co species and electron-rich Mo species (Liu et al., 2018). It is believed that the Se anion can affect the electron transfer between Co and Mo species, which is important for boosting catalytic ability (Yan et al., 2019). Besides, the formation of CoOOH is the primary cause to promote OER activity (Liu et al., 2015), and the increased 3d−4p repulsion between the center of the metal d band and the center of the p band of the Se site further promotes the rapid transfer of dioxygen molecules, thus improving OER performance (Li et al., 2017).
To understand the effects of the structure and composition of prepared catalyst on the electrochemical performance, several CoSe2/MoSe2 catalysts were collected at different selenization temperatures and the HER and OER performance were evaluated by LSV analysis (Figure S2). It can be seen that the CoSe2/MoSe2 sample obtained at 450°C (CoSe2/MoSe2-450) possesses better electrocatalytic properties than other counterparts, which can be ascribed to its superior structure. As shown in (Figure S3), with the selenization temperature increasing, the size of nanoparticles on the surface of nanosheets increased as well, indicating higher crystallinity. Generally, larger particle size will reduce the active surface of catalyst (Zhang et al., 2017b; Chen et al., 2019a). Therefore, when the selenization temperature elevated to 500°C (CoSe2/MoSe2-500), the catalytic performance slightly declined owing to its larger particle size and lower active surface. In addition, (Figure S4) displays the composition of the CoSe2/MoSe2 catalysts achieved at a different selenization temperature. As can be seen, when the selenization process proceeded at low temperature, the obtained CoSe2/MoSe2 catalyst has poor MoSe2 phase and low crystallinity, which are responsible for the poor electrochemical catalytic performance of the catalysts (CoSe2/MoSe2-350 and CoSe2/MoSe2-400). Therefore, the catalyst synthesized at 450°C shows the best performance, benefiting from the appropriate crystal structure and phase composition.
The electrochemically active surface area (ECSA) of as-prepared catalyst was evaluated by the double-layer capacitance (Cdl), which was measured by CV in a non-Faradaic reaction potential range (Deng et al., 2015). The Cdl values of the CoSe2/MoSe2 (1.6 mF cm−2) is higher than those of CoSe2 (0.63 mF cm−2) and MoSe2 (0.8 mF cm−2), as shown in (Figure 5), suggesting more active sites of the CoSe2/MoSe2 catalyst. Furthermore, the smaller Rct value for the CoSe2/MoSe2 catalyst in the EIS measurement (Figure S5) implies the promoted charge transfer and boosted kinetics, which can be ascribed to the abundant interfaces and synergetic effect between the CoSe2 and MoSe2.
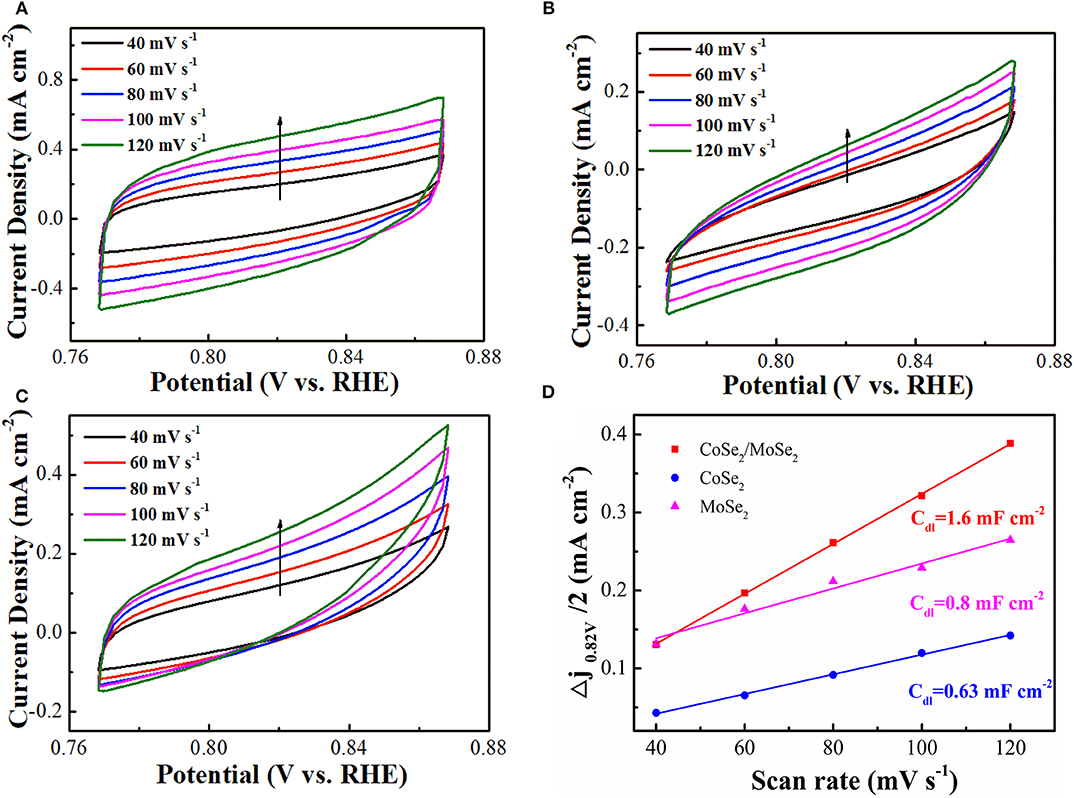
Figure 5. Electrochemical double-layer capacitance with the CV curves acquired at different scanning rates from 40, 60, 80, 100, and 120 mV s−1: (A) CoSe2/MoSe2, (B) CoSe2, and (C) MoSe2; (D) current densities (Δj = janode – jcathode, at 0.82 V) as a function of scanning rates of CoSe2/MoSe2, CoSe2, and MoSe2 with the corresponding slope being twice that of the Cdl values.
The structural stability is another significant parameter for catalysts in HER and OER. (Figures 4C,F) show a galvanostatic for CoSe2/MoSe2 catalyst in both the HER and OER processes. The morphology and composition of the catalyst after galvanostatic cycling are characterized by SEM and XPS. The CoSe2/MoSe2 could well inherit the pristine sheet-like structure, demonstrating good structural stability. In addition, the fine XPS spectra of the Co 2p, Mo 3d, Se 3d acquired from the sample of CoSe2/MoSe2 after galvanostatic measurement confirm the reservation of CoSe2 and MoSe2 (Figure S6), indicating phase stability during the electrochemical reactions.
To investigate its practical application of the obtained catalyst, an overall water splitting electrolyzer is assembled with CoSe2/MoSe2 as electrodes in 1 M KOH. It can decompose water at a low cell voltage of 1.63 V (current density at 10 mA·cm−2) (Figure 6A), and the efficiency is similar to those constituting of the noble-metal-based cathode and anode (RuO2 vs. Pt/C). Moreover, the overall water splitting performance of the CoSe2/MoSe2 is better than those of other recently reported non-noble metals at the same current density, such as (Ni,Co)0.85Se NSAs (1.65 V) (Xiao et al., 2018), a-CoSe/Ti mesh (1.65 V) (Liu et al., 2015), CoOx-CoSe (1.64 V) (Xu et al., 2016), Co0.85Se@NC (1.76 V) (Meng et al., 2017), CoB2/CoSe2 (1.73 V) (Guo et al., 2017), NiSe2/Ni (1.64 V) (Zhang et al., 2018), 1T/2H MoSe2/MXene (1.64 V) (Li et al., 2019), and Ni3Se2/CF (1.65 V) (Shi et al., 2015) (Table S3). Additionally, CoSe2/MoSe2 electrolyzer exhibits a slight increase in the potential after being cycled for 12 h in alkaline solution (Figure 6B).
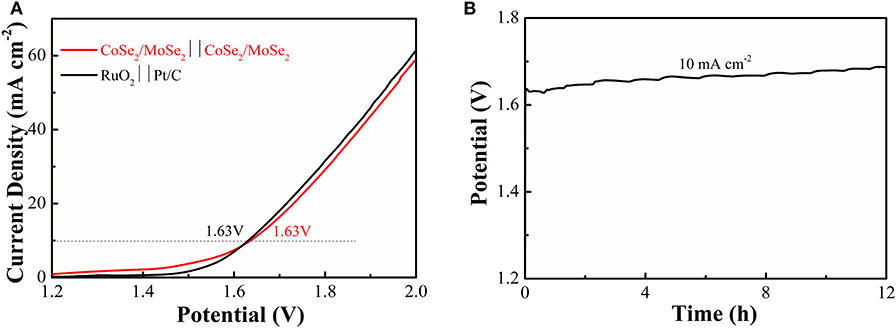
Figure 6. (A) LSV curves of water splitting with CoSe2/MoSe2 as the anode and cathode; (B) galvanostatic testing of the CoSe2/MoSe2-based water splitting electrolyzer for 12 h at 10 mA cm−2.
Conclusion
In summary, a novel hierarchical 0D−2D Co/Mo selenide was developed by a facile in situ phase separation strategy. Benefiting from its unique structure and composition, the constructed CoSe2/MoSe2 catalyst exhibits small η10 of 280 mV and 90 mV and Tafel slopes of 86.8 mV·dec−1 and 84.8 mV·dec−1 for OER and HER, respectively. Furthermore, the electrolyzer comprising CoSe2/MoSe2 as the bifunctional catalyst shows a small water splitting cell voltage of 1.63 V at a current density of 10 mA·cm−2. This work provides insights into rational design and development of economical and valid bifunctional catalysts for overall water splitting.
Data Availability Statement
All datasets generated for this study are included in the article/Supplementary Material.
Author Contributions
LX implemented the experiment, analyzed the data and wrote the article. HS, XL, XZ, and BG participated in the formulation of the experimental scheme. YZ, KH, and PC revised the article.
Funding
This work was financially supported by the National Natural Science Foundation of China (Nos. 51572100, 61434001, and 31500783).
Conflict of Interest
The authors declare that the research was conducted in the absence of any commercial or financial relationships that could be construed as a potential conflict of interest.
Supplementary Material
The Supplementary Material for this article can be found online at: https://www.frontiersin.org/articles/10.3389/fchem.2020.00382/full#supplementary-material
References
Amiinu, I. S., Pu, Z., Liu, X., Owusu, K. A., Monestel, H. G. R., Boakye, F. O., et al. (2017). Multifunctional Mo–N/C@MoS2 electrocatalysts for her, oer, orr, and Zn–air batteries. Adv. Funct. Mater. 27:1702300. doi: 10.1002/adfm.201702300
Chen, J., Ge, Y., Feng, Q., Zhuang, P., Chu, H., Cao, Y., et al. (2019a). Nesting Co3Mo binary alloy nanoparticles onto molybdenum oxide nanosheet arrays for superior hydrogen evolution reaction. ACS Appl. Mater. Interfaces 11, 9002–9010. doi: 10.1021/acsami.8b19148
Chen, J., Pan, A., Wang, Y., Cao, X., Zhang, W., Kong, X., et al. (2019b). Hierarchical mesoporous MoSe2@CoSe/N-doped carbon nanocomposite for sodium ion batteries and hydrogen evolution reaction applications. Energy Storage Mater. 21, 97–106. doi: 10.1016/j.ensm.2018.10.019
Chen, Y., Ren, Z., Fu, H., Zhang, X., Tian, G., and Fu, H. (2018a). NiSe-Ni0.85Se heterostructure nanoflake arrays on carbon paper as efficient electrocatalysts for overall water splitting. Small 14:1800763. doi: 10.1002/smll.201800763
Chen, Z., Wang, W., Huang, S., Ning, P., Wu, Y., Gao, C., et al. (2019c). Well-defined CoSe2@MoSe2 hollow heterostructured nanocubes with enhanced dissociation kinetics for overall water splitting. Nanoscale 12, 326–335. doi: 10.1039/C9NR08751
Chen, Z., Wu, R., Liu, Y., Ha, Y., Guo, Y., Sun, D., et al. (2018b). Ultrafine Co nanoparticles encapsulated in carbon-nanotubes-grafted graphene sheets as advanced electrocatalysts for the hydrogen evolution reaction. Adv. Mater. 30:1802011. doi: 10.1002/adma.201802011
Deng, Z. H., Li, L., Ding, W., Xiong, K., and Wei, Z. D. (2015). Synthesized ultrathin MoS2 nanosheets perpendicular to graphene for catalysis of hydrogen evolution reaction. Chem. Commun. 51, 1893–1896. doi: 10.1039/C4CC08491H
Fang, Z., Peng, L., Lv, H., Zhu, Y., Yan, C., Wang, S., et al. (2017). Metallic transition metal selenide holey nanosheets for efficient oxygen evolution electrocatalysis. ACS Nano 11, 9550–9557. doi: 10.1021/acsnano.7b05481
Gao, J., Li, Y., Shi, L., Li, J., and Zhang, G. (2018). Rational design of hierarchical nanotubes through encapsulating CoSe2 nanoparticles into MoSe2/C composite shells with enhanced lithium and sodium storage performance. ACS Appl. Mater. Interfaces 10, 20635–20642. doi: 10.1021/acsami.8b06442
Guo, Y., Yao, Z., Shang, C., and Wang, E. (2017). Amorphous Co2B grown on CoSe2 nanosheets as a hybrid catalyst for efficient overall water splitting in alkaline medium. ACS Appl. Mater. Interfaces 9, 39312–39317. doi: 10.1021/acsami.7b10605
Hou, Y., Lohe, M. R., Zhang, J., Liu, S., Zhuang, X., and Feng, X. (2016). Vertically oriented cobalt selenide/NiFe layered-double-hydroxide nanosheets supported on exfoliated graphene foil: an efficient 3D electrode for overall water splitting. Energy Environ. Sci. 9, 478–483. doi: 10.1039/C5EE03440J
Jamesh, M. I., and Sun, X. (2018). Recent progress on earth abundant electrocatalysts for oxygen evolution reaction (OER) in alkaline medium to achieve efficient water splitting-a review. J. Power Sources 400, 31–68. doi: 10.1016/j.jpowsour.2018.07.125
Jaramillo, T. F., Jørgensen, K. P., Bonde, J., Nielsen, J. H., Horch, S., and Chorkendorff, I. (2007). Identification of active edge sites for electrochemical H2 evolution from MoS2 nanocatalysts. Science 317:100. doi: 10.1126/science.1141483
Kong, D., Wang, H., Lu, Z., and Cui, Y. (2014). CoSe2 nanoparticles grown on carbon fiber paper: an efficient and stable electrocatalyst for hydrogen evolution reaction. J. Am. Chem. Soc. 136, 4897–4900. doi: 10.1021/ja501497n
Kwak, I. H., Im, H. S., Jang, D. M., Kim, Y. W., Park, K., Lim, Y. R., et al. (2016). CoSe2 and NiSe2 nanocrystals as superior bifunctional catalysts for electrochemical and photoelectrochemical water splitting. ACS Appl. Mater. Interfaces 8, 5327–5334. doi: 10.1021/acsami.5b12093
Lai, F., Yong, D., Ning, X., Pan, B., Miao, Y. E., and Liu, T. (2017). Bionanofiber assisted decoration of few-layered MoSe2 nanosheets on 3D conductive networks for efficient hydrogen evolution. Small 13:1602866. doi: 10.1002/smll.201602866
Li, N., Zhang, Y., Jia, M., Lv, X., Li, X., Li, R., et al. (2019). 1T/2H MoSe2-on-MXene heterostructure as bifunctional electrocatalyst for efficient overall water splitting. Electrochim. Acta 326:134976. doi: 10.1016/j.electacta.2019.134976
Li, W., Gao, X., Xiong, D., Wei, F., Song, W. G., Xu, J., et al. (2017). Hydrothermal synthesis of monolithic Co3Se4 nanowire electrodes for oxygen evolution and overall water splitting with high efficiency and extraordinary catalytic stability. Adv. Energy Mater. 7:1602579. doi: 10.1002/aenm.201602579
Liu, C., Wang, K., Zheng, X., Liu, X., Liang, Q., and Chen, Z. (2018). Rational design of MoSe2-NiSe@carbon heteronanostructures for efficient electrocatalytic hydrogen evolution in both acidic and alkaline media. Carbon 139, 1–9. doi: 10.1016/j.carbon.2018.06.034
Liu, S., Jiang, Y., Yang, M., Zhang, M., Guo, Q., Shen, W., et al. (2019). Highly conductive and metallic cobalt-nickel selenide nanorods supported on Ni foam as an efficient electrocatalyst for alkaline water splitting. Nanoscale 11, 7959–7966. doi: 10.1039/C8NR10545F
Liu, T., Liu, Q., Asiri, A. M., Luo, Y., and Sun, X. (2015). An amorphous CoSe film behaves as an active and stable full water-splitting electrocatalyst under strongly alkaline conditions. Chem. Commun. 51, 16683–16686. doi: 10.1039/C5CC06892
Liu, X., Liu, Y., and Fan, L. Z. (2017). MOF-derived CoSe2 microspheres with hollow interiors as high-performance electrocatalysts for the enhanced oxygen evolution reaction. J. Mater. Chem. A 5, 15310–15314. doi: 10.1039/C7TA04662F
Luo, Y., Li, X., Cai, X., Zou, X., Kang, F., Cheng, H. M., et al. (2018). Two-dimensional MoS2 confined Co(OH)2 electrocatalysts for hydrogen evolution in alkaline electrolytes. ACS Nano 12, 4565–4573. doi: 10.1021/acsnano.8b00942
Mao, S., Wen, Z., Ci, S., Guo, X., Ostrikov, K., and Chen, J. (2015). Perpendicularly oriented MoSe2/graphene nanosheets as advanced electrocatalysts for hydrogen evolution. Small 11, 414–419. doi: 10.1002/smll.201401598
Meng, T., Qin, J., Wang, S., Zhao, D., Mao, B., and Cao, M. (2017). In situ coupling of Co0.85Se and N-doped carbon via one-step selenization of metal-organic frameworks as a trifunctional catalyst for overall water splitting and Zn-air batteries. J. Mater. Chem. A 5, 7001–7014. doi: 10.1039/C7TA01453H
Mu, C. H., Qi, H. X., Song, Y. Q., Liu, Z. P., Ji, L. X., Deng, J. G., et al. (2016). One-pot synthesis of nanosheet-assembled hierarchical MoSe2/CoSe2 microcages for the enhanced performance of electrocatalytic hydrogen evolution. RSC Adv. 6, 23–30. doi: 10.1039/C5RA21638A
Najafi, L., Bellani, S., Oropesa-Nuñez, R., Prato, M., Martín-García, B., Brescia, R., et al. (2019). Carbon nanotube-supported MoSe2 holey flake:Mo2C ball hybrids for bifunctional pH-universal water splitting. ACS Nano 13, 3162–3176. doi: 10.1021/acsnano.8b08670
Qu, B., Li, C., Zhu, C., Wang, S., Zhang, X., and Chen, Y. (2016). Growth of MoSe2 nanosheets with small size and expanded spaces of (002) plane on the surfaces of porous N-doped carbon nanotubes for hydrogen production. Nanoscale 8, 16886–16893. doi: 10.1039/C6NR04619C
Qu, B., Yu, X., Chen, Y., Zhu, C., Li, C., Yin, Z., et al. (2015). Ultrathin MoSe2 nanosheets decorated on carbon fiber cloth as binder-free and high-performance electrocatalyst for hydrogen evolution. ACS Appl. Mater. Interfaces 7, 14170–14175. doi: 10.1021/acsami.5b02753
Shi, J., Hu, J., Luo, Y., Sun, X., and Asiri, A. M. (2015). Ni3Se2 film as a non-precious metal bifunctional electrocatalyst for efficient water splitting. Catal. Sci. Technol. 5, 4954–4958. doi: 10.1039/C5CY01121C
Tang, C., Cheng, N., Pu, Z., Xing, W., and Sun, X. (2015). NiSe nanowire film supported on nickel foam: an efficient and stable 3D bifunctional electrode for full water splitting. Angew. Chem. Int. Ed. 54, 9351–9355. doi: 10.1002/anie.201503407
Tang, H., Dou, K., Kaun, C. C., Kuang, Q., and Yang, S. (2014). MoSe2 nanosheets and their graphene hybrids: synthesis, characterization and hydrogen evolution reaction studies. J. Mater. Chem. A 2, 360–364. doi: 10.1039/C3TA13584E
Tang, Q., and Jiang, D. E. (2016). Mechanism of hydrogen evolution reaction on 1T-MoS2 from first principles. ACS Catal. 6, 4953–4961. doi: 10.1021/acscatal.6b01211
Trasatti, S. (1972). Work function, electronegativity, and electrochemical behaviour of metals: iii. electrolytic hydrogen evolution in acid solutions. J. Electroanal. Chem. 39, 163–184. doi: 10.1016/S0022-0728(72)80485-6
Trasatti, S. (1984). Electrocatalysis in the anodic evolution of oxygen and chlorine. Electrochim. Acta 29, 1503–1512. doi: 10.1016/0013-4686(84)85004-5
Wan, S., Jin, W., Guo, X., Mao, J., Zheng, L., Zhao, J., et al. (2018). Self-templating construction of porous CoSe2 nanosheet arrays as efficient bifunctional electrocatalysts for overall water splitting. ACS Sustain. Chem. Eng. 6, 15374–15382. doi: 10.1021/acssuschemeng.8b03804
Wang, B., Li, S., Wu, X., Liu, J., Tian, W., and Chen, J. (2016). Self-assembly of ultrathin mesoporous CoMoO4 nanosheet networks on flexible carbon fabric as a binder-free anode for lithium-ion batteries. New J. Chem. 40, 2259–2267. doi: 10.1039/c5nj02910d
Wang, B., Wang, Z., Wang, X., Zheng, B., Zhang, W., and Chen, Y. (2018a). Scalable synthesis of porous hollow CoSe2-MoSe2/carbon microspheres for highly efficient hydrogen evolution reaction in acidic and alkaline media. J. Mater. Chem. A 6, 12701–12707. doi: 10.1039/C8TA03523G
Wang, C., Zhang, P., Lei, J., Dong, W., and Wang, J. (2017a). Integrated 3D MoSe2@Ni0.85Se nanowire network with synergistic cooperation as highly efficient electrocatalysts for hydrogen evolution reaction in alkaline medium. Electrochim. Acta 246, 712–719. doi: 10.1016/j.electacta.2017.06.028
Wang, P., Pu, Z., Li, W., Zhu, J., Zhang, C., Zhao, Y., et al. (2019). Coupling NiSe2-Ni2P heterostructure nanowrinkles for highly efficient overall water splitting. J. Catal. 377, 600–608. doi: 10.1016/j.jcat.2019.08.005
Wang, X., Li, F., Li, W., Gao, W., Tang, Y., and Li, R. (2017b). Hollow bimetallic cobalt-based selenide polyhedrons derived from metal-organic framework: an efficient bifunctional electrocatalyst for overall water splitting. J. Mater. Chem. A 5, 17982–17989. doi: 10.1039/C7TA03167J
Wang, X., Zheng, B., Yu, B., Wang, B., Hou, W., Zhang, W., et al. (2018b). In situ synthesis of hierarchical MoSe2-CoSe2 nanotubes as an efficient electrocatalyst for the hydrogen evolution reaction in both acidic and alkaline media. J. Mater. Chem. A 6, 7842–7850. doi: 10.1039/C8TA01552J
Wu, K., Zhan, J., Xu, G., Zhang, C., Pan, D., and Wu, M. (2018). MoO3 nanosheet arrays as superior anode materials for Li- and Na-ion batteries. Nanoscale 10, 16040–16049. doi: 10.1039/C8NR03372B
Xiao, K., Zhou, L., Shao, M., and Wei, M. (2018). Fabrication of (Ni,Co)0.85Se nanosheet arrays derived from layered double hydroxides toward largely enhanced overall water splitting. J. Mater. Chem. A 6, 7585–7591. doi: 10.1039/C8TA01067F
Xie, J., Zhang, H., Li, S., Wang, R., Sun, X., Zhou, M., et al. (2013). Defect-rich MoS2 ultrathin nanosheets with additional active edge sites for enhanced electrocatalytic hydrogen evolution. Adv. Mater. 25, 5807–5813. doi: 10.1002/adma.201302685
Xu, X., Du, P., Chen, Z., and Huang, M. (2016). An electrodeposited cobalt-selenide-based film as an efficient bifunctional electrocatalyst for full water splitting. J. Mater. Chem. A 4, 10933–10939. doi: 10.1039/C6TA03788G
Xue, X., Zhang, J., Saana, I. A., Sun, J., Xu, Q., and Mu, S. (2018). Rational inert-basal-plane activating design of ultrathin 1T′ phase MoS2 with a MoO3 heterostructure for enhancing hydrogen evolution performances. Nanoscale 10, 16531–16538. doi: 10.1039/C8NR05270K
Yan, H., Xie, Y., Wu, A., Cai, Z., Wang, L., Tian, C., et al. (2019). Anion-modulated HER and OER activities of 3D Ni-V-based interstitial compound heterojunctions for high-efficiency and stable overall water splitting. Adv. Mater. 31:1901174. doi: 10.1002/adma.201901174
Yang, L. J., Deng, Y. Q., Zhang, X. F., Liu, H., and Zhou, W. J. (2018). MoSe2 nanosheet/MoO2 nanobelt/carbon nanotube membrane as flexible and multifunctional electrodes for full water splitting in acidic electrolyte. Nanoscale 10, 9268–9275. doi: 10.1039/C8NR01572D
Yang, X., Lu, A. Y., Zhu, Y., Hedhili, M. N., Min, S., Huang, K. W., et al. (2015). CoP nanosheet assembly grown on carbon cloth: a highly efficient electrocatalyst for hydrogen generation. Nano Energy 15, 634–641. doi: 10.1016/j.nanoen.2015.05.026
Zhang, J., Wang, Y., Zhang, C., Gao, H., Lv, L., Han, L., et al. (2018). Self-supported porous NiSe2 nanowrinkles as efficient bifunctional electrocatalysts for overall water splitting. ACS Sustainable Chem. Eng. 6, 2231–2239. doi: 10.1021/acssuschemeng.7b03657
Zhang, L., Wang, T., Sun, L., Sun, Y., Hu, T., Xu, K., et al. (2017a). Hydrothermal synthesis of 3D hierarchical MoSe2/NiSe2 composite nanowires on carbon fiber paper and their enhanced electrocatalytic activity for the hydrogen evolution reaction. J. Mater. Chem. A 5, 19752–19759. doi: 10.1039/C7TA05352E
Zhang, Q., Li, P., Zhou, D., Chang, Z., Kuang, Y., and Sun, X. (2017b). Superaerophobic ultrathin Ni-Mo alloy nanosheet array from in situ topotactic reduction for hydrogen evolution reaction. Small 13:1701648. doi: 10.1002/smll.201701648
Zhang, X., Zhang, Y. Y., Zhang, Y., Jiang, W. J., Zhang, Q. H., Yang, Y. G., et al. (2019). Phase-controlled synthesis of 1T-MoSe2/NiSe heterostructure nanowire arrays via electronic injection for synergistically enhanced hydrogen evolution. Small Methods 3:1800317. doi: 10.1002/smtd.201800317
Zhao, G., Li, P., Rui, K., Chen, Y., Dou, S. X., and Sun, W. (2018). CoSe2/MoSe2 heterostructures with enriched water adsorption/dissociation sites towards enhanced alkaline hydrogen evolution reaction. Chem. Eur. J. 24, 11158–11165. doi: 10.1002/chem.201801693
Zhao, X., Zhang, H., Yan, Y., Cao, J., Li, X., Zhou, S., et al. (2017). Engineering the electrical conductivity of lamellar silver-doped cobalt(II) selenide nanobelts for enhanced oxygen evolution. Angew. Chem. Int. Ed. 56, 328–332. doi: 10.1002/anie.201609080
Zheng, Y. R., Wu, P., Gao, M. R., Zhang, X. L., Gao, F. Y., Ju, H. X., et al. (2018). Doping-induced structural phase transition in cobalt diselenide enables enhanced hydrogen evolution catalysis. Nat. Commun. 9:2533. doi: 10.1038/s41467-018-04954-7
Keywords: cobalt selenide, molybdenum selenide, hierarchical heterostructure, bifunctional electrocatalysts, overall water splitting
Citation: Xia L, Song H, Li X, Zhang X, Gao B, Zheng Y, Huo K and Chu PK (2020) Hierarchical 0D−2D Co/Mo Selenides as Superior Bifunctional Electrocatalysts for Overall Water Splitting. Front. Chem. 8:382. doi: 10.3389/fchem.2020.00382
Received: 02 March 2020; Accepted: 14 April 2020;
Published: 19 May 2020.
Edited by:
Guanglin Xia, Fudan University, ChinaReviewed by:
Shichun Mu, Wuhan University of Technology, ChinaFei Ma, Xi'an Jiaotong University, China
Copyright © 2020 Xia, Song, Li, Zhang, Gao, Zheng, Huo and Chu. This is an open-access article distributed under the terms of the Creative Commons Attribution License (CC BY). The use, distribution or reproduction in other forums is permitted, provided the original author(s) and the copyright owner(s) are credited and that the original publication in this journal is cited, in accordance with accepted academic practice. No use, distribution or reproduction is permitted which does not comply with these terms.
*Correspondence: Xuming Zhang, eHVtemhhbmcmI3gwMDA0MDt3dXN0LmVkdS5jbg==; Yang Zheng, eXpoZW5nJiN4MDAwNDA7d3VzdC5lZHUuY24=