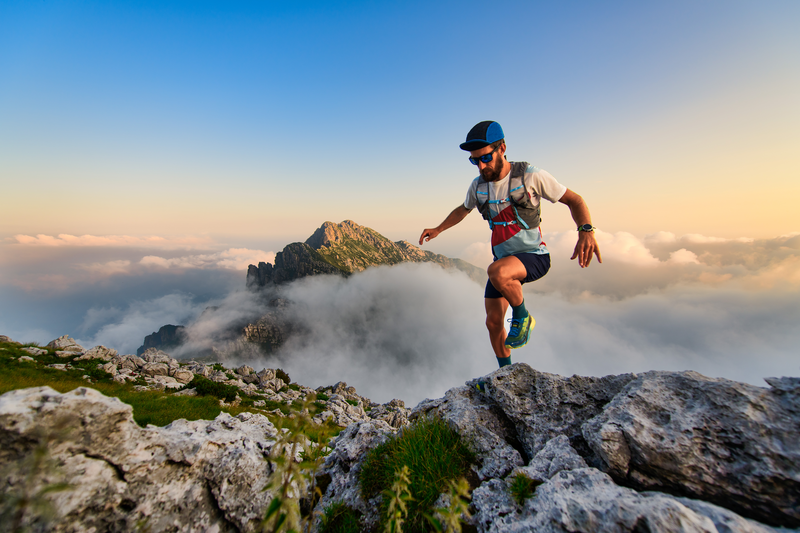
94% of researchers rate our articles as excellent or good
Learn more about the work of our research integrity team to safeguard the quality of each article we publish.
Find out more
ORIGINAL RESEARCH article
Front. Chem. , 05 May 2020
Sec. Catalysis and Photocatalysis
Volume 8 - 2020 | https://doi.org/10.3389/fchem.2020.00334
This article is part of the Research Topic Catalysis & Photocatalysis Editor’s Pick 2021 View all 14 articles
It is highly promising to design and develop efficient and economical electrocatalysts for oxygen evolution reaction (OER) in alkaline solution. In this work, we prepare FeCoNi sulfide composites (including FeS, Co3S4, and Ni3S4) derived from in situ sulfurization of precursor oxides on carbon cloth (CC), which are used to become an OER catalyst. Such catalyst shows excellent OER performance, low overpotential, small Tafel slope, and high electrochemical stability, and it is a promising electrocatalyst for OER in alkaline media.
The excessive consumption of fossil energy and the resulting serious environmental problems have triggered strong demand for renewable alternative energy (Chow et al., 2003; Zheng et al., 2014). Hydrogen energy is regarded as a clean and ideal energy carrier that could replace fossil energy (Dresselhaus and Thomas, 2001; Zheng et al., 2017, 2018b). Electrochemical water splitting provides us a promising strategy to largely produce hydrogen (Turner, 2004; Lu et al., 2017). However, hydrogen evolution is seriously restricted by anodic water oxidation due to the multi-electron transfer process and high activation energy barrier (Yin et al., 2010; Yang et al., 2017; Ke et al., 2018; Zheng et al., 2018a; Tang et al., 2019). Therefore, efficient catalysts to reduce activation energy should be developed to boost the water oxidation process. Noble metal oxides (RuO2 and IrO2) exhibit excellent catalytic characters in oxygen evolution reaction (OER), but their widespread applications are limited due to scarce resources and high costs (Lee et al., 2012; Reier et al., 2012). Hence, it is necessary to develop efficient and economical OER electrocatalysts.
In recent years, transition-metal oxides and hydroxyl oxide have attracted great interest for catalysts (Lu et al., 2016; Guo et al., 2017; Zhang et al., 2017; Jin et al., 2018; Zhao et al., 2018). Specifically, ferric oxyhydroxide (FeOOH) has shown efficient activity for the OER process (Chemelewski et al., 2014; Luo et al., 2017; Park et al., 2017). Regardless of its abundant reserves and low cost, its performance for OER has certain disparities in comparing with the noble metal catalysts. Many ways have been taken to improve catalysis performance, such as enhancing the conductivity of materials, increasing the specific surface area of materials, doping heteroatom modification, and so on (Feng et al., 2016a,b; Kuang et al., 2017; Li F. et al., 2018). Research shows that transition metal sulfides have better oxygen evolution catalysis performance than oxides because transit metal sulfides have diverse element composition, controllable electronic structure, and fast charge transfer speed (Liu et al., 2016; Chai et al., 2018; Li H. et al., 2018; Zhang et al., 2018).
In this manuscript, we design and develop FeCoNiS sulfides derived from in situ sulfurization of precursor oxides on carbon cloth (CC) through two-step hydrothermal methods. At first, FeCoNi-FeOOH nanoarray on CC is prepared through hydrothermal method. Secondly FeCoNiS sulfides derived from in-situ sulfurization is prepared through the second hydrothermal method. It shows excellent OER activity needing overpotentials of 220.5 and 269.9 mV to attain current densities of 10 and 100 mA cm−2 in 1.0 M KOH. It is a promising electrocatalyst for OER in alkaline media.
X-ray diffraction (XRD) patterns of these catalysts are shown in Figure 1A. There are two broad diffraction peaks on the bottom curve, which are the amorphous peaks of CC. The middle curve is the XRD pattern of the precursor oxides without sulfide treatment. The peaks at 11.95, 16.87, 26.91, 35.26, 46.69, 56.21, and 64.78° can be indexed to the (110), (200), (310), (211), (411), (521), and (541) planes of FeOOH phase (PDF No. 97-003-1136). The XRD pattern of the product after sulfide treatment is on the top. The diffraction peak intensity is obviously lower than that of the middle curve. For better structural analysis of the product, the powder of the precursor oxides and sulfide products scrapped from CC are characterized by XRD again. XRD curves are shown in Figures 1B,C. The main component of precursor oxides is still FeOOH. Considered that Co and Ni are in the precursor, we name the precursor as FeCoNi-FeOOH. The XRD curve of sulfide product shows that there are new phases, including Fe3O4 (PDF No.97-005-0272), FeS (PDF No. 04-003-4477), Ni3S4 (PDF No. 97-003-6721), and Co3S4 (PDF No. 00-047-1738). Part of FeOOH is reduced to Fe3O4, so the product is named FeCoNiS-FeOx.
Figure 1. (A) X-ray diffraction (XRD) patterns of different oxygen evolution reaction (OER) catalysts. (B) XRD patterns comparison of different catalysts powder. (C) XRD pattern of the product after sulfidation treatment.
Scanning electronic microscopy (SEM) pattern of the precursor (FeCoNi-FeOOH) is shown in Figure 2A. There are specific and uniform nanowires array on the surface of CC. SEM pattern of FeCoNiS-FeOx is shown in Figure 2B. Obviously, the precursor (FeCoNi-FeOOH) nanowires are smooth, and the product (FeCoNiS-FeOx) is relatively rough. This means that the product has structural change after sulfidation, which corresponded to the XRD patterns in Figures 1B,C. The catalyst nanowire feature is also shown in transmission electron microscopy (TEM) characterization (Figure 2C). Image taken from the product shows about 50-nm-thick nanowires. High-resolution TEM (HRTEM) reveals that the product is highly crystallized with well-resolved lattice fringes (Figure 2D). The interplanar spacing of 0.331 nm could be assigned to the (310) plane of FeOOH.
Figure 2. (A) SEM pattern of precursor (FeCoNi-FeOOH). (B) SEM pattern of FeCoNiS-FeOx. (C) TEM pattern of FeCoNiS-FeOx. (D) HRTEM pattern of FeCoNiS-FeOx.
The corresponding energy-dispersive X-ray (EDX) elemental mapping images of FeCoNiS-FeOx are shown in Figure 3A, which demonstrate unique distribution of Fe, Co, Ni, and S elements. EDX pattern is shown in Figure 3B, which exhibits types and relative amounts of different elements based on the position and intensity of element spectral lines.
Figure 3. (A) Energy-dispersive X-ray (EDX) mapping images of FeCoNiS-FeOx. (B) EDX element distribution pattern.
X-ray photoelectron spectroscopy (XPS) of FeCoNiS-FeOOH is shown in Figure 4, which was performed to characterize the chemical states of different elements. Figure 4A is full-scale XPS spectrum, further revealing the presences of Fe, Co, Ni, S, and O elements in the catalyst. As shown in Figure 4B, high-spin Fe3+ of FeOOH contains unpaired electrons and therefore exhibit multiplet structures in Fe 2p3/2 area. The characteristic peaks of Fe 2p1/2 is at 725.8 eV. The satellite peaks (identified as “Sat.”) are at 719.3 and 732.3 eV, which are relevant to Fe 2p3/2 and Fe 2p1/2 of FeOOH (Biesinger et al., 2011; Zeng et al., 2012; Zhou et al., 2018). There are two peaks at 714.4 and 723.9 eV, which are relevant to Fe3O4. In Figure 4C, there exhibit two spin-orbit doublets. The first doublet is at 778.6 and 793.5 eV, assigned to Co 2p3/2 and Co 2p1/2 of Co3+, and the second doublet was at 781.9 and 797.8 eV, arising from Co 2p3/2 and Co 2p1/2 of Co2+. In addition, two broad peaks located at 803.6 and 786.7 eV are attributed to the satellites, which indicated the presence of Co3S4 (Xiao et al., 2014; Liu et al., 2015; Gao et al., 2018; Wang X. et al., 2018).
Figure 4. X-ray photoelectron spectroscopy (XPS) spectra of FeCoNiS-FeOx catalyst. (A) Survey spectrum. (B) Fe 2p. (C) Co 2p. (D) Ni 2p. (E) S 2p. (F) O 1s.
In the Ni 2p spectrum (Figure 4D), there exist two main peaks at 855.7 and 873.5 eV assignable, respectively to Ni 2p3/2 and Ni 2p1/2 spin orbit doublets and two satellite peaks (862.4 and 880.1 eV). By deconvolution of the two main peaks, the Ni 2p3/2 orbit comprises two peaks with binding energy of 853.6 and 856.7 eV, which corresponded, respectively to the Ni2+ and Ni3+ oxidation states, and the Ni 2p1/2 orbit can also be fitted into two peaks belonging to Ni2+ (871.5 eV) and Ni3+ (875.2 eV) (Hu et al., 2015; Qin et al., 2016; Sivanantham et al., 2016). There show S 2p3/2 and S 2p1/2 peaks at 161.7 and 162.8 eV in Figure 4E, which can be related to S2− (Wang H. et al., 2018). The component peak at 164.1 eV is characteristics of a metal–sulfur (M-S) bond (Ning et al., 2018). The peaks of 168.9 and 170.1 eV can be attributed to due to air oxidation (Cheng et al., 2015). In the O 1s region (Figure 4F), the peaks of 530.0, 531.9, and 533.8 eV are observed on the surface of the catalyst, which are corresponding to O2−, hydroxyl group, and adsorbed water molecules, respectively (Luo et al., 2017).
The catalysis performance of the catalyst in water oxidation reaction is evaluated by linear sweep voltammetry (LSV), shown in Figure 5A. For comparison, LSV curves of different catalysts with similar loading amounts, including CC, RuO2, FeCoNi-FeOOH, FeCoNi-FeOx, FeCoNiS-FeOx, are also evaluated. Overpotentials in the same current density are often used to estimate the OER performance. FeCoNiS-FeOOH/CC exhibits outstanding OER performance with driving 100 mA cm−2 at a low overpotential of 269.9 mV, which is superior to RuO2/CC and FeCoNi-FeOOH under the same conditions. FeCoNi-FeOx is prepared through second hydrothermal method without sulfurizing reagent. The OER activity of FeCoNi-FeOx is lower than FeCoNi-FeOOH. The existence of Fe3O4 will not enhance catalytic reactivity. The main active site of FeCoNiS-FeOx is FeCoNi sulfides. Tafel plots of different OER catalysts were shown in Figure 5B, which are used to evaluate the catalytic kinetics. The Tafel slope of FeCoNiS-FeOx is 45.1 mV dec−1, lower than that of RuO2/CC (52.3 mV dec−1). It demonstrates that the FeCoNiS-FeOx catalyst has more rapid reaction velocity in OER catalytic reaction.
Figure 5. (A) Linear sweep voltammetry (LSV) curves of different oxygen evolution reaction (OER) catalysts. (B) Tafel curves of different OER catalysts. (C) LSV curve of FeCoNiS-FeOx catalyst and another curve after 500 CV cycles. (D) Time-dependent current density curve of FeCoNiS-FeOx OER catalyst under constant potential.
Another important performance, stability of catalyst, is investigated by cyclic voltammetry and potentiostatic method. As shown in Figure 5C, there shows a comparison of two polarization curves, including original curve and another curve after 500 CV cycles. When the potential is 1.5 V, the current density is only 3% decrease after 500 cycles, which demonstrates that the FeCoNiS-FeOx catalyst has a good cycle life. In Figure 5D, the electrochemical stability of FeCoNiS-FeOOH/CC is tested by potentiostatic electrolysis at a constant potential of 1.48 V for 30 h. There is only 5% decay of current density, which demonstrated the good long-term durability of the catalyst. Multistep chronopotentiometric curve of FeCoNiS-FeOx is shown in Figure 6. There are 12 steps and the increment of current density is 20 mA cm−2 per 500 s. In every step, the corresponding potential remains constant. These results indicate that the catalyst has excellent conductivity and good mass transportation.
Figure 6. Multistep chronopotentiometric of oxygen evolution reaction (OER) catalyst. The current density started at 60 mA cm−2 and finished at 280 mA cm−2, with an increment of 20 mA cm−2 per 500 s without ohmic potential drop (IR) correction.
In this paper, a two-step hydrothermal routine is adopted to prepare FeCoNiS-FeOx catalyst. At first, hydroxide nanowire array precursor is prepared. The precursor nanowire arrays serve as backbones for the catalyst not only constructs effective conductive channels but also provides rich active sites. Secondly, the final product is prepared via anion exchange and redox reactions with Na2S as sulfurizing reagent. The catalyst shows excellent OER activity needing overpotentials of 220.5 and 269.9 mV to attain current densities of 10 and 100 mA cm−2 in 1.0 M KOH. Typically, the catalyst also shows long-term electrochemical stability for at least 30 h. The good catalysis performance is due to FeS, Co3S4, and Ni3S4. CC as substrate could enhance the conductivity of the material. Nanowire structure could increase the surface area of materials and expose more active sites. Most importantly, transition-metal sulfide could optimize material structure and give a full play to the synergy effect between different elements. FeCoNiS-FeOx catalyst is a promising electrocatalyst for OER in alkaline media.
Ferric nitrate [Fe(NO3)3.9H2O, Mw = 404.00], nickel nitrate [Ni(NO3)2.6H2O, Mw = 290.79], cobalt nitrate [Co(NO3)2.6H2O, Mw = 291.03], ammonium fluoride NH4F, Mw = 37.0), urea [CO(NH2)2, Mw = 60.06], potassium hydroxide (KOH, Mw = 56.1) are provided by Shanghai Aladdin Ltd. Sodium sulfide (Na2S, Mw = 78.04) and ruthenium chloride (RuCl3.3H2O ≥ 43%) are bought from Sigma-Aldrich Co. Ltd. CC is supplied by Jingchong electronics technology company. The surface must be free from oil and dirt, then acetone, hydrochloric acid (3 mol/L), ethanol, and ultrapure water are used to clean the surface of CC. Ultrapure water (18.2 MΩ.cm) is used to prepare all aqueous solutions in this work. None of the reagents as received are further purified.
Fe(NO3)3.9H2O 0.323 g, Ni(NO3)2.6H2O 0.058 g, Co(NO3)2.6H2O 0.058 g, NH4F 0.03 g, CO(NH2)2 0.12 g are added to 20 ml ultrapure water to form mixture solution after 30 min stirring. The final solution and the pretreated CC are sealed in a 30-ml Teflon-lined stainless-steel high-pressure reactor and maintained at 120°C for 5 h. Then, the product is naturally cooled to room temperature. The product is taken from the reactor and washed for three times with ultrapure water and ethanol successively. Dried under 60°C for 2 h.
First, 0.035 g sodium sulfide is added to 20 ml ultrapure water with stirring. The formed solution and the precursor are sealed in a 30-ml Teflon-lined stainless-steel high-pressure reactor and maintain at 120°C for 3 h. After naturally cooling to room temperature, the product is washed with ultrapure water and ethanol successively. At last, the product is dried for 2 h under 60°C.
A diffractometer (RigakuD/MAX 2550, Cu Kα radiation, λ = 1.5418 Å) is used to perform XRD characterization. The scan range is from 5 to 80° with a scanning rate of 5°/min. SEM characterizations are realized on a MERLIN compact SEM with the accelerating voltage of 20 kV. TEM characterizations are realized on TEM of Zeiss Libra 200FE with operation voltage of 200 kV. An ESCALABMK II X-ray photoelectron spectrometer is used to measure XPS spectrum with Mg as the exciting source.
A CHI 660E electrochemical analyzer (CH Instruments, Inc., Shanghai) is used to perform all the electrochemical tests. In order to better characterize the electrode reaction, a three-electrode system is adopted. The catalysts/CC is used as working electrode. Mercuric oxide electrode (Hg-HgO) is as contrast electrode. Graphite rod is as auxiliary electrode. Potassium hydroxide solution (1.0 M) is used as the working electrolyte solution. All experiments are realized at 25°C. All potentials for LSV curves are calibrated on reversible hydrogen electrode (RHE) scale [E (RHE) = E + 0.059 × 14 + 0.098]. Unless stated otherwise, all LSV potentials are calibrated with ohmic potential drop (IR) due to solution resistance. Overpotentials (ΔE) are calculated based on the equation ΔE = E (RHE) - IR - 1.23.
All datasets generated for this study are included in the article.
WT has done the experimental work. MH, ZS, and WY helped in characterization. XZ has written the manuscript. CD and SZ have revised the manuscript.
This work was supported by the Natural Science Foundation of Shandong Province (ZR2014BL025).
The authors declare that the research was conducted in the absence of any commercial or financial relationships that could be construed as a potential conflict of interest.
Biesinger, M. C., Payne, B. P., Grosvenor, A. P., Lau, L. W. M., Gerson, A. R., and Smart, R. S. C. (2011). Resolving surface chemical states in XPS analysis of first row transition metals, oxides and hydroxides: Cr, Mn, Fe, Co and Ni. Appl. Surface Sci. 257, 2717–2730. doi: 10.1016/j.apsusc.2010.10.051
Chai, Y.-M., Shang, X., Liu, Z.-Z., Dong, B., Han, G.-Q., Gao, W.-K., et al. (2018). Ripple-like NiFeCo sulfides on nickel foam derived from in-situ sulfurization of precursor oxides as efficient anodes for water oxidation. Appl. Surface Sci. 428, 370–376. doi: 10.1016/j.apsusc.2017.09.122
Chemelewski, W. D., Lee, H. C., Lin, J. F., Bard, A. J., and Mullins, C. B. (2014). Amorphous FeOOH oxygen evolution reaction catalyst for photoelectrochemical water splitting. J. Am. Chem. Soc. 136, 2843–2850. doi: 10.1021/ja411835a
Cheng, N., Liu, Q., Asiri, A. M., Xing, W., and Sun, X. (2015). A Fe-doped Ni3S2particle film as a high-efficiency robust oxygen evolution electrode with very high current density. J. Mater. Chem. A 3, 23207–23212. doi: 10.1039/c5ta06788j
Chow, J., Kopp, R. J., and Portney, P. R. (2003). Energy resources and global development. Science 302, 1528–1531. doi: 10.1126/science.1091939
Dresselhaus, M. S., and Thomas, I. L. (2001). Alternative energy technologies. Nature 414, 332–337. doi: 10.1038/35104599
Feng, J. X., Xu, H., Dong, Y. T., Ye, S. H., Tong, Y. X., and Li, G. R. (2016a). FeOOH/Co/FeOOH hybrid nanotube arrays as high-performance electrocatalysts for the oxygen evolution reaction. Angew. Chem. Int. Ed Engl. 55, 3694–3698. doi: 10.1002/anie.201511447
Feng, J. X., Ye, S. H., Xu, H., Tong, Y. X., and Li, G. R. (2016b). Design and synthesis of FeOOH/CeO2 heterolayered nanotube electrocatalysts for the oxygen evolution reaction. Adv. Mater. 28, 4698–4703. doi: 10.1002/adma.201600054
Gao, Z., Chen, C., Chang, J., Chen, L., Wang, P., Wu, D., et al. (2018). Porous Co3S4@Ni3S4 heterostructure arrays electrode with vertical electrons and ions channels for efficient hybrid supercapacitor. Chem. Eng. J. 343, 572–582. doi: 10.1016/j.cej.2018.03.042
Guo, X., Kong, R.-M., Zhang, X., Du, H., and Qu, F. (2017). Ni(OH)2 nanoparticles embedded in conductive microrod array: an efficient and durable electrocatalyst for alkaline oxygen evolution reaction. ACS Catal. 8, 651–655. doi: 10.1021/acscatal.7b03406
Hu, Q., Ma, W., Liang, G., Nan, H., Zheng, X., and Zhang, X. (2015). Anion-exchange reaction synthesized CoNi2S4 nanowires for superior electrochemical performances. RSC Adv. 5, 84974–84979. doi: 10.1039/c5ra18625k
Jin, Y., Huang, S., Yue, X., Du, H., and Shen, P. K. (2018). Mo- and Fe-Modified Ni(OH)2/NiOOH nanosheets as highly active and stable electrocatalysts for oxygen evolution reaction. ACS Catal. 8, 2359–2363. doi: 10.1021/acscatal.7b04226
Ke, Z., Wang, H., He, D., Song, X., Tang, C., Liu, J., et al. (2018). Co2P nanoparticles wrapped in amorphous porous carbon as an efficient and stable catalyst for water oxidation. Front. Chem. 6:580. doi: 10.3389/fchem.2018.00580
Kuang, M., Wang, Q., Ge, H., Han, P., Gu, Z., Al-Enizi, A. M., et al. (2017). CuCoOx/FeOOH Core–shell nanowires as an efficient bifunctional oxygen evolution and reduction catalyst. ACS Energy Lett. 2, 2498–2505. doi: 10.1021/acsenergylett.7b00835
Lee, Y., Suntivich, J., May, K. J., Perry, E. E., and Shao-Horn, Y. (2012). Synthesis and activities of rutile IrO2 and RuO2 nanoparticles for oxygen evolution in acid and alkaline solutions. J. Phys. Chem. Lett. 3, 399–404. doi: 10.1021/jz2016507
Li, F., Du, J., Li, X., Shen, J., Wang, Y., Zhu, Y., et al. (2018). Integration of FeOOH and Zeolitic imidazolate framework-derived nanoporous carbon as an efficient electrocatalyst for water oxidation. Adv. Energy Mater. 8:1702598. doi: 10.1002/aenm.201702598
Li, H., Chen, S., Zhang, Y., Zhang, Q., Jia, X., Zhang, Q., et al. (2018). Systematic design of superaerophobic nanotube-array electrode comprised of transition-metal sulfides for overall water splitting. Nat. Commun. 9:2452. doi: 10.1038/s41467-018-04888-0
Liu, D., Lu, Q., Luo, Y., Sun, X., and Asiri, A. M. (2015). NiCo2S4 nanowires array as an efficient bifunctional electrocatalyst for full water splitting with superior activity. Nanoscale 7, 15122–15126. doi: 10.1039/c5nr04064g
Liu, T., Sun, X., Asiri, A. M., and He, Y. (2016). One-step electrodeposition of Ni–Co–S nanosheets film as a bifunctional electrocatalyst for efficient water splitting. Int. J. Hydr. Energy 41, 7264–7269. doi: 10.1016/j.ijhydene.2016.03.111
Lu, W., Liu, T., Xie, L., Tang, C., Liu, D., Hao, S., et al. (2017). In situ derived CoB nanoarray: a high-efficiency and durable 3D bifunctional electrocatalyst for overall alkaline water splitting. Small 13:1700805. doi: 10.1002/smll.201700805
Lu, Z., Qian, L., Xu, W., Tian, Y., Jiang, M., Li, Y., et al. (2016). Dehydrated layered double hydroxides: Alcohothermal synthesis and oxygen evolution activity. Nano Res. 9, 3152–3161. doi: 10.1007/s12274-016-1197-4
Luo, W., Jiang, C., Li, Y., Shevlin, S. A., Han, X., Qiu, K., et al. (2017). Highly crystallized α-FeOOH for a stable and efficient oxygen evolution reaction. J. Mater. Chem. A 5, 2021–2028. doi: 10.1039/c6ta08719a
Ning, Y., Ma, D., Shen, Y., Wang, F., and Zhang, X. (2018). Constructing hierarchical mushroom-like bifunctional NiCo/NiCo2S4@NiCo/Ni foam electrocatalysts for efficient overall water splitting in alkaline media. Electrochim. Acta 265, 19–31. doi: 10.1016/j.electacta.2018.01.150
Park, G., Kim, Y. I., Kim, Y. H., Park, M., Jang, K. Y., Song, H., et al. (2017). Preparation and phase transition of FeOOH nanorods: strain effects on catalytic water oxidation. Nanoscale 9, 4751–4758. doi: 10.1039/c6nr09790a
Qin, Z., Chen, Y., Huang, Z., Su, J., Diao, Z., and Guo, L. (2016). Composition-dependent catalytic activities of noble-metal-free NiS/Ni3S4 for hydrogen evolution reaction. J. Phys. Chem. C 120, 14581–14589. doi: 10.1021/acs.jpcc.6b05230
Reier, T., Oezaslan, M., and Strasser, P. (2012). Electrocatalytic Oxygen Evolution Reaction, (OER). on Ru, Ir, and Pt catalysts: a comparative study of nanoparticles and bulk materials. ACS Catal. 2, 1765–1772. doi: 10.1021/cs3003098
Sivanantham, A., Ganesan, P., and Shanmugam, S. (2016). Hierarchical NiCo2S4nanowire arrays supported on ni foam: an efficient and durable bifunctional electrocatalyst for oxygen and hydrogen evolution reactions. Adv. Funct. Mater. 26:201600566. doi: 10.1002/adfm.201600566
Tang, Y., Zheng, S., Xue, H., and Pang, H. (2019). Regulation of the Ni(2+). Content in a Hierarchical Urchin-Like MOF for high-performance electrocatalytic oxygen evolution. Front. Chem. 7:411. doi: 10.3389/fchem.2019.00411
Turner, J. A. (2004). Sustainable hydrogen production. Science 305, 972–974. doi: 10.1126/science.1103197
Wang, H., Yang, J., Yang, L., Zhang, G., Liu, C., Tang, H., et al. (2018). FeCoNi sulphide-derived nanodots as electrocatalysts for efficient oxygen evolution reaction. Funct. Mater. Lett. 11:1850058. doi: 10.1142/s1793604718500583
Wang, X., Liu, C., Li, Q., Li, H., Xu, J., Chu, X., et al. (2018). 3D Heterogeneous Co3O4@Co3S4 Nanoarrays grown on Ni Foam as a binder-free electrode for lithium-ion batteries. ChemElectroChem 5, 309–315. doi: 10.1002/celc.201701050
Xiao, J., Wan, L., Yang, S., Xiao, F., and Wang, S. (2014). Design hierarchical electrodes with highly conductive NiCo2S4 nanotube arrays grown on carbon fiber paper for high-performance pseudocapacitors. Nano Lett. 14, 831–838. doi: 10.1021/nl404199v
Yang, L., Xie, L., Ren, X., Wang, Z., Liu, Z., Du, G., et al. (2017). Hierarchical CuCo2S4 nanoarrays for high-efficient and durable water oxidation electrocatalysis. Chem. Commun. 54, 78–81. doi: 10.1039/c7cc07259g
Yin, Q., Tan, J. M., Besson, C., Geletii, Y. V., Musaev, D. G., Kuznetsov, A. E., et al. (2010). A fast soluble carbon-free molecular water oxidation catalyst based on abundant metals. Science 328, 342–345. doi: 10.1126/science.1185372
Zeng, L., Ren, W., Zheng, J., Wu, A., and Cui, P. (2012). Synthesis of water-soluble FeOOH nanospindles and their performance for magnetic resonance imaging. Appl. Surface Sci. 258, 2570–2575. doi: 10.1016/j.apsusc.2011.10.093
Zhang, L., Zhang, R., Ge, R., Ren, X., Hao, S., Xie, F., et al. (2017). Facilitating active species generation by amorphous NiFe-Bi layer formation on NiFe-LDH nanoarray for efficient electrocatalytic oxygen evolution at alkaline pH. Chem. A Eur. J. 23, 11499–11503. doi: 10.1002/chem.201702745
Zhang, S., Sun, Y., Liao, F., Shen, Y., Shi, H., and Shao, M. (2018). Co9S8-CuS-FeS trimetal sulfides for excellent oxygen evolution reaction electrocatalysis. Electrochim. Acta 283, 1695–1701. doi: 10.1016/j.electacta.2018.07.133
Zhao, Q., Yang, J., Liu, M., Wang, R., Zhang, G., Wang, H., et al. (2018). Tuning electronic Push/Pull of Ni-based hydroxides to enhance hydrogen and oxygen evolution reactions for water splitting. ACS Catal. 8, 5621–5629. doi: 10.1021/acscatal.8b01567
Zheng, X., Song, X., Wang, X., Zhang, Z., Sun, Z., and Guo, Y. (2018a). Nickel–copper bimetal organic framework nanosheets as a highly efficient catalyst for oxygen evolution reaction in alkaline media. N. J. Chem. 42, 8346–8350. doi: 10.1039/c8nj01035h
Zheng, X., Zhang, J., Sun, Z., Zhang, Z., and Xi, D. (2018b). Fabrication of Amorphous Cu-Co-P Nanofilms on CuCo2O4 anoarrays by in situ electrochemical reduction for efficient hydrogen evolution in alkaline solution. Eur. J. Inorg. Chem. 2018, 3565–3569. doi: 10.1002/ejic.201800492
Zheng, Y., Jiao, Y., Zhu, Y., Cai, Q., Vasileff, A., Li, L. H., et al. (2017). Molecule-level g-C3N4 coordinated transition metals as a new class of electrocatalysts for oxygen electrode reactions. J. Am. Chem. Soc. 139, 3336–3339. doi: 10.1021/jacs.6b13100
Zheng, Y., Jiao, Y., Zhu, Y., Li, L. H., Han, Y., Chen, Y., et al. (2014). Hydrogen evolution by a metal-free electrocatalyst. Nat. Commun. 5:3783. doi: 10.1038/ncomms4783
Keywords: oxygen evolution reaction, electrocatalyst, FeCoNi sulfides, nanowires array, in situ sulfurization
Citation: Teng W, Huo M, Sun Z, Yang W, Zheng X, Ding C and Zhang S (2020) FeCoNi Sulfides Derived From In situ Sulfurization of Precursor Oxides as Oxygen Evolution Reaction Catalyst. Front. Chem. 8:334. doi: 10.3389/fchem.2020.00334
Received: 16 December 2019; Accepted: 31 March 2020;
Published: 05 May 2020.
Edited by:
Xing Huang, ETH Zürich, SwitzerlandReviewed by:
Yun Hau Ng, City University of Hong Kong, Hong KongCopyright © 2020 Teng, Huo, Sun, Yang, Zheng, Ding and Zhang. This is an open-access article distributed under the terms of the Creative Commons Attribution License (CC BY). The use, distribution or reproduction in other forums is permitted, provided the original author(s) and the copyright owner(s) are credited and that the original publication in this journal is cited, in accordance with accepted academic practice. No use, distribution or reproduction is permitted which does not comply with these terms.
*Correspondence: Xiangjiang Zheng, enhqNDQwOEAxMjYuY29t; Caifeng Ding, ZGluZ2NhaWZlbmdAcXVzdC5lZHUuY24=
Disclaimer: All claims expressed in this article are solely those of the authors and do not necessarily represent those of their affiliated organizations, or those of the publisher, the editors and the reviewers. Any product that may be evaluated in this article or claim that may be made by its manufacturer is not guaranteed or endorsed by the publisher.
Research integrity at Frontiers
Learn more about the work of our research integrity team to safeguard the quality of each article we publish.