- 1School of Chemistry and Materials Engineering, Fuyang Normal University, Fuyang, China
- 2Anhui Province Key Laboratory for Degradation and Monitoring of Pollution of the Environment, Fuyang, China
A fluorescent and colorimetric chemosensor L based on rhodamine 6G was designed, synthesized, and characterized. Based on a two-step reaction, the chemosensor L effectively recognized Hg2+. The interaction between the chemosensor and Hg2+ was confirmed by ultraviolet–visible spectrophotometry, fluorescence spectroscopy, electrospray ionization–mass spectrometry, Fourier-transform infrared spectroscopy, and frontier molecular orbital calculations. The chemosensor L was also incorporated into test strips and silica gel plates, which demonstrated good selectivity and high sensitivity for Hg2+.
Introduction
Hg2+ is known to be very dangerous to human health because of its extreme toxicity (Chen et al., 2012). Even extremely low levels of Hg2+ accumulation in the human body can lead to numerous diseases in human organs (Pan et al., 2018; Peng et al., 2018; Xu et al., 2018). Thus, it is important to develop methods to detect Hg2+ with good sensitivity and high selectivity.
Compared to other common methods for Hg2+ detection, fluorescent chemosensors have received considerable attention because of their distinct advantages (Nolna and Lippard, 2008; Zhang et al., 2008; Lee et al., 2015; Xie et al., 2015; He et al., 2016; Sakunkaewkasem et al., 2018; Bai et al., 2019; Yuan et al., 2019). As a result, a growing number of fluorescent chemosensors for Hg2+ have been reported in the past few decades (Chen et al., 2011; Wang et al., 2012; Park et al., 2013; Cheng et al., 2015; Hong et al., 2016; Lee et al., 2016; Erdemir et al., 2017; Bai et al., 2018; Singh et al., 2018).
Many reported Hg2+ chemosensors were designed in one of two ways based on one-step reactions. In the first type of sensor, the interactions between a ligand and Hg2+ results in changes in fluorescence (Figure 1A). The main disadvantage of this type of sensor is its vulnerability to interference from other metal ions, especially Cu2+, Cd2+, and Fe3+ (Wang et al., 2011; Kau et al., 2012; Rao et al., 2012; Zhang et al., 2013; Kim et al., 2014; Fang et al., 2016; Alibert et al., 2017; Huang et al., 2019). In the second type of sensor, special chemical reactions (e.g., desulfurization between the ligands and Hg2+) generate a new product with a different fluorescence spectrum (Figure 1B).
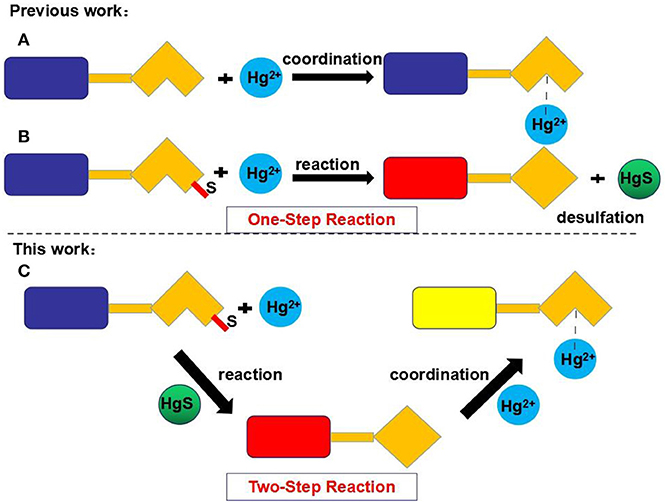
Figure 1. Strategies for the chemosensing of Hg2+. Type A: The coordination between ligand and Hg2+. Type B: The desulfurization between ligand and Hg2+. Type C: The two-step reaction mechanism.
In contrast, the fluorescent chemosensor L developed in this study recognizes Hg2+ through a two-step reaction based on rhodamine 6G. The chemosensor L first undergoes a desulfurization reaction with Hg2+, and the resulting product then interacts with Hg2+ (Figure 1C). As demonstrated in this study, L can selectively and sensitively detect Hg2+ without interference from other cations, including Cu2+, Cd2+, and Fe3+. Moreover, test strips and silica gel plates based on L also exhibit good selectivity for Hg2+.
Materials and Physical Methods
1H nuclear magnetic resonance (NMR) and 13C NMR spectra were recorded using a Bruker instrument at 400 MHz with tetramethylsilane as the internal standard and deuterated dimethyl sulfoxide (DMSO-d6) as the solvent. Ultraviolet–visible (UV–vis) absorption spectra were recorded on a Shimadzu UV-1601 spectrophotometer. Luminescence spectra were recorded on a Horiba Fluoromax-4-NIR spectrometer. Melting points were measured on an X-4 digital melting point apparatus (uncorrected). Infrared (IR) spectra were obtained on a Nicolet 5700 FT-IR spectrophotometer. Fluorescent lifetime and fluorescence quantum yield were measured using a Horiba Fluoromax-4 spectrometer. For fluorescent lifetime measurements, the fluorescence signals were collimated and focused onto the entrance slit of a monochromator, and the output plane was equipped with a photomultiplier tube. The fluorescent decay was then analyzed by least-squares regression, and the quality of the exponential fit was evaluated by the goodness of fit (χ2). Mass spectra were recorded with a Shimadzu LCMS-IT/TOF mass spectrometer. All reagents used were of analytical grade.
Synthesis of Compound L
The synthesis of L is outlined in Figure 2. Compound C was synthesized according to the reported procedure (Hong et al., 2016). Compound C (0.5 mmol) and 2-hydroxy-1-naphthaldehyde (0.5 mmol) were then dissolved in 20 ml of ethanol and refluxed for 12 h with stirring, forming an orange precipitate. The crude product was filtered and purified by silica gel chromatography (ethyl acetate:petroleum ether = 3:1) to obtain the fluorescent chemosensor L. Yield 81%, m.p. >300°C, 1H NMR (see Figure S1) (400 MHz, DMSO-d6, δ): 13.84 (s, 1H), 8.76 (d, J = 8.7 Hz, 1H), 7.98 (dd, J = 5.4, 3.2 Hz, 1H), 7.92 (d, J = 8.4 Hz, 1H), 7.72 (d, J = 9.3 Hz, 1H), 7.64 (d, J = 7.5 Hz, 1H), 7.59 (dd, J = 6.0, 2.6 Hz, 2H), 7.42 (t, J = 7.2 Hz, 1H), 7.21 (t, J = 7.3 Hz, 1H), 7.07 (dd, J = 5.3, 3.0 Hz, 1H), 6.72 (d, J = 9.3 Hz, 1H), 6.32 (s, 2H), 6.01 (s, 2H), 5.12 (s, 2H), 3.76 (t, J = 6.7 Hz, 2H), 3.12 (dd, J = 9.2, 5.8 Hz, 4H), 1.81 (s, 6H), 1.20 (t, J = 7.1 Hz, 6H). 13C NMR (see Figure S2) (100 MHz, DMSO) δ 191.24, 175.39, 159.96, 151.58, 151.49, 148.69, 137.52, 137.11, 134.29, 133.46, 129.31, 129.25, 128.26, 127.65, 125.93, 125.03, 124.94, 123.77, 122.80, 119.19, 119.00, 106.77, 103.12, 96.17, 73.51, 49.53, 44.63, 37.92, 17.53, 14.56. HRMS (ESI, see Figure S3) m/z: [M+H]+ Calcd for C39H39N4O2S: 627.2788; Found 627.2786.
General Spectroscopic Methods
A solution of metal ions was prepared from the nitrate salts of K+, Na+, Ag+, Cu2+, Co2+, Ca2+, Cd2+, Mg2+, Ba2+, Pb2+, Fe2+, Ni2+, Zn2+, Mn2+, Hg2+, Al3+, Y3+, Ce3+, and Fe3+ (China Pharmaceutical Co. Ltd., used without further purification). The ligand concentration was kept constant at 1.0 × 10−5 M during spectral measurements. The probe solution was prepared in HEPES buffer (10 mM, pH 7.4)/CH3CN (40:60, V/V).
Results and Discussion
The spectral properties of L were studied in HEPES buffer (Figures 3, 4). When the concentration of Hg2+ increased to 10 equivalents, a new absorption peak at 526 nm appeared (Figure 3). In the fluorescence spectrum, an emission peak appeared at 550 nm upon excitation at 526 nm (Figure 4). The other cations did not result in the same spectral changes as Hg2+. Meanwhile, the color of the solution also changed significantly when 10 equivalents of Hg2+ was added to L. This color change could be observed directly by the naked eye.
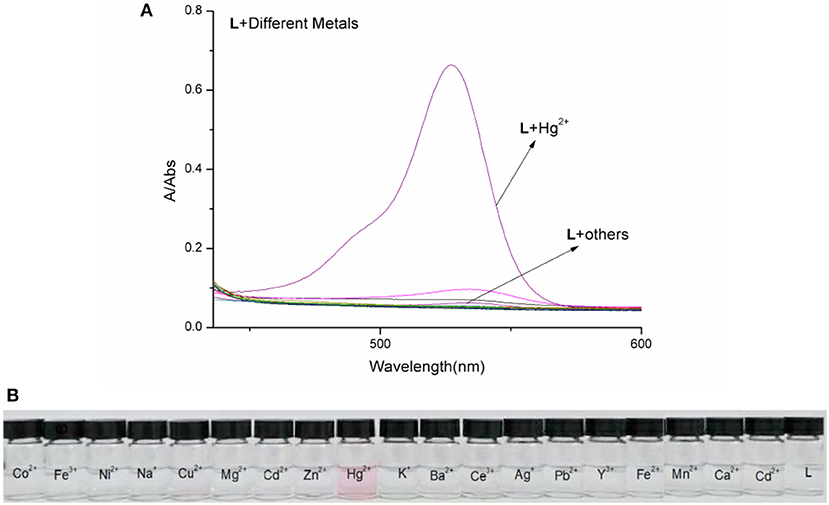
Figure 3. (A) Absorption spectra of L (1.0 × 10−5 M) in the presence of various metal ions in HEPES buffer. (B) Photographs of L (1.0 × 10−5 M) in the presence of various metal ions.
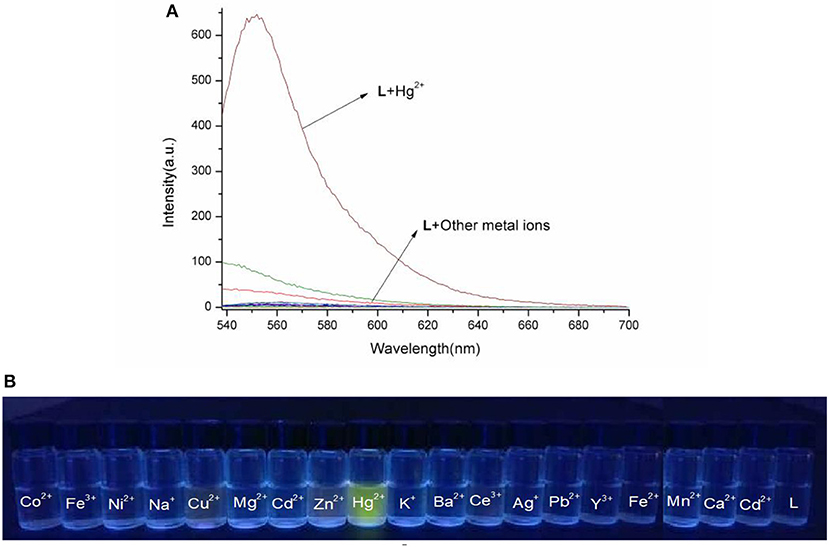
Figure 4. (A) Fluorescence spectra of L (1.0 × 10−5 M) in the presence of various metal ions in HEPES buffer (λex = 526 nm). (B) Photographs of chemosensor L (1.0 × 10−5 M) in the presence of various metal ions under the hand-held ultraviolet lamp.
Based on competition experiments, the detection of Hg2+ by L was not affected by the presence of other cations, including Cu2+, Cd2+, and Fe3+ (Figures S4, S5, ESI). Surprisingly, the spectral properties depended on the ratio of [Hg2+] to [L]. When the ratio did not exceed 1, the spectra did not change markedly before or after Hg2+ was added into the solution of L. The emission peak at 550 nm did not appear (Figures S6, S7, ESI), while no change was observed in the UV–vis spectrum. The fluorescence intensity at 550 nm increased strongly when the ratio exceeded 1, and a new absorption peak at 526 nm appeared. These results are unusual in comparison to previously reported chemosensors for Hg2+ (Figures 3, 4).
To clarify the unusual results, electrospray ionization–mass spectrometry (ESI-MS) was conducted with different ratios of [Hg2+] to [L]. When the ratio did not exceed 1, the ion peak was detected at 611.3018 (m/z), corresponding to the desulfurization reaction product LO (Figure S8, ESI). This implies that L was transformed to LO under the action of Hg2+ provided that the ratio of [Hg2+] to [L] did not exceed 1 (Figure 5). Under this condition, Hg2+ did not lead to the spirolactam ring opening of L. Thus, the fluorescent emission peak at 550 nm did not appear, nor did the absorption peak at 526 nm. Meanwhile, no color change of the solution was observed by the naked eye. The first desulfurization step was completed when [Hg2+]:[L] = 1. When the ratio exceeded 1, the ion peak at m/z 813.2609 was classified as [LO–Hg2++H+]+ (Figure S9, ESI). Under these conditions, Hg2+ caused spirolactam ring opening, generating the emission peak at 550 nm and the absorption peak at 526 nm. In addition, the solution color changed from colorless to pink. After the completion of the first step, Hg2+ transformed the closed spirolactam structure into a ring-opened amide in the second step.
The IR spectra support the above two-step reaction mechanism (Figure S10, ESI). The peak at 1,153 cm−1, which was assigned to the stretching vibration of C=S, disappeared during the first step. The peak at 1,518 cm−1, corresponding to the stretching vibration of C=O, vanished in the second step. Therefore, L detected Hg2+ through a two-step reaction (Figure 5).
To understand the interaction between the chemosensor L and Hg2+, a titration experiment was carried out. The fluorescent intensity at 550 nm hardly changed when [Hg2+]:[L] changed from 0 to 1, and the absorption spectrum of L did not change significantly. However, the fluorescent intensity increased sharply when [Hg2+]:[L] changed from 1 to 2, and the intensity of the new absorption peak at 526 nm increased greatly. As shown in Figure 3, two inflection points were observed in the absorption spectrum of L + Hg2+, indicating that L might interact with Hg2+ in two steps. The stoichiometric ratio between L and Hg2+ was 1:1 or 1:2. The above results are in accordance with the Job plot (Figure 6 and Figures S11–S13, ESI).
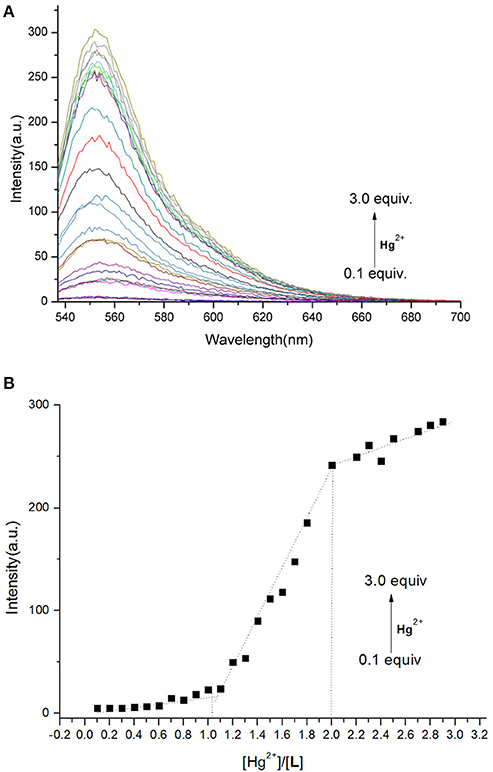
Figure 6. (A) Fluorescence spectra of L (1.0 × 10−5 M) in the presence of different concentrations of Hg2+ in HEPES buffer (λex = 526 nm). (B) Plot of fluorescence intensity at 550 nm vs. Hg2+ concentration in the range of 0.1–3.0 equiv.
The calculated frontier molecular orbitals and energy levels of L, LO, and LO–Hg2+ confirmed the interaction between L and Hg2+. As shown in Figure 7, the molecular orbital structure of L was clearly different from those of LO and LO–Hg2+. The energy gaps between the highest occupied molecular orbital (HOMO) and lowest unoccupied molecular orbital (LUMO) in L, LO, and LO–Hg2+ were calculated to be 0.16, 0.15, and 0.69 eV, respectively. The results indicate that Hg2+ and L were combined via a two-step reaction, which effectively decreased the energy gap and stabilized the system.
The detection limit of L toward Hg2+ was determined to be 0.012 × 10−7 M (Figure S14, ESI). Moreover, the fluorescence lifetime and fluorescence quantum yield of L–Hg2+ were measured to study the fluorescent properties of L (Table S1, ESI).
To evaluate the practical application potential of the chemosensor L, test strips and silica gel plates were prepared using L. When Hg2+ was added to the test strips, a clear change in color from colorless to pink was observed, and the fluorescence was enhanced (Figure 8). In addition, when “Hg2+” was written on the silica gel plate using Hg2+ solution, the silica gel plate underwent significant changes. The results indicate that L can be used to create smart materials for the detection of Hg2+ in aqueous solution.
Conclusions
In summary, a chemosensor L based on rhodamine 6G was designed, synthesized, and characterized. L was demonstrated to sensitively and selectively recognize Hg2+ via a two-step reaction, different from the one-step mechanisms by which reported chemosensors interact with Hg2+. The interaction between L and Hg2+ was confirmed by UV–vis spectrophotometry, fluorescence spectroscopy, ESI-MS, IR spectroscopy, and frontier molecular orbital calculations. In addition, L could be incorporated into test strips and silica gel plates to effectively detect Hg2+.
Data Availability Statement
All datasets generated for this study are included in the article/Supplementary Material.
Author Contributions
C-BB and RQ designed the work and wrote the manuscript. C-BB, W-GW, JZ, BW, and LZ carried out the experiments. CW, S-SC, and SY performed the spectroscopic experiments. RQ revised and edited the manuscript. All authors reviewed the manuscript and have agreed to its publication.
Funding
This work was supported by the National Natural Science Foundation of China (No. 21302019), Anhui Provincial Natural Science Foundation (No. 1908085QB78), the Key Projects of Natural Science Research of Anhui Province Colleges and Universities (No. KJ2019ZD38), the Key Projects of Support Program for Outstanding Young Talents in Anhui Province Colleges and Universities (No. gxyqZD2016068), the Key Program for Young Talents of Fuyang Normal University (No. rcxm201902), National Undergraduate Training Programs for Innovation and Entrepreneurship (Nos. 201810371058 and 201810371054), and the Horizontal Cooperation Project of Fuyang Municipal Government and Fuyang Normal University (Nos. XDHX201730 and XDHX201731).
Conflict of Interest
The authors declare that the research was conducted in the absence of any commercial or financial relationships that could be construed as a potential conflict of interest.
Supplementary Material
The Supplementary Material for this article can be found online at: https://www.frontiersin.org/articles/10.3389/fchem.2020.00014/full#supplementary-material
References
Alibert, A., Vaiano, P., Caporale, A., Consales, M., Ruvo, M., and Cusano, A. (2017). Fluorescent chemosensors for Hg2+ detection in aqueous environment. Sens. Actuators B Chem. 247, 727–735. doi: 10.1016/j.snb.2017.03.026
Bai, C. B., Fan, H. Y., Qiao, R., Wang, S. N., Wei, B., Meng, Q., et al. (2019). Synthesis of methionine methyl ester-modified coumarin as the fluorescent-colorimetric chemosensor for selective detection Cu2+ with application in molecular logic gate. Spectrochim. Acta. A 216, 45–51. doi: 10.1016/j.saa.2019.03.016
Bai, C. B., Qiao, R., Liao, J. X., Xiong, W. Z., Zhang, J., Chen, S. S., et al. (2018). A highly selective and reversible fluorescence “off-on-off” chemosensor for Hg2+ based on rhodamine-6G dyes derivative and its application as a molecular logic gate. Spectrochim. Acta. A 202, 252–259. doi: 10.1016/j.saa.2018.05.050
Chen, X., Pradhan, T., Wang, F., Kim, J. S., and Yoon, J. (2011). Fluorescent chemosensors based on spiroring-opening of xanthenes and related derivatives. Chem. Rev. 112, 1910–1956. doi: 10.1021/cr200201z
Chen, Y., Zhu, C., Yang, Z., Li, J., Jiao, Y., He, W., et al. (2012). A new “turn-on” chemodosimeter for Hg2+: ICT fluorophore formation via Hg2+-induced carbaldehyde recovery from 1, 3-dithiane. Chem. Commun. 48, 5094–5096. doi: 10.1039/c2cc31217d
Cheng, H. Z., Li, G., and Liu, M. (2015). A metal-enhanced fluorescence sensing platform based on new mercapto rhodamine derivatives for reversible Hg2+ detection. J. Hazard. Mater. 287, 402–411. doi: 10.1016/j.jhazmat.2015.01.046
Erdemir, S., Yuksekogul, M., Karakurt, S., and Kocyigit, O. (2017). Dual-channel fluorescent probe based on bisphenol a-rhodamine for Zn2+ and Hg2+ through different signaling mechanisms and its bioimaging studies. Sens. Actuators B Chem. 241, 230–238. doi: 10.1016/j.snb.2016.10.082
Fang, Y. W., Zhang, B. G., Chen, J., Kong, L., Yang, L. M., Bi, H., et al. (2016). An aie active probe for specific sensing of Hg2+ based on linear conjugated bis-schiff base. Sens. Actuators B Chem. 229, 338–346. doi: 10.1016/j.snb.2016.01.130
He, W. L., Dong, L. B., Liu, Y., and Lin, Y. W. (2016). Fluorescent chemosensors manipulated by dual/triple interplaying sensing mechanisms. Chem. Soc. Rev. 45, 6449–6461. doi: 10.1039/C6CS00413J
Hong, M., Lu, X., Chen, Y., and Xu, D. (2016). A novel rhodamine-based colorimetric and fluorescent sensor for Hg2+ in water matrix and living cell. Sens. Actuators B Chem. 232, 28–36. doi: 10.1016/j.snb.2016.03.125
Huang, L., Yang, Z., Zhou, Z., Li, Y., Tang, S., Xiao, W., et al. (2019). A dual colorimetric and near-infrared fluorescent turn-on probe for Hg2+ detection and its applications. Dyes Pigments 163, 118–125. doi: 10.1016/j.dyepig.2018.11.047
Kau, P., Kau, S., and Singh, K. (2012). Bis (N-methylindolyl). methane-based chemical probes for Hg2+ and Cu2+ and molecular implication gate operating in fluorescence mode. Org. Biomol. Chem. 10, 1497–1501. doi: 10.1039/c2ob06793e
Kim, I., Lee, E. N., Jeong, J. Y., Chung, H. Y., Cho, K. B., and Lee, E. (2014). Micellar and vesicular nanoassemblies of triazole-based amphiphilic probes triggered by mercury, (II). ions in a 100% aqueous medium. Chem. Commun. 50, 14006–14009. doi: 10.1039/C4CC06742H
Lee, J. J., Kim, S. Y., Nam, E., Lee, Y. S., Lim, H. M., and Kim, C. (2016). A PET-based fluorometric chemosensor for the determination of mercury (II) and pH, and hydrolysis reaction-based colorimetric detection of hydrogen sulfide. Dalton Trans. 45, 5700–5712. doi: 10.1039/C6DT00147E
Lee, M., Kim, S. J., and Sessler, L. J. (2015). Small molecule-based ratiometric fluorescence probes for cations, anions, and biomolecules. Chem. Soc. Rev. 44, 4185–4191. doi: 10.1039/C4CS00280F
Nolna, M. E., and Lippard, J. S. (2008). Tools and tactics for the optical detection of mercuric ion. Chem. Rev. 108, 3443–3480. doi: 10.1021/cr068000q
Pan, L. S., Li, K., Li, L. L., Li, Y. M., Shi, L., and Liu, H. Y. (2018). A reaction-based ratiometric fluorescent sensor for the detection of Hg(II) ions in both cells and bacteria. Chem. Commun. 54, 4955–4958. doi: 10.1039/C8CC01031E
Park, S., Kim, W., Swamy, K. M. K., Lee, H. Y., Jung, J. Y., Kim, G., et al. (2013). Rhodamine hydrazone derivatives bearing thiophene group as fluorescent chemosensors for Hg2+. Dyes Pigments 99, 323–328. doi: 10.1016/j.dyepig.2013.05.015
Peng, D., Zhang, L., Liang, P. R., and Qiu, D. J. (2018). Rapid detection of mercury ions based on nitrogen-doped graphene quantum dots accelerating formation of manganese porphyrin. ACS Sens. 3, 1040–1047. doi: 10.1021/acssensors.8b00203
Rao, S. A., Kim, D. Y., Wang, J. T., Kim, H. K., Wang, H. S., and Ahn, H. K. (2012). Reaction-based two-photon probes for mercury ions: fluorescence imaging with dual optical windows. Org. Lett. 14, 2598–2601. doi: 10.1021/ol3009057
Sakunkaewkasem, S., Petdum, A., Panchan, W., Sirirak, J., Charoenpanich, A., Sooksimuang, T., et al. (2018). Dual-analyte fluorescent sensor based on [5] helicene derivative with super large stokes shift for the selective determinations of Cu2+ or Zn2+ in buffer solutions and its application in a living cell. ACS Sens. 3, 1016–1023. doi: 10.1021/acssensors.8b00158
Singh, G., Ran, S., Sharmai, G., Kalra, P., Singh, N., and Verma, V. (2018). Coumarin-derived organosilatranes: functionalization at magnetic silica surface and selective recognition of Hg2+ ion. Sens. Actuators B Chem. 266, 861–872. doi: 10.1016/j.snb.2018.03.036
Wang, F., Nam, S. W., Guo, Z., Park, S., and Yoon, J. (2012). A new rhodamine derivative bearing benzothiazole and thiocarbonyl moieties as a highly selective fluorescent and colorimetric chemodosimeter for Hg2+. Sens. Actuators B Chem. 161, 948–953. doi: 10.1016/j.snb.2011.11.070
Wang, Y., Huang, Y., Li, B., Zhang, L., Song, H., Jiang, H., et al. (2011). A cell compatible fluorescent chemosensor for Hg2+ based on a novel rhodamine derivative that works as a molecular keypad lock. RSC Adv. 1, 1294–1300. doi: 10.1039/c1ra00488c
Xie, H. P., Guo, Q. F., Wang, Y. L., Yang, S., Yao, H. D., and Yang, Y. G. (2015). A dansyl-rhodamine ratiometric fluorescent probe for Hg2+ based on fret mechanism. J. Fluoresc. 25, 319–325. doi: 10.1007/s10895-015-1511-7
Xu, D., Yu, S., Yin, Y., Wang, S., Lin, Q., and Yuan, Z. (2018). Sensitive colorimetric Hg2+ detection via amalgamation-mediated shape transition of gold nanostars. Front. Chem. 6:566. doi: 10.3389/fchem.2018.00566
Yuan, X., Leng, H. T., Guo, Q. Z., Wang, Y. C., Li, Z. J., Yang, W. W., et al. (2019). A fret-based dual-channel turn-on fluorescence probe for the detection of Hg2+ in living cells. Dyes Pigments 161, 403–410. doi: 10.1016/j.dyepig.2018.09.078
Zhang, P., Shi, B. B., Zhang, Y. M., Lin, Q., Yao, H., You, X. M., et al. (2013). A selective fluorogenic chemodosimeter for Hg2+ based on the dimerization of desulfurized product. Tetrahedron 69, 10292–10298. doi: 10.1016/j.tet.2013.10.024
Keywords: chemosensor, Hg2+, rhodamine 6G, test strips, silica gel plates
Citation: Bai C-B, Wang W-G, Zhang J, Wang C, Qiao R, Wei B, Zhang L, Chen S-S and Yang S (2020) A Fluorescent and Colorimetric Chemosensor for Hg2+ Based on Rhodamine 6G With a Two-Step Reaction Mechanism. Front. Chem. 8:14. doi: 10.3389/fchem.2020.00014
Received: 15 May 2019; Accepted: 07 January 2020;
Published: 19 February 2020.
Edited by:
Yun-Bao Jiang, Xiamen University, ChinaReviewed by:
Juyoung Yoon, Ewha Womans University, South KoreaTony D. James, University of Bath, United Kingdom
Copyright © 2020 Bai, Wang, Zhang, Wang, Qiao, Wei, Zhang, Chen and Yang. This is an open-access article distributed under the terms of the Creative Commons Attribution License (CC BY). The use, distribution or reproduction in other forums is permitted, provided the original author(s) and the copyright owner(s) are credited and that the original publication in this journal is cited, in accordance with accepted academic practice. No use, distribution or reproduction is permitted which does not comply with these terms.
*Correspondence: Rui Qiao, qiaorui@mail.ipc.ac.cn