- Guangxi Key Laboratory of Green Processing of Sugar Resources, College of Biological and Chemical Engineering, Guangxi University of Science and Technology, Liuzhou, China
Three-dimensional (3D) graphene-based hydrogels have attracted great interest for applying in supercapcacitors electrodes, owing to their intriguing properties that combine the structural interconnectivities and the outstanding properties of graphene. However, the pristine graphene hydrogel can not satisfy the high-performance demands, especial in high specific capacitance. Consequently, novel graphene-based composite hydrogels with increased electrochemical properties have been developed. In this mini review, a brief summary of recent progress in the research of the three-dimensional graphene-based composite hydrogel for flexible supercapacitors electrodes materials is presented. The latest progress in the graphene-based composite hydrogel consisting of graphene/metal, graphene/polymer, and atoms doped graphene is discussed. Furthermore, future perspectives and challenges in graphene-based composite hydrogel for supercapacitor electrodes are also expressed.
Introduction
In recent years, supercapacitors have been attracted intensive investigation for sustainable energy application, because of their advantages in excellent power density and high charge/discharge rates (Li et al., 2018). With the rapid growth of wearable electronics, flexible supercapacitors that can work under consecutive bending or stretching are urgently needed. However, it remains a great challenge to obtain supercapacitors with excellent electrochemical performance and good flexibility. Since the supercapacitors mainly consist of two electrodes and a separated membrane, the electrodes are often considered to be a key factor (Xu et al., 2018). Thus, it is critically important to develop innovative materials for flexible supercapacitor electrodes.
As a unique two-dimensional (2D) carbon material, graphene has gained tremendous attention in various application aspects due to its fast-charged carrier mobility, excellent conductivity and largely tunable surface area (Sattar, 2019). Based on the extraordinary physico-chemical properties, graphene and its functionalized derivatives (graphene oxide, GO) can be applied in supercapacitors as the electrode materials. Previous studies have demonstrated that the supercapacitors based on graphene can possess excellent specific capacitance (Horn et al., 2019). Unfortunately, the restacking or irreversible agglomeration of graphene sheets can suppress the high conductivity and decrease accessible surface area, which limit the improvement of capacitive performance. To tackle these challenges, three-dimensional (3D) graphene-based architectures including foam, hydrogels, sponges are developed.
Among various 3D macroscopic structures, graphene hydrogels consist of interconnected porous networks with large specific surface areas have received particular attention (Lu et al., 2017). These hydrogels provide multidimensional ion/electron transport pathways with the intrinsic properties of graphene, which makes them promising candidates for supercapacitor electrodes. Great achievements of supercapacitors based on graphene hydrogels have been obtained, while the pristine graphene hydrogel cannot meet the requirements in practical application (Ma et al., 2018). Functional materials or dopants such as metal oxides or hydroxide, conducting polymers, and so on have been introduced to the graphene hydrogels to further improve the electrochemical performance. In this mini-review, a brief retrospect on graphene-based composite hydrogel materials for flexible supercapacitor electrodes will be provided.
Graphene-Based Composite Hydrogel Electrode Materials
Graphene-Metal Composite Hydrogels Electrode Materials
Usually, metal oxides or hydroxides exhibit high pseudo-capacitance mainly due to their faradic reaction beyond formation of electrical double-layers in the charge-discharge processes. Thus, metal oxides or hydroxides including MnO2, Ni(OH)2, and etc. have been widely incorporated into graphene to form composite materials to obtain enhanced performance.
Researches have been focused on coupling MnO2 with other materials (Xu et al., 2019) for supercapacitor electrodes, and different structures of MnO2 have also been incorporated in graphene hydrogel. Zhang et al. (2016) prepared micro-nanostructured pompon-like MnO2/graphene hydrogel composites, and the hydrogel with a MnO2 content of 50% displayed a good capacitive behavior (445.7 F/g at 0.5 A/g). Tran et al. (2017) synthesized graphene/α-MnO2 nanowire hydrogel with a high specific capacitance (397 F/g) at 1.0 A/g. Meng et al. (2018) designed a glucose and ammonia reduction system to synthesize δ-MnO2/graphene hydrogel with a capacity of 200.6 F/g.
As a transition metal hydroxide, Ni(OH)2 have been commonly used. In view of the structure, the Ni(OH)2 nanoplate (Mao et al., 2016) and nanoflower structure (Wang et al., 2016) have been designed to form 3D graphene-based frameworks, and the capacitance can be achieve to 782 F/g at 0.2 A/g and 1,632 F/g at 1 A/g, respectively. Recently, Li et al. (2019) coupled Ni(OH)2 nanosheets with nitrogen-enriched graphene hydrogel, which featured a specific capacitance of 896 F/g at 0.5 A/g.
Besides, in consideration of the advantage of Co and Ni, the strategy of combining Ni with Co was developed. Hwang et al. (2018) embedded Ni-Co hydroxide nanoneedles in graphene hydrogel, and the nanocomposite exhibited a specific capacitance of 544 C/g at 2 A/g. Tiruneh et al. (2018) designed a binder-free hybrid graphene hydrogel with nickel cobalt sulfide embedded, which can exhibit a capacity of about 1,000 F/g at 0.75 A/g with outstanding stability.
In addition, as one of the most promising nanomaterials, TiO2 has also been focused. Liu et al. (2017) obtained rice-like TiO2/graphene composite hydrogel, and the interaction between TiO2 nanoparticles and graphene hydrogel can endow the composite hydrogel with superior physicochemical properties, resulting in an excellent capacity of 372.3 F/g at 0.2 A/g. In addition, to realize synergic effect of organic and inorganic materials, Zhang et al. (2018b) synthesized RuO2/graphene hydrogel, and then adsorbed 1,4-naphthoquinone (NQ) molecules onto the hydrogel to form an hybrid graphene hydrogel, and the hydrogel containing ~14.6% RuO2 also can show a superior specific capacitance of 450.8 F/g.
Graphene-Polymer Composite Hydrogels Electrode Materials
Conductive polymers with the reverse doping-dedoping behavior have been extensively incorporated into 3D graphene hydrogels to fabricate high-performance electrodes. One of the successful conducting polymers is polyaniline (PANI), which has good environmental stability and high pseudocapcacitance. Various types of PANI including nanorods and nanowires have been used to form graphene/PANI composite hydrogel, and these hydrogels can show improved specific capacitance (Chen et al., 2017; Xu et al., 2017). To retain the essential features of the native hydrogel, the thin PANI layer wrapped on the graphene hydrogel by Gao et al. (2016) using an electrodeposition method, and the hydrogel displayed a good specific capacitance (710 F/g at 2 A/g). In addition, the hydrogel structure and the preparation temperature are also important. Wu et al. (2016) found that the phase-separated structure can produce much channels for electrolyte diffusion in PANI/graphene hydrogel, leading to a specific capacitance as high as 783 F/g at 27.3 A/g. Zou et al. (2018b) used m-phenylenediamine (mPD) to preserve the conjugated structure of PANI, and the composite hydrogel showed an improved capacity of 514.3 F/g. They also developed double-crosslinked network functionalized graphene/PANI hydrogel with high specific capacitance and mechanical strength (Zou et al., 2018a). For the preparation temperature, Ates et al. (2018) showed that the high capacitance can be kept at ~99% of its pristine value for the hydrogel prepared at 25°C, in comparison of that at 0°C.
Due to the ultrahigh theoretical capacitance and mechanical flexibility, polypyrrole (PPy) has also attracted considerable attention. Wu and Lian (2017) synthesized graphene/PPy hydrogel with a specific capacitance of 363 F/cm3 at 1.0 mA/cm3 using hydroquinone as a functionalized molecule. Since the ion transportation may be restricted by the compact morphology of PPy/graphene composite, the strategy of PPy wrapped graphene was developed. Pattananuwat and Aht-ong (2017) controlled nanoporous structure for PPy coated on graphene hydrogel surface with aiding of the surfactant, and a high specific capacitance (640.8 F/g at 1 A/g) can be achieved. They also used poly(3,4-ethylenedioxythiophene) (PEDOT) with PPy to form the synergistic effect, and the resultant hydrogel exhibited a specific capcacitance of 342 F/g at 0.5 A/g (Pattananuwat and Aht-ong, 2016). Recently, a hybrid PPy/rGO hydrogel in-situ electropolymerization preparation of PPy on the outside layer of graphene was reported by Zhang et al. (2019), and the specific capacitance is 340 F/g at 1 A/g.
Except for PANI and PPy, biopolymer such as lignosulfonate that a derivate of lignin can be regarded as a candidate for electrode material due to its electroactive components. Xiong et al. (2016) prepared lignosulfonate/graphene hydrogel with a maximum capacity of 549.5 F/g at 1 A/g. Li et al. (2017a) also reported lignosulfonate functionalized graphene hydrogels, which can present a specific capacitance of 432 F/g. These renewable composite hydrogels exhibit great potential in supercapacitor as electrodes materials.
Doped Graphene Composite Hydrogels Electrode Materials
As known, chemical doping is an effective strategy to modify the intrinsic properties of the materials. For graphene materials, nitrogen doping has been found to be an important method to provide graphene composite with high capacitance (Jin et al., 2019). For example, Liao et al. (2016) used urea and a small amount of ammonia to prepare N-doped graphene hydrogel, and the different N-types in graphene can resulted in an excellent specific capacitance (387.2 F/g at 1 A/g). Except for urea and ammonia, other materials containing N also have been used. Jiang et al. (2016) designed N-doped graphene hydrogel with a good specific capacitance (167.7 F/g at 1 A/g) by using carbohydrazide. Liu et al. (2016) used ammonium bicarbonate to prepared porous N-doped graphene hydrogel, and the hydrogel with high N content (10.8 at%) showed a high specific capacitance of 194.4 F/g. Gao et al. (2019) synthetized nitrogen-doped graphene-based hydrogels with concentrated sulfuric acid and o-phenylenediamine (oPDA), and the optimal hydrogel showed specific capacitance about 519.8 F/g.
Compared to single-atom doping, multiple doping can exhibit a synergetic effect and further improve the capacitive behavior of the materials. Typically, nitrogen and sulfur can be doped into graphene concurrently. After co-doping, the pseudo capacitance of the graphene will be increase, because of the redox faradic reactions existed at nitrogen-containing groups and sulf-containing species. Tran et al. (2016) outlined that N and S co-doped graphene hydrogel with holy defect can show a wonderful specific capacitance of 538 F/g at 0.5 mV/s, and the electrochemical property of the hydrogel can be modulated by the level of N and S doping. Li et al. (2017b) synthesized N/S co-doped graphene hydrogels with hierarchical pores, which can demonstrate a very good specific capacitance (251 F/g at 0.5 A/g) surprisingly. Zhang et al. (2018a) developed a one-step method to synthesize N/S co-doped graphene hydrogel, and the as-prepared hydrogel can show a capacity of 1,063 C/g at 1 A/g. Kong et al. (2018) constructed N,S-codoped graphene hydrogel with 3D hole, and the abundant in-plane pores leading to an outstanding specific capacitance of 320.0 F/g at 1 A/g.
Concluding Remarks
In this work, the recent advances in three-dimensional graphene-based composite hydrogel were reviewed in term of their use as flexible supercapacitors electrode materials. As can be seen, much effort has been devoted in the field of 3D graphene-based composite hydrogel electrode materials. A variety of materials including metals, polymers, dopants have been reported to construct high performance graphene-based composite hydrogel, and the excellent capacitive behavior of the typical composite hydrogel can be achieved (Table 1). Nevertheless, a massive effort is still needed before real practical applications become possible. For one, the exhibited capacitance values of the hydrogel still need to be further enhanced. In particular, large scale production of graphene-based composite hydrogel with high capacitance and quality is still a challenge. More importantly, for industry-level applications, technical movements should focus on more cost-effective and straightforward approaches for the fabrication of 3D graphene-based composite hydrogel rather than the design of complicated nanomaterials. It is believed that continuous breakthroughs in the graphene-based composite hydrogel will be made with the further research and development in this exciting field, and the hydrogel can play a great role in flexible capacitive devices in the near future.
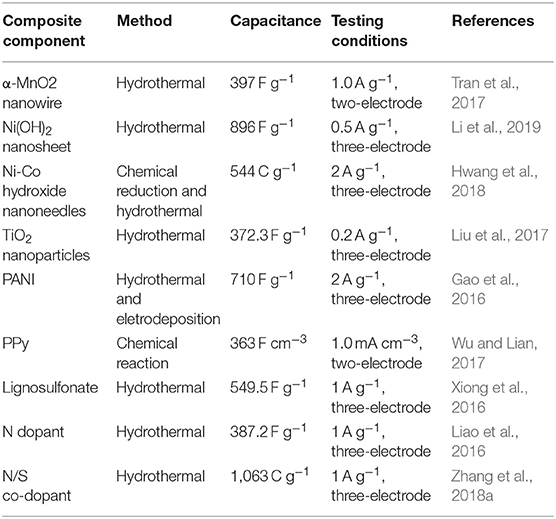
Table 1. Characteristics of typical graphene-based composite hydrogel for supercapacitor electrodes.
Author Contributions
All authors listed have made a substantial, direct and intellectual contribution to the work, and approved it for publication.
Funding
This work was supported by the Natural Science Foundation of Guangxi Province (No. 2018GXNSFBA281084) and the Middle-aged and Young Teachers Basic Ability Promotion Project of Guangxi (No.2019KY0371).
Conflict of Interest
The authors declare that the research was conducted in the absence of any commercial or financial relationships that could be construed as a potential conflict of interest.
References
Ates, M., El-Kady, M., and Kaner, R. B. (2018). Three-dimensional design and fabrication of reduced graphene oxide/polyaniline composite hydrogel electrodes for high performance electrochemical supercapacitors. Nanotechnology 29:175402. doi: 10.1088/1361-6528/aaae44
Chen, J., Song, J., and Feng, X. (2017). Facile synthesis of graphene/polyaniline composite hydrogel for high-performance supercapacitor. Polym. Bull. 74, 27–37. doi: 10.1007/s00289-016-1695-2
Gao, S., Zhang, L., Qiao, Y., Dong, P., Shi, J., and Cao, S. (2016). Electrodeposition of polyaniline on three-dimensional graphene hydrogel as a binder-free supercapacitor electrode with high power and energy densities. RSC Adv. 6, 58854–58861. doi: 10.1039/C6RA06263F
Gao, X., Han, G., Song, H., Chang, Y., Xiao, Y., Zhang, Y., et al. (2019). Purified nitrogen-doped reduced graphene oxide hydrogels for high-performance supercapacitors. J. Electroanal. Chem. 834, 206–215. doi: 10.1016/j.jelechem.2019.01.004
Horn, M., Gupta, B., MacLeod, J., Liu, J., and Motta, N. (2019). Graphene-based supercapacitor electrodes: addressing challenges in mechanisms and materials. Curr. Opin. Green Sust. Chem. 17, 42–48. doi: 10.1016/j.cogsc.2019.03.004
Hwang, M., Kang, J., Seong, K., Kim, D. K., Jin, X., Antink, W. H., et al. (2018). Ni-Co hydroxide nanoneedles embedded in graphene hydrogel as a binder-free electrode for high-performance asymmetric supercapacitor. Electrochim. Acta. 270, 156–164. doi: 10.1016/j.electacta.2018.03.075
Jiang, M., Xing, L. B., Zhang, J. L., Hou, S. F., Zhou, J., Si, W., et al. (2016). Carbohydrazide-dependent reductant for preparing nitrogen-doped graphene hydrogels as electrode materials in supercapacitor. Appl. Surf. Sci. 368, 388–394. doi: 10.1016/j.apsusc.2016.02.009
Jin, Y., Meng, Y., Fan, W., Lu, H., Liu, T., and Wu, S. (2019). Free-standing macro-porous nitrogen doped graphene film for high energy density supercapacitor. Electrochim. Acta 318, 865–874. doi: 10.1016/j.electacta.2019.06.107
Kong, W., Zhu, J., Zhang, M., Liu, Y., and Hu, J. (2018). Three-dimensional N- and S-codoped graphene hydrogel with in-plane pores for high performance supercapacitor. Micropor. Mesopor. Mat. 268, 260–267. doi: 10.1016/j.micromeso.2018.04.029
Li, F., Wang, X., and Sun, R. (2017a). A metal-free and flexible supercapacitor based on redox-active lignosulfonate functionalized graphene hydrogels. J. Mater. Chem. A 5, 20643–20650. doi: 10.1039/C7TA03789A
Li, J., Hao, H., Wang, J., Li, W., and Shen, W. (2019). Hydrogels that couple nitrogen-enriched graphene with Ni(OH)2 nanosheets for high-performance asymmetric supercapacitors. J. Alloy. Compd. 782, 516–524. doi: 10.1016/j.jallcom.2018.12.188
Li, J., Zhang, G., Fu, C., Deng, L., Sun, R., and Wong, C. P. (2017b). Facile preparation of nitrogen/sulfur co-doped and hierarchical porous graphene hydrogel for high-performance electrochemical capacitor. J. Power Sources 345, 146–155. doi: 10.1016/j.jpowsour.2017.02.011
Li, W., Zhang, B., Lin, R., Ho-Kimura, S., He, G., Zhou, X., et al. (2018). A dendritic nickel cobalt sulfide nanostructure for alkaline battery electrodes. Adv. Funct. Mater. 28, 1705937. doi: 10.1002/adfm.201705937
Liao, Y., Huang, Y., Shu, D., Zhong, Y., Hao, J., He, C., et al. (2016). Three-dimensional nitrogen-doped graphene hydrogels prepared via hydrothermal synthesis as high-performance supercapacitor materials. Electrochim Acta 194, 136–142. doi: 10.1016/j.electacta.2016.02.067
Liu, D., Fu, C., Zhang, N., Zhou, H., and Kuang, Y. (2016). Three-dimensional porous nitrogen doped graphene hydrogel for high energy density supercapacitors. Electrochim. Acta 213, 291–297. doi: 10.1016/j.electacta.2016.07.131
Liu, Y., Gao, T., Xiao, H., Guo, W., Sun, B., Pei, M., et al. (2017). One-pot synthesis of rice-like TiO2/graphene hydrogels as advanced electrodes for supercapacitors and the resulting aerogels as high-efficiency dye adsorbents. Electrochim. Acta 229, 239–252. doi: 10.1016/j.electacta.2017.01.142
Lu, H., Zhang, S., Guo, L., and Li, W. (2017). Applications of graphene-based composite hydrogels: a review. RSC Adv. 7, 51008–51020. doi: 10.1039/C7RA09634H
Ma, H., Zhou, Q., Wu, M., Zhang, M., Yao, B., Gao, T., et al. (2018). Tailoring the oxygenated groups of graphene hydrogel for high-performance supercapacitors with large areal mass loadings. J. Mater. Chem. A 6, 6587–6594. doi: 10.1039/C7TA10843E
Mao, L., Guan, C., Huang, X., Ke, Q., Zhang, Y., and Wang, J. (2016). 3D graphene-nickel hydroxide hydrogel electrode for high-performance supercapacitor. Electrochim. Acta 196, 653–660. doi: 10.1016/j.electacta.2016.02.084
Meng, X., Lu, L., and Sun, C. (2018). Green synthesis of three-dimensional MnO2/graphene hydrogel composites as a high-performance electrode material for supercapacitors. ACS Appl. Mater. Interf. 2018, 16474–16481. doi: 10.1021/acsami.8b02354
Pattananuwat, P., and Aht-ong, D. (2016). One-step method to fabricate the highly porous layer of poly (pyrrole/(3, 4-ethylenedioxythiophene)/) wrapped graphene hydrogel composite electrode for the flexibile supercapacitor. Mater. Lett. 184, 60–64. doi: 10.1016/j.matlet.2016.08.031
Pattananuwat, P., and Aht-ong, D. (2017). Controllable morphology of polypyrrole wrapped graphene hydrogel framework composites via cyclic voltammetry with aiding of poly (sodium 4-styrene sulfonate) for the flexible supercapacitor electrode. Electrochim. Acta 224, 149–160. doi: 10.1016/j.electacta.2016.12.036
Sattar, T. (2019). Current review on synthesis, composites and multifunctional properties of graphene. Topics Curr. Chem. 377:10. doi: 10.1007/s41061-019-0235-6
Tiruneh, S. N., Kang, B. K., Kwag, S. H., Lee, Y. H., Kim, M., and Yoon, D. H. (2018). Synergistically active NiCo2S4 nanoparticles coupled with holey defect graphene hydrogel for high-performance solid-state supercapacitors. Chem-Eur. J. 24, 3263–3270. doi: 10.1002/chem.201705445
Tran, N. Q., Kang, B. K., Woo, M. H., and Yoon, D. H. (2016). Enrichment of pyrrolic nitrogen by hole defects in nitrogen and sulfur co-doped graphene hydrogel for flexible supercapacitors. ChemsusChem 9, 2261–2268. doi: 10.1002/cssc.201600668
Tran, T. S., Tripathi, K. M., Kim, B. N., You, I. K., Park, B. J., Han, Y. H., et al. (2017). Three-dimensionally assembled graphene/α-MnO2 nanowire hybrid hydrogels for high performance supercapacitors. Mater. Res. Bull. 96, 395–404. doi: 10.1016/j.materresbull.2017.04.012
Wang, R., Jayakumar, A., Xu, C., and Lee, J. M. (2016). Ni(OH)2 nanoflowers/graphene hydrogels: a new assembly for supercapacitors. ACS Sustainable Chem. Eng. 4, 3736–3742. doi: 10.1021/acssuschemeng.6b00362
Wu, J., Zhang, Q., Zhou, A., Huang, Z., Bai, H., and Li, L. (2016). Phase-separated polyaniline/graphene composite electrodes for high-rate electrochemical supercapacitors. Adv. Mater. 28, 10211–10216. doi: 10.1002/adma.201601153
Wu, X., and Lian, M. (2017). Highly flexible solid-state supercapacitor based on graphene/polypyrrole hydrogel. J Power Sources 362, 184–191. doi: 10.1016/j.jpowsour.2017.07.042
Xiong, C., Zhong, W., Zou, Y., Luo, J., and Yang, W. (2016). Electroactive biopolymer/graphene hydrogels prepared for high-performance supercapacitor electrodes. Electrochim. Acta 211, 941–949. doi: 10.1016/j.electacta.2016.06.117
Xu, H., Liu, J., Chen, Y., Li, C. L., Tang, J., and Li, Q. (2017). Synthesis of three-dimensional nitrogen-doped graphene/polyaniline hydrogels for high performance supercapacitor application. J. Mater. Sci-Mater. EL 28, 10674–10683. doi: 10.1007/s10854-017-6842-5
Xu, K., Li, S., Yang, J., and Hu, J. (2018). Hierarchical hollow MnO2 nanofibers with enhanced supercapacitor performance. J. Colloid Interf. Sci. 513, 448–454. doi: 10.1016/j.jcis.2017.11.052
Xu, K., Shen, Y., Zhang, K., Yang, F., Li, S., and Hu, J. (2019). Hierarchical assembly of manganese dioxide nanosheets on one-dimensional titanium nitride nanofibers for high-performance supercapacitors. J. Colloid Interf. Sci. 552, 712–718. doi: 10.1016/j.jcis.2019.05.093
Zhang, N., Fu, C., Liu, D., Li, Y., Zhou, H., and Kuang, Y. (2016). Three-dimensional pompon-like MnO2/graphene hydrogel composite for supercapacitor. Electrochim. Acta 210, 804–811. doi: 10.1016/j.electacta.2016.06.004
Zhang, W., Chen, Z., Guo, X., Jin, K., Wang, Y. X., Li, L., et al. (2018a). N/S co-doped three-dimensional graphene hydrogel for high performance supercapacitor. Electrochim. Acta 278, 51–60. doi: 10.1016/j.electacta.2018.05.018
Zhang, X., Zhang, J., Chen, Y., Cheng, K., Yan, J., Zhu, K., et al. (2019). Freestanding 3D Polypyrrole@reduced graphene oxide hydrogels as binder-free electrode materials for flexible asymmetric supercapacitors. J. Colloid Interf Sci. 536, 291–299. doi: 10.1016/j.jcis.2018.10.044
Zhang, Z., Guo, B., Wang, X., Li, Z., Yang, Y., and Hu, Z. (2018b). Design and synthesis of an organic (naphthoquinone) and inorganic (RuO2) hybrid graphene hydrogel composite for asymmetric supercapacitors. New J. Chem. 42, 14956–14964. doi: 10.1039/C8NJ01908H
Zou, Y., Liu, R., Zhong, W., and Yang, W. (2018a). Mechanically robust double-crosslinked network functionalized graphene/polyaniline stiff hydrogels for superior performance supercapacitors. J. Mater. Chem. A 6, 8568–8578. doi: 10.1039/C8TA00860D
Keywords: flexible supercapacitor, electrode materials, grahpene-based hydrogel, three-dimensional architecture, composite materials
Citation: Lai E, Yue X, Ning W, Huang J, Ling X and Lin H (2019) Three-Dimensional Graphene-Based Composite Hydrogel Materials for Flexible Supercapacitor Electrodes. Front. Chem. 7:660. doi: 10.3389/fchem.2019.00660
Received: 15 August 2019; Accepted: 17 September 2019;
Published: 01 October 2019.
Edited by:
Wenyao Li, Shanghai University of Engineering Sciences, ChinaCopyright © 2019 Lai, Yue, Ning, Huang, Ling and Lin. This is an open-access article distributed under the terms of the Creative Commons Attribution License (CC BY). The use, distribution or reproduction in other forums is permitted, provided the original author(s) and the copyright owner(s) are credited and that the original publication in this journal is cited, in accordance with accepted academic practice. No use, distribution or reproduction is permitted which does not comply with these terms.
*Correspondence: Enping Lai, nemodhu@163.com; Haitao Lin, lhthost@163.com