- College of Chemistry, Chemical Engineering and Environmental Engineering, Liaoning Shihua University, Fushun, China
Na and cyano-group co-modified g-C3N4 was easily synthesized and its physicochemical property was completely analyzed. The results manifested that Na and cyano-group modification could heighten visible light absorbed ability and accelerate photoinduced charge separation. When resultant Na and cyano-group co-modified g-C3N4 was splitting water H2 evolution, its H2 evolution rate was obviously improved. Furthermore, it also kept excellent stable capacity of H2 evolution and stability of chemical structure. Hence, this present study does not only develop an efficient strategy to boost photocatalytic property of g-C3N4 based catalysts, but also provides useful guidance for designing more effective photocatalysts.
Introduction
In past decades, with excessive consumption of fossil energy (petroleum, coal, natural gas, etc.), human beings are facing enormous challenge in energy shortage. Hence, the utilization of new energy has been a hot topic. Among them, as a reproducible energy, solar energy exhibits some merits, such as generalization, abundance, clean. As a result, as one of methods for using solar energy, photocatalytic technology has attracted wide attention. All kinds of photocatalysts (TiO2, ZnO, SnO2, etc.) have been prepared and applied in photocatalytic filed (Chen and Mao, 2007; Shekofteh-Gohari et al., 2018). However, to adequately use solar energy, the development of more kind photocatalysts is of significance.
Recently, due to narrow band gap, low cost and easy preparation, graphitic carbon nitride (g-C3N4) has gained worldwide view (Wang et al., 2009; Liu et al., 2015). Whereas, unmodified g-C3N4 has many unfavorable factors, such as easy combination of photoinduced electrons and holes. It is difficult to meet the needs of industrialization. Therefore, to gain the improved photocatalytic performance, some strategies have been employed for modifying g-C3N4. One effective strategy was doped metal (Chen et al., 2009; Sun et al., 2017) or non-metal (Zhou et al., 2015; Li et al., 2016; Thaweesak et al., 2017). The separation rate of photogenerated charge could be effectively enhanced by this method. Other strategies were also applied for boosting photocatalytic performance of the g-C3N4. Zheng et al. produced hollow-like g-C3N4, its photocatalytic performance was improved via increasing specific surface area and enhancing light absorption (Zheng et al., 2016). Lin et al. modified the crystallinity and polymerization degree of g-C3N4 to improve photocatalytic property (Lin et al., 2018). Zhang et al. established isotype heterojunction to facilitate photogenerated charge separation, which could improve the efficiency of visible light photocatalytic splitting water to produce hydrogen (Zhang et al., 2012). And other g-C3N4 based heterojunctions, including BiOBr/g-C3N4 (Ye et al., 2013), g-C3N4/Bi2WO6 (Ge et al., 2011), MoS2/g-C3N4 (Ge et al., 2013), g-C3N4/TiO2 (Huang et al., 2015), ZnO/g-C3N4 (He et al., 2015), In2O3/g-C3N4 (Cao et al., 2014), FeOx/g-C3N4 (Cheng et al., 2016), were successfully established. Obviously, a great many of techniques were exploited to boost photocatalytic property of g-C3N4. From practical application perspective, the more modified methods are developed, the large-scale application is the simpler.
Therefore, in this paper, Na and cyano-group co-modified g-C3N4 was successfully synthesized. The results manifested that Na and cyano-group modification could heighten visible light absorbed ability and accelerate the photoinduced charge separation, when resulted Na and cyano-group co-modified g-C3N4 was applied for splitting water H2 evolution, its H2 evolution rate obviously higher than previous g-C3N4. Furthermore, it also kept excellent stable capacity of H2 evolution and stability of chemical structure. Hence, this present study does not only develop an effective strategy to boost the photocatalytic property of g-C3N4, but also supplies useful guidance for designing efficient photocatalysts.
Experimental
Synthesis of Catalysts
Melamine (Analytical Grade) and NaOH (Analytical Grade) were purchased in Sinopharm Chemical Reagent Co. Ltd, China. Deionized water was used in this study.
g-C3N4 was obtained by thermopolymerization of melamine (10 g) at 550°C for 120 min in a covered crucible under the air, and denoted as MCN. Na and cyano-group co-modified g-C3N4 was synthesized by following step: MCN (0.5 g) and NaOH (0.1 g) were grinding for 10 min. Resulting hybrid was heated at 450°C for 120 min under N2 atmosphere in tube furnace. After the tube furnace cool down, the product was washed by enough deionized water and ethanol and dried. The product was denoted as Na-CMCN.
Characterizations and Photocatalytic Experiments
The detail was supplied in Supporting Information.
Results and Discussion
In Figure 1, the XRD patterns of MCN and Na-CMCN were characterized. Both of them exhibited two peaks (13.1 and 27.4°). The stronger peak belonged to (002) peak, resulted from the interlayer stack of g-C3N4 sheets (Wang et al., 2010). The weak peak belonged to (100) peak, caused by in-plane packing of g-C3N4. By comparison, two peaks of Na-CMCN become weaker than MCN, demonstrating that g-C3N4-basic structure might be destroyed at a certain extent due to the introduction of NaOH.
The FTIR of as-prepared MCN and Na-CMCN were showed in Figure 2. The typical intensity peak of all samples showed at 808, 1,100–1,600, and 3,000–3,500 cm−1 three sections, respectively. The structure of heptazine rings was showed at the first section (Fang et al., 2017). The second section demonstrated the CN heterocycle and the –NH2 and –OH were reflected at the final section (Dante et al., 2011). Compared with MCN, 2,180 cm−1 for Na-CMCN could be found, which belonged to the cyano groups (C=N) (Liang et al., 2018; Yuan et al., 2018). This result meant that cyano groups could be introduced in g-C3N4-basic structure during the post-treatment at the existence of NaOH.
In addition, the surface chemical composition of MCN and Na-CMCN was measured by XPS in Figure 3. In C 1s spectrum (Figure 3A), 284.6 and 288.2 eV two peaks could be found. The first peak belonged to the outside elements of C, the last peak ascribed to N-C = N (Liang et al., 2019a). In addition, three components were exhibited in N 1s spectra at 400.9, 399.4, and 398.5 eV in Figure 3B, corresponding to the N-H or N-H2, the tertiary nitrogen and the sp2 hybridized CN in the heptazine rings, respectively (Chen et al., 2017; Wang et al., 2019). Interestingly, in Figure 3C, the peak was detected in the high-resolution Na 1s spectrum over Na-CMCN, but no peak was found in MCN, which denoted that Na element was successfully doped in the Na-CMCN basic structure. Furthermore, the Na spectra of Na-CMCN located at 1073.3 eV, which indicated that Na+ ions was doped in the g-C3N4 through Na-N bond (Sudrajat, 2018; Tripathi and Narayanan, 2019).
The electronic band structure for MCN and Na-CMCN was measured by electron paramagnetic resonance spectra (EPR) in Figure 4. Compared with MCN, the EPR signal intensity of Na-CMCN has clearly increased. This phenomenon indicated that more unpaired electrons were presented in the localized heterocyclic ring of Na-CMCN (Zhang and Wang, 2013).
The morphologies of the MCN and Na-CMCN were surveyed using SEM and TEM. MCN exhibited block structure in Figures 5A,C. For Na-CMCN, in Figure 5B, the part of block structure was desquamated. In Figure 5D, Na-CMCN presented block structure, besides several pores formed on its surface, which might be due to the NaOH corrosion.
In addition, N2 adsorption-desorption isotherms of the MCN and Na-CMCN were measured in Figure 6. Two photocatalysts presented similar IV N2 adsorption isotherms. The BET surface areas of MCN and Na-CMCN were 9.7 and 7.6 m2 g−1, respectively. There was not evident change for their surface areas.
Figure 7 demonstrates the light absorption of MCN and Na-CMCN. MCN and Na-CMCN presented the similar absorb edge, the main difference was that Na-CMCN possessed enhanced light absorption in comparison to MCN between 400 and 800 nm, which indicated that the improvement of visible light photocatalytic property over Na-CMCN might be anticipated.
The separation rate of photoinduced charge carriers for MCN and Na-CMCN were performed on photoluminescence (PL) curves in Figure 8A. Na-CMCN sample indicated the lower PL signal in comparison to MCN sample. The decreased peak intensity was resulted from the separation of the charge carries (Yu et al., 2014; Liang et al., 2019b). Evidently, Na-CMCN exhibited better separation effect of photoinduced charge. Furthermore, transient photocurrent responses of MCN and Na-CMCN were measure and shown in Figure 8B. A stable photocurrent response was demonstrated in each on and off cycle. The current density of the Na-CMCN was higher than that of the MCN, which indicates that the Na-CMCN had better separation ability of photoinduced charge (Tian et al., 2019). The result firmly confirmed the result of PL spectra.
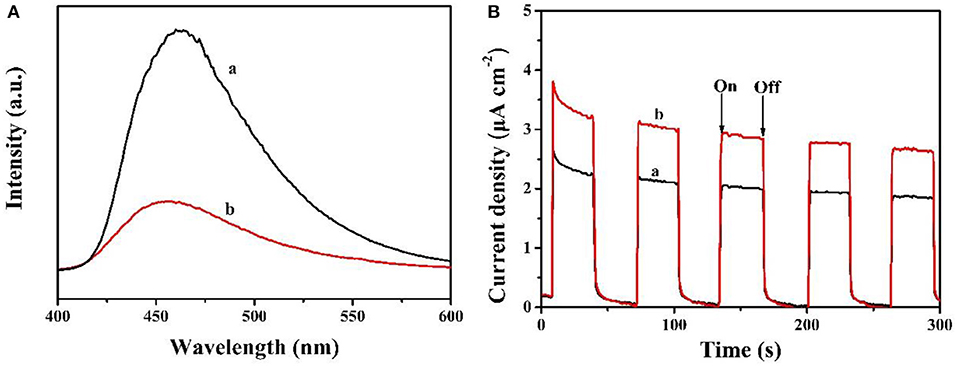
Figure 8. (A) Photoluminescence curves and (B) Transient photocurrent curves of (a) MCN and (b) Na-CMCN.
Then, the photocatalytic performance of as-prepared MCN and Na-CMCN was investigated. Figure 9A presents the photocatalytic capacity for splitting water H2 evolution over both of photocatalysts. With the extension of the illumination time, H2 was continuous produced. Obviously, Na-CMCN showed better performance for H2 evolution in the same time. Whereafter, to further compared their photocatalytic activity, Inset shows H2 evolution rate (HER) of both of photocatalysts. Obviously, Na-CMCN emerged higher activity for H2 evolution in the same time. Its HER was 3.07-folds more than MCN (985 vs. 320). Not only that, a recycled experiment for H2 evolution over as-prepared Na-CMCN was also measured. As shown in Figure 9B, after 3 times recycled experiments, Na-CMCN exhibited no significant deactivation, indicating that as-prepared Na-CMCN is an effective and steady visible light photocatalyst.
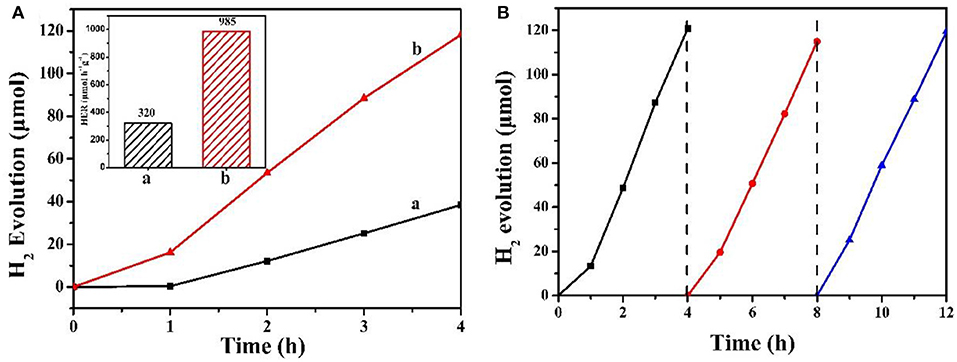
Figure 9. (A) Photocatalytic H2 evolution rate of (a) MCN and (b) Na-CMCN (Inset is HRE). (B) Stability test for H2 photosynthesis over Na-CMCN.
In addition, reacted Na-CMCN was analyzed using XRD and FTIR. The results were presented in Figure 10. By comparison, two distinct XRD diffraction peaks and infrared representative stretching vibration modes of fresh Na-CMCN and used Na-CMCN were almost changeless, implying that chemical structure of Na-CMCN maintained very steady.
Conclusion
In this paper, a facile and successful method was used to prepare Na and cyano-group co-modified g-C3N4 (Na-CMCN). The results found that Na and cyano-group modification could heighten visible light absorb ability and accelerate photoinduced charge separation, resulting that Na-CMCN exhibited higher HER than previous g-C3N4 (985 vs. 320). Not only that, it also maintained excellent stable capacity of H2 evolution and stability of chemical structure. As a result, this present study does not only develop an effective strategy to boost photocatalytic property of g-C3N4, but also supplies useful guidance for projecting efficient photocatalysts.
Data Availability Statement
All datasets generated for this study are included in the manuscript/Supplementary Files.
Author Contributions
GL: measurement of performance. LS: design of catalyst. SY and LY: characterization of catalyst.
Funding
This work was supported by basic research projects of Liaoning Provincial Education Department (No. L2017LQN004) and talent scientific research fund of LSHU (No. 2016XJJ-080).
Conflict of Interest
The authors declare that the research was conducted in the absence of any commercial or financial relationships that could be construed as a potential conflict of interest.
Supplementary Material
The Supplementary Material for this article can be found online at: https://www.frontiersin.org/articles/10.3389/fchem.2019.00639/full#supplementary-material
References
Cao, S. W., Liu, X. F., Yuan, Y. P., Zhang, Z. Y., Liao, Y. S., Fang, J., et al. (2014). Solar-to-fuels conversion over In2O3/g-C3N4 hybrid photocatalysts. Appl. Catalysis B 147, 940–946. doi: 10.1016/j.apcatb.2013.10.029
Chen, W., Hua, Y. X., Wang, Y., Huang, T., Liu, T. Y., and Liu, X. H. (2017). Two-dimensional mesoporous g-C3N4 nanosheet-supported MgIn2S4 nanoplates as visible-light-active heterostructures for enhanced photocatalytic activity. J. Catalysis 349, 8–18. doi: 10.1016/j.jcat.2017.01.005
Chen, X., and Mao, S. S. (2007). Titanium dioxide nanomaterials: synthesis, properties, modifications, and applications. Chem. Rev. 107, 2891–2959. doi: 10.1021/cr0500535
Chen, X., Zhang, J., Fu, X., Antonietti, M., and Wang, X. (2009). Fe-g-C3N4-catalyzed oxidation of benzene to phenol using hydrogen peroxide and visible light. J. Am. Chem. Soc. 131, 11658–11659. doi: 10.1021/ja903923s
Cheng, R., Zhang, L., Fan, X., Wang, M., Li, M., and Shi, J. (2016). One-step construction of FeOx modified g-C3N4 for largely enhanced visible-light photocatalytic hydrogen evolution. Carbon 101, 62–70. doi: 10.1016/j.carbon.2016.01.070
Dante, R. C., Martín-Ramos, P., Correa-Guimaraes, A., and Martín-Gil, J. (2011). Synthesis of graphitic carbon nitride by reaction of melamine and uric acid. Mater. Chem. Phys. 130, 1094–1102. doi: 10.1016/j.matchemphys.2011.08.041
Fang, W., Liu, J., Yu, L., Jiang, Z., and Shangguan, W. (2017). Novel (Na, O) co-doped g-C3N4 with simultaneously enhanced absorption and narrowed bandgap for highly efficient hydrogen evolution. Appl. Catalysis B 209, 631–636. doi: 10.1016/j.apcatb.2017.03.041
Ge, L., Han, C., and Liu, J. (2011). Novel visible light-induced g-C3N4/Bi2WO6 composite photocatalysts for efficient degradation of methyl orange. Appl. Catalysis B 108–109, 100–107. doi: 10.1016/j.apcatb.2011.08.014
Ge, L., Han, C., Xiao, X., and Guo, L. (2013). Synthesis and characterization of composite visible light active photocatalysts MoS2-g-C3N4 with enhanced hydrogen evolution activity. Int. J. Hydrog. Energy 38, 6960–6969. doi: 10.1016/j.ijhydene.2013.04.006
He, Y., Wang, Y., Zhang, L., Teng, B., and Fan, M. (2015). High-efficiency conversion of CO2 to fuel over ZnO/g-C3N4 photocatalyst. Appl. Catalysis B 168–169, 1–8. doi: 10.1016/j.apcatb.2014.12.017
Huang, Z., Sun, Q., Lv, K., Zhang, Z., Li, M., and Li, B. (2015). Effect of contact interface between TiO2 and g-C3N4 on the photoreactivity of g-C3N4/TiO2 photocatalyst: (0 0 1) vs. (1 0 1) facets of TiO2. Appl. Catalysis B 164, 420–427. doi: 10.1016/j.apcatb.2014.09.043
Li, J., Zhang, Y., Zhang, X., Huang, J., Han, J., Zhang, Z., et al. (2016). S, N dual-doped graphene-like carbon nanosheets as efficient oxygen reduction reaction electrocatalysts. ACS Appl. Mater. Interfaces 9, 398–405. doi: 10.1021/acsami.6b12547
Liang, L., Cong, Y., Yao, L., Wang, F., and Shi, L. (2018). One step to prepare Cl doped porous defect modified g-C3N4 with improved visible-light photocatalytic performance for H2 production and rhodamine B degradation. Mater. Res. Express 115:510. doi: 10.1088/2053-1591/aade38
Liang, L., Shi, L., and Wang, F. (2019a). Fabrication of large surface area nitrogen vacancy modified graphitic carbon nitride with improved visible-light photocatalytic performance. Diamond Relat. Mater. 91, 230–236. doi: 10.1016/j.diamond.2018.11.025
Liang, L., Shi, L., Wang, F., Yao, L., Zhang, Y., and Qi, W. (2019b). Synthesis and photo-catalytic activity of porous g-C3N4: promotion effect of nitrogen vacancy in H2 evolution and pollutant degradation reactions. Int. J. Hydrog. Energy 44, 16315–16326. doi: 10.1016/j.ijhydene.2019.05.001
Lin, L., Ren, W., Wang, C., Asiri, A. M., Zhang, J., and Wang, X. (2018). Crystalline carbon nitride semiconductors prepared at different temperatures for photocatalytic hydrogen production. Appl. Catalysis B 231, 234–241. doi: 10.1016/j.apcatb.2018.03.009
Liu, J., Liu, Y., Liu, N., Han, Y., Zhang, X., Huang, H., et al. (2015). Metal-free efficient photocatalyst for stable visible water splitting via a two-electron pathway. Science 347, 970–974. doi: 10.1126/science.aaa3145
Shekofteh-Gohari, M., Habibi-Yangjeh, A., Abitorabi, M., and Rouhi, A. (2018). Magnetically separable nanocomposites based on ZnO and their applications in photocatalytic processes: a review. Crit. Rev. Environ. Sci. Technol. 48, 806–857. doi: 10.1080/10643389.2018.1487227
Sudrajat, H. (2018). A one-pot, solid-state route for realizing highly visible light active Na-doped gC3N4 photocatalysts. J. Solid State Chem. 257, 26–33. doi: 10.1016/j.jssc.2017.09.024
Sun, B. W., Li, H. J., Yu, H. Y., Qian, D. J., and Chen, M. (2017). In situ synthesis of polymetallic Co-doped g-C3N4 photocatalyst with increased defect sites and superior charge carrier properties. Carbon 117, 1–11. doi: 10.1016/j.carbon.2017.02.063
Thaweesak, S., Wang, S., Lyu, M., Xiao, M., Peerakiatkhajohn, P., and Wang, L. (2017). Boron-doped graphitic carbon nitride nanosheets for enhanced visible light photocatalytic water splitting. Dalton Trans. 46, 10714–10720. doi: 10.1039/C7DT00933J
Tian, N., Xiao, K., Zhang, Y., Lu, X., Ye, L., Gao, P., et al. (2019). Reactive sites rich porous tubular yolk-shell g-C3N4 via precursor recrystallization mediated microstructure engineering for photoreduction. Appl. Catalysis B 253, 196–205. doi: 10.1016/j.apcatb.2019.04.036
Tripathi, A., and Narayanan, S. (2019). Skeletal tailoring of two-dimensional π-conjugated polymer (g-C3N4) through sodium salt for solar-light driven photocatalysis. J. Photochem. Photobiol. A 373, 1–11. doi: 10.1016/j.jphotochem.2018.12.031
Wang, X., Maeda, K., Thomas, A., Takanabe, K. G., Xin Carlsson, J. M., et al. (2009). A metal-free polymeric photocatalyst for hydrogen production from water under visible light. Nat. Mater. 8, 76–80. doi: 10.1038/nmat2317
Wang, Y., Zhang, J., Wang, X., Antonietti, M., and Li, H. (2010). Boron- and fluorine-containing mesoporous carbon nitride polymers: metal-free catalysts for cyclohexane oxidation. Angew. Chem. Int. Edn. 49, 3356–3359. doi: 10.1002/anie.201000120
Wang, Y., Zhou, X., Xu, W., Sun, Y., Wang, T., Zhang, Y., et al. (2019). Zn-doped tri-s-triazine crystalline carbon nitrides for efficient hydrogen evolution photocatalysis. Appl. Catalysis A 582:117118. doi: 10.1016/j.apcata.2019.117118
Ye, L., Liu, J., Jiang, Z., Peng, T., and Zan, L. (2013). Facets coupling of BiOBr-g-C3N4 composite photocatalyst for enhanced visible-light-driven photocatalytic activity. Appl. Catalysis B 142–143, 1–7. doi: 10.1016/j.apcatb.2013.04.058
Yu, J., Wang, K., Xiao, W., and Cheng, B. (2014). Photocatalytic reduction of CO2 into hydrocarbon solar fuels over g-C3N4-Pt nanocomposite photocatalysts. Phys. Chem. Chem. Phys. 16, 11492–11501. doi: 10.1039/c4cp00133h
Yuan, J., Liu, X., Tang, Y., Zeng, Y., Wang, L., Zhang, S., et al. (2018). Positioning cyanamide defects in g-C3N4: engineering energy levels and active sites for superior photocatalytic hydrogen evolution. Appl. Catalysis B 237, 24–31. doi: 10.1016/j.apcatb.2018.05.064
Zhang, G., and Wang, X. (2013). A facile synthesis of covalent carbon nitride photocatalysts by Co-polymerization of urea and phenylurea for hydrogen evolution. J. Catalysis 307, 246–253. doi: 10.1016/j.jcat.2013.07.026
Zhang, J., Zhang, M., Sun, R. Q., and Wang, X. (2012). A facile band alignment of polymeric carbon nitride semiconductors to construct isotype heterojunctions. Angew. Chem. Int. Edn. 51, 10145–10149. doi: 10.1002/anie.201205333
Zheng, D., Cao, X. N., and Wang, X. (2016). Precise formation of a hollow carbon nitride structure with a janus surface to promote water splitting by photoredox catalysis. Angew. Chem. Int. Edn. 55, 11512–11516. doi: 10.1002/anie.201606102
Keywords: graphitic carbon nitride, Na and cyano-group co-modification, photocatalysis, H2 evolution, sustainable
Citation: Liu G, Yan S, Shi L and Yao L (2019) The Improvement of Photocatalysis H2 Evolution Over g-C3N4 With Na and Cyano-Group Co-modification. Front. Chem. 7:639. doi: 10.3389/fchem.2019.00639
Received: 10 August 2019; Accepted: 05 September 2019;
Published: 19 September 2019.
Edited by:
Bingsen Zhang, Institute of Metals Research (CAS), ChinaReviewed by:
Yangming Lin, Max Planck Institute for Chemical Energy Conversion, GermanyXianmo Gu, Institute of Coal Chemistry (CAS), China
Copyright © 2019 Liu, Yan, Shi and Yao. This is an open-access article distributed under the terms of the Creative Commons Attribution License (CC BY). The use, distribution or reproduction in other forums is permitted, provided the original author(s) and the copyright owner(s) are credited and that the original publication in this journal is cited, in accordance with accepted academic practice. No use, distribution or reproduction is permitted which does not comply with these terms.
*Correspondence: Lei Shi, c2hpbGVpX2hpdCYjeDAwMDQwO3FxLmNvbQ==