- 1Department of Pure and Industrial Chemistry, University of Nigeria, Nsukka, Nigeria
- 2Department of Pharmaceutical and Medicinal Chemistry, University of Nigeria, Nsukka, Nigeria
Bacterial resistance to antibiotics has become one of the most challenging problems of infectious disease treatment. Ten new derivatives of benzenesulphonamide bearing carboxamide functionality were synthesized and investigated for their in vivo anti-inflammatory, in vitro anti-microbial and anti-oxidant activities. The base promoted reactions of the appropriate amino acids with substituted benzenesulphonyl chlorides gave the benzene sulphonamides (3a-j) in excellent yields. Palladium mediated amidation of the benzenesulphonamides (3a-j) and butylamine gave the new carboxamides (4a-j) in excellent yield. Compounds 4a and 4c inhibited carrageenan induced rat-paw edema at 94.69, 89.66, and 87.83% each at 1, 2, and 3 h, respectively. In the antimicrobial activity, compound 4d (MIC 6.72 mg/mL) was most potent against E. coli, compound 4h (MIC 6.63 mg/mL) was the most active against S. aureus, compound 4a (MIC 6.67 and 6.45 mg/mL) was most active against P. aeruginosa and S. typhi, respectively, compound 4f (MIC 6.63 mg/mL) was the most active against B. subtilis, compounds 4e and 4h (MIC 6.63 mg/mL) each were the most active against C. albicans, while compound 4e (MIC 6.28 mg/mL) was most active against A. niger. Only compound 4e (IC50 0.3287 mg/mL) had comparable activity with Vitamin C (IC50 0.2090 mg/mL).
Introduction
A major category of human diseases is bacterial infection. Resistance to almost all commercially available antibacterial drugs has been observed in both wild and laboratory strains of disease causing bacteria (Hayley and Paul, 2010). Prestinaci et al. (2015) reported four antibiotic-resistant pathogens of global concern, including S. aureus, K. pneumoniae, S. typhi, and M. tuberculosis. The resistance mechanisms are genetically encoded and under appropriate conditions, resistance genes can propagate through the environment (Christopher, 2000). This vast increase in resistance mechanisms often negates treatment by entire classes of antimicrobial compounds. Thus, the development of novel classes of antimicrobial compounds is urgently needed.
Reactive-Oxygen-Specie (ROS) is produced in an event of microbial invasion (Spooner and Yilmaz, 2011). Excess ROS can lead to oxidative stress (Circu and Aw, 2010). Some of these microorganisms are opportunistic pathogens implicated in chronic inflammatory conditions, including cystic fibrosis (Bylund et al., 2006).
Sulphonamides constitute an important class of drugs. They are quite stable and tolerated in human beings (Shet et al., 2013). They are the basis of several groups of drugs with various types of pharmacological agents possessing carbonic anhydrase inhibitory activity (Supuran, 2008), antibacterial (Ali et al., 2009), anticancer (Ghorab et al., 2014), anti-HIV (Selvam et al., 2008), antidiabetic (Hosseinzadeha et al., 2013), anti-influenza (Tang et al., 2011), antioxidant (Siddique et al., 2013), anti-inflammatory (Mahtab et al., 2014), antimicrobial (Chandak, 2012), antitrypanosomal (Papadopoulou et al., 2012), anticonvulsant (Bhat et al., 2006), anti-insomnia (Aissaoui et al., 2008), diuretics (Jainswal et al., 2004), and antileukemic (Nakayama et al., 2005) activities to mention but a few.
Carboxamides are ubiquitous in their function in drug molecules as a pharmacophore (Montalbetti and Falque, 2005). Carboxamides have been reported as antihelmintic (Ugwu et al., 2018a), antitubercular (Ugwu et al., 2014) anti-trypanosomal (Ugwu et al., 2018b) agents. They are also present in drug molecules used in the blockage of cholesterol synthesis (Graul and Castaner, 1997), treatment of hypertension and andina (Ananthanarayanan et al., 1993), blockade of angiotensin-II receptors (deGasparo and Whitebread, 1995), inhibition of angiotensin converting enzymes (Patchett, 1993), treatment of HIV (Roskoski, 2003), and management of heart disease (Hogan et al., 2000), to mention but a few.
This work was designed based on the reported biological activities of benzenesulphonamide derivatives and carboxamides and the need to develop newer antimicrobial agents that will have an added advantage of reducing the reactive oxygen species and inflammation implicated during microbial invasion while also acting as an antimicrobial agent using L-amino acids and substituted benzenesulphonamides.
We herein report the synthesis of some sulphonamides bearing carboxamide functionalities with good anti-inflammatory, antimicrobial, and comparable antioxidant activities.
Experimental
Synthesis of Substituted Benzene Sulphonamoylalkanamides (3a-j)
Sodium carbonate (NaCO3, 1.59 g, 15 mmol) was added to a solution of amino acids (2, 12.5 mmol) in water (15 mL) with continuous stirring until all the solutes dissolved. The solution was cooled to −5°C and the appropriate benzenesulphonyl chloride (1, 15 mmol) was added in four portions over a period of 1 h. The slurry was further stirred at room temperature for 4 h. The progress of the reaction was monitored using TLC (MeOH/DCM) 1:9). Upon completion, the mixture was acidified using 20% aqueous hydrochloric acid to pH2. The products (3a-j) were obtained in their analytical grade after washing with tartaric acid solution of pH 2.2. The products were dried over self-indicating fused silica gel in a desiccator (Ugwu et al., 2017).
Palladium catalyzed amidation of unactivated carboxylic acid and butylamine.
Butylamine (1.0 mmol) and Pd(dba)2 (0.1 mmol) at room temperature were added to a suspension of substituted benzenesulphonamides (3a-j, 1.0 mmol) in dry toluene (40 mL) equipped with Dean-Stark apparatus for azeotropic removal of water, and then refluxed for 12 h. On completion (as monitored by TLC) the amide products precipitated out in their pure form from the reaction mixture by adding 40 mL n-hexane. The carboxamides (4a-j) were obtained via suction filtration, washed with n-hexane and dried over fused silica gel or concentrated using rotary evaporator and dried over vacuum in the case of oily products (Scheme 1).
N-Butyl-1-[(4-Methylphenyl)Sulphonyl]Pyrolidine-2-Carboxamide (4a)
Yield (0.22 g, 66.7%), Mp, 128–130°C. UV (λmax): 213.00 nm(ε = 427.5 m2/mol). FTIR (KBr, cm−1): 3,160 (NH), 2,959 (C-H aromatic), 2,871, 2,795 (C-H aliphatic), 1,629 (C = O), 1,569, 1,450 (C = C), 1,342, 1,394 (2S = O), 1,200, 1,156 (SO2NH), 1,092, 1,055 (C-N)0.1 HNMR (DMSOd6, 400 MHz)δ: 7.68–7.64 (m, 2H, ArH), 7.35–7.33 (m, 2H, ArH), 3.82 (m, 1H, CH-C = O), 3.22 (m, 1H, CHa of CH2-N), 3.09–3.07 (m, 1H, CHb of CH2-N), 2.67 (m, 2H, CH2-NH), 2.24 (s, 3H, CH3-Ar), 1.69 (m, 2H, CH2CHC = O), 1.47 (m, 2H, CH2-CH2-N), 1.28–1.26 (m, 4H, 2CH2, CH2CH2-CH3), 0.85–0.80 (m, 3H, CH3-CH2) 0.13CNMR (DMSOd6, 400 MHz)δ: 175.22 (C = O), 143.17, 136.28, 130.07, 127.63 (four aromatic carbons), 63.71, 48.75, 38.99, 31.18, 30.29, 24.66, 21.50, 19.80,14.10 (nine aliphatic carbons). HRMS (m/z): 325.1588 (M+H), calculated, 325.1586.
N-Butyl-1-(Phenylsulphonyl)Pyrrolidine-2-Carboxamide (4b)
Yield (0.27 g, 87.1%), Mp, 114–116°C. UV (λmax): 202.00 nm(ε = 398.3 m2/mol). FTIR (KBr, cm−1): 3,489 (NH), 2,960 (C-H aromatic), 2,874, 2,796 (C-H aliphatic), 1,629 (C = O), 1,569, 1,446 (C = C), 1,394, 1,334 (2S = O), 1,193, 1,156 (SO2NH), 1,088, 1,014 (C-N)0.1HNMR (DMSOd6, 400 MHz)δ: 7.80–7.78 (d, J = 8.24 Hz, 2H, ArH), 7.62–7.52 (m, 3H, ArH), 3.88–3.86 (t, J = 3.42 Hz, 1H, CH-C = O), 3.14–3.11 (t, J = 6.88 Hz, 2H, CH2-N), 2.68–2.65 (m, 2H, CH2-NH), 1.70 (m, 2H, CH2-CH-C = O), 1.51–1.43 (m, 2H, CH2-CH2-N), 1.29–1.24 (m, 4H, 2CH2, CH2-CH2-CH3), 0.84–0.81 (m, 3H, CH3-CH2)0.13CNMR (DMSOd6, 400 MHz)δ: 174.99 (C = O), 139.20, 132.97, 129.61, 127.56 (four aromatic carbons), 63.74, 48.72, 31.18, 30.38, 24.67, 19.79, 14.11 (seven aliphatic carbons). HRMS (m/z): 311.1430 (M+H), calculated, 311.1429.
N-Butyl-4-Methyl-2-(4-Methylbenzenesulphonamido)Pentanamide (4c)
Yield (0.31 g, 88.6%), Mp, 110–112°C. UV (λmax): 202.00 nm(ε = 445.2 m2/mol). FTIR (KBr, cm−1): 3,250 (NH), 3,049 (C-H aromatic), 2,960, 2,866, 2,751 (C-H aliphatic), 1,640 (C = O), 1,580, 1,461 (C = C), 1,394, 1,342 (2S = O), 1,286, 1,159 (SO2NH), 1,099, 977 (C-N)0.1HNMR (DMSOd6, 400 MHz)δ: 8.18–8.16 (d, J = 8.40 Hz, 1H, NH), 7.86–7.80 (m, 2H, Ar), 6.72–6.70 (m, 2H, ArH), 3.36–3.35 (t, J = 4.40 Hz, 1H, CH-C = O), 3.16–3.14 (m, 2H, CH2-NH), 3.06–3,01 (m, 6H, 3CH2), 2.46 (s, 3H, CH3-Ar), 1.14–1.10 (m, 9H, 3CH3). 13CNMR (DMSOd6, 400 MHz)δ: 167.86 (C = O), 154.70, 137.58, 131.70, 127.16, 125.18, 123.91 (six aromatic carbons), 64.26, 52.65, 46.07, 39.32, 36.45, 8.93 (six aliphatic carbons). HRMS (m/z): 340.1822 (M+), calculated, 340.1821.
N-Butyl-4-Methyl-2-[(Phenylsulphonyl)Amino]Pentanamide (4d)
Yield (0.25 g, 78.1%), Mp, 116–118°C. UV (λmax): 202.00 nm(ε = 422.1 m2/mol). FTIR (KBr, cm−1): 3,258 (NH), 3,064 (C-H aromatic), 2,960, 2,870 (C-H aliphatic), 1,640 (C = O), 1,573, 1,428 (C = C), 1,387, 1,338 (2S = O), 1,290, 1,152 (SO2NH), 1,014, 973 (C-N)0.1HNMR (DMSOd6, 400 MHz)δ: 7.73–7.71 (d, J = 7.80 Hz, 2H, ArH), 7.56–7.47 (m, 3H, ArH), 3.19 (m, 1H, CH-C = O), 2.61–2.57 (m, 2H, CH2-NH), 1.72–1.65 (m, 1H, CH-(CH3)2), 1.44–1.38 (m, 2H, CH2-CHC = O), 1.31–1.18 (m, 4H, CH2), 0.91–0.87 (m, 3H, CH3), 0.82–0.72 (m, 6H, 2(CH3)). 13CNMR (DMSOd6, 400 MHz)δ: 174.30 (C = O), 141.38, 132.58, 129.37, 127.13 (four aromatic carbons), 56.48, 43.28, 29.77, 24.51, 23.47, 22.61, 19.72, 14.04 (eight aliphatic carbons). HRMS (m/z): 327.1744 (M+H), calculated, 327.1742.
N-Butyl-2-{[(4-Methylphenyl)Sulphonyl]Amino}-4-(Methylsulphanyl)Butanamide (4e)
Yield (0.36 g, 100%), Mp, 124–126°C. UV (λmax): 202.00 nm(ε = 399.4 m2/mol). FTIR (KBr, cm−1): 3,250 (NH), 3,049 (C-H aromatic), 2,960, 2,922, 2,870 (C-H aliphatic), 2,624 (S-CH3), 1,640 (C = O), 1,580, 1,521, 1,435, 1,402 (C = C), 1,361, 1,316 (2S = O), 1,208, 1,152 (SO2NH), 1,088, 1,047 (C-N)0.1HNMR (DMSOd6, 400 MHz)δ: 7.62–7.60 (d, J = 7.32 Hz, 2H, ArH), 7.32–7.30 (d, J = 7.92 Hz, 2H, ArH), 3.17–3.14 (t, J = 5.18 Hz, 1H, CH-C = O), 2.67–2.64 (m, 2H, CH2-NH), 2.39–2.32 (m, 3H, CH3-Ar), 2.25 (s, 3H, CH3-S), 1.99–1.92 (t, J = 5.64 Hz, 2H, CH2-S), 1.78–1.76 (m, 2H, CH2-CH), 1.46–1.39 (m, 2H, CH2-CH2-NH), 1.29–1.20 (m, 2H, CH2-CH3), 0.87–0.84 (t, J = 5.20 Hz, 3H, CH3-CH2). 13CNMR (DMSOd6, 400 MHz)δ: 172.08 (C = O), 143.00, 137.84, 130.00, 127.28 (four aromatic carbons), 56.68, 41.18, 38.90, 33.54, 29.88, 21.48, 19.67, 15.03, 14.04 (nine aliphatic carbons). HRMS (m/z): 357.1310 (M-H), calculated, 357.1307.
N-Butyl-2-(4-Methylphenylsulphonyl)-2-[(Phenylsulphonyl)Amino]Butanmide (4f)
Yield (0.30 g, 92%), Mp, 120–121°C. UV (λmax): 202.00 nm(ε = 406.9 m2/mol). FTIR (KBr, cm−1): 3,246 (NH), 3,067 (CH aromatic), 2,917, 2,918 (CH aliphatic), 2,657 (-S-CH3), 1,710 (C = O), 1,584, 1,416 (C = C aromatic),1,326, 1,233 (2S = O), 1,155, 1,088, 969, 928 (C-N)0.1HNMR (DMSOd6, 400 MHz)δ: 7.88–7.87 (d, J = 7.40 Hz, 2H, ArH), 7.65–7.64 (t, J = 7.32 Hz, 3H, ArH), 3.15–3.13 (t, J = 6.22 Hz7, 1H, CH-C = O), 2.66–2.64 (m, 2H, CH2-NH), 2.23 (s, 3H, CH3-S), 1.97–1.95 (m, 2H, CH2-S), 1.79–1.77 (m, 2H, CH2-CH), 1.42–1.38 (m, 2H, CH2-CH2-NH), 1.27–1.24 (m, 2H, CH2-CH3), 0.84–0.82 (t, J = 5.20 Hz, 3H, CH3-CH2). 13CNMR (DMSOd6, 400 MHz)δ: 173.38 (C = O), 143.46, 138.65, 130.50, 127.28 (four aromatic carbons), 56.68, 41.18, 38.90, 33.54, 29.88, 19.67, 15.03, 14.04 (eight aliphatic carbons). HRMS (m/z): 344.1229 (M+), calculated 344.1228.
N-Butyl-3-Hydroxy-2-{[(4-Methyl)Sulphonyl]Amino}Butanamide (4g)
Yield (0.28 g, 84.8%), Mp, 162–164°C. UV (λmax): 213.00 nm(ε = 421.7 m2/mol). FTIR (KBr, cm−1): 3,399 (OH), 3,250 (NH), 2,959 (C-H aromatic), 2,934, 2,874 (C-H aliphatic), 1,603 (C = O), 1,513, 1,465 (C = C), 1,379, 1,327 (2S = O), 1,159, 1,122 (SO2NH), 1,092, 1,036 (C-N, C-O)0.1HNMR (DMSOd6, 400 MHz)δ:7.69–7.57 (m, 2H, ArH), 7.48–7.46 (m, 2H, ArH), 3.77–3.76 (d, J = 6,32, Hz, 1H, CH-C = O), 3.08–3.06 (m, 1H, CH-OH), 2.56–2.53 (m, 2H, CH2-NH), 1.41–1.39 (m, 2H, CH2-CH2-NH), 1.33–1.31 (m, 2H, CH2-CH3), 0.98–0.96 (d, J = 5.96 Hz, 3H, CH3-CHOH), 0.83–0.81 (m, 3H, CH3-CH2). 13CNMR (DMSOd6, 400 MHz)δ: 172.64 (C = O), 142.44, 132.94, 128.71, 127.84 (four aromatic carbons), 68.01, 60.14, 38.95, 29.70, 21.56, 19.64, 19.09, 14.02 (eight aliphatic carbons). HRMS (m/z): 327.1380 (M-H), calculated, 328.1379.
N-Butyl-3-Hydroxy-2-[(Phenylsulphonyl)Amino]Butanamide (4h)
Yield (0.29 g, 90.6%), Mp, 140–142°C. UV (λmax): 213.00 nm(ε = 362.1 m2/mol). FTIR (KBr, cm−1): 3,401 (OH), 3,191 (NH), 3,056 (C-H aromatic), 2,960, 2,933, 2,870 (C-H aliphatic), 1,733 (C = O), 1,595, 1,528, 1,476 (C = C), 1,387, 1,305 (2S = O), 1,238, 1,141 (SO2NH), 1,077, 973 (C-N, C-O)0.1HNMR (DMSOd6, 400 MHz)δ: 7.75 (m, 2H, ArH), 7.59–7.51 (m, 3H, ArH), 3.70–3.69 (d, J = 4.56 Hz, 1H, CH-C = O), 3.082 (m, 1H, CH-OH), 2.67–2.65 (m, 2H, CH2-NH), 1.43–1.41 (m, 2H, CH2-CH2-NH), 1.28–1.22 (m, 2H, CH2-CH3), 0.92–0.89 (d, J = 5.96 Hz, 3H, CH3-CHOH), 0.84–0.79 (m, 3H, CH3-CH2). 13CNMR (DMSOd6, 400 MHz)δ: 171.60 (C = O), 140.44, 132.94, 129.61, 127.24 (four aromatic carbons), 68.01, 60.14, 38.95, 29.70, 19.64, 19.09, 14.02 (seven aliphatic carbons). HRMS (m/z): 315.1379 (M+H), calculated, 315.1378.
N-Butyl-3-Hydroxy-2-{[(4-Methylphenyl)Sulphonyl]Amino}Propanamide (4i)
Yield (0.29 g, 90.6%), Mp = 134–136°C. UV (λmax): 203.00 nm(ε = 330.9 m2/mol). FTIR (KBr, cm−1): 3,466 (OH), 3,243 (NH), 3,060 (C-H aromatic), 2,960, 2,933, 2,873 (C-H aliphatic), 1,733 (C = O), 1,595, 1,494, 1,461 (C = C), 1,365, 1,324 (2S = O), 1,163, 1,122 (SO2NH), 1,092, 1,033 (C-N, C-O)0.1HNMR (DMSOd6, 400 MHz)δ: 7.89–7.87 (d, J = 7.36 Hz, 1H, NH), 7.62–7.61 (d, J = 6.44 Hz, 2H, ArH), 7.32–7.30 (d, J = 6.44 Hz, 2H, ArH), 3.51–3.48 (m, 1H, CH), 3.30 (s-br, 1H, OH), 2.47–2.46 (m, 2H, CH2-NH), 2.33(s, 3H, CH3-Ar), 1.62–1.58 (m, 2H, CH2-OH), 1.34–1.29 (m, 2H, CH2-CH2-NH), 1.09–1.01 (m, 2H, CH2-CH3) 0.76–0.71 (t, J = 5.48 Hz, 3H, CH3-CH2)0.13CNMR (DMSOd6, 400 MHz)δ: 172.36 (C = O), 149.93, 147.24, 128.72, 124.78 (four aromatic carbons), 60.89, 37.32, 24.85, 21. 44, 19.54, 15.96, 11.46 (seven aliphatic carbons). HRMS (m/z): 314.1303 (M+), calculated, 314.1300.
N-Butyl-3-Hydroxy-2-[(Phenylsulphonyl)Amino]Propanamide (4j)
Yield (0.28 g, 93.3%), Mp = 120–122°C. UV (λmax): 203.00 nm(ε = 364.7 m2/mol). FTIR (KBr, cm−1): 3,391 (OH), 3,243 (NH), 2,963 (C-H aromatic), 2,875 (C-H aliphatic), 1,733 (C = O), 1,599, 1,446 (C = C), 1,387, 1,320 (2S = O), 1,252, 1,160 (SO2NH), 1,092, 1,026 (C-N, C-O)0.1HNMR (DMSOd6, 400 MHz)δ: 7.94–7.93 (d, J = 7.36 Hz, 1H, NH), 7.63–7.62 (d, J = 6.44 Hz, 2H, ArH), 7.31–7.29 (d, J = 6.40 Hz, 3H, ArH), 3.53–3.49 (m, 1H, CH), 3.30 (s-br, 1H, OH), 2.44–2.42 (m, 2H, CH2-NH), 1.58–1.56 (m, 2H, CH2-OH), 1.44–1.42 (m, 2H, CH2-CH2-NH), 1.06–1.04 (m, 2H, CH2-CH3) 0.66–0.64 (t, J = 6.32 Hz, 3H, CH3-CH2)0.13CNMR (DMSOd6, 400 MHz)δ: 170.76 (C = O), 148.43, 146.64, 127.74, 124.78 (four aromatic carbons), 63.44, 38.44, 37.32, 24.85, 15.96, 11.46 (six aliphatic carbons). HRMS (m/z): 299.1069 (M-H), calculated, 299.1066.
In silico Studies
Drug Targets
Peroxisomes are essential organelles which participate in multiple important metabolic processes, including the β-oxidation of fatty acids, plasmalogen synthesis, and the metabolism of reactive oxygen species (ROS) (Islinger et al., 2012). Human peroxiredoxin 5 (PRDX5) (PDB code: 1HD2), aperoxisome, is a thioredoxin reductase which reduces H2O2, alkyl hydroperoxides and peroxynitrite (Knoops et al., 2011). PRDX5 is a novel type of mammalian thioredoxin peroxidase widely expressed in tissues and located cellularly to mitochondria, peroxisomes, and cytosol. Functionally, PRDX5 has been implicated in antioxidant protective mechanisms as well as in signal transduction in cells (Declercq et al., 2001).
Phosphodiesterase-4 (PDE4) (PDB code: 4WCU) is an enzyme found in some specific celltypes, and is involved in the degradation of the second messenger, cAMP. As a result, 4WCU has a pivotal role in cell signaling. This has made it a target for clinical drug development of various indications, including anti-inflammation and several others (Zhang et al., 2005).
Glucosamine-6-phosphate synthase (GlcN-6-P) (PDB code: 2VF5) is a very useful target in antimicrobial chemotherapy as outlined by Ezeokonkwo et al. (2017) and Festus et al. (2018). 2VF5 is responsible for the metabolism of hexosamine which is an important process in the biosynthesis of amino sugars. In the biosynthesis of amino sugars, uridine 5′-diphospho-N-acetyl-d-glucosamine (UDP-GlcNAc) is formed. UDP-GlcNAc an important component of thepeptido glycan layer mostly found in the bacterial and fungal cell walls. Inactivation of GlcN-6-P synthase for a short period is very dangerous for fungal cells.
Molecular Docking Studies
Three drugs targets were selected to study the in silico antioxidant, anti-inflammatory, and antibacterial activities of the synthesized compounds. The targets used for the antioxidant and anti-inflammatory studies are the human peroxiredoxin 5 (PDB code: 1HD2) and phosphodiesterase 4 (PDE4) (PDB: 4WCU), respectively. Glucosamine-6-phosphate synthase (PDB Code: 2VF5) was used for antibacteria study.
The 3-Dimensional crystal structures of 1HD2, 4WCU, and 2VF5 with their co-crystallized ligands were retrieved from the protein data bank repository (https://www.rcsb.org/). These proteins were treated in Discovery Studio where multiple chains and water of crystallization were removed. The synthesized compounds were drawn using Accelrys Draw 4.1. Both the prepared proteins and compounds were energy minimized using MMFF94x force field. The energy minimized compounds were docked into the binding cavities of the proteins. The binding free energy for each compound against the target was calculated. Biovia Discovery Studio v16.1.0.15350 software was used for analysis of molecular docking studies. Molinspiration software (www.molinspiration.com) was used to generate the physicochemical properties in Table 7.
Biological Studies
In vivo Anti-inflammatory Activities Determination
Male albino rats weighing 300 g where purchased from the Department of Biochemistry, University of Nigeria, Nsukka, and kept at room temperature in a light controlled animal house. They were fasted with free access to water for at least 12 h prior to the experiments. The tested compounds were prepared as suspension in vehicle (0.5% methylcellulose) and celecoxib was used as a standard drug. The positive control received celecoxib while the negative control received only the vehicle. Oedema was produced by injecting 0.2 mL of a solution of 1% carrageenan in the hindpaw. The rats were injected intraperitoneally with 1 mL suspension in 0.5% methylcellulose of the tested compounds and reference drug. Paw volume was measured by water displacement with aplethysmometer (UGO BASILE) before, 0.5, 1, 2, and 3 h after treatment. The percentage was calculated by the following equation (Abdel-Aziz et al., 2014):
where D represents the difference in paw volume before and after drug administration to the rats and C represents the difference of volume in the control groups. The approval for the use of animal was obtained from the University of Nigeria committee on experimental animal use.
In vitro Antimicrobial Activity
The antimicrobial properties of the novel compounds were investigated by general sensitivity testing and minimum inhibitory concentration (MIC), with respect to freshly cultured targeted organisms. The seven organisms used in this study are two Gram positive bacteria [Staphylococcus aureus (ATCC 12600), Bacillus subtilis (ATCC 6633)], three gram negative bacteria [Eschericha coli (O157:H7), Pseudomonas aeruginosa (ATCC 27853), Salmonellatyphi (H56)], and two fungi [Candida albicans (ATCC MYA 2876), Aspergillus niger (ATCC 16404)] were obtained from the Department of Pharmaceutics, University of Nigeria, Nsukka.
Antimicrobial Sensitivity Testing
Sensitivity test agar plates were seeded with 0.1 mL of overnight culture of microorganism. The seeded plates were allowed to set after which cups were made in each sector previously drawn on the backside of the bottom plate using marker. Using a sterile pipette, each cup was filled with six drops of their corresponding carboxamides (100 mg/mL). The solubility solvent was DMF. All the plates were incubated at 37°C for 24 h for bacteria and 48 h for fungi. Zones of clearance around each cup allowed inhibition and the diameter of such zones to be measured. The procedure was repeated for tetracycline (standard bacteria), fluconazole (fungi standard), and DMF (solvent). Muller Hinton agar was used for the fungi in place of nutrient agar for bacteria (Adeniyi and Odedola, 1996).
Minimum Inhibitory Concentration (MIC) Testing
Serial dilutions of the carboxamides were prepared from 100 mg/mL solution of the compounds to give 100, 50, 25, and 12.5 mg/mL. Six drops of each dilution was added to the corresponding cup of seeded microorganisms and the agar previously marked. The cork borer used to make the cup is 8 mm in diameter. The plates were incubated at 37°C for 24 and 48 h in the case of fungi. The diameter of the zone of inhibition was measured and the value subtracted from the diameter of the borer to give the inhibition zone diameter (IZD). The graph of IZD (Prestinaci et al., 2015) against the log of concentration was plotted for each plate containing a specific compound and a microorganism. The anti-log of the intercept on x-axis gives the MIC (Adeniyi and Odedola, 1996). The procedure was repeated for tetracycline and fluconazole.
In vitro Antioxidant Studies
DPPH Radical Scavenging Activity
The new carboxamides were screened for free radical scavenging activity by 2,2-diphenyl-1-picrylhydrazyl (DPPH) method (Liyana-Pathiranan and Shahidi, 2005). Compounds of different concentrations were prepared in distilled ethanol, with 1 mL of each compound solutions having different concentrations (1.0, 2.0, 3.0, 4.0, and 5.0 mg/mL) were taken indifferent test tubes, 4 mL of 0.1 mM ethanol solution of DPPH was added and shaken vigorously. The test tubes were then incubated in a dark room temperature for 20 min. A DPPH blank was prepared without the compound and ethanol was used for the baseline correction. Changes (decrease) in the absorbance at 517 nm were measured using a UV-Visible spectrometer. The radical scavenging activities were expressed as the inhibition percentage and were calculated using:
Where Ac, Absorbance of control; As, Absorbance of sample.
Results and Discussion
Chemistry
Substituted benzenesulphonamides (3a-j) were synthesized from the reaction of various L-amino acids (2) and substituted benzenesulphonyl chloride (1) in aqueous medium. Reaction of compounds (3a-j) with the appropriate alkyl amine in the presence of catalytic amount of Pd2(dba)3 afforded the target compounds (4a-j, Scheme 2) which were characterized using FTIR, NMR, and HRMS.
Spectral Characterization
The FTIR spectra of the carboxamides showed a N-H band between 3,489 and 3,160 cm−1. The C = O band appeared between 1,733 and 1,603 cm−1. These bands indicates successful coupling of the aliphatic amines with the benzenesulphonamides.
The appearance of the peaks between 3.16–3.01, 2.86–2.42, 1.92–1.01, and 0.92–0.64 ppm in the proton NMR is supportive of the formation of the target product.
The carbon-13 NMR showed all the peaks expected of successful coupled products. The C = O peak appeared between 167.86 and 175.22 ppm. All the aromatic and aliphatic peaks were accounted for in the carbon-13 NMR.
The high resolution mass spectrometer (HRMS) peak of the derivatives appeared either as molecular ions (M+), M+H+, or M–H−. The results corresponded to three decimals with the calculated values. The spectra used for the characterization of the new compounds are available as Supporting Materials.
Molecular Docking
Table 1 shows the binding free energy of our synthesized compounds docked into the binding sites of the receptors: 1HD2, 4WCU, and 2VF5. There were significant binding affinities of the compounds with the receptors when compared to the standard drugs. Compound 4e showed comparable in silico antioxidant activity (−13.05 kcal/mol) as the standard drug (vitamin C) (−13.04 kcal/mol). Likewise, compound 4g also showed similar in silico anti-inflammatory (−11.20 kcal/mol) comparable to indomethacin (−11.38 kcal/mol). However, even though the compounds showed good binding affinity with 2VF5, none showed antibacterial activity that is comparable to ciprofloxacin. We went further to gain insight into the nature of the binding interactions between the compounds and the receptors. Figure 1 shows the stereo view of compound 4e in the binding cavity of 1HD2, while Figure 2 illustrates how the atoms of compound 4e interacted with the amino acid residues of 1HD2.
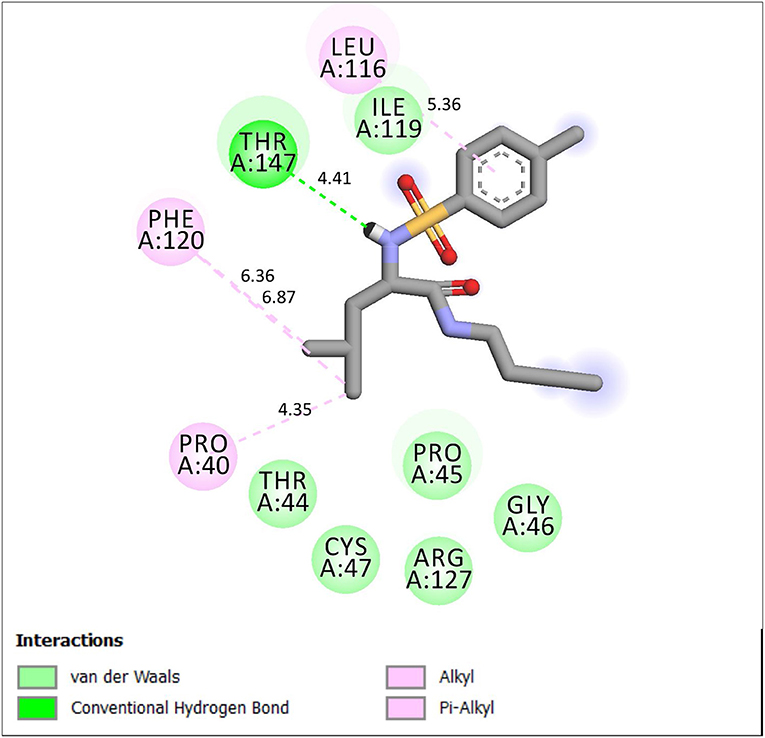
Figure 2. 2D representation of binding interaction of compound 4e and the amino acid residues of 1HD2.
Compound 4e is well-fitted in the binding cavity of 1HD2, sharing the same binding sites with the native ligand. It can be seen from Figure 2 that various chemical interactions played vital roles. There was H-bond interaction between the compound and THR 147 through a H-bond distance of 4.47Å. The π-electrons of the methylphenyl group interacted with LEU 116 through π-alkyl interaction. Other π-alkyl interactions involved PHE 120 and PRO 40. ILE 119, PRO 45, THR 45, and GLY 46 can hydrophobically interact with compound 4e.
Compound 4g chemically interacted with 4WCU (Figure 3). There were six hydrogen bonds formed in this interaction. The following amino acid residues were involved: HIS 160, ASN 209, THR 271, GLU 230, and HIS 204. π-S interaction was observed the S-atom of 4g and HIS 204. The details are shown in Figure 3. These interactions were compared with the interactions seen when indomethacin is bound to the binding sites of 4WCU (Figure 4). HIS 200 and ASP 201 formed H-bonds with indomethacin. Details of these interactions are shown in Figure 4. By utilizing different amino acid residues in their interactions, it is possible that compound 4g and indomethacin may have different mechanisms of reaction in eliciting anti-inflammatory actions.
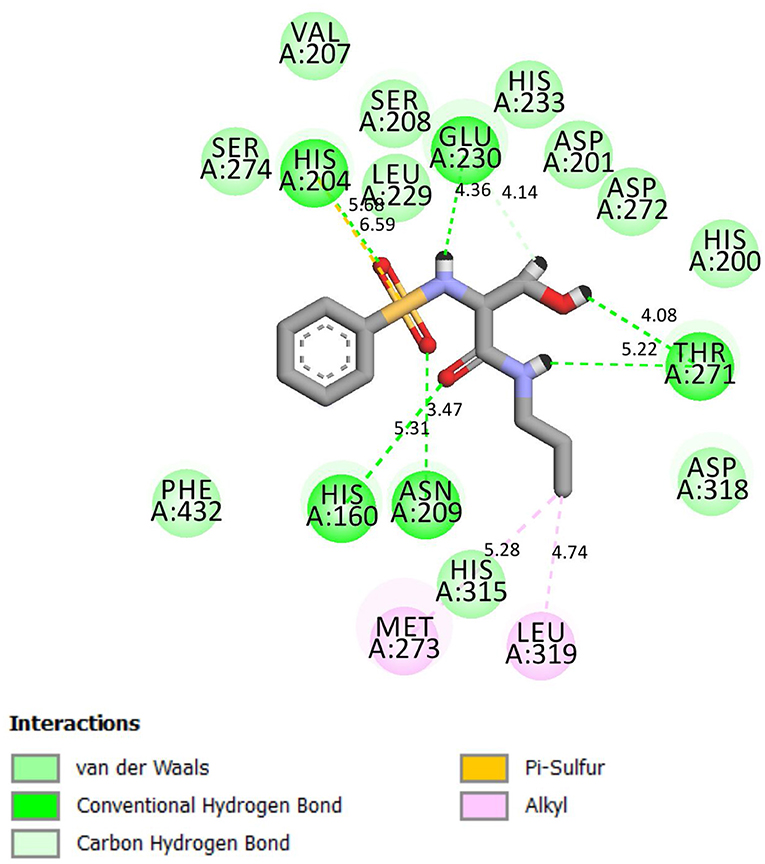
Figure 3. 2D representation of binding interaction of compound 4g and the amino acid residues of 4WCU.
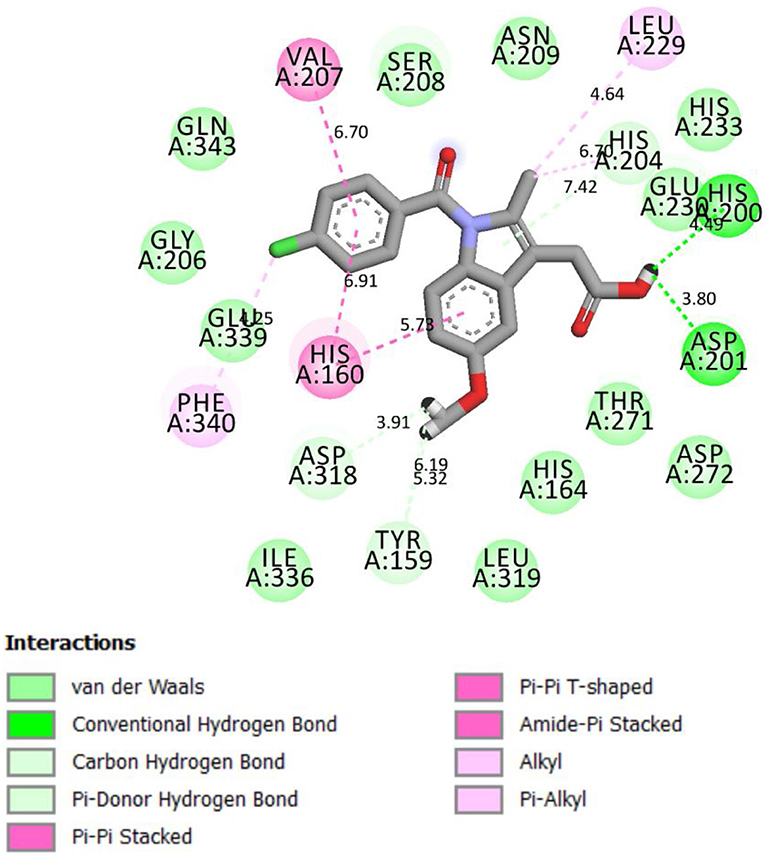
Figure 4. 2D representation of binding interaction of indomethacin and the amino acid residues of 4WCU.
Biological Studies
In vivo Anti-inflammatory Activities
The in vivo anti-inflammatory activity showed that all the novel compounds (Table 2) tested had a fascinating inhibition of inflammation (94.69–89.38%) when compared with NSAID indomethacin (78.76%) at 1 h. The most active were compounds 4a and 4c with percentage inhibitions of 94.69% each at 1 h. The compounds showed better anti-inflammatory activities at 1 h of the experiment. It was observed that the anti-inflammatory activities decreased with increase in time.
In vitro Antimicrobial Activities
General sensitivity of compounds against microorganism
Minimum inhibitory concentration (mg/mL). The antimicrobial studies revealed that most of the novel compounds (MIC 8.90(−6.28 mg/mL) were more potent than the reference drugs (MIC 9.65(−8.39 mg/mL) against the tested microorganisms (Tables 3, 4). Compound 4d (MIC 6.72 mg/mL) was the most active against E. coli. Compound 4h (MIC 6.63 mg/mL) was the most potent against S. aureus. Compound 4a (MIC 6.67 and 6.45 mg/mL) was the most active against P. aeruginosa and S. typhi, respectively. Compound 4f (MIC 6.63 mg/mL) was the most potent against B. subtilis. Compounds 4e and 4h (MIC 6.63 mg/mL) each were the most active against C. albicans, while compound 4e (MIC 6.28 mg/mL) was the most potent against A. niger.
The structure activity relationship study revealed that the p-toluenesulphonamide derivatives possessed better antimicrobial properties than the benzenesulphonamide analogs. This finding underscores the importance of the methyl group at position 4 of the phenyl ring mimicking the structure of p-amino benzoic acid which is needed for the synthesis of folic acid by the microorganism.
In vitro Antioxidant Activities
In vitro antioxidant activities (% scavenging activity)
In vitro antioxidant activities (IC50). The in vivo antioxidant activities (Tables 5, 6) revealed that all the novel compounds had antioxidant activities, though lower than Vitamin C. Only compound 4e (IC50 0.3287 mg/mL) had comparable activity with Vitamin C (IC50 0.2090 mg/mL) at 0.25 mg/mL.
DPPH assay is used to predict antioxidant activities by mechanism in which the compounds tested inhibit lipid oxidation, so scavenging of DPPH radical and therefore can determine free radical scavenging capacity. The method is used widely due to relatively short time required for the analysis. DPPH free radical is very stable and reacts with compounds that can donate hydrogen atoms. The assay measures the reducing ability of antioxidants toward the DPPH radical.
Physicochemical properties. Table 7 shows the physicochemical properties of the synthesized compounds which are useful in the assessment of the drug-likeness.
Lipinski's rule of five helps to evaluate the bioavailability for oral formulations. An oral drug with a good bioavailability should have MW ≤ 500, HBD ≤ 5, HBA ≤ 10, and Log P(o/w) ≤ 5. A violation of more than one parameter may be an indication of poor bioavailability. Table 7 shows that the synthesized compounds are in agreement with the Lipinski's rule of five. In addition, the TPSA, which is a reflection of the compound's hydrophilicity, is very important in protein-ligand interactions. NRB ≤ 10 and TPSA ≤ 140 Å2 would have a high probability of good oral bioavailability in rats. The compounds reported in this research possessed TPSA < 140 and NRB < 10 and as such would not pose oral bioavailability problems if formulated.
Conclusions
In this paper, we have described an efficient, ecofriendly, and versatile approach to obtain substituted benzenesulphonamides bearing carboxamide. All the compounds were evaluated for their anti-inflammatory, antimicrobial and oxidant activities. Compounds 4a and 4c were the most active anti-inflammatory agents, compound 4d was the most active against E. coli, compound 4h was most active against S. aureus, compound 4a was most active against P. aeruginosa and S. typhi, compound 4f was the most active against B. subtilis, compounds 4e and 4h was the most active against C. albicans, compound 4e was most active against A. niger. Compound 4e had comparable activity to Vitamin C. The derivatives are promising drug candidates which can take care of inflammation and oxidative stress that occurs during microbial invasion while acting as antimicrobial agents.
Data Availability
All datasets generated for this study are included in the manuscript/Supplementary Files.
Ethics Statement
The animal study was reviewed and approved by Ethics committee on animal use of the University of Nigeria.
Author Contributions
All authors listed have made a substantial, direct and intellectual contribution to the work, and approved it for publication.
Conflict of Interest Statement
The authors declare that the research was conducted in the absence of any commercial or financial relationships that could be construed as a potential conflict of interest.
Supplementary Material
The Supplementary Material for this article can be found online at: https://www.frontiersin.org/articles/10.3389/fchem.2019.00634/full#supplementary-material
References
Abdel-Aziz, H. A., Al-Rashood, K. A., and ElTahir, K. E. H. (2014). Synthesis of N-benzenesulphonamid-1H-pyrazoles bearing arylsulphonyl moiety: novel celecoxib analogs as potent anti-inflammatory agents. Eur. J. Med. 80, 416–422. doi: 10.1016/j.ejmech.2014.04.065
Adeniyi, B. A., and Odedola, H. A. (1996). Antimicrobial potentials of DiospyrosMespiliforrmis (Ebenaceae). Afr. J. Med. Sci. 255, 211–224.
Aissaoui, H., Koberstein, R., Zumbrunn, C., Gatfield, J., Brisbare-Roch, C., and Jenck, F. (2008). N-glycine-sulphonamides as potent dual Orexin-1/Orexin-2 receptor antagonists. Bioorg. Med. Chem. Lett. 18:5733. doi: 10.1016/j.bmcl.2008.09.079
Ali, S. A., Qasir, A. J., and Saor, K. Y. (2009). Synthesis of some sulfonamide derivatives with expected antibacterial activity. Iraqi J. Mark. Rec. Cons. Protection 1, 85–93.
Ananthanarayanan, V. S., tetreault, S., and Saint-Jean, A (1993). Interaction of calcium channel antagonists with calcium: spectroscopic and modeling studies on diltiazem and its Ca2+ complex. J. Med. Chem. 36:1332. doi: 10.1021/jm00062a004
Bhat, M. A., Siddiqui, N., and Khan, S. A. (2006). Synthesis of novel sulphonamides as potential antibacterial, antifungal and antimalarial agent. Indian. J. Chem. 54B:134. doi: 10.4103/0250-474X.22984
Bylund, J., Burges, L. A., Cescutti, P., Ernst, R. K., and Speert, D. P. (2006). Exopolysaccharides from BurkholderiaCenocepacia inhibit neutrophil chemotaxis and scavenge reactive oxygen species. J. Biol. Chem. 281, 2526–2532. doi: 10.1074/jbc.M510692200
Chandak, H. S. (2012). Synthesis of isoxazolylbenzenesulphonamide derived from N-[4-(2,3-dibromo-2-arylpropanoyl)phenyl]benzenesulphonamide. Der. Pharma. Chem. 4, 1054–1057.
Christopher, W. (2000). Molecular mechanisms that confer antibacterial drug resistance. Nature 406,775–781. doi: 10.1038/35021219
Circu, M. L., and Aw, T. Y. (2010). Reactive oxygen species, cellular redox system and apoptosis. Free Radic. Biol. Med. 48, 749–762. doi: 10.1016/j.freeradbiomed.2009.12.022
Declercq, J. P., Evrard, C., Clippe, A., Stricht, D. V., Bernard, A., and Knoops, B. (2001). Crystal structure of human peroxiredoxin 5, a novel type of mammalian peroxiredoxin at 1.5 a resolution. J. Mol. Biol. 311:751. doi: 10.1006/jmbi.2001.4853
deGasparo, M., and Whitebread, S. (1995). Binding of valsartan to mammalian angio-tensin ATI receptors. Regul. Pept. 59:311. doi: 10.1016/0167-0115(95)00085-P
Ezeokonkwo, M. A., Ogbonna, O. N., Okafor, S. N., Godwin-Nwakwasi, E. U., Ibeanu, F. N., and Okoro, U. C. (2017). Angular phenozaxine ethers as potent multi-microbial targets inhibitors: design, synthesis, and molecular docking studies. Front. Chem. 5:107. doi: 10.3389/fchem.2017.00107
Festus, C., Anthony, C. E., Ibeji, C. U., Okafor, S. N., Onwudiwe, D. C., Aderoju, A. O., et al. (2018). Synthesis, characterization, antimicrobial activity and DFT studies of 2-(pyrimidin-2-ylamino)naphthalene-1,4-dione and its Mn(II), Co(II), Ni(II), and Zn(II) complexes. J. Mol. Struct. 1163, 455–464. doi: 10.1016/j.molstruc.2018.03.025
Ghorab, M. M., Bashandy, M. S., and Alsaid, M. S. (2014). Novel thiophene derivatives with sulphonamides, isoxazole, benzothiazole, quinoline and anthracene moieties as potential anticancer agents. Acta Pharm. 64, 419–431. doi: 10.2478/acph-2014-0035
Graul, A., and Castaner, J. (1997). Atovarstatin calcium. Drugs Future 22:968. doi: 10.1358/dof.1997.022.09.423212
Hayley, W., and Paul, W. (2010). Understanding antibiotic resistance. Pharm. J. 274:501. Available online at: https://www.pharmaceutical-journal.com/download
Hogan, B. L., Williams, M., Idiculla, A., Veysoglu, T., and Parente, E. (2000). Development and validation of a liquid chromatographic method for the determination of the related substances of ramipril in Altace capsules. J. Pharm. Biomed. Anal. 23:651. doi: 10.1016/S0731-7085(00)00342-3
Hosseinzadeha, N., Serajb, S., Bakhshi-dezffolia, M. E., Hasanic, M., khoshneviszadeha, M., Fallah-Bonekohald, S., et al. (2013). Synthesis and antidiabetic evaluation of benzenesulfonamide derivatives. Iran. J. Pharm. Res. 12, 325–330. Available online at: http://www.ijpr.ir
Islinger, M., Grille, S., Fahimi, H. D., and Schrader, M. (2012). The peroxisome: an update onmysteries. Histochem. Cell Biol. 137, 547–574. doi: 10.1007/s00418-012-0941-4
Jainswal, M., Khadikar, P. V., and Supran, C. T. (2004). Topological modeling of lipophilicity diuretic activity and carbonic inhibition activity of benzenesulphonamides: a molecular connectivity approach. Bioorg. Med. Chem. Lett. 14:5666. doi: 10.1016/j.bmcl.2004.08.051
Knoops, B., Goemaere, J., Van der Eecken, V., and Declercq, J. P. (2011). Peroxiredoxin 5:structure, mechanism, and function of the mammalian atypical 2-Cys peroxiredoxin. Antioxid. Redox. Signal 15, 817–829. doi: 10.1089/ars.2010.3584
Liyana-Pathiranan, C. M., and Shahidi, F. (2005). Antibacterial and antioxidant activities of AdiantumPedatium L. J. Phytol. 3, 26–32. doi: 10.1021/jf049320i
Mahtab, R., Srivastava, A., Gupta, N., Kushwaha, S. K., and Tripathi, A. (2014). Synthesis of novel 2-benzylbenzo[d]thiazole-6-sulfonamide derivatives as potential anti-inflammatory agents. J. Chem. Pharm. Sci. 7, 34–38.
Montalbetti, C. A. G. N., and Falque, V. (2005). Amide bond formation and peptide coupling. Tetrahedron 61:10852. doi: 10.1016/j.tet.2005.08.031
Nakayama, T., Sakamoto, S., Sassa, S., Suzuki, S., Kudo, H., and Nagasawa, H. (2005). Paradoxical effect of cytosine arabinoside on mouse leukemia cell line L1210 cells. Anticancer Res. 25:157–160. Available online at: https://pdfs.semanticscholar.org/1be2/8466368a6addffb786142a8c6d7884fda1cc.pdf
Papadopoulou, V. M., Bloomer, D. W., Rosenzweig, S. H., Chatelain, E., Kaiser, M., Wilkinson, R. S., et al. (2012). Novel-3-nitro-1-H-1,2,4-triazole-based amides and sulphonamides as potential antitrypanosomal agents. J. Med. Chem. 55, 5554–5565. doi: 10.1021/jm300508n
Patchett, A. A. (1993). Excursions in drug discovery. J. Med. 36:2051–2058. doi: 10.1021/jm00067a001
Prestinaci, F., Pezzotti, P., and Pantosti, A. (2015). Antimicrobial resistance: a global multifaceted phenomenon. Pathog. Glob. Health 109, 309–318. doi: 10.1179/2047773215Y.0000000030
Roskoski, R. Jr. (2003). Sti-571: an anticancer protein-tyrosine kinase inhibitor. Biochem. Biophys. Res. Commu. 309:717. doi: 10.1016/j.bbrc.2003.08.055
Selvam, P., Murugesh, N., Chandramohan, M., Debyser, Z., and Witvrouw, M. (2008). Design, synthesis and anti-HIV activity of novel isatin-sulphonamides. Indian J. Pharm. Sci. 70, 779–782. doi: 10.4103/0250-474X.49121
Shet, P. M., Vaidya, V. P., Mahadevan, K. M., Shivananda, M. K., Sreenivasa, S., and Vijaya-Kumar, G. R. (2013). Synthesis, characterization and antimicrobial studies of novel sulphonamides containing substituted naphthofuroyl group. Res. J. Chem. Sci. 3, 15–20. Available online at: www.isca.in
Siddique, M., Saeed, A. B., Ahmad, S., and Dogar, N. A. (2013). Synthesis and biological evaluation of hydrazide based sulphonamides. J. Scient. Innovat. Res. 2, 628–634. Available online at: http://www.jsirjounal.com
Spooner, R., and Yilmaz, O. (2011). The role of Reactive-Oxygen-Specie in microbial persistence and inflammation. Int. J. Mol. Sci. 12, 334–352 doi: 10.3390/ijms12010334
Supuran, C. T. (2008). Carbonic anhydrase: novel therapeutic applications for inhibitors and activators. Nat. Rev. Drug Disc. 7:168. doi: 10.1038/nrd2467
Tang, G., Lin, X., Qiu, Z., Li, W., Zhu, L., Wang, L., et al. (2011). Design and synthesis of benzenesulfonamide derivatives as potent anti-influenza hemagglutinin inhibitors. ACS Med. Chem. Lett. 2, 603–607. doi: 10.1021/ml2000627
Ugwu, D. I., Ezema, B. E., Eze, F. U., and Ugwuja, D. I. (2014). Synthesis and structural activity relationship study of antitubercular carboxamides. Int. J. Med. Chem. 2014, 1–18. doi: 10.1155/2014/614808
Ugwu, D. I., Okoro, U. C., and Mishra, N. K. (2018a). Synthesis, characterization and anthelmintic activity evaluation of pyrimidine derivatives bearing carboxamide and sulphonamide moieties. J. Serbian Chem. Soc. 83, 401–409. doi: 10.2298/JSC170127109U
Ugwu, D. I., Okoro, U. C., and Mishra, N. K. (2018b). Synthesis, characterization and in vivo antitrypanosomal activities of new carboxamides bearing quinoline moiety. PLoS ONE 13:e0191234. doi: 10.1371/journal.pone.0191234
Ugwu, D. I., Okoro, U. C., Ukoha, P. O., Okafor, S., Ibezim, A., and Kumar, N. M. (2017). Synthesis, characterization, molecular docking and in vivo antimalarial properties of new carboxamides bearing sulphonamide. Eur. J. Med. Chem. 135, 349–369. doi: 10.1016/j.ejmech.2017.04.029
Keywords: benzenesulphonamide, carboxamide, antioxidant, anti-inflammatory, antimicrobial
Citation: Eze FU, Okoro UC, Ugwu DI and Okafor SN (2019) Biological Activity Evaluation of Some New Benzenesulphonamide Derivatives. Front. Chem. 7:634. doi: 10.3389/fchem.2019.00634
Received: 13 July 2019; Accepted: 02 September 2019;
Published: 18 September 2019.
Edited by:
Simone Brogi, Department of Pharmacy, University of Pisa, ItalyReviewed by:
Ahmed A. Al-Amiery, National University of Malaysia, MalaysiaMustafa Özyürek, Istanbul University-Cerrahpasa, Turkey
Copyright © 2019 Eze, Okoro, Ugwu and Okafor. This is an open-access article distributed under the terms of the Creative Commons Attribution License (CC BY). The use, distribution or reproduction in other forums is permitted, provided the original author(s) and the copyright owner(s) are credited and that the original publication in this journal is cited, in accordance with accepted academic practice. No use, distribution or reproduction is permitted which does not comply with these terms.
*Correspondence: Florence Uchenna Eze, ZmxvcmVuY2UuYWxpJiN4MDAwNDA7dW5uLmVkdS5uZw==