- School of Pharmacy, Hyogo University of Health Sciences, Kobe, Japan
The most significant feature of heterogeneous semiconductor photocatalysis is that both oxidation and reduction occur in a one-pot process. Thus, photocatalysis leads to unique redox organic reactions that cannot be achieved by conventional techniques using oxidants or reductants. Semiconductor photocatalysis is expected to be a new method for fine chemical syntheses of highly valuable molecules such as chiral medicines. However, the use of semiconductor photocatalysts in stereoselective reactions has been limited so far. This mini-review highlights recent progress in stereoselective organic reactions using semiconductor photocatalysts, briefly summarizing the enantio- and diastereoselective reactions based on the currently available literature.
Introduction
Chirality is a fundamentally important topic in science because biologically active species such as enzymes selectively recognize a single enantiomer. Therefore, asymmetric synthesis has attracted extensive attention not only in organic chemistry but also in the medicinal, pharmaceutical and agricultural sciences. Up to now, highly stereoselective synthesis of chiral compounds has been made on a plant scale by conventional stereo-controlling methods (Crawley and Trost, 2012).
Heterogeneous photocatalysis on semiconductors is a unique redox methodology compared to traditional techniques using oxidants or reductants. Such photocatalysis, especially using titanium dioxide (TiO2), has attracted much attention in many fields (Fujishima et al., 2000). Despite significant advances, little attention has been focused on the use of semiconductors for synthetic organic chemistry (Fox, 1987; Fagnoni et al., 2007; Shiraishi and Hirai, 2008; Kohtani and Miyabe, 2014; Lang et al., 2014a,b; Kisch, 2017; Kou et al., 2017; Ma et al., 2018). Semiconductor photocatalysis has several great advantages (Kohtani et al., 2012): (1) It leads to unique one-pot redox transformations. (2) Particular reductants or oxidants are not necessary. (3) It avoids the use of dangerous and harmful reagents. (4) It proceeds under mild conditions (normal temperature and pressure). (5) Semiconductors such as TiO2 are chemically stable, easily separable, and reusable. Thus, such photocatalysis shows great promise to become “green” chemical processes. Moreover, semiconductor photocatalysis is expected to grow as a new synthetic method for preparing highly valuable molecules such as chiral medicines.
Enantioselective synthesis using homogeneous photocatalysts such as chiral metal complexes has attracted wide attention in recent years (Amador and Yoon, 2016; Megan et al., 2016). However, the use of semiconductors in asymmetric synthesis has been limited so far. To our knowledge, ~10 reports have been published on successful examples of enantio- and diastereoselective reactions using semiconductor photocatalysts. This mini-review highlights the progress in stereoselective chemical transformations on photoirradiated surfaces of semiconductor particles, briefly summarizing representative examples of enantio- and diastereoselective reactions based on the currently available literature.
Enantioselective Reactions
In 1990, Wang et al. (1990) reported that the enantioselective photoreduction of 3-methyl-2-oxobutanoic acid 1 proceeded in aqueous methanol suspension containing platinum loaded TiO2 (Pt/TiO2) and chiral 2,2'-bis(diphenylphosphino)-1,1'-binaphthyl (BINAP)-Rh complex to give 2-hydroxy-3-methylbutanoic acid 2 in 75% yield and 60% ee (Figure 1A). The mechanism involved in this asymmetric induction is still unclear. In this transformation, the photo-generated conduction band (CB) electrons must migrate toward Pt on TiO2. A route involving the transfer of electrons accumulated on Pt to the chiral BINAP-Rh complex and the subsequent reduction of 1 by the BINAP-Rh anion is proposed as a possible mechanism. Chiral metal catalysts possessing BINAP ligands are known to be efficient catalysts for the enantioselective hydrogenation of various olefinic and ketonic substrates in the presence of gaseous hydrogen (H2) in the dark (Shimizu et al., 2007). Accumulated electrons on Pt will reduce protons (H+) to produce H2. Therefore, it is possible that substrate 1 is reduced by gaseous H2 and the chiral BINAP-Rh complex as an alternate mechanism.
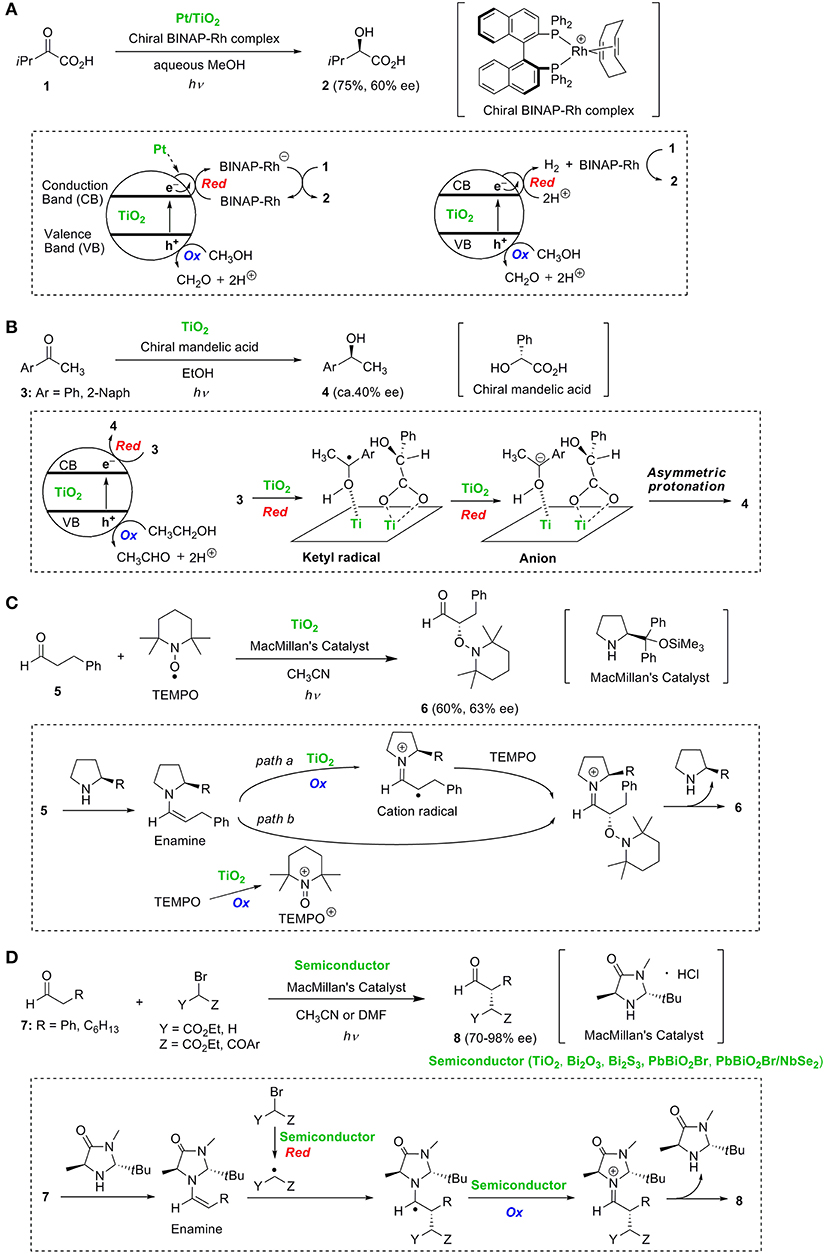
Figure 1. Enantioselective reactions: (A) hydrogenation of α-keto acid 1, (B) hydrogenation of aromatic ketones 3, (C) α-oxyamination of aldehyde 5, and (D) α-alkylation of aldehydes 7.
Kohtani et al. (2018) reported the novel surface-selective and enantioselective hydrogenation of aromatic ketones 3 induced by chiral α-hydroxy acids-coadsorbed on TiO2. When (R)-mandelic acid was used as the chiral reagent, the S-enantiomers of secondary alcohols 4 were predominantly obtained with reasonable enantioselectivities (ca. 40% ee) (Figure 1B). The enantioselectivities were strongly affected by the chiral reagents. Chiral mandelic acids having hydroxyl, phenyl and carboxy groups at the chiral carbon atom showed the best enantioselective stereocontrol. Interestingly, P25 (anatase/rutile = ca. 9/1) and an anatase TiO2 sample (JRC-TIO-13) exhibited relatively high enantioselectivities, whereas another anatase TiO2 (JRC-TIO-7) and the rutile TiO2 led to low % ee. Thus, the enantioselectivity was affected by the TiO2 crystalline samples. The reduction of ketones 3 on TiO2 proceeds via electron transfer to 3 leading to a ketyl radical species and further electron transfer to form an anion (Kohtani et al., 2014). Asymmetric induction is achieved through stereoselective protonation of the anion species by (R)-mandelic acid co-adsorbed on the TiO2 surface.
Jang's group reported TiO2-induced enantioselective α-oxyamination of aldehyde 5 with 2,2,6,6-tetramethylpiperidine-N-oxyl (TEMPO) by the use of a chiral amine catalyst (Figure 1C) (Ho et al., 2011). This reaction proceeds via a chiral enamine intermediate, generated from aldehyde 5 and MacMillan's catalyst, to give oxyamination product 6 in 60% yield with 63% ee. Two pathways are proposed for the oxidative transformation of the enamine to the iminium cation intermediate. The first pathway involves the oxidation of the enamine to a cation radical followed by stereoselective trapping of the cation radical by TEMPO (path a in Figure 1C). The second pathway involves the stereoselective reaction of the enamine with a cation species (TEMPO+) generated by the oxidation of TEMPO (path b in Figure 1C). The successful application to a tandem Michael addition-oxyamination was reported using N719 dye-sensitized TiO2 photocatalyst under visible light irradiation (Yoon et al., 2012).
Cherevatskaya et al. (2012) reported visible-light promoted enantioselective alkylation of aldehydes 7 by the use of several semiconductors and MacMillan's catalyst (Figure 1D). Later, Riente et al. (2014) achieved highly enantioselective α-alkylation of 7 using bismuth-based semiconductor materials (Bi2O3 and Bi2S3) possessing a small band gap and MacMillan's catalyst under sunlight (Figure 1D). Li et al. (2015) studied enantioselective alkylation of aldehydes using a nanocomposite material of PbBiO2Br nanoparticles with a NbSe2 nanosheet. The key step in these reactions is presumed to be the stereoselective addition of alkyl radicals to the chiral enamine intermediates.
Shi et al. (2014) developed an enantioselective molecular imprinting technique for photoelectrochemical and photocatalytic recognition of enantiomers. They reported the chiral recognition and enantioselective decomposition of amino acids on chiral molecular-imprinted ZnO and anatase TiO2 crystallites. Interestingly, the use of anatase TiO2 crystallites with specifically exposed (001) facets led to higher enantioselective recognition, presumably caused by abundant surface hydroxyls on the (001) facet (Shi et al., 2014).
Diastereoselective Reactions and Asymmetric Synthesis
Marinković and Hoffmann reported the radical addition of tertiary amines to α,β-unsaturated lactones using semiconductor photocatalyst powders of TiO2 and ZnS (Marinković and Hoffmann, 2001, 2003). They further developed the diastereoselective radical tandem addition-cyclization reaction of (5R)-menthyloxyfuran-2(5H)-one 9 with aromatic tertiary amines using TiO2 or ZnS (Figure 2A) (Marinković and Hoffmann, 2004). Two stereoisomeric tetrahydroquinoline derivatives 10 and 11 were obtained with reasonable diastereoselectivities. The key radical was initially generated via the single electron oxidation of N, N-dimethylaniline. The stereoselective addition of the alkyl radical to lactone 9 followed by intramolecular radical trapping on the aromatic ring led to the cyclized adduct 10 as the major product.
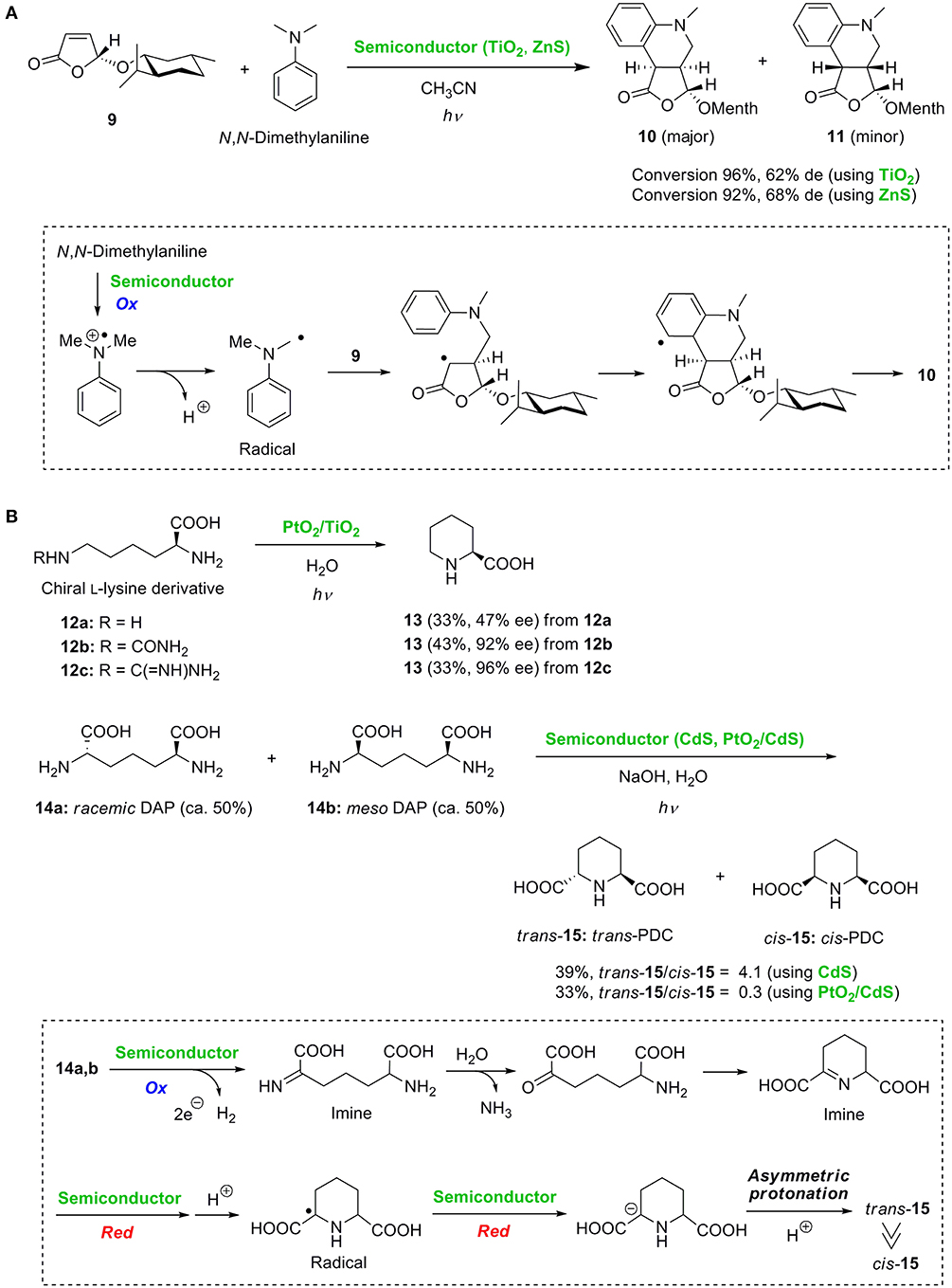
Figure 2. (A) Diastereoselective tandem radical addition-cyclization reaction and (B) asymmetric synthesis of piperidine-2-carboxylic acid and diastereoselective cyclization.
Ohtani et al. (1990, 2001) reported the deaminocyclization of chiral L-lysine derivatives 12a-c and 2,6-diaminopimelic acids (DAP) 14a and 14b to piperidine derivatives 13 and 15, respectively (Figure 2B). In the presence of PtO2/TiO2, the deaminocyclization of L-lysine 12a gave the piperidine-2-carboxylic acid 13 in moderate enantioselectivity, probably due to the competitive oxidation of the two amino groups of 12a. Excellent enantioselectivities were achieved by protection of the α-amino group. When the protected L-lysine derivatives 12b and 12c were employed, piperidine-2-carboxylic acid 13 was synthesized in 92 and 96% ee, respectively. Additionally, by deaminocyclization of a 1:1 mixture of racemic DAP 14a and meso DAP 14b, preferential production of trans-PDC 15 to cis-PDC 15 was achieved by changing the catalyst from CdS to PtO2/CdS (Figure 2B). The diastereoselectivity was determined at the final photocatalytic hydrogenation of the cyclic imine intermediate formed by oxidative deamination and cyclization. cis-15 would be obtained when the hydrogenation proceeds via syn-addition of hydrogen atoms to the C = N bond of the cyclic imine. In contrast, trans-15 is selectively produced when reduction takes place via stepwise electron transfer and subsequent stereoselective protonation as illustrated in Figure 2B. Consequently, the opposite diastereoselective preparation of trans-PDC and cis-PDC was achieved by simply changing the catalyst.
Photobiocatalysis for Asymmetric Synthesis
Photobiocatalysis employing isolated enzymes or lysates involves three types of electron relay systems (Gulder and Seel, 2019): (1) photosensitizers (metal complexes or semiconductors, etc.), (2) reaction sites (enzymes), and (3) electron mediators such as methylviologen, nicotinamide adenine dinucleotide (phosphate) (NAD(P)+/NAD(P)H), and flavin mononucleotide (FMN/FMNH2). Excited electrons generated at the photosensitizer are relayed to the reaction site via the electron mediators. In addition, sacrificial electron donors (e.g., tertiary amines or water) are required to prevent oxidative self-degradation of the photosensitizers. Successful examples of highly enantioselective reactions using semiconductor photocatalysts have been reported. The asymmetric reduction of alkenes using old yellow enzymes in cooperation with CdSe quantum dots (Burai et al., 2012), gold nanoparticle- loaded TiO2 (Au/TiO2), or vanadium doped TiO2 (Mifsud et al., 2014) has been investigated. Moreover, stereoselective activation of C-H bonds during peroxygenase-catalyzed hydroxylation of alkylbenzenes and alkanes has been achieved using Au/TiO2 (Zhang et al., 2017, 2018).
Conclusion and Outlook
This mini-review focuses on the enantio- and diastereoselective organic reactions occurring in several semiconductor photocatalyses. As mentioned in this review, our group found that enantioselective hydrogenation on TiO2 was strongly affected by the surface structure of TiO2 (Kohtani et al., 2018). Recently, it was also demonstrated that adsorption of chiral molecules on a specific semiconductor nanoparticle surface (mercury sulfide: HgS) was associated with the growth of chiral semiconductor nanoparticles (Kuno et al., 2018). Thus, one promising strategy may be the use of highly uniform semiconductor nanocrystals with specific exposure of the reactive facets. If these facets could be selectively covered with stable chiral compounds, enantioselective reactions would be greatly enhanced. Therefore, increasing attention should be given to the development of specifically reactive facets on semiconductor materials for stereoselective organic transformations.
Author Contributions
All authors listed have made a substantial, direct and intellectual contribution to the work, and approved it for publication.
Funding
This work was supported in part by JSPS KAKENHI Grant-in-Aid for Scientific Research (C) Grant Numbers 16K08188 (to HM) and 19K05681 (to SK).
Conflict of Interest Statement
The authors declare that the research was conducted in the absence of any commercial or financial relationships that could be construed as a potential conflict of interest.
References
Amador, A. G., and Yoon, T. P. (2016). A chiral metal photocatalyst architecture for highly enantioselective photoreactions. Angew. Chem. Int. Ed. 55, 2304–2306. doi: 10.1002/anie.201511443
Burai, T. N., Panay, A. J., Zhu, H., Lian, T., and Lutz, S. (2012). Light-driven quantum dot-mediated regeneration of FMN to drive reduction of ketoisophorone by old yellow enzyme. ACS Catal. 2, 667–670. doi: 10.1021/cs300085h
Cherevatskaya, M., Neumann, M., Füldner, S., Harlander, C., Kümmel, S., Dankesreiter, S., et al. (2012). Visible-light-promoted stereoselective alkylation by combining heterogeneous photocatalysis with organocatalysis. Angew. Chem Int. Ed. 51, 4062–4066. doi: 10.1002/anie.201108721
Crawley, M. L., and Trost, B. M. (2012). Applications of Transition Metal Catalysis in Drug Discovery and Development: An Industrial Perspective. Hoboken, NJ: John Wiley & Sons, Inc.
Fagnoni, M., Dondi, D., Ravelli, D., and Albini, A. (2007). Photocatalysis for the formation of the C-C bond. Chem. Rev. 106, 2725–2756. doi: 10.1021/cr068352x
Fox, M. A. (1987). Selective formation of organic compounds by photoelectrosynthesis at semiconductor particles. Top. Curr. Chem. 142, 71–99.
Fujishima, A., Rao, T. N., and Tryk, D. A. (2000). Tianium dioxide photocatalysis. J. Photochem. Photobiol. C Photochem. Rev. 1, 1–21. doi: 10.1016/S1389-5567(00)00002-2
Gulder, T., and Seel, C. J. (2019). Biocatalysis fueled by light: on the versatile combination of photocatalysis and enzymes. ChemBioChem 20, 1871–1897. doi: 10.1002/cbic.201800806
Ho, X.-H., Kang, M.-J., Kim, S.-J., Park, E., and Jang, H.-Y. (2011). Green organophotocatalysis. TiO2-induced enantioselective α-oxyamination of aldehydes. Catal. Sci. Tech. 6, 923-926. doi: 10.1039/c1cy00183c
Kisch, H. (2017). Semiconductor photocatalysis for chemoselective radical coupling reactions. Acc. Chem. Res. 50, 1002–1010. doi: 10.1021/acs.accounts.7b00023
Kohtani, S., Kamoi, Y., Yoshioka, E., and Miyabe, H. (2014). Kinetic study on photocatalytic hydrogenation of acetophenone derivatives on titanium dioxide. Catal. Sci. Tech. 4, 1084–1091. doi: 10.1039/c3cy00879g
Kohtani, S., Kawashima, A., Masuda, F., Sumi, M., Kitagawa, Y., Yoshioka, E., et al. (2018). Chiral α-hydroxy acid-coadsorbed TiO2 photocatalysts for asymmetric induction in hydrogenation of aromatic ketones. Chem. Commun. 54, 12610–12613. doi: 10.1039/c8cc07295g
Kohtani, S., and Miyabe, H. (2014). “Titanium dioxide-induced photocatalytic reduction for organic synthesis” in Titanium Dioxide: Chemical Properties, Applications and Environmental Effects, ed J. Brown (New York, NY: Nova Science Publishers Inc., 157–176.
Kohtani, S., Yoshioka, E., and Miyabe, H. (2012). “Photocatalytic hydrogenation on semiconductor particles” in Hydrogenation, eds I. Karamé and C. Rijeka (Oxford: Intech), 291–308. doi: 10.5772/45732
Kou, J., Lu, C., Wang, J., Chen, Y., Xu, Z., and Varma, R. S. (2017). Selectivity enhancement in heterogeneous photocatalytic transformations. Chem. Rev. 117, 1445–1514. doi: 10.1021/acs.chemrev.6b00396
Kuno, J., Imamura, Y., Katouda, M., Tashiro, M., Kawai, T., and Nakashima, T. (2018). Inversion of optical activity in the synthesis of mercury sulfide nanoparticles: role of ligand coordination. Angew. Chem. Int. Ed. 57, 12022–12026. doi: 10.1002/anie.201807191
Lang, X., Chen, X., and Zhao, J. (2014a). Heterogeneous visible light photocatalysis for selective organic transformations. Chem. Soc. Rev. 43, 473–486. doi: 10.1039/c3cs60188a
Lang, X., Ma, W., Chen, C., Ji, H., and Zhao, J. (2014b). Selective aerobic oxidation mediated by TiO2 photocatalysis. Acc. Chem. Res. 47, 355–363. doi: 10.1021/ar4001108
Li, X., Wang, J., Xu, D., Sun, Z., Zhao, Q., Peng, W., et al. (2015). NbSe2 nanosheet supported PbBiO2Br as a high performance photocatalyst for the visible light-driven asymmetric alkylation of aldehyde. ACS Sustainable Chem. Eng. 3, 1017–1022. doi: 10.1021/acssuschemeng.5b00182
Ma, D., Liu, A., Li, S., Lu, C., and Chen, C. (2018). TiO2 photocatalysis for C–C bond formation. Catal. Sci. Tech. 8, 2030–2045. doi: 10.1039/C7CY01458A
Marinković, S., and Hoffmann, N. (2001). Efficient radical addition of tertiary amines to electron-deficient alkenes using semiconductors as photochemical sensitisers. Chem. Commun. 1576–1578. doi: 10.1039/b104387k
Marinković, S., and Hoffmann, N. (2003). Semiconductors as sensitisers for the radical addition of tertiary amines to electron deficient alkenes. Int. J. Photoenergy 5, 175–182. doi: 10.1155/S1110662X03000308
Marinković, S., and Hoffmann, N. (2004). Diastereoselective radical tandem addition-cyclization reactions of aromatic tertiary amines by semiconductor-sensitized photochemical electron transfer. Eur. J. Org. Chem. 2004, 3102–3107. doi: 10.1002/ejoc.200400102
Megan, H. S., Twilton, J., and MacMillan, D. W. C. (2016). Photoredox catalysis in organic chemistry. J. Org. Chem. 81, 6898–6926. doi: 10.1021/acs.joc.6b01449
Mifsud, M., Gargiulo, S., Iborra, S., Arends, I. W. C. E., Hollmann, F., and Corma, A. (2014). Photobiocatalytic chemistry of oxidoreductases using water as the electron donor. Nat. Commun. 5, 3145–3150. doi: 10.1038/ncomms4145
Ohtani, B., Kusakabe, S., Okada, K., Tsuru, S., Nishimoto, S., Amino, Y., et al. (2001). Photocatalytic stereoselective N-cyclization of 2,6-diaminopimelic acid into piperidine-2,6-dicarboxylic acid by an aqueous suspension of activated cadmium(II) sulfide particles. J. Chem. Soc. Perkin Trans. 2, 201–209. doi: 10.1039/b0069671
Ohtani, B., Tsuru, S., Nishimoto, S., and Kagiya, T. (1990). Photocatalytic one-step syntheses of cyclic imino acids by aqueous semiconductor suspensions. J. Org. Chem. 55, 5551–5553. doi: 10.1021/jo00308a005
Riente, P., Matas Adams, A., Albero, J., Palomares, E., and Pericàs, M. A. (2014). Light-driven organocatalysis using inexpensive, nontoxic Bi2O3 as the photocatalyst. Angew. Chem. Int. Ed. 53, 9613–9616. doi: 10.1002/anie.201405118
Shi, H., Chen, C., Tang, B., and Zhao, G. (2014). Photoelectrochemical enantioselective recognition of amino acid enantiomers on (001) facet TiO2 surface. Electrochim. Acta 146, 359–364. doi: 10.1016/j.electacta2014.08.135
Shimizu, H., Nagasaki, I., Matsumura, K., Sayo, N., and Saito, T. (2007). Developments in asymmetric hydrogenation from an industrial perspective. Acc. Chem. Res. 40, 1385–1393. doi: 10.1021/ar700101x
Shiraishi, Y., and Hirai, T. (2008). Selective organic transformations on titanium oxide-based photocatalysts. J. Photochem. Photobiol. C Photochem. Rev. 1, 1–21. doi: 10.1016/j.jphotochemrev.2008.05.001
Wang, H., Sakata, T., Azuma, M., Ohta, T., and Takaya, H. (1990). Photocatalytic asymmetric reduction of 3-methyl-2-oxobutanoic acid with chiral Rh(I) complexes and powdery semiconductors. Chem. Lett. 19, 1331–1334. doi: 10.1246/cl.1990.1331
Yoon, H.-S., Ho, X.-H., Jang, J., Lee, H.-J., Kim, S.-J., and Jang, H.-Y. (2012). N719 dye-sensitized organophotocatalysis: enantioselective tandem michael addition/oxyamination of aldehydes. Org. Lett. 14, 3272–3275. doi: 10.1021/ol3011858
Zhang, W., Burek, B. O., Fernández-Fueyo, E., Alcalde, M., Bloh, J. Z., and Hollmann, F. (2017). Selective activation of C-H bonds in a cascade process combining photochemistry and biocatalysis. Angew. Chem. Int. Ed. 56, 15451–15455. doi: 10.1002/anie.201708668
Keywords: enantioselective reactions, diastereoselective reactions, semiconductor photocatalysis, heterogeneous photocatalysis, titanium dioxide, chiral reagents
Citation: Kohtani S, Kawashima A and Miyabe H (2019) Stereoselective Organic Reactions in Heterogeneous Semiconductor Photocatalysis. Front. Chem. 7:630. doi: 10.3389/fchem.2019.00630
Received: 15 April 2019; Accepted: 02 September 2019;
Published: 18 September 2019.
Edited by:
Amer Hakki, Gulf Organisation for Research & Development, QatarReviewed by:
Cláudia Gomes Silva, University of Porto, PortugalJonathan Zacharias Bloh, DECHEMA Forschungsinstitut (DFI), Germany
Copyright © 2019 Kohtani, Kawashima and Miyabe. This is an open-access article distributed under the terms of the Creative Commons Attribution License (CC BY). The use, distribution or reproduction in other forums is permitted, provided the original author(s) and the copyright owner(s) are credited and that the original publication in this journal is cited, in accordance with accepted academic practice. No use, distribution or reproduction is permitted which does not comply with these terms.
*Correspondence: Shigeru Kohtani, kohtani@huhs.ac.jp; Hideto Miyabe, miyabe@huhs.ac.jp