- 1Shanghai Key Laboratory of Green Chemistry and Chemical Processes, Shanghai Engineering Research Center of Molecular Therapeutics and New Drug Development, School of Chemistry and Molecular Engineering, East China Normal University, Shanghai, China
- 2NYU-ECNU Center for Computational Chemistry at NYU Shanghai, Shanghai, China
The homogeneous cycloaddition reaction of CO2 and epichlorohydrin catalyzed by amine-functionalized ionic liquid (AFIL) to yield cyclic carbonate is reported in this study. The AFIL has the dual function of ionic liquid and organic base. The experimental study indicates that AFIL can efficiently catalyze the conversion of CO2 and epichlorohydrin to the product 3-chloro-1,2-propylene. The mechanistic study based on DFT calculations reveals that the imidazolium ring in AFIL primarily catalyzes the ring-opening reaction of epichlorohydrin, while the protonated amine group is responsible for stabilizing the Br− anion in the nucleophilic attack. This study provides a deep insight into the catalytic roles of AFIL and also inspires us to design efficient dual function catalysts for CO2 utilization.
Introduction
The concentration of carbon dioxide (CO2) in the atmosphere continues to increase due to the deforestation and burning of fossil fuels, which makes earth's environment worse than before (Hunt et al., 2010; Monastersky, 2013). The rational conversion of CO2 to valuable chemicals has attracted wide attentions all over the world in decades (Rochelle, 2009; Jones, 2011). Since the carbon-oxygen bonds in CO2 are quite stable in thermodynamics, CO2 is too inert to be activated (Aresta et al., 2013; Chong and Kinjo, 2015). One of effective methodologies for CO2 conversion is to use high-energy materials such as epoxides to react with CO2 to yield oxidized low-energy synthetic targets (Sakakura et al., 2007). The epichlorohydrin, as one of significant functional epoxides (Padwa and Murphree, 2005; D′Elia et al., 2015), is the main raw material for the synthesis of epoxy resin and glycerol in chemical industry. Thus, it is desirable to perform the cycloaddition of CO2 with epoxides to give rise to the valuable chemicals such as cyclic carbonates.
Various homogeneous catalysts including metal salts (Ma et al., 2012), organic bases (Yano et al., 1997), and ionic liquids (Sheldon, 2001; Song et al., 2014) have been applied to promote the cycloaddition reactions of CO2 with epoxides. Among these catalysts, ionic liquids have a profound effect on activity and selectivity. Ionic liquid catalysts are deeded as considerable potential materiel in industrial application. Ionic liquids are effective solvents or catalysts for the cycloaddition of CO2 with epoxides on account of its unique characters such as thermal and chemical stability, selective solubility toward organic and inorganic materials, and high recyclable.
Peng reported the reaction of quantitative conversion of propylene oxide (PO) to propylene carbonates (PC) catalyzed by 1-butyl-3-methyl-imidazolium (BMimBF4) ionic liquid (Peng and Deng, 2001). Xiao achieved the chemical conversion of CO2 with epoxides to yield cyclic carbonates under mild conditions by using immobilized ionic liquid catalyst (Xiao et al., 2006). Sun synthesized a series of hydroxyl-functionalized ionic liquids which possess efficient reactivity toward the coupling of epoxide and CO2 (Sun et al., 2008). Wang reported the one-pot conversion of CO2, ethylene oxide (EO) and amines to 3-aryl-2-oxazolidinones, which were catalyzed by the binary ionic liquids composed of 1-butyl-3-methyl-imidazolium bromide (BmimBr) and 1-butyl-3-methyl-imidazolium acetate (Wang et al., 2013). Appayuri groups developed a facile and efficient synthesis of styrene carbonate via cycloaddition of CO2 to styrene oxide over ordered mesoporous MCM-41-Imi/Br catalyst (Appaturi and Adam, 2013). Anthofer et al. (2014, 2015) synthesized hydroxy-functionalized mono- and bis-imidazolium bromides for the cycloaddition traction between CO2 and epoxides and found that the activity of the latter was higher than that of the former. Elageed et al. (2016) developed a selective procedure for the synthesis of 5-substituted N-aryloxazolidinones by mixing the epoxides with arylcarbamates catalyzed by ionic liquids. Arayachukiat et al. (2017) and Yingcharoen et al. (2019) investigated the hydrogen-bonding effect of organic catalysts on the cycloaddition of CO2 to epoxides.
In addition, the previous theoretical studies mainly focused on elucidating the mechanisms of reactions of CO2 with different oxides catalyzed by catalysts. For instance, Sun calculated the reaction pathways of CO2 with the propylene oxide catalyzed by the alkylmethylimidazolium chlorine using the density functional theory (DFT) method (Sun and Zhang, 2007). Whiteoak et al. (2012) performed a DFT calculation on the mechanism of CO2 conversion to carbonates catalyzed by organic catalyst. Girard et al. (2014) calculated the mechanism of CO2 with the styrene oxide catalyzed by BmimBr. Luo et al. (2016) and Huang et al. (2017) performed detailed DFT calculations on the reaction mechanisms of amines, CO2 and EO catalyzed by the binary ionic liquids BmimBr and BmimOAc. Further, Ji et al. (2018) researched an efficient catalyst that was composed of imidazolium ionic liquids and organic bases for catalyzing the cycloaddition of CO2 and epoxides under atmospheric pressure. The mixed system of ionic liquid and organic base exhibits distinctly higher catalytic activity than using single component alone. Their DFT calculation study on the mechanism of synergistic catalysis revealed that the ionic liquids played an important role in the ring-opening reaction of epoxide and the organic bases facilitated the generation of bicarbonate ion.
In this work, we report a new AFIL catalyst whose design is inspired by the idea of combination of ionic liquids and organic bases. When the new catalyst was used to catalyze the cycloaddition reaction of CO2 with epichlorohydrin, it exhibited the synergistic catalysis effect better than the single catalyst of ionic liquid or organic base. The cycloaddition reaction of CO2 with epichlorohydrin catalyzed by AFIL catalyst has a good yield of the product of cyclic carbonate. We also performed a detailed DFT calculation on its mechanism to account for the synergistic catalysis effect of AFIL.
Computational and Experimental Details
Computational Methods
All the DFT calculations throughout this work were carried out in the Gaussian09 software package (Frisch et al., 2009). The geometrical structures of all reactants, intermediates and products were directly optimized in solution phase. In order to obtain accurate calculated results, we employ the method of functional ωB97X-D (Chai and Head-Gordon, 2008) combined with a large basis set 6-31+G* (Rassolov et al., 2001), denoted as ωB97X-D/6-31+G*. The solvent effect is described by the SMD (Dolg et al., 1987; Andrae et al., 1990) model with the value of dielectric constant being 20.8. The frequency analysis is performed at the same computational level to identify the minima or transition states on potential energy surfaces. The calculation conditions for the reaction temperature and pressure were chosen as 353 K and 1 standard atmosphere pressure, which was as same to the experimental conditions. The high temperature was considered to make a significant contribution of TΔS in Gibbs free energy and the units for energies are in kcal/mol. More details about the optimized structures are provided in Supplementary Material.
Experimental Methods
Chemicals and Materials
1-Butylimidazole, N,N-diethylbenzylamine (DEBA) and 1,3-bis(bromomethyl)benzene were purchased from J&K with AR grade. CO2 was supplied by Doumaoai Factory with a purity of 99.9%. Epichlorohydrin, diethylamine (DEA), potassium carbonate (K2CO3), acetonitrile, toluene, ether were obtained from Sinopharm with AR grade. All chemicals were used without further purification.
NMR spectra were recorded on Bruker Ascend 400 instruments with tetramethylsilane as the internal standard. GC analysis was performed by using a Shimadzu GC−14 B equipped with a capillary column DM-1701 (60 m-0.32 mm-0.25 mm) equipped with a flame ionization detector.
Synthesis of Ionic Liquid Catalysts
1-Butylimidazole (0.188 g, 2 mmol) in acetonitrile (50 mL) and 1,3-bis(bromomethyl)benzene (2.64 g, 10 mmol) in toluene (50 mL) were mixed in a 250 mL round bottom flask and stirred at 85°C for 24 h. The precipitate was filtered and washed with Et2O (10 mL × 3) to ensure 1,3-bis(bromomethyl)benzene was removed completely. A white solid (1) was obtained after vacuum dried in the yield of 82%. 1H NMR (400 MHz, DMSO-d6, TMS): δ 9.40 (s, 1H), 7.85 (s, 2H), 7.49–7.35 (m, 4H), 5.46 (s, 2H), 4.70 (s, 2H), 4.20 (t, J = 6.0 Hz, 2H), 1.82–1.75 (m, 2H), 1.28–1.23 (m, 2H), 0.90 (t, J = 6.0 Hz, 2H).
In a solution of 1 (0.776 g, 2 mmol), K2CO3 (1.38 g, 10 mmol) and acetonitrile (100 mL), DEA (1.46 g, 20 mmol) was added dropwise. The mixture was stirred at room temperature for 24 h. The oily precipitate was washed with Et2O (10 mL × 3). AFIL was obtained as a yellow viscous liquid in the yield of 88% after vacuum dried. 1H NMR (400 MHz, DMSO-d6, TMS): δ 9.37 (s, 1H), 7.85 (s, 2H), 7.37–7.26 (m, 4H), 5.43 (s, 2H), 4.20 (t, J = 6.0 Hz, 2H), 3.51 (s, 2H), 2.46–2.40 (m, 4H), 1.81–1.74 (m, 2H), 1.27–1.22 (m, 2H), 0.97–0.88 (m, 9H). The ionic liquid 1-benzyl-3-butylimidazolium bromide (BnBimBr) was synthesized by a reported method (Ji et al., 2018).
Cycloaddition Reaction of CO2 and Epichlorohydrin
Epichlorohydrin (0.925 g, 10 mmol) and AFIL (0.38 g, 0.1 mmol) were put into a 50 mL stainless steel autoclave equipped with a magnetic stirrer. The reaction was carried out under atmospheric pressure of CO2 at 80°C. After the completion of reaction, the autoclave was cooled to room temperature. In order to remove the ionic liquid, Et2O (50 mL) was added to the reaction mixture and filtered. The filtrate was subsequently analyzed by gas chromatography to determine yield using dodecane as internal standard. The product was purified by chromatography on silica gel and characterized structurally by NMR spectroscopy.
3-Chloro-1,2-propylene carbonate: Colorless liquid, 1H NMR (400 MHz, CDCl3, TMS) δ 5.01–4.95(m, 1H), 4.57 (t, J = 8.0 Hz, 1H), 4.39–4.35 (m, 1H), 3.81–3.77 (m, 1H), 3.72–3.68 (m, 1H). 13C NMR (100 MHz, CDCl3, TMS) δ 154.24, 74.33, 66.99, 43.74.
Results and Discussion
Structures of the Ionic Liquid Catalysts
The structures and synthetic procedures of ionic liquid catalysts for the cycloaddition of CO2 and epichlorohydrin were shown in Figure 1.
Ionic Liquids Catalyzed CO2 Cycloaddition Reaction
To investigate the intramolecular synergistic catalytic system of imidazolium and amino functional group in AFIL, BnBimBr, DEBA and AFIL were applied to catalyze the cycloaddition of CO2 and epichlorohydrin. The synergistic catalytic effect of organic base and ionic liquid for the conversion of CO2 was shown in Table 1. When BnBimBr was used alone, a moderate yield of 50% of 3-chloro-1,2-propylenecarbonate was obtained after 3 h under atmospheric CO2 pressure (entry 1, Table 1). If DEBA was used alone, a poor yield of 14% was obtained (entry 2, Table 1). However, the AFIL gave excellent yield of 85% (entry 3, Table 1), indicating the intramolecular synergistic catalytic system of AFIL for accelerating the cycloaddition reaction.
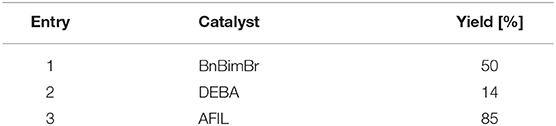
Table 1. The synergistic catalytic effect of organic base and ionic liquid for the cycloaddition of CO2 and epichlorohydrin.
Reaction conditions: Epichlorohydrin (10 mmol), CO2 (0.1 MPa), catalyst (0.1 mmol), 80°C, 3 h.
The optimized experimental conditions such as reaction time, reaction temperature and catalyst amount on the reaction have been systemically investigated. The effect of different reaction times on the product yield was shown in Figure 2. The yield of the 3-chloro-1,2-propylenecarbonate increased with raising reaction time from 1 to 6 h. After 3 h, the growth trend of the yield slowed down obviously. When the reaction time was 6 h, the yield could reach 97%.
Reaction condition: Epichlorohydrin (10 mmol), CO2 (0.1 MPa), AFIL (0.1 mmol), 80°C, GC yield.
The effect of reaction temperatures was listed in Figure 3. The yield increased significantly with the temperature from 50 to 80°C. When the reaction temperature higher than 80°C, the yield barely increased.
Reaction condition: Epichlorohydrin (10 mmol), CO2 (0.1 MPa), AFIL (0.1 mmol), 6 h, GC yield. The effect of catalyst amount on the reaction was also investigated (Figure 4). In the absence of any catalyst, the reaction did not proceed. However, in the presence of the catalyst, the reaction took place immediately. When the catalyst amount increased from 0.1 to 0.5 mol%, the yield increased from 71 to 94%. The highest yield of 3-chloro-1,2-propylenecarbonate was obtained under the amount of AFIL increased to 1 mol%. Further increasing the amount of AFIL to 2 mol%, the yield was not increasing anymore.
Reaction condition: Epichlorohydrin (10 mmol), CO2 (0.1 MPa), 80°C, 6 h, GC yield.
Protonation State of Ionic Liquid AFIL
The synthesized AFIL possesses the dual functions of both ionic liquids and organic bases. The previous experimental and theoretical studies (Elageed et al., 2016; Ji et al., 2018) have revealed that the ionic liquids having imidazolium rings could assist the reaction of ring-opening of epoxides by forming hydrogen bonding interaction with epoxides. On the other hand, it has been reported that the yields of products for CO2 conversion catalyzed by the tertiary amines such as DBU (Heldebrant et al., 2005) and DABCO (Ji et al., 2018) increased remarkably in the presence of water. In the reactions of CO2 and epoxides catalyzed by ionic liquids, the appropriate amount of water would facilitate the formation of bicarbonate salts effectively. The reason may be that CO2 can dissolve in H2O to form carbonic acid (H2CO3) which dissociates as bicarbonate () and proton. The tertiary amine groups in ionic liquids possess strong basicity so that they can prompt the dissociation of H2CO3 and increase the dissolution of CO2 in solution. In the first step, we should consider the protonation state of amine group of AFIL in solution because the chemical equilibrium between AFIL and H2CO3 might exist in the solution phase.
The calculated DFT results in Figure 5 indicate that the proton abstraction from H2CO3 by AFIL, denoted as Int-a1, occurs easily to form the complex Int2-a2, releasing an energy of 14.1 kcal/mol. The process of proton transfer from carbonic acid to Int-a1 has no barrier and the formed complex Int2-a2 is quite stable, which demonstrates that the ionic liquid AFIL exists in a protonated state in solution. The releasing of from the complex Int2-a2 needs only 3.5 kcal/mol and the protonated Int-a3 is ready for the subsequent catalytic reaction.
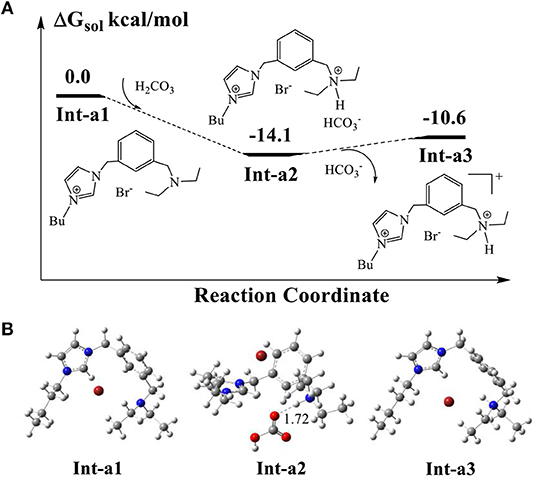
Figure 5. (A) Calculated energy profile for proton transfer from H2CO3 to AFIL. (B) Optimized structures of intermediates corresponding to (A). The units of bond lengths are in Å.
Ring-Opening Reaction of Epichlorohydrin
The previous studies (Elageed et al., 2016; Luo et al., 2016; Huang et al., 2017; Ji et al., 2018) supported that the imidazolium rings of ionic liquids could form hydrogen bonding interaction with epoxides. In AFIL, the moiety of 2-buthylimidazole can assist the ring-opening process of epoxides through hydrogen-bonding interaction with them. Figures 6A,B show the calculated energy profile for the ring-opening reaction of epichlorohydrin as well as the corresponding optimized structures. The protonated Int-a3 in Figure 5 and epichlorohydrin form the stable complex Int-b1 through the bridged oxygen atom of epichlorohydrin. The calculated energy of Int-b1 is −14.9 kcal/mol, which is stable than the isolated Int-a3 and epichlorohydrin by 4.3 kcal/mol. The distance of hydrogen bonding interaction in Int-b1 is 2.08 Å. In addition, we notice that the Br− stays 2.18 Å away from protonated amine group and forms a weak hydrogen bonding interaction, with the distance being 2.18 Å.
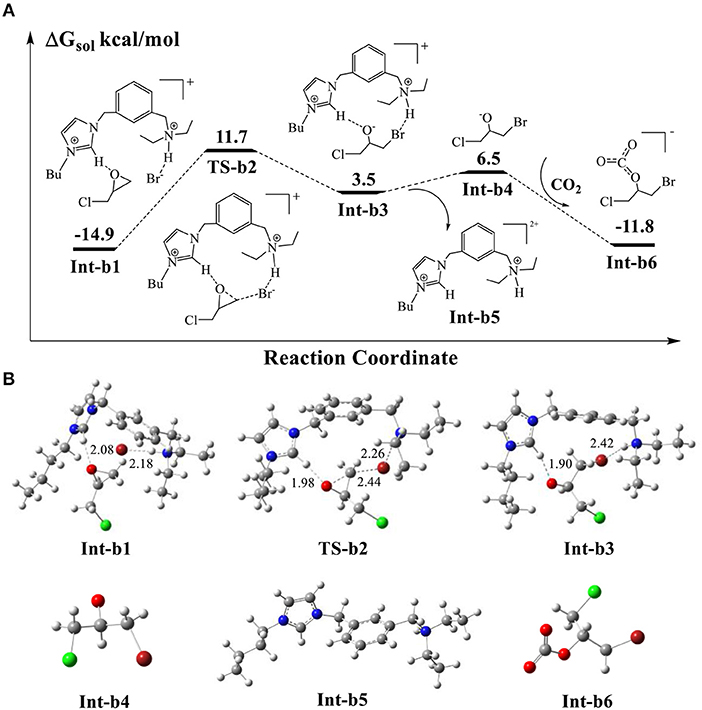
Figure 6. (A) Calculated energy profile for the ring-opening reaction of epichlorohydrin assisted by the hydrogen-bond of AFIL. (B) Optimized structures of intermediates and transition states corresponding to (A). The units of bond lengths are in Å.
Next, the Br− attacks the carbon atom of epichlorohydrin via the transition state TS-b2, with a modest energy barrier of 26.6 kcal/mol. If a count ion is considered in this step, the calculated barrier would be 27.7 kcal/mol and the influence of count ion on barrier is not obvious. With the help of hydrogen bonding interaction of imidazole ring, the O-C bond cleaves to give rise to the ring-opening intermediate Int-b3. We note in Int-b3 that the Br− stabilized by amine group forms covalent bond with the carbon atom of cleaved O-C bond. The oxygen atom carries negative charge, which is stabilized by the acid C-H bond of imidazolium ring. Such an oxy anion species is very active so that the addition reaction could occur by the electrophilic attack of free CO2. It is noticed that the optimized structure of Int-b3 in Figure 6 shows that the ring-opening product is embedded in the catalytic cavity formed by the surrounded ionic liquid molecule. The direct addition of CO2 to the oxy anion species in the complex Int-b3 would encounter a high energy barrier due to the steric effect of surrounded molecule. By taking the thermal dynamics of complexes in solution into account, we propose a reaction pathway for the CO2 addition. It is possible that the complex Int-b3 could dissociate into the isolated oxy anion species Int-b4 and the ionic liquid Int-b5 by breaking the H-bond interaction, with absorbing an energy of 3.0 kcal/mol. Then, the direct electrophilic attack of CO2 to Int-b4 yields the stable addition product Int-b6. This process of CO2 addition is extremely exothermic by 18.3 kcal/mol. The DFT scan calculation (Supplementary Material) shows that the energy profile of CO2 attack is downhill in energy, without an obvious barrier. For the whole ring-opening process, the O-C bond cleavage in the epichlorohydrin of Int-b1 is the rate-determining step, with the calculated barrier of 26.6 kcal/mol.
Generation of Cyclic Carbonate
The final step is the intramolecular ring-closing initiated from the substrate Int-b6. Figure 7 shows the calculated energy profile for the ring-closing process. As shown in TS-b7, one of the oxygen atoms in the carboxyl COO group attacks the terminal carbon atom to form a new O-C bond, while the C-Br bond is being broken at the same time. In the optimized TS-b7, the distances of O-C and C-Br atoms are 1.93 and 2.49 Å, respectively, which indicates that it follows a typical SN2 mechanism. The five-member-ring complex Int-b8 is 4.0 kcal/mol lower than that of Int-b6 in energy. In the structure of Int-b8, the Br− has to stay far away from the cyclic carbonate, since the carbonate itself contains a few atoms such as the oxygen atoms that carry negative charges.
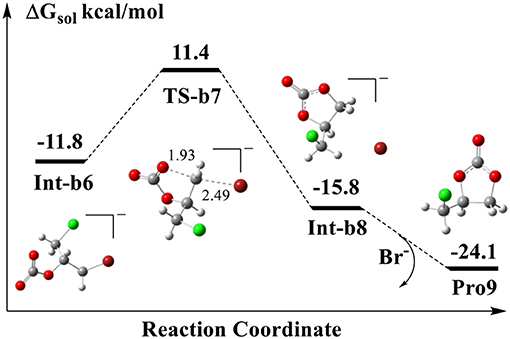
Figure 7. Calculated energy profile for the ring-closing reaction to yield the cyclic carbonate. The units of bond lengths are in Å.
We notice that in the structure of Int-b1 of Figure 6, the Br− anion is stabilized by the N-H bond of amine group. Later, it assists the ring-opening in TS-b2 by attacking the carbon atom and forms the C-Br bond with the carbon atom in Int-b3. After the ring-closing via TS-b7, it is evident that the Br− is released again through the cleavage of the covalent C-Br bond. Our DFT calculation shows that if the free Br− reforms the complex Int-a3 with the Int-b3 and yields the final cylic carbonate Pro9, this process is exothermic in thermodynamics and the energy is lower than that of Int-b8 by 8.3 kcal/mol. The barrier of rate-determining step for the ring-closing process is 23.2 kcal/mol, lower than that step of ring-opening by 3.4 kcal/mol.
Finally, the whole mechanism of CO2 conversion catalyzed by AFIL is summarized in Scheme 1. The protonated ionic liquid Int-a3 catalyzes the ring-opening of Int-b1 to the ring-opening intermediate Int-b3. The direct addition of CO2 to the released Int-b4 yields Int-b6. The species Int-b6 undergoes the ring-closing to yield Int-b8. The Int-b8 gives rise to the final product Pro9 by releasing the Br− who reforms the catalytic Int-a3 with Int-b5.
Conclusion
In this work, we report a new synthesized amine-functional ionic liquid (AFIL) which could catalyze the reaction of CO2 and epichlorohydrin to yield cyclic carbonate. The catalytic efficiency of AFIL for converting CO2 into cyclic carbonate is much higher than that of the respective BnBimBr and DEBA, which exhibit a bifunctional catalytic activity for CO2 conversion. Our DFT calculations indicate that the amine group of AFIL is in a protonated state in solution. The protonated amine group in AFIL could stabilize Br− to attack the carbon atom of epichlorohydrin and assist its ring-opening. Meanwhile, the imidazolium ring in AFIL also could assist the ring-opening of epichlorohydrin through a hydrogen bonding interaction. The rate-determining step of the whole reaction is the ring-opening process, which is in line with the findings in the previous studies. The mechanistic study on the reaction catalyzed by AFIL inspires us to design new multi-functional green catalysts for CO2 conversion with high efficiency.
Data Availability
All datasets generated for this study are included in the manuscript/Supplementary Files.
Author Contributions
FX and GG designed the experiments, DFT calculations, and analyzed the data. TD and LJ performed experiments. HG performed calculations. All the authors wrote the paper together.
Funding
This work was supported by the National Natural Science Foundation of China (Grant Nos. 21433004 and 21573072).
Conflict of Interest Statement
The authors declare that the research was conducted in the absence of any commercial or financial relationships that could be construed as a potential conflict of interest.
Acknowledgments
We acknowledge the support of the NYU-ECNU Center for Computational Chemistry at NYU Shanghai. We also thank the ECNU Multifunctional Platform for Innovation (001) for providing computer time.
Supplementary Material
The Supplementary Material for this article can be found online at: https://www.frontiersin.org/articles/10.3389/fchem.2019.00615/full#supplementary-material
References
Andrae, D., Haussermann, U., Dolg, M., Stoll, H., and Preuss, H. (1990). Energy-adjusted ab initio pseudopotentials for the second and third row transition elements. Theor. Chim. Acta 77, 123–141. doi: 10.1007/BF01114537
Anthofer, M. H., Wilhelm, M. E., Cokoja, M., Drees, M., Herrmann, W. A., Kühn, F. E., et al. (2015). Hydroxy-functionalized imidazolium bromides as catalysts for the cycloaddition of CO2 and epoxides to cyclic carbonates. ChemCatChem 7, 94–98. doi: 10.1002/cctc.201402754
Anthofer, M. H., Wilhelm, M. E., Cokoja, M., Markovits, I. I. E., Pöthig, A., Mink, J., et al. (2014). Cycloaddition of CO2 and epoxides catalyzed by imidazolium bromides under mild conditions: influence of the cation on catalyst activity. Catal. Sci. Technol. 6, 1749–1758. doi: 10.1039/c3cy01024d
Appaturi, J. N., and Adam, F. (2013). A facile and efficient synthesis of styrene carbonate via cycloaddition of CO2 to styrene oxide over ordered mesoporous MCM-41-Imi/Br catalyst. Appl. Catal. B Environ. 136, 150–159. doi: 10.1016/j.apcatb.2013.01.049
Arayachukiat, S., Kongtes, C., Barthel, A., Vummaleti, S. V. C., Poater, A., Wannakao, S., et al. (2017). Ascorbic acid as a bifunctional hydrogen bond donor for the synthesis of cyclic carbonates from co2 under ambient conditions. ACS Sustain. Chem. Eng. 5, 6392–6397. doi: 10.1021/acssuschemeng.7b01650
Aresta, M., Dibenedetto, A., and Angelini, A. (2013). Catalysis for the valorization of exhaust carbon: from CO2 to chemicals, materials, and fuels. Technological use of CO2. Chem. Rev. 114, 1709–1742. doi: 10.1021/cr4002758
Chai, J.-D., and Head-Gordon, M. (2008). Long-range corrected hybrid density functionals with damped atom-atom dispersion corrections. Phys. Chem. Chem. Phys. 10, 6615–6620. doi: 10.1039/b810189b
Chong, C. C., and Kinjo, R. (2015). Hydrophosphination of CO2 and subsequent formate transfer in the 1,3,2-diazaphospholene-catalyzed N-formylation of amines. Angew. Chem. Int. Ed. 54, 12116–12120. doi: 10.1002/anie.201505244
D′Elia, V., Pelletier, J. D. A., and Basset, J. M. (2015). Cycloadditions to epoxides catalyzed by group III-V transition-metal complexes. Chem. Cat. Chem 7, 1906–1917. doi: 10.1002/cctc.201500231
Dolg, M., Wedig, U., Stoll, H., and Preuss, H. (1987). Energy-adjusted ab initio pseudopotentials for the first row transition elements. J. Chem. Phys. 86, 866–872. doi: 10.1063/1.452288
Elageed, E. H. M., Chen, B., Wang, B., Zhang, Y., Wu, S., Liu, X., et al. (2016). Selective synthesis of 5-substituted N-aryloxazolidinones by cycloaddition reaction of epoxides with arylcarbamates catalyzed by the ionic liquid BmimOAC. Eur. J. Org. Chem. 21, 3650–3656. doi: 10.1002/ejoc.201600474
Frisch, M. J., Trucks, G. W., Schlegel, H. B., Scuseria, G. E., Robb, M. A., Cheeseman, J. R., et al. (2009). Gaussian 09, Revision B.01. Wallingford: Gaussian, Inc.
Girard, A. L., Simon, N., Zanatta, M., Marmitt, S., Gonçalves, P., and Dupont, J. (2014). Insights on recyclable catalytic system composed of task-specific ionic liquids for the chemical fixation of carbon dioxide. Green Chem. 16, 2815–2825. doi: 10.1039/c4gc00127c
Heldebrant, D. J., Jessop, P. G., Thomas, C. A., Eckert, C. A., and Liotta, C. L. (2005). The reaction of 1,8-diazabicyclo[5.4.0]undec-7-ene (DBU) with carbon dioxide. J. Org. Chem. 70, 5335–5338. doi: 10.1021/jo0503759
Huang, M. M., Luo, Z. J., Zhu, T., Chen, J., Zhang, J. Z. H., and Xia, F. (2017). A theoretical study of the substituent effect on reactions of amines, carbon dioxide and ethyleneoxide catalyzed by binary ionic liquids. RSC Adv. 7, 51521–51527. doi: 10.1039/c7ra09485j
Hunt, A. J., Sin, E. H., Marriott, R., and Clark, J. H. (2010). Generation, capture, and utilization of industrial carbon dioxide. ChemSusChem 3, 306–322. doi: 10.1002/cssc.200900169
Ji, L. Z., Luo, Z. J., Zhang, Y., Wang, R., Ji, Y., Xia, F., et al. (2018). Imidazolium ionic liquids/organic bases: efficient intermolecular synergistic catalysts for the cycloaddition of CO2 and epoxides under atmospheric pressure. Mol. Catal. 446, 124–130. doi: 10.1016/j.mcat.2017.12.026
Jones, C. W. (2011). CO2 capture from dilute gases as a component of modern global carbon management. Annu. Rev. Chem. Biomol. Eng. 2, 31–52. doi: 10.1146/annurev-chembioeng-061010-114252
Luo, Z., Wang, B., Liu, Y., Gao, G. H., and Xia, F. (2016). Reaction mechanisms of carbon dioxide, ethylene oxide and amines catalyzed by ionic liquids BmimBr and BmimOAc: a DFT study. Phys. Chem. Chem. Phys. 18, 27951–27957. doi: 10.1039/c6cp05291f
Ma, J., Liu, J., Zhang, Z., and Han, B. (2012). The catalytic mechanism of KI and the co-catalytic mechanism of hydroxyl substances for cycloaddition of CO2 with propylene oxide. Green Chem. 14, 2410–2420. doi: 10.1039/c2gc35711a
Monastersky, R. (2013). Global carbon dioxide levels near worrisome milestone. Nature 497, 13–14. doi: 10.1038/497013a
Padwa, A., and Murphree, S. (2005). Epoxides and aziridines – a mini review ARKIVOC 3, 6–33 doi: 10.3998/ark.5550190.0007.302
Peng, J., and Deng, Y. (2001). Cycloaddition of carbon dioxide to propylene oxide catalyzed by ionic liquids. New J. Chem. 25, 639–641. doi: 10.1039/b008923k
Rassolov, V. A., Ratner, M. A., Pople, J. A., Redfern, P. C., and Curtiss, L. A. (2001). 6-31G* basis set for third-row atoms. J. Comput. Chem. 109, 1223. doi: 10.1002/jcc.1058
Rochelle, G. T. (2009). Amine scrubbing for CO2 capture. Science 325, 1652–1654. doi: 10.1126/science.1176731
Sakakura, T., Choi, J. C., and Yasuda, H. (2007). Transformation of carbon dioxide. Chem. Rev. 107, 2365–2387. doi: 10.1021/cr068357u
Sheldon, R. (2001). Catalytic reactions in ionic liquids. Chem. Commun. 23, 2399–2407. doi: 10.1039/B107270F
Song, Y., Cheng, C., and Jing, H. (2014). Aza-crown ether complex cation ionic liquids: preparation and applications in organic reactions. Chem. Eur. J. 20, 12894–12900. doi: 10.1002/chem.201403118
Sun, H., and Zhang, D. (2007). Density functional theory study on the cycloaddition of carbon dioxide with propylene oxide catalyzed by alkylmethylimidazolium chlorine ionic liquids. J. Phys. Chem. A 111, 8036–8043. doi: 10.1021/jp073873p
Sun, J., Zhang, S., Cheng, W., and Ren, J. (2008). Hydroxyl-functionalized ionic liquid: a novel efficient catalyst for chemical fixation of CO2 to cyclic carbonate. Tetrahedron Lett. 49, 3588–3591. doi: 10.1016/j.tetlet.2008.04.022
Wang, B., Elageed, E. H. M., Zhang, D., Yang, S., Wu, S., Zhang, G., et al. (2013). One-pot conversion of carbon dioxide, ethylene oxide, and amines to 3-Aryl-2-oxazolidinones catalyzed with binary ionic liquids. ChemCatChem 6, 278–283. doi: 10.1002/cctc.201300801
Whiteoak, C. J., Nova, A., Maseras, F., and Kleij, A. W. (2012). Merging Sustainability with organocatalysis in the formation of organic carbonates by using CO2 as a feedstock. ChemSusChem 5, 2032–2038. doi: 10.1002/cssc.201200255
Xiao, L., Li, F., Peng, J., and Xia, C. (2006). Immobilized ionic liquid/zinc chloride: Heterogeneous catalyst for synthesis of cyclic carbonates from carbondioxide and epoxides. J. Mol. Catal. 253, 265–269. doi: 10.1016/j.molcata.2006.03.047
Yano, T., Matsui, H., Koike, T., Ishiguro, H., Fujihara, H., Yoshihara, M., et al. (1997). Magnesium oxide-catalysed reaction of carbon dioxide with an epoxide with retention of stereochemistry. Chem. Commun. 12, 1129–1130. doi: 10.1039/a608102i
Yingcharoen, P., Kongtes, C., Arayachukiat, S., Suvarnapunya, K., Vummaleti, S. V. C., Wannakao, S., et al. (2019). Assessing the pKa-dependent activity of hydroxyl hydrogen bond donors in the organocatalyzed cycloaddition of carbon dioxide to epoxides: experimental and theoretical study Adv. Synth. Catal. 361, 366–373. doi: 10.1002/adsc.201801093
Keywords: CO2 conversion, amine-functionalized ionic liquids, cyclic carbonates, DFT calculations, mechanism
Citation: Chen J, Gao H, Ding T, Ji L, Zhang JZH, Gao G and Xia F (2019) Mechanistic Studies of CO2 Cycloaddition Reaction Catalyzed by Amine-Functionalized Ionic Liquids. Front. Chem. 7:615. doi: 10.3389/fchem.2019.00615
Received: 29 May 2019; Accepted: 23 August 2019;
Published: 10 September 2019.
Edited by:
Shuanglin Qu, Hunan University, ChinaReviewed by:
Wei Guan, Northeast Normal University, ChinaAlbert Poater, University of Girona, Spain
Copyright © 2019 Chen, Gao, Ding, Ji, Zhang, Gao and Xia. This is an open-access article distributed under the terms of the Creative Commons Attribution License (CC BY). The use, distribution or reproduction in other forums is permitted, provided the original author(s) and the copyright owner(s) are credited and that the original publication in this journal is cited, in accordance with accepted academic practice. No use, distribution or reproduction is permitted which does not comply with these terms.
*Correspondence: Guohua Gao, ghgao@chem.ecnu.edu.cn; Fei Xia, fxia@chem.ecnu.edu.cn
†These authors have contributed equally to this work