- Department of Applied Chemistry, College of Science, Northeast Agricultural University, Harbin, China
A novel Al3+ chemosensor NPA was designed and synthesized on basis of the mechanism of ICT and CHEF. Upon addition of Al3+, the probe NPA displayed a bright green fluorescence under UV radiation and visual color change from yellow to colorless. Spectrum titrations showed that NPA could be recognized as a fluorescent turn-on probe with 10−8 M detection level. The probe was successfully applied in real water sample and test paper. More important, NPA-Al3+ complex were used as a fluorescent turn-off probe for the detection of ClO− with the detection as low as 2.34 × 10−8 M. The performance of NPA to Al3+ and NPA-Al3+ complex to ClO− demonstrated that NPA could be served as a sensitive probe and exhibit INHIBIT logic gate behavior with Al3+ and ClO− as inputs.
Introduction
Aluminum, the third most abundant metal element in the Earth's crust, is widely used in human's daily life including pharmaceuticals, textile, kitchen utensils, and paper industries (Zhang et al., 2016; Kaur and Kaur, 2017). Moreover, Al3+ ion widely exists in the environment, normally in natural waters and many plants, which can enter the human body through foods and water. However, excess aluminum can damage the human nervous system, and has tightly relation to many diseases such as Alzheimer's disease, Parkinson's disease, anemia, dementia, encephalopathy, and gastrointestinal diseases (Helal et al., 2013; Liu et al., 2014, 2017; Jiang et al., 2015; Zeng et al., 2018). In addition, hypochlorous acid (HOCl), known as an important reactive oxygen species (ROS) in many living organisms, plays a vital role in many biological processes (Zhu et al., 2014). Hypochlorite is a key microbicide that is used for natural defense because it behaves as a strong nucleophilic non-radical oxidant (Shi et al., 2010). However, it also results in many pathological diseases, especially is implicated in inflammation-associated injury including hepatic ischemia-reperfusion injury, lung injury, rheumatoid arthritis, and atherosclerosis. Moreover, excessive or misplaced of hypochlorite can also cause detrimental effect on tissues (Lei et al., 2017; Lin et al., 2017; Shen et al., 2017). Therefore, the determination of Al3+ and ClO− in biological samples is of great importance.
In the last few decades, the designing a molecular system, which displayed significant changes in electronic, magnetic, or optical signals even at low concentration during the detection for a specific guest species, was a hot topic for many researchers (Kim et al., 2013; Liu et al., 2014; Kang Y. et al., 2016; Lim et al., 2017). Among them, small-molecule optical probes, which exhibited many merits including high selectivity and sensitivity, tunability, simple manipulation, and direct visualization, were employed as a powerful tool in the facet of trace analysis and rapid detection for various analytes (Qin and Yang, 2015; Wang et al., 2015; Simon et al., 2016; Liu et al., 2017; Murugan et al., 2017). Up to now, lots of papers that one probe only for the detection of one special analyte such as Al3+ ion (Tang et al., 2015; Gupta and Kumar, 2016; Xie et al., 2017) or Hypochlorous acid (Zhang et al., 2017) had been reported, but the design idea, one fluorescent probe for successively recognition of two different analytes, had gained increasing attention in considering its high efficiency and potential cost reduction (Wang et al., 2014; Ye et al., 2014; Zhao et al., 2015; Wen and Fan, 2016; Xie et al., 2016; Zhai et al., 2016; Zhu et al., 2016; Wu et al., 2017). Many excellent probes had been reported for relay recognition of two different ions through fluorescent “off-on-off” (Borasea et al., 2015; He et al., 2015; Zhao et al., 2015; Rai et al., 2016; Bhattacharyya et al., 2017; Das et al., 2017; Jo et al., 2017; Dwivedi et al., 2018; Feng et al., 2018; Lim et al., 2018) or “on-off-on” (Diao et al., 2016; Zhao et al., 2016; Sarkar et al., 2017) mode. Moreover, 1, 8-naphthalimide fluorophore, holding many excellent photophysics properties, such as high photostability, visible absorption and fluorescence emission, large Stokes' shift and high fluorescence quantum yield (Dimov et al., 2014; Yu and Zhang, 2014; Kang L. et al., 2016; Li et al., 2018; Fu et al., 2019), was successfully applied in designing fluorescent probes toward various analytes.
Taking above statements into consideration, in this paper, we prepared and characterized a 1, 8-naphthalimide-based fluorescent probe NPA for the detection of Al3+ embodied in colorimetric and fluorescent turn-on was ascribed to the co-contribution of intramolecular charge transfer (ICT) and chelation enhanced fluorescence (CHEF). The binding stoichiometry between the NPA and Al3+ had been clarified according to various spectroscopic measurements and data analysis. Moreover, the performance of in situ formed NPA-Al3+ complex for the detection of ClO− was investigated. The NPA-Al3+ complex exhibited a fluorescent turn-off response in the detection of ClO−. Inspiringly, to the best of our knowledge, a single probe for sequential detection of Al3+ and ClO− through fluorescence “off-on-off” mode is scarcely documented.
Materials and Methods
Reagents and Instrument
All the solvents and reagents (analytical or spectroscopic grade) were purchased commercially and used as received. Metal salts [NaClO4, KClO4, Mg(ClO4)2, Ba(ClO4)2, Zn(ClO4)2•6H2O, Cu(ClO4)2•6H2O, AgNO3, Cd(NO3)2, Pb(NO3)2, Co(NO3)2•6H2O, Ni(NO3)2•6H2O, Ca(NO3)2•4H2O, Al(NO3)3•9H2O, MnSO4•H2O, HgCl2, FeCl2•4H2O] were obtained from commercial suppliers, and used as received without further purification. 1H NMR and 13C NMR spectra were recorded on a AV- 600 spectrometer in DMSO-d6 solution. The chemical shifts (δ) are reported in ppm and coupling constants (J) in Hz relative to TMS (0.00) for 1H NMR and 13C NMR. Mass spectra were measured on a Waters Xevo UPLC/G2-SQ Tof MS spectrometer. Absorption spectra were recorded using a Pgeneral TU-1901 UV-vis spectrophotometer. Fluorescence measurements were performed on a Perkin Elmer LS55 fluorescence spectrometer at room temperature.
Synthesis of the Probe NPA
NPA was prepared in four steps from naphthalimide as the starting material, as shown in Scheme 1. Compound 1–4 were synthesized using the literature method (Kang L. et al., 2016; Li et al., 2018).
The compound 4 (330 mg, 0.97 mmol) and 2-amino-propane-1, 3-diol (72 mg, 0.8 mmol) were dissolved in ethanol (45 mL) and stirred over anhydrous Et3N (80 mg, 0.58 mmol) for 24 h at room temperature. The crude product was purified by column chromatography with CH3OH/CHCl3 (1:20, v/v) as the eluent and further purified by recrystallization from ethanol to get orange-yellow solid NPA (210 mg, yield: 46%); m.p: 195–196°C.1HNMR (600 MHz, DMSO) (Supplementary Figure 1): δ (ppm) (d, J = 7.2 Hz, 1H), 8.43 (d, J = 8.5 Hz, 1H), 8.39 (d, J = 8.1 Hz, 1H), 7.83–7.75 (m, 1H), 7.50 (d, J = 8.5 Hz, 1H), 7.35 (d, J = 8.1 Hz, 1H), 4.71 (t, J = 5.4 Hz, 2H), 4.07–3.97 (m, 2H), 3.82–3.72 (m, 1H), 3.54–3.45 (m, 2H), 3.46–3.38 (m, 2H), 3.27 (s, 4H), 3.10 (s, 2H), 2.80 (s, 4H), 1.64–1.54 (m, 2H), 1.39–1.30 (m, 2H), 0.92 (t, J = 7.4 Hz, 3H). 13C NMR (151 MHz, DMSO) (Supplementary Figure 2): δ (ppm) 168.62, 163.32, 162.81, 155.32, 131.96, 130.42, 130.26, 128.89, 125.84, 125.10, 122.37, 115.42, 114.92, 60.86, 59.69, 52.53, 52.40, 51.91, 38.91, 29.51, 19.59, 13.52. MS (ESI) (Supplementary Figure 3): m/z [NPA+H+]+ calcd 469.2451, found 469.2458.
Preparation of Solutions for Spectral Detection
All stock solutions (10 mM) including metal cations and the anions were prepared with distilled water, while the stock solution of compound NPA (0.1 mM) was prepared in CH3OH (100 mL). One milliliter of NPA solution (0.1 mM) was diluted in 9 mL CH3OH to make the test solutions (10 μM). For fluorescence measurements, excitation was set at 400 nm, and the excitation and emission slit widths were 10 and 10 nm, respectively. All spectroscopic measurements were performed in CH3OH at room temperature.
Stock solution of NPA (0.1 mM) was prepared by NPA (0.01 mmoL) was dissolved in CH3OH (100 mL), the test solutions of NPA (10 μM) was prepared by adding 1 mL of NPA stock solution (0.1 mM) was diluted in 9 mL CH3OH in CH3OH.
Stock solutions (10 mM) including the metal cations and the anions were prepared with ultrapure water, respectively. For spectrum measurement, the test solutions were prepared by adding certain amount of stock solution using a pipette into NPA stock solution. For fluorescence measurements, excitation was set at 400 nm, and the excitation and emission slit widths were 10 and 10 nm, respectively.
Determination of Binding Constant and Detection Limit
According to the fluorescence intensity data, the binding constant of NPA with Al3+ was calculated based on the modified Benesi–Hildebrand equation (Li et al., 2018) as followed. Where, Fmax, F and Fmin are the fluorescence intensities of NPA in the presence of Al3+ at saturation, at an intermediate Al3+ concentration, and absence of Al3+, respectively. K is the stability constant.
The limit of detection (LOD) of Al3+ was calculated on the basis of 3δ/K according to the fluorescence changes, δ is the standard deviation of the blank solution, and K is slope of calibration curve (Borasea et al., 2015; Zeng et al., 2018).
Results and Discussion
The UV-Vis Spectra Responses of Probe NPA
Various metal ions: K+, Na+, Ca2+, Mg2+, Ba2+, Pb2+, Cu2+, Co2+, Ni2+, Fe2+, Cd2+, Hg2+, Mn2+, Ag+, Zn2+, and Al3+ were used to observe the selectivity of probe NPA (10 μM) and their UV-vis spectra were measured in CH3OH. As shown in Figure 1, NPA exhibited a characteristic absorbance band at 400 nm in the presence of tested metal ions (K+, Na+, Ag+, Ca2+, Mg2+, Ba2+, Pb2+, Cu2+, Co2+, Ni2+, Fe2+, Cd2+, Hg2+, Mn2+, Zn2+) and there was almost no difference compared with that of NPA itself. However, upon the addition of Al3+, the maximum absorbance peak of NPA was blue-shifted with 21 nm from 399 to 378 nm, and the solution color was changed from yellow to colorless. This result might attribute to the decrease of conjugated degree resulting from the decrease of electron donating ability of piperazine ring to the naphthalimide after the complexation with Al3+ (De Silva et al., 1997; Urano et al., 2015; Kang L. et al., 2016).
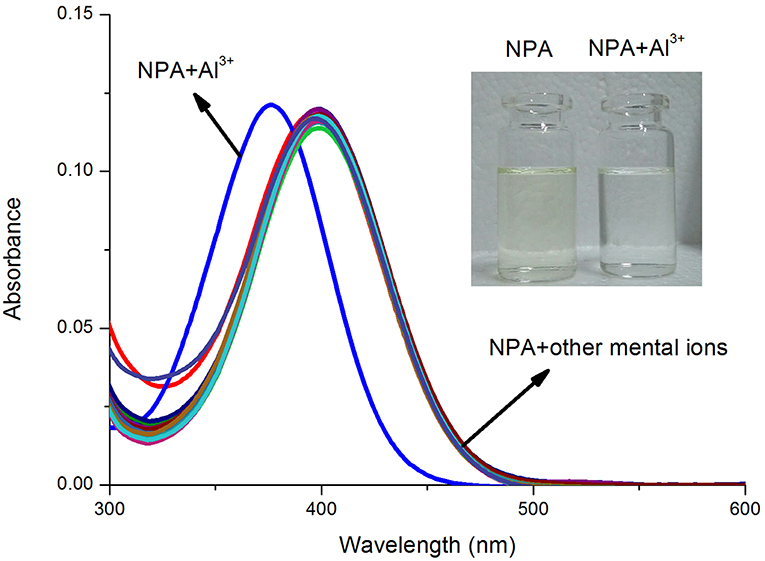
Figure 1. UV-vis absorbance spectra of NPA (10 μM) in the absence and presence of various mental ions (50 μM) in CH3OH. Inset: The color change of NPA (10 μM) in the presence of Al3+ ions (5 equiv.) in CH3OH under natural light.
In addition, the quantitative sensing of Al3+ ion was elucidated by UV-vis titration of probe NPA in CH3OH solution (Figure 2). NPA alone showed a major absorbance band at 399 nm, a new band at 378 nm appeared upon addition of Al3+ and the intensity increased gradually and then kept constant until the amount added of Al3+ was more than 20 μM. The good relationship was found between the ratio of absorbance (A378/A399) vs. the concentration of Al3+ (Supplementary Figure 4), and the limit of detection (LOD) for Al3+ was calculated as 3.61 × 10−8 M on the basis of 3 δ/K (where δ is deviation of the blank signal and K is slope of calibration curve; Borasea et al., 2015; Zeng et al., 2018). These results indicated that NPA could be used as an absorbance-ratiometric probe for the detection of Al3+.
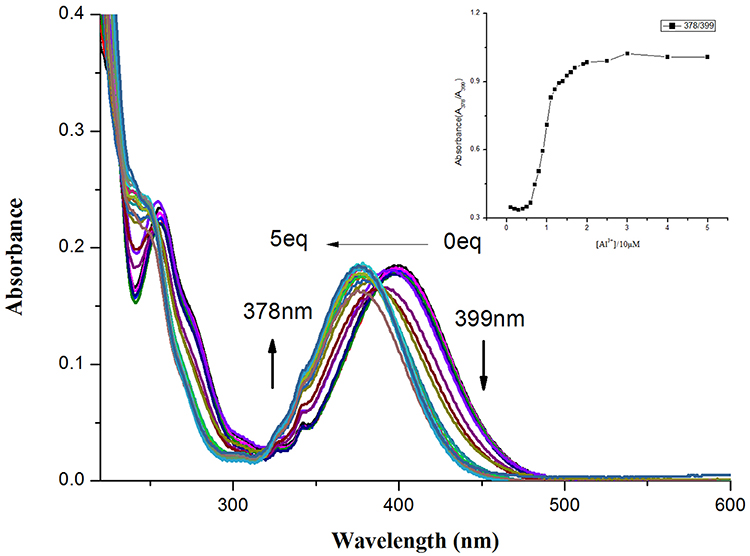
Figure 2. UV-vis spectral of NPA (10 μM) upon addition of increasing concentration of Al3+ (0–5 equiv.) in CH3OH. Insert: Plot of absorbance intensity ratio (A378/A399) as a function of the Al3+ concentration.
Fluorescence Spectral Responses of NPA
The fluorescence properties of NPA were investigated in the presence of a variety of metal ions in CH3OH (Figure 3). NPA itself exhibited almost no fluorescence emission upon excitation at 400 nm. However, the addition of Al3+ (5 equiv.) into NPA induced an obvious fluorescence enhancement at 505 nm, which might be attributed to the CHEF effect through the formation of a rigid system after binding with Al3+ (Kang L. et al., 2016; Zeng et al., 2019). In contrast, few fluorescence changes were observed in the presence of other tested metal ions, demonstrating that NPA was highly selective for Al3+ over competing metal ions.
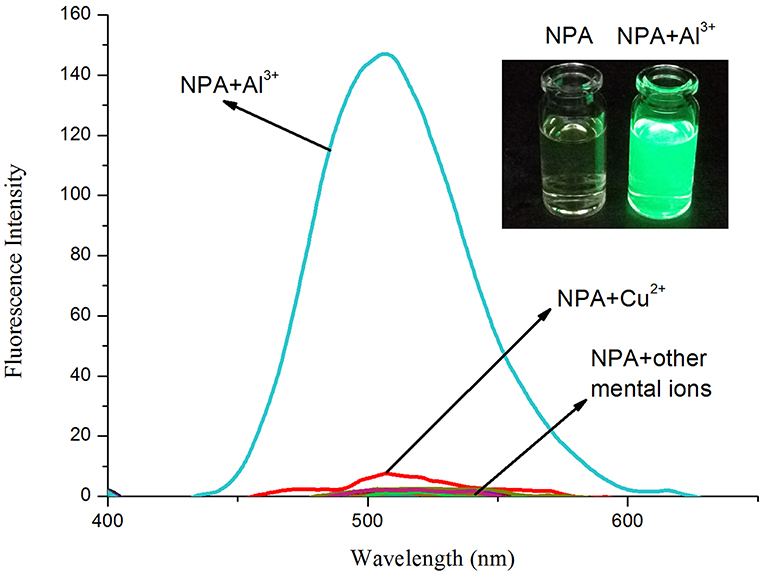
Figure 3. Fluorescence spectral changes (λex = 400 nm) of NPA (10 μM) in the presence of various mental ions (50 μM) in CH3OH. Inset: The color change of NPA (10 μM) in the presence of Al3+ ions (5 equiv.) in CH3OH under 365 nm UV lamp.
To further validate the utility of probe NPA, the fluorescence titrations of NPA were performed by gradually increasing the concentration of Al3+ (Figure 4). The emission intensity of NPA at 505 nm progressively increased upon addition of Al3+, and the emission intensity remained constant when the quantity of Al3+ added was over 15 μM (Figure 4, Insert). Moreover, the fluorescence enhancement of sensor NPA depending on the concentration of Al3+ was in a linear manner (Supplementary Figure 5). The detection limit (LOD) for Al3+ ion according to fluorescence changes was measured to be 2.03 × 10−8 M on the basis of 3 δ/K (Borasea et al., 2015; Zeng et al., 2018). The result clearly demonstrated that the probe NPA was highly efficient in sensing Al3+ at nanomolar level.
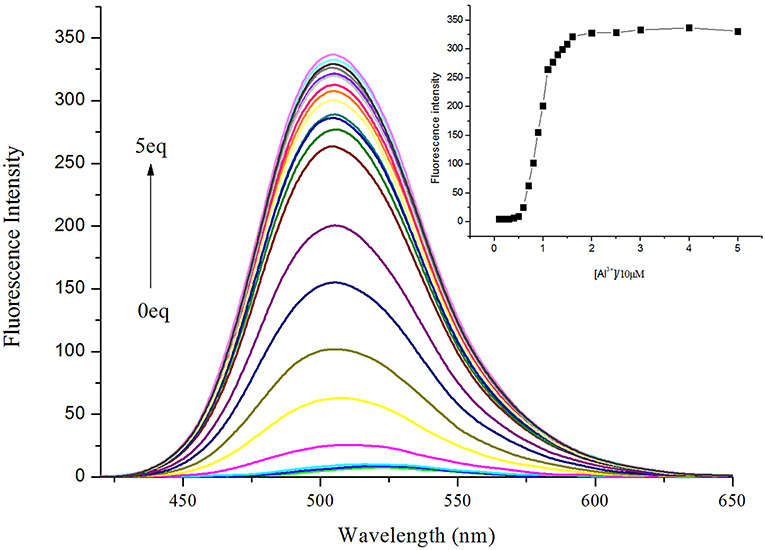
Figure 4. Fluorescence emission spectral (λex = 400 nm) of NPA (10 μM) with increasing concentration of Al3+ in CH3OH. Inset: Plot of fluorescence intensity at 505 nm as a function of the Al3+ concentration.
Further, tolerance of fluorescence intensity of NPA-Al3+ complex in presence of other metal ions was tested (Figure 5). All competitive metal ions had no obvious interference on the Al3+ detection, indicated that NPA-Al3+ complex was hardly affected by these coexistent metal ions. Accordingly, NPA can be used as selective fluorescent probe for Al3+ determination without disturbance of other competing metal ions.
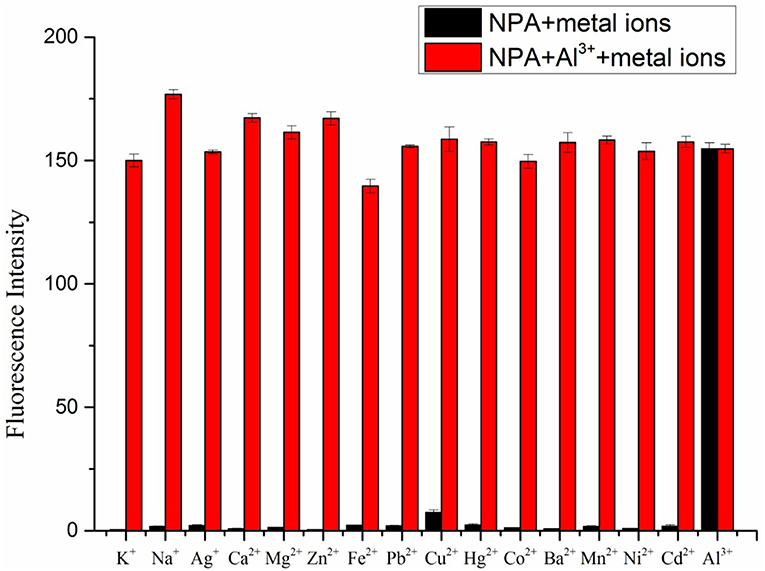
Figure 5. Fluorescence intensity of NPA (10 μM) at 505 nm upon addition of Al3+ (50 μM) in the presence of various mental ions (50 μM) (λex = 400 nm). (Error bar was represented as mean ± standard deviation, n = 3).
Response Time Studies
As for an excellent fluorescent probe, fluorescent stability and response time are two crucial factors. Hence, the changes of fluorescent intensity of NPA and the response time of NPA to Al3+ were investigated (Supplementary Figure 6). The results demonstrated that the fluorescence signal of NPA remained stable for a long time in the absence of Al3+, implying the NPA had good fluorescence stability. Moreover, after addition of Al3+, the fluorescence intensity of NPA at the 505 nm reached the maximum within 10 s and maintained constant more than 3 min, indicating the unique feature of high complexation ability of NPA with Al3+.
Binding Stoichiometry and Sensing Mechanism
The total concentration of Al3+ and ligand was 50 μM with the molar ratio of Al3+ changed from 0.1 to 0.9. The fluorescence mission was measured for each sample in CH3OH with the excitation wavelength at 400 nm. The maximum point appeared at a mole fraction of 0.5 (Figure 6), indicating a 1:1 stoichiometry of the binding mode between NPA and Al3+, and which was further clarified by a peak at m/z 594.1575, which was assignable to [NPA – 2H+ + K++ Al3+ + ]+ (calcd. m/z 594.1547) in the ESI mass spectrum (Figure 7).
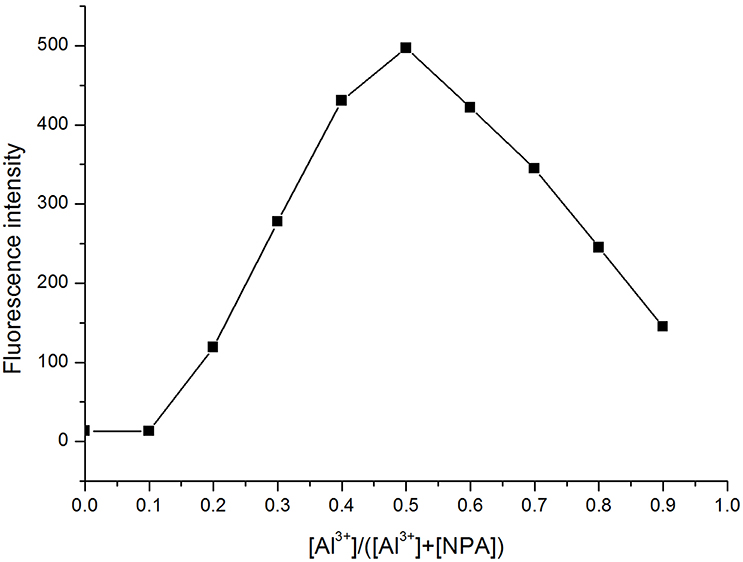
Figure 6. Job's plot of NPA with Al3+ in CH3OH. {[Al3+]/([Al3+] + [NPA])} is the molar fraction of Al3+ ion.
According to the above results, the association constant was calculated as 7.06 × 104 M−1 according to the fluorescence titration data (Supplementary Figure 7), basing on the Benesi-Hildebrand plot (Li et al., 2018).
To better evaluate the interaction of NPA with Al3+, 1H NMR spectra of NPA were constructed in the absence and presence of different equivalent Al3+ in DMSO (Figure 8). The protons of H1 and H2 of NPA at around 2.80 and 3.27 ppm were shifted downfield to 3.42 upon the addition of Al3+. In addition, the peak of NPA at around 3.10 ppm was also shifted downfield to 3.42 ppm, indicated that the two nitrogen atoms of piperazine ring might coordinate the Al3+. Moreover, the proton of amide was disappeared supporting the occurrence of deprotonation upon the interaction of amide with the Al3+. According to the above results, the two nitrogen atoms of piperazine ring and the amide nitrogen atom might coordinate with Al3+ (Scheme 2).
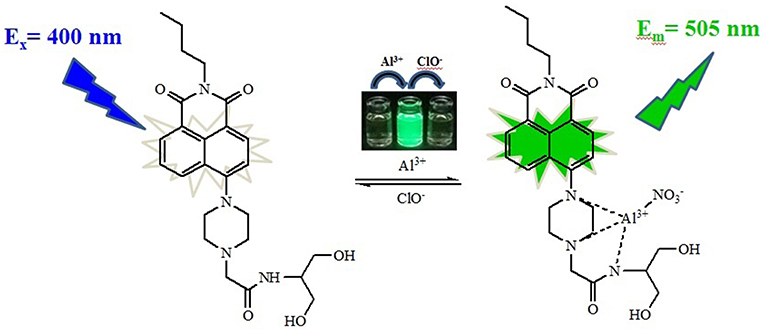
Scheme 2. Proposed mechanism for the fluorescent sensing of NPA and its in situ complex with Al3+ and ClO−.
Reversibility of NPA Toward Al3+
The fluorescence intensity enhancement of NPA on interaction with Al3+ was found to be reversible by using EDTA as the recovering reagent. When the strong metal ion chelating agent EDTA was added gradually to a mixture of NPA-Al3+, the UV-vis absorbance spectral and fluorescence spectral of the solution of NPA-Al3+ almost recovered to the original condition in the absence of Al3+ (Supplementary Figures 8, 9). These results indicating that recognition process can be made reversible merely by treatment with EDTA. Besides, as for an excellent chemosensor, reversibility and regeneration are crucial for the fabrication of apparatus to sense Al3+.
Application of NPA for Al3+ Analysis in Test Paper
Interestingly, the noticeable colorimetric changes of the system and qualitative recognition of Al3+ in solution were confirmed by the simple test strips. The required test strips were immersed in CH3OH (10 mL) including Al3+ and then dried in air. The color changes in various concentrations of Al3+ under sunlight and under 365 nm UV light were illustrated in Supplementary Figure 10. Visual color changed from yellow to colorless and bright-green fluorescence increased gradually with increasing amounts of Al3+ were clearly observed. This result showed that NPA might be used as a portable detector for Al3+.
Application of NPA for Al3+ Analysis in Water Samples
In order to verify the practical application of NPA, ultrapure water and tap water samples were analyzed by the proposed fluorimetric method. The results were summarized in Supplementary Table 1 with satisfactory recovery of Al3+, indicating that the present fluorescent probe seem to be applicable for the determination of Al3+ in environmental analysis.
Fluorescent “On-Off” Sensing of ClO− by NPA-Al3+ Complex
In order to further explore the performance of NPA-Al3+ complex to different anionic, the fluorescence spectra of NPA-Al3+ complex were investigated in presence of various 100 μM anions (ClO−, ROO−, H2O2, OH−, NO−, Cl−, Br−, , , ) in CH3OH (Figure 9). The result showed that fluorescence intensity of NPA-Al3+ complex was affected to tolerable degree by I−, H2O2, and except for ClO−, which completely quenched the fluorescence of NPA-Al3+ complex. This result suggested that the NPA-Al3+ complex could distinguish ClO− anion over other ROO−, H2O2, OH−, NO−, Cl−, Br−, , , by fluorescence “on-off” mode.
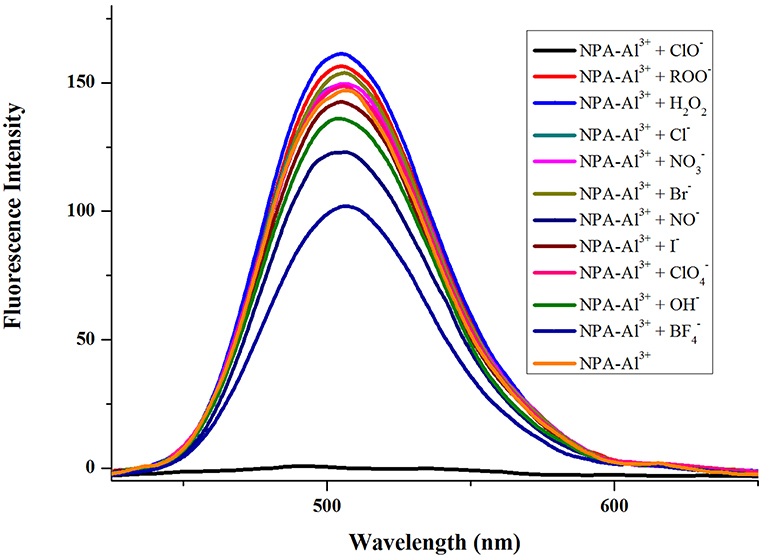
Figure 9. Fluorescence spectra (λex = 400 nm) of NPA-Al3+ system in presence of various anions (100 μM) in CH3OH.
Furthermore, competition experiments were carried out by addition of various anions (100 μM) to the solution of NPA-Al3+ in the presence of 100 μM of ClO−. As shown in Figure 10, competitive anion had no prominent interference with the determination of ClO−, which meant that NPA-Al3+ could perform as a highly selective probe for ClO− via a fluorescence “turn-off” mechanism.
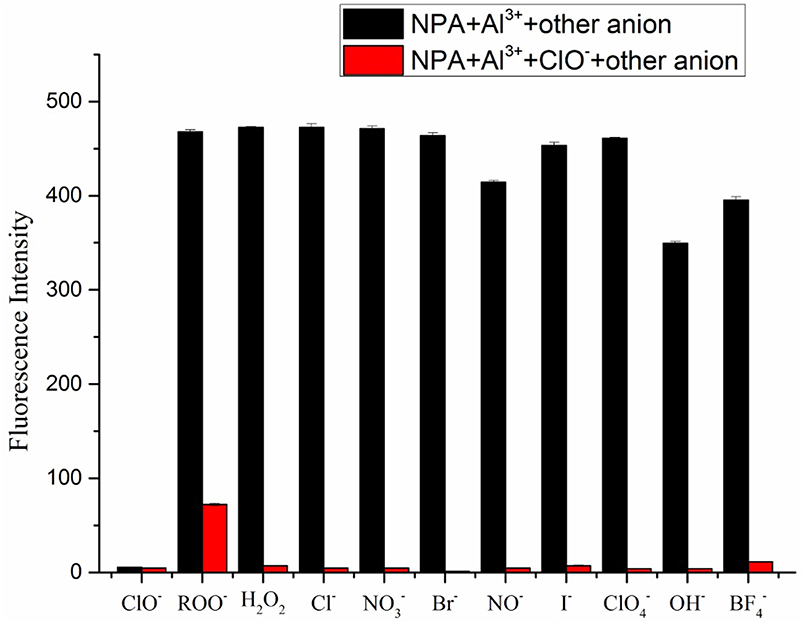
Figure 10. Changes in emission intensity of the NPA-Al3+ complex at 505 nm upon addition of 100 μM of various anions followed by addition of ClO− (λex = 400 nm). (Error bar was represented as mean ± standard deviation, n = 3).
To further investigate the binding ability and limit of detection of the NPA-Al3+ complex with ClO−. The complex sensing capability was studied in detail analysis with fluorescence titration (Figure 11). Upon addition of ClO− to the solution containing NPA-Al3+ complex, fluorescent intensity at 505 nm gradually diminishes, and 40 μM of ClO− could lead to complete fluorescent quenching (Figure 11, inset). Obviously, the fluorescence intensity of NPA-Al3+ complex depending on the concentration of ClO− was in a linear manner (Supplementary Figure 11). According to the fluorescence titration data, the detection limit of NPA-Al3+ complex was determined and be found to 2.34 × 10−8 M (Supplementary Table 3). Furthermore, fluorescence quenching in situ also indicated that the occurrence of dissociation of NPA-Al3+ complex, and the NPA was regenerated upon the addition of ClO−, which further supported by the verified experiments as followed. Firstly, the peak at m/z 469.2438 appeared in ESI–MS spectrum of NPA-Al3+ complex upon addition of ClO− in CH3OH, which was assignable to [NPA+H+]+ (calcd m/z 469.2451; Supplementary Figure 12) compared with the ESI–MS spectrum of NPA itself (Supplementary Figure 13). Moreover, the UV-vis absorbance and fluorescence spectrum of NPA-Al3+ complex in the absence and presence of ClO− were measured (Figure 12), respectively. The result showed that both of them were almost the same as that of NPA itself. Lastly, the titration of 1H NMR (Supplementary Figure 14) and 13C NMR (Supplementary Figure 15) were also investigated to clarify the sensing mechanism. The result displayed that NPA was regenerated to some extent upon the addition of ClO− to the NPA-Al3+ complex. The above result implies that the NPA-Al3+ complex could act as a fluorescent turn-off probe for ClO− recognition. According to above results, the “off-on-off” mechanism was achieved with sequence specificity (Al3+ and ClO−) in CH3OH (Scheme 2). In addition, the results of the comparison between NPA and those reported sensors (one fluorescent probe for successively recognition of two different analytes) were summarized in Supplementary Table 2.
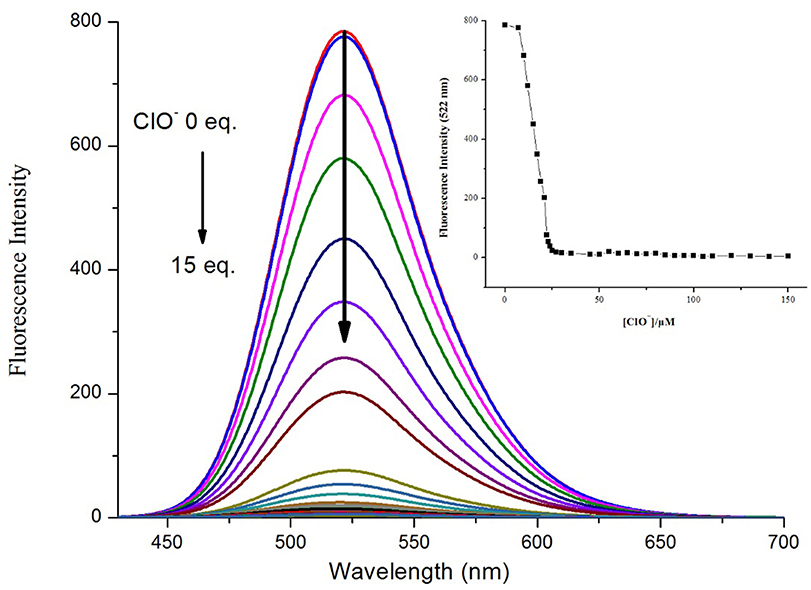
Figure 11. Fluorescence spectral (λex = 400 nm) of NPA-Al3+ complex at different added concentration of ClO− in CH3OH. Insert: Plot of fluorescence intensity of verse ClO− concentration in in CH3OH.
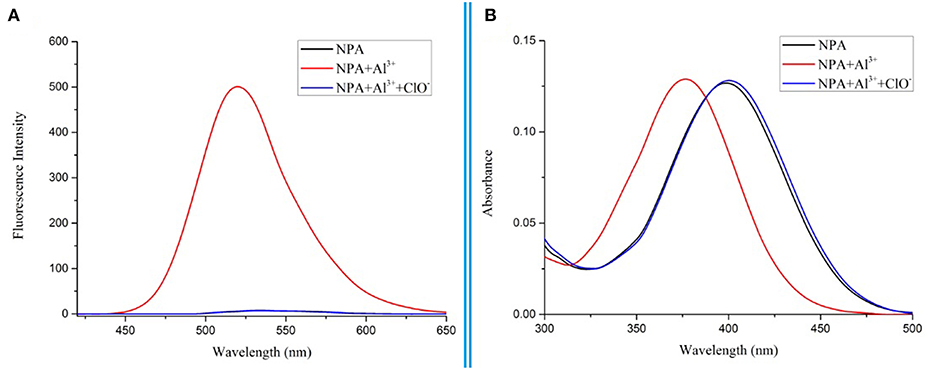
Figure 12. Fluorescence spectrum (A) and UV-vis absorbance spectrum (B) of NPA in CH3OH while adding Al3+ (3 eq.) and ClO− (10 eq.) to the solution.
Application as Logic Gate Function
NPA alone displayed very weak fluorescence emission. Maximum emission at 505 nm appeared after coordination of NPA with the Al3+. Moreover, when ClO− was added to the above solution, the emission intensity at 505 nm was quenched. In addition, the fluorescence “off-on-off” response for Al3+ and ClO− were carried out (Supplementary Figure 16), and the result showed that cycle times was more than 5 according to NPA fluorescent signal upon the alternate addition of Al3+ and ClO−. Due to the remarkable fluorescence changes of probe NPA in the presence Al3+ and ClO−, it would be able to construct a two output combinatorial logic circuit with two signal inputs that are input 1 (Al3+) and input 2 (ClO−; Figure 13A). Input 1 caused fluorescence enhancement, equivalent to a YES operation, while input 2 resulted in fluorescence quenching, thereby enforcement of the NOT gate. In the presence of both inputs, the quenching (by Input 2) has precedence over the fluorescence enhancement by Input 1, which was in accordance with the truth table illustrated in Figure 13B. Hence, by monitoring the emission maxima at 505 nm by two inputs (Al3+ and ClO−), a monomolecular circuit displaying an INHIBIT logic function could be achieved.
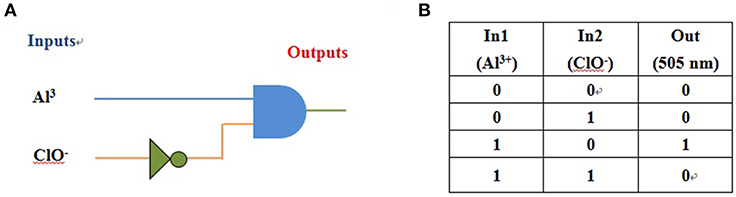
Figure 13. (A) The logic circuit displaying memory unit with two inputs (Al3+ and ClO−) and one output; (B) corresponding truth table.
Conclusion
In conclusion, we had developed a naphthalimide-based sensor NPA which exhibited a turn-on fluorescence response toward Al3+ with a bright green fluorescence under UV radiation and visual color change from yellow to colorless detected by naked-eye. The binding stoichiometry of NPA with Al3+ was 1:1 confirmed by job's plot, HRMS and 1H NMR and 13C NMR titration. The application of NPA for detection and assessing the existence of Al3+ in real sample was also successfully achieved. Moreover, NPA-Al3+ complex were further used as a fluorescent turn-off probe for the detection of ClO− with the detection as low as 2.34 × 10−8 M. The INHIBIT molecular logic gate was effectively constructed by the performance of NPA to Al3+ and NPA-Al3+ complex to ClO−. The development of multi-functional chemosensor for the detection of biological-related analyst in pure water is our future work.
Data Availability
The raw data supporting the conclusions of this manuscript will be made available by the authors, without undue reservation, to any qualified researcher.
Author Contributions
Z-YX constructed the workflow and completed the paper. N-NL synthesized and purified the compound. X-JS and T-TL measured and interpreted the data the UV/Vis and fluorescence experiments. HF and FY performed the real sample analysis.
Funding
This work was supported by Heilongjiang Postdoctoral Funds for scientific research initiation (No. LBH-Q14023) and the Research Science Foundation in Technology Innovation of Harbin (No. 2017RAQXJ022).
Conflict of Interest Statement
The authors declare that the research was conducted in the absence of any commercial or financial relationships that could be construed as a potential conflict of interest.
Acknowledgments
We would like to thank our team leader Professor Ying Fu (Northeast Agricultural University) for financial supporting in paper publishing.
Supplementary Material
The Supplementary Material for this article can be found online at: https://www.frontiersin.org/articles/10.3389/fchem.2019.00549/full#supplementary-material
References
Bhattacharyya, A., Ghosh, S., Makhal, S. C., and Guchhait, N. (2017). Harnessing a pyrimidine based molecular switch to construct reversible test strips for F−/AcO− with respect to Al3+: a colorimetric approach. Spectrochim. Acta Part A Mol. Biomol. Spectrosc. 179, 242–249. doi: 10.1016/j.saa.2017.02.036
Borasea, P. N., Thalea, P. B., Sahoo, S. K., and Shankarlinga, G. S. (2015). An “off–on” colorimetric chemosensor for selective detection of Al3+, Cr3+ and Fe3+: its application in molecular logic gate. Sens. Actuators B Chem. 215, 451–458. doi: 10.1016/j.snb.2015.04.013
Das, D. K., Kumar, J., Bhowmick, A., Bhattacharyya, P. K., and Banu, S. (2017). 2-Hydroxyacetophenone and ethylenediamine condensed Schiff base: fluorescent sensor for Al3+ and , biological cell imaging and INHIBIT logic gate. Inorg. Chim. Acta 462, 167–173. doi: 10.1016/j.ica.2017.03.003
De Silva, A. P., Gunaratne, H. Q., Gunnlaugsson, T., Huxley, A. J., McCoy, C. P., Rademacher, J. T., et al. (1997). Signaling recognition events with fluorescent sensors and switches. Chem. Rev. 97, 1515–1566. doi: 10.1021/cr960386p
Diao, Q. P., Ma, P., Lv, L. L., Li, T., Sun, Y., Wang, X. H., et al. (2016). A water-soluble and reversible fluorescent probe for Al3+ and F− in living cells. Sens. Actuators B Chem. 229, 138–144. doi: 10.1016/j.snb.2016.01.136
Dimov, S. M., Georgiev, N. I., Asiri, A. M., and Bojinov, V. B. (2014). Synthesis and sensor activity of a PET-based 1,8-naphthalimide probe for Zn2+ and pH determination. J. Fluoresc. 24, 1621–1628. doi: 10.1007/s10895-014-1448-2
Dwivedi, R., Singh, D. P., Chauhan, B. S., Srikrishna, S., Panday, A. K., Choudhury, L. H., et al. (2018). Intracellular application and logic gate behavior of a ‘turn off-on-off' type probe for selective detection of Al3+ and F− ions in pure aqueousmedium. Sens. Actuators B Chem. 258, 881–894. doi: 10.1016/j.snb.2017.11.173
Feng, E., Fan, C., Wang, N. S., Liu, G., and Pu, S. Z. (2018). A highly selective diarylethene chemosensor for colorimetric detection of CN− and fluorescent relay-detection of Al3+/Cr3+. Dyes Pigm. 151, 22–27. doi: 10.1016/j.dyepig.2017.12.041
Fu, Y., Pang, X. X., Wang, Z. Q., Chai, Q., and Ye, F. (2019). A highly sensitive and selective fluorescent probe for determination of Cu(II) and application in live cell imaging. Spectrochim. Acta Part A Mol. Biomol. Spectrosc. 208, 198-205. doi: 10.1016/j.saa.2018.10.005
Gupta, A., and Kumar, N. (2016). A review of mechanisms for fluorescent “turn-on” probes to detect Al3+ ions. RSC Adv. 6, 106413–106434. doi: 10.1039/C6RA23682K
He, L., Liu, C., and Xin, J. H. (2015). A novel turn-on colorimetric and fluorescent sensor for Fe3+ and Al3+ with solvent-dependent binding properties and its sequential response to carbonate. Sens. Actuators B Chem. 213, 181–187. doi: 10.1016/j.snb.2015.02.060
Helal, A., Kim, S. H., and Kim, H. S. (2013). A highly selective fluorescent turn-on probe for Al3+ via Al3+-promoted hydrolysis of ester. Tetrahedron 69, 6095–6099. doi: 10.1016/j.tet.2013.05.062
Jiang, Y., Sun, L. L., Ren, G. Z., Niu, X., Hu, W. Z., and Hu, Z. Q. (2015). A new fluorescence turn-on probe for aluminum (III) with high selectivity and sensitivity, and its application to bioimaging. Chemistryopen 4, 378–382. doi: 10.1002/open.201402169
Jo, T. G., Bok, K. H., Han, J., Lim, M. H., and Kim, C. (2017). Colorimetric detection of Fe3+ and Fe2+ and sequential fluorescent detection of Al3+ and pyrophosphate by an imidazole-based chemosensor in a near-perfect aqueous solution. Dyes Pigm. 139, 136–147. doi: 10.1016/j.dyepig.2016.11.052
Kang, L., Xing, Z. Y., Ma, X. Y., Liu, Y. T., and Zhang, Y. (2016). A highly selective colorimetric and fluorescent turn-on chemosensor for Al3+ based on naphthalimide derivative. Spectrochim. Acta Part A Mol. Biomol. Spectrosc. 167, 59–65. doi: 10.1016/j.saa.2016.05.030
Kang, Y., Zheng, X. J., and Jin, L. P. (2016). A microscale multi-functional metal-organic framework as a fluorescence chemosensor for Fe(III), Al(III) and 2-hydroxy-1-naphthaldehyde. J. Colloid Interf. Sci. 471, 1–6. doi: 10.1016/j.jcis.2016.03.008
Kaur, N., and Kaur, B. (2017). Spectral studies on anthracene based dual sensor for Hg2+ and Al3+ ions with two distinct output modes of detection. Spectrochim. Acta Part A Mol. Biomol. Spectrosc. 181, 60–64. doi: 10.1016/j.saa.2017.03.029
Kim, H., Kim, K. B., Song, E. J., Hwang, I. H., Noh, J. Y., Kim, P. G., et al. (2013). Turn-on selective fluorescent probe for trivalent cations. Inorg. Chem. Commun. 36, 72–76. doi: 10.1016/j.inoche.2013.08.025
Lei, K., Sun, M., Du, L., Zhang, X., Yu, H., Wang, S., et al. (2017). Alsaedi, Sensitive determination of endogenous hydroxyl radical in live cell by a BODIPY based fluorescent probe. Talanta 170, 314–321. doi: 10.1016/j.talanta.2017.04.004
Li, N. N., Zeng, S., Li, M. Q., Ma, Y. Q., Sun, X. J., Xing, Z. Y., et al. (2018). A highly selective naphthalimide-based chemosensor: “naked-eye” colorimetric and fluorescent turn-on recognition of Al3+ and its application in practical samples, test paper and logic gate. J. Fluoresc. 28, 347–357. doi: 10.1007/s10895-017-2197-9
Lim, C., An, M., Seo, H., Huh, J. H., Pandith, A., Helal, A., et al. (2017). Fluorescent probe for sequential recognition of Ga3+ and pyrophosphate nions. Sens. Actuators B Chem. 241, 789–799. doi: 10.1016/j.snb.2016.11.002
Lim, C., Seo, H., Choi, J. H., Kim, K. S., Helal, A., and Kim, H. S. (2018). Highly selective fluorescent probe for switch-on Al3+ detection and switch-off F− detection. J. Photochem. Photobiol. A Chem. 356, 312–320. doi: 10.1016/j.jphotochem.2018.01.012
Lin, Q. S., Huang, Y. L., Fan, X. X., Zheng, X. L., Chen, X. L., Zhan, X. Q., et al. (2017). A ratiometric fluorescent probe for hypochlorous acid determination: excitation and the dual-emission wavelengths at NIR region. Talanta 170, 496–501. doi: 10.1016/j.talanta.2017.04.024
Liu, S. D., Zhang, L. W., Zan, W. Y., Yao, X. J., Yang, Y., and Liu, X. (2014). A novel HBT-based Schiff base for colorimetric detection of aluminum: synthesis, characterization, spectral and DFT computational studies. Sens. Actuators B Chem. 192, 386–392. doi: 10.1016/j.snb.2013.10.134
Liu, Y. J., Tian, X. Y., Fan, F. L., Jiang, F. L., and Liu, Y. (2017). Fabrication of an acylhydrazone based fluorescence probe for Al3+. Sens. Actuators B chem. 240, 916–925. doi: 10.1016/j.snb.2016.09.051
Murugan, A. S., Pandi, M., and Annaraj, J. (2017). A single and simple receptor as a multifunctional chemosensor for the Al3+/Cu2+ ions and its live cell imaging applications. ChemistrySelect 2, 375–383. doi: 10.1002/slct.201601646
Qin, J. C., and Yang, Z. Y. (2015). Bis-Schiff base as a donor-acceptor fluorescent probe: recognition of Al3+ ions in near 100% aqueous solution. J. Photochem. Photobiol. A Chem. 303, 99–104. doi: 10.1016/j.jphotochem.2015.02.008
Rai, A., Kumari, N., Srivastava, A. K., Singh, S. K., Srikrishna, S., and Mishra, L. (2016). Rhodamine hydrazone as OFF–ON–OFF type selective sequential sensor of Al3+ and ions. J. Photochem. Photobiol. A Chem. 319, 78–86. doi: 10.1016/j.jphotochem.2016.01.003
Sarkar, D., Ghosh, P., Gharami, S., Mondal, T. K., and Murmu, N. (2017). A novel coumarin based molecular switch for the sequential detection of Al3+ and F−: application in lung cancer live cell imaging and construction of logic gate. Sens. Actuators B Chem. 242, 338–346. doi: 10.1016/j.snb.2016.11.059
Shen, S. L., Ning, J. Y., Zhang, X. F., Zhang, X. F., Miao, J. Y., and Zhao, B. X. (2017). Through-bond energy transfer-based ratiometric fluorescent probe for the imaging of HOC1 in living cells. Sens. Actuators B chem. 244, 907–913. doi: 10.1016/j.snb.2017.01.073
Shi, J., Li, Q. Q., Zhang, X., Peng, M., Qin, J. G., and Li, Z. (2010). Simple triphenylamine-based luminophore as a hypochlorite. Sens. Actuators B chem. 145, 583–587. doi: 10.1016/j.snb.2009.11.003
Simon, T., Shellaiah, M., Srinivasadesikan, V., Lin, C. C., Ko, F. H., and Sun, K. W. (2016). A simple pyrene based AIEE active schiff base probe for selective naked eye and fluoresence off-on detection of trivalent cations with live cell application. Sens. Actuators B Chem. 231, 18–29. doi: 10.1016/j.snb.2016.02.136
Tang, J. L., Li, C. Y., Li, Y. F., Lu, X., and Qi, H. R. (2015). A highly sensitive and selective fluorescent probe for trivalent aluminum ion based on rhodamine derivative in living cells. Anal. Chim. Acta 888, 155–161. doi: 10.1016/j.aca.2015.07.033
Urano, Y., Kamiya, M., Kanda, K., Ueno, T., Hirose, K., and Nagano, T. (2015). Evolution of fluorescein as a platform for finely tunable fluorescence probes. J. Am. Chem. Soc. 127, 4888–4894. doi: 10.1021/ja043919h
Wang, H. H., Wang, B., Shi, Z. H., Tang, X. L., Dou, W., Han, Q. X., et al. (2015). A two-photon probe for Al3+ in aqueous solution and its application in bioimaging. Biosens. Bioelectron. 65, 91–96. doi: 10.1016/j.bios.2014.10.018
Wang, L., Yang, L. L., and Cao, D. R. (2014). A visual and fluorometric probe for Al(III) and Fe(III) using diketopyrrolopyrrole-based Schiff base. Sens. Actuators B Chem. 202, 949–958. doi: 10.1016/j.snb.2014.06.052
Wen, X., and Fan, Z. (2016). Linear Schiff-base fluorescence probe with aggregation-induced emission characteristics for Al3+ detection and its application in live cell imaging. Anal. Chim. Acta 945, 75–84. doi: 10.1016/j.aca.2016.09.036
Wu, H. L., Peng, H. P., Wang, F., Zhang, H., Chen, C. G., Zhang, J. W., et al. (2017). Two 1,8-naphthalimides as proton-receptor fluorescent sensors for detecting pH. J. Appl. Spectrosc. 83, 931–937. doi: 10.1007/s10812-017-0386-6
Xie, H., Wu, Y. L., Huang, J., Zeng, F., Wu, H., Xia, X. T., et al. (2016). A ratiometric fluorescent probe for aluminum ions based-on monomer/excimer conversion and its applications to real samples. Talanta 151, 8–13. doi: 10.1016/j.talanta.2016.01.015
Xie, J. Y., Li, C. Y., Li, Y. F., Fu, Y. J., Nie, S. X., and Tan, H. Y. (2017). A near-infrared chemosensor for determination of trivalent aluminum ions in living cells and tissues. Dyes Pigm. 136, 817–824.doi: 10.1016/j.dyepig.2016.09.046
Ye, X. P., Sun, S. B., Li, Y. D., Zhi, L. H., Wu, W. N., and Wang, Y. (2014). A highly sensitive, single selective, fluorescent sensor for Al3+ detection and its application in living cell imagin. J. Lumin. 155, 180–184. doi: 10.1016/j.jlumin.2014.06.050
Yu, C. W., and Zhang, J. (2014). Copper (II)-responsive “off-on” chemosensor based on a naphthalimide derivative. Asian J. Org. Chem. 3, 1312–1316. doi: 10.1002/ajoc.201402160
Zeng, S., Li, S. H., Sun, X. J., Li, M. Q., Xing, Z. Y., and Li, J. L. (2019). A benzothiazole-based chemosensor for significant fluorescent turn-on and ratiometric detection of Al3+ and its application in cell imaging. Inorg. Chim. Acta 486, 654–662. doi: 10.1016/j.ica.2018.11.042
Zeng, S., Li, S. J., Sun, X. J., Li, M. Q., Ma, Y. Q., Xing, Z. Y., et al. (2018). A naphthalene-quinoline based chemosensor for fluorescent “turn-on” and absorbance-ratiometric detection of Al3+ and its application in cells imaging. Spectrochim. Acta Part A Mol. Biomol. Spectrosc. 205, 276–286. doi: 10.1016/j.saa.2018.07.039
Zhai, B., Li, Z. Y., Wu, Z. L., and Cui, J. Z. (2016). A novel europium metal-organic framework as luminescent probe for detecting Al3+. Inorg. Chem. Commun. 71, 23–26. doi: 10.1016/j.inoche.2016.06.031
Zhang, X. X., Wang, R. J., Liu, G., Fan, C. B., and Pu, S. Z. (2016). A highly selective fluorescence probe for Al3+ on based a new diarylethene with a 6-(hydroxymethyl) picolinohydrazide unit. Tetrahedron 72, 8449–8455. doi: 10.1016/j.tet.2016.11.007
Zhang, Y. R., Liu, Y., Feng, X., and Zhao, B. X. (2017). Recent progress in the development of fluorescent probes for the detection of hypochlorous acid. Sens. Actuators B Chem. 240, 18–36. doi: 10.1016/j.snb.2016.08.066
Zhao, B., Xu, Y., Deng, Q., Kan, W., Fang, Y., Wang, L. Y., et al. (2016). Modified 1H-phenanthro[9,10-d]imidazole derivative with the double acetohydrazide as fluorescent probe for sequential detection of Ni2+ and Al3+ with ‘on–off–on' response. Tetrahedron Lett. 57, 953–958. doi: 10.1016/j.tetlet.2016.01.065
Zhao, J. Y., Zhao, Y. K., Xu, S., Luo, N. Z., and Tang, R. R. (2015). A selective fluorescent probe for relay recognition of Al3+ and Cu2+ through fluorescence “off-on-off” functionality. Inorg. Chim. Acta 438, 105–111. doi: 10.1016/j.ica.2015.09.007
Zhu, B. C., Xu, Y. H., Liu, W. Q., Shao, C. X., Wu, H. F., Jiang, H. L., et al. (2014). A highly selective colorimetric probe for fast and sensitive detection of hypochlorite in absolute aqueous solution. Sens. Actuators B chem. 191, 473–478. doi: 10.1016/j.snb.2013.10.057
Keywords: chemosensor, naphthalimides, Al3+, ClO−, logic gate
Citation: Sun X-J, Liu T-T, Fu H, Li N-N, Xing Z-Y and Yang F (2019) A Naphthalimide-Based Fluorescence “Off-on-Off” Chemosensor for Relay Detection of Al3+ and ClO−. Front. Chem. 7:549. doi: 10.3389/fchem.2019.00549
Received: 10 April 2019; Accepted: 19 July 2019;
Published: 02 August 2019.
Edited by:
Shusheng Zhang, Linyi University, ChinaReviewed by:
Chun-Yan Li, College of Chemistry, Xiangtan University, ChinaQi Lin, Northwest Normal University, China
Copyright © 2019 Sun, Liu, Fu, Li, Xing and Yang. This is an open-access article distributed under the terms of the Creative Commons Attribution License (CC BY). The use, distribution or reproduction in other forums is permitted, provided the original author(s) and the copyright owner(s) are credited and that the original publication in this journal is cited, in accordance with accepted academic practice. No use, distribution or reproduction is permitted which does not comply with these terms.
*Correspondence: Zhi-Yong Xing, zyxing@neau.edu.cn