- 1Institute of Applied Sciences and Intelligent Systems, National Research Council (CNR-ISASI), Pozzuoli, Italy
- 2Department of Mathematics and Physics, University of Campania “L. Vanvitelli”, Caserta, Italy
- 3Institute of Food Sciences, National Research Council (CNR-ISA), Avellino, Italy
Localized electric fields have become, in recent years, a source of inspiration to researchers and laboratories thanks to a huge amount of applications derived from it, including positioning of microparticles as building blocks for electrical, optical, and magnetic devices. The possibility of producing polymeric materials with surface charge thus opens new perspectives for applications where process simplicity and cost-effectiveness of flexible electronics are of fundamental importance. In particular, the influence of surface charges is widely studied and is a critical issue especially when new materials and functional technologies are introduced. Here, we report a voltage-free pyro-electrification (PE) process able to induce a permanent dipole orientation into polymer sheets under both mono- and bipolar distribution. The technique makes use of the pyroelectric effect for generating electric potentials on the order of kilovolts by an easy-to-accomplish thermal treatment of ferroelectric lithium niobate (LN) crystals. The PE allows us to avoid the expensive and time-consuming fabrication of high-power electrical circuits, as occurs in traditional generator-based techniques. Since the technique is fully compatible with spin-coating-based procedures, the pyro-electrified polymer sheets are easily peeled off the surface of the LN crystal after PE completion, thus providing highly stable and freestanding charged sheets. We show the reliability of the technique for different polymers and for different applications ranging from live cell patterning to biofilm formation tests for bacteria linked to food-processing environments.
Introduction
The possibility of tailoring the material surface properties has allowed an increasingly active field for a wide variety of researches and applications ranging from materials science, nanotechnology, and electronics to biological and medical systems (Stuart et al., 2010). One possibility for functionalizing surfaces and thus tuning interfacial properties is to use specific chemical treatment or to design and induce the electrostatic charges in specific locations. In particular, materials that show net electrostatic charges have been the key issue for fruitful applications in electronics, mechanics, and biological systems. Supports with localized charges are widely used to control the behavior of thin-film electronic devices (Jacobs and Whitesides, 2001). Moreover, obtaining templates with a pattern of electrostatic charges becomes very useful in applications including sorting and self-assembling of micro- and nano-particles (Palleau et al., 2011; Zhao et al., 2011), macromolecules (Seemann et al., 2007; Zhao et al., 2011; Xi et al., 2012), or other building blocks (Cole et al., 2010). Recently, the programming and patterning of electric charges have been widely demonstrated by simple and effective processes in ferroelectric substrates (Grilli et al., 2008b; Esseling et al., 2013; Gennari et al., 2013; Carrascosa et al., 2015; Chen et al., 2016) devoting much attention to the possibility of using polymeric materials thanks to their ability to be economically produced on a large scale and with the further advantage of flexible thin-film technology. The techniques for charging polymeric surfaces are numerous and are quite well-established. The most common approaches require an external voltage source as in the case of contact poling (Hill et al., 1994) or corona poling (Rychkov et al., 2011). However, these techniques present severe limitations. In the case of contact poling, a large charge injection could produce a detrimental dielectric breakdown of the films (Hill et al., 1994; DeRose et al., 2006), while in the case of corona poling, the good homogeneity of the polarization is not guaranteed due to the difficult control of the high field intensity required. In addition, the poled films present often several surface damages due to various reactive and energy species, such as ozone or nitrogen oxides, that are produced by the corona discharge (Sprave et al., 1996).
Here, we propose a simple and voltage-free process based on pyro-electrification (PE), capable of producing polar orientation and 2D polar patterning in freestanding polymer sheets. Compared to conventional electrification techniques, the PE is electrode-free and is able to produce high voltages by a simple thermal treatment in ferroelectric crystals of lithium niobate (LN), thus simplifying the whole process significantly. Moreover, we can produce both homogeneous and bipolar charge distributions simply by using one-domain or homemade periodically poled LN crystals obtaining pyro-electrified polymer sheets with a net surface charge. Recently, we investigated the presence of such charge by analyzing the second harmonic signal and by observing the adhesion and spreading of eukaryotic cells on PE polymers that would be otherwise cytophobic surfaces (Rega et al., 2016a,b; Lettieri et al., 2017). Moreover, we evaluated in vitro the ability of bacteria to form biofilms very rapidly (Gennari et al., 2018).
It is recognized that the adhesion and proliferation of different types of cells on polymeric materials depend on surface characteristics and can be significantly influenced by the surface charge on that material (Robertus et al., 2010; Li et al., 2018; Liu et al., 2018; Ma et al., 2019).
Polystyrene (PS) and poly (methyl methacrylate) (PMMA) are materials typically used in labware equipment. They are cytophobic and require a chemical or physical treatment to promote cell adhesion. The most common treatments make use of sulfuric acid, or chromic acid, which can produce different kinds of functional groups on the surface, such as sulfonate, hydroxyl, or carboxyl. Another family of techniques makes use of protein adsorption, such as fibronectin, laniline, and Arginylglycylaspartic acid (RGD) peptide that chemically mimic the extracellular microenvironment and alter the structure of the surface. On the other hand, physical treatments able to activate the surface of traditional cytophobic surfaces by mild oxidation involve ultraviolet light or corona discharge stimulation. Regardless of the method, the cell adhesion promotion requires an electrostatically charged surface. All of these treatments are well-established but are laborious and expensive with additional drawbacks related to potentially polluting materials.
Here, we show the possibility of obtaining charged polymeric surfaces capable of interacting electrostatically with cell cultures under safe conditions by an easy and economical procedure, tracing the route as an alternative tool for all those applications in which the use of chemical agents or complicated physical treatments can be detrimental for both environment and cell cultures.
Materials and Methods
The ferroelectric LN is a rhombohedral crystal belonging to the point group 3 m that exhibits pyroelectricity at room temperature. The spontaneous polarization Ps changes according to ΔPiαpiΔT, where Pi is the coefficient of the polarization vector, pi is the pyroelectric coefficient, and ΔT is the temperature variation. At room temperature, the equilibrium condition makes the charge of the spontaneous polarization Ps to be balanced fully by the environmental screening charges, and no electric field exists (Grilli et al., 2008a). The temperature variation changes the polarization magnitude and perturbs this equilibrium, causing a lack or excess of surface screening charge (Bhowmick et al., 2017). As a consequence, an electrostatic state appears and generates a high-intensity electric field at the crystal surface (Bhowmick et al., 2017) with mono- or bipolar properties depending on the nature of the LN crystal, mono-domain in the first case and periodically poled (PPLN) in the second one. The PPLN crystals were homemade by standard electric field poling onto photoresist patterned samples (Detrait et al., 1998; Huang et al., 2012; Pagliarulo et al., 2018) and consisted of an array of ferroelectric domains with opposite polarization. The pyroelectric effect generates an array of surface charges with opposite sign, following the pattern of the reversed domains. Recently, we demonstrated for the first time the possibility of using the pyroelectric effect for a wide variety of applications ranging from biological to soft matter manipulation application (Ferraro et al., 2010; Mecozzi et al., 2016, 2017; Rega et al., 2019).
The PE exploits this electric field during an appropriate thermal treatment. The PE induces a permanent orientation of the dipoles when exceeding the glass transition temperature of the polymer, namely, when the dipole molecules can be easily oriented. This technique guarantees simplicity and cost-effectiveness since only a polymeric solution is needed, spin coated on an LN crystal and thermal treated on conventional hotplates. The fundamental role is played by the LN crystal since it supports, heats, and polarizes the polymer layer, which, at the end of the process, is easily removed, obtaining a freestanding charged sheet.
The Pyro-electrification
Figure 1 shows the schematic view of the procedure.
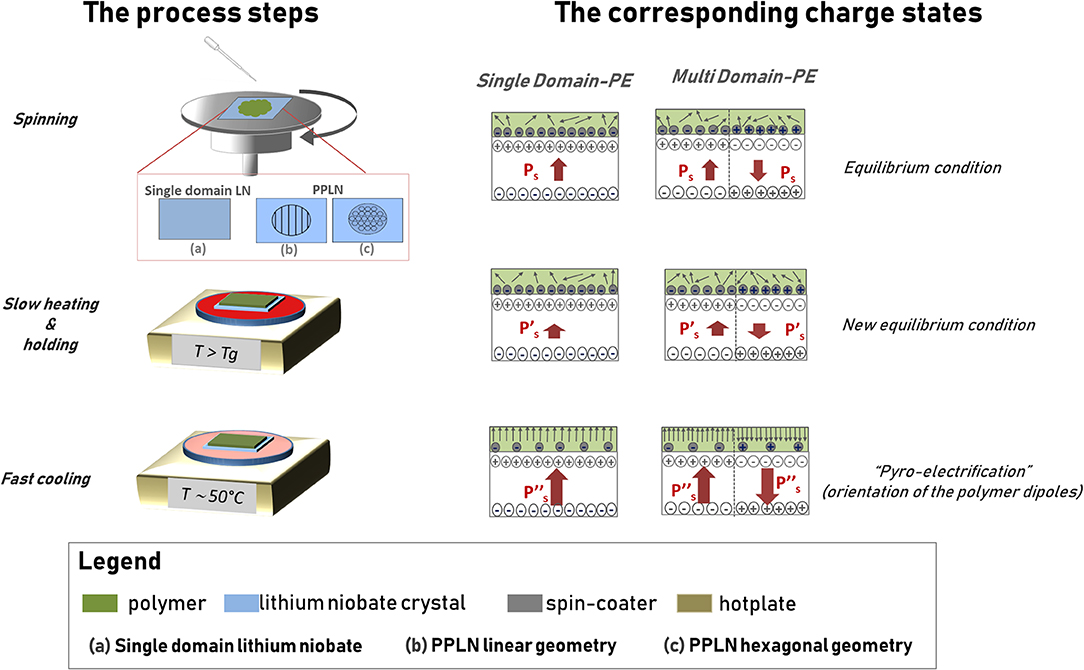
Figure 1. Schematic view of the pyro-electrification (PE) technique. The column on the left shows the view of the process steps, while the column on the right shows the view of the corresponding charge states in the polymer sheet and in the lithium niobate (LN) crystal.
The LN crystal sample is spin coated with a polymer solution at room temperature and then heated by a digitally controlled hotplate according to a thermal treatment based on three steps identified here by the following labels: slow heating (Stuart et al., 2010), holding (Jacobs and Whitesides, 2001), and fast cooling (Palleau et al., 2011). The slow heating induces a temperature rise from room temperature (Ti) up to the glass transition temperature of the polymer (Tf) at a rate of 1°C/min. The holding step keeps the sample at the final temperature Tf for 10 min. In the fast cooling step, the polymer-coated crystal is moved to a second hotplate set at a lower temperature Tl ~50°C. The slow rate of temperature increase in the first step and the holding state are of crucial importance for keeping the electrostatic equilibrium between the slight polarization change of the LN and the screening charges on the surface, prior to reaching the glass transition of the polymer. Once this state is reached, the polymer becomes very amorphous and the dipole molecules can orient easily under the action of the strong electric field generated onto the surface of the crystal by the pyroelectric effect. The monotonic cooling step is necessary to provide large ΔT that generates a steady and strong electric field capable of poling the polymer and, simultaneously, freezing the poled state into the polymer itself. The poled polymer layer can be peeled off from the surface of the crystal proving a freestanding sheet with the same polarization orientation of the LN crystal that drove the PE process.
The technique can be performed under two different configurations and with different polymer solutions. The single-domain PE (SD-PE) uses a single domain crystal, while the multi-domain PE (MD-PE) makes use of a periodically poled crystal PPLN with different geometries. In the case of the SD-PE configuration, the polymer layer exhibits a polarization charge with single orientation, while in the case of the MD-PE configuration, the polymer shows the pattern of domains with reversed polarization according to the ferroelectric domain pattern of the driving PPLN crystal. The driving crystal can be re-used indefinitely after appropriate solvent cleaning.
The Polymer Solutions
Solid-state polymers were used as received, without further purification (Sigma-Aldrich, Milan, Italy). Polysulfone (PSU) transparent pellets (Mw 35,000) were dissolved at 80% w/w in anisole and stirred at 70°C for 3 h. PS powder (Mw 350,000) was dissolved at 60% w/w in anisole and stirred at 70°C for 6 h. PMMA (Mw 996,000) was dissolved at 15% w/w in anisole and stirred at 70°C for 3 h. The resulting polymer solutions of PSU, PS, and PMMA were stored at 4°C.
The Periodically Poled LN Crystals
The LN crystals were bought from Crystal Technology Inc., Palo Alto, California, in the form of both sides polished into 500 μm-thick c-cut 3-inch. wafers and were cut into square samples (2 × 2 cm2) by a standard diamond saw. The PPLNs were obtained by standard electric field poling onto photoresist-patterned samples (Huang et al., 2012; Bhowmick et al., 2017; Pagliarulo et al., 2018). Two geometries were considered: linear (period, 200 μm) and square array of hexagons (period, 200 μm).
The Bare Sheets (Control)
The freestanding PSU, PS, and PMMA bare sheets were obtained by spin coating a 2 × 2 cm2 sized glass coverslip at 4,000 RPM for 2 min with the polymer solution and by peeling off the slide accurately just after solvent evaporation.
The SH-SY5Y Human Neuroblastoma and the NIH-3T3 Mouse Embryonic Fibroblast Cells
The SH-SY5Y and NIH-3T3, cells lines were purchased from European Collection of Authenticated Cell Cultures (ECACC) (Sigma-Aldrich, Milan, Italy). They were routinely grown in Dulbecco's modified Eagle's medium (DMEM) containing 4.5 g/L D-glucose and supplemented with 2 mM L-glutamine, penicillin (100 units/ml), and streptomycin (100 μg/ml), and containing 20% (v/v) fetal bovine serum (FBS) (GIBCO, Gaithersburg, MD, USA). For the cell culture experiments, the SH-SY5Y and NIH-3T3 were detached by means of Trypsin/Ethylenediaminetetraacetic acid dipotassium salt dihydrate (EDTA) solution (Sigma, Milan, Italy), resuspended in DMEM−20% FBS, seeded at a concentration of 1.0 × 105 cells/ml on the MD-PE sheets (immersed in DMEM medium at 37°C for 1 h prior to use), and then incubated into conventional 30 mm-diameter Petri dishes at 37°C and in a saturated humidity atmosphere containing 95% air and 5% CO2. Cells were allowed to grow in DMEM−20% FBS on different substrates for 24 h. Cell adhesion and spreading were observed over 24 h under a standard inverted optical microscope (AxioVert, Carl Zeiss, Jena, Germany).
Immunofluorescence
The cells were cultured 24 h on the surface of interest and then fixed by standard procedures. The cells were then stained by Alexa Fluor 488 phalloidin and by blue fluorescent Hoechst 33,342 dye and trihydrochloride trihydrate (Molecular Probes Invitrogen) for visualizing nuclei and actin filaments.
Bacterial Strain and Culture Conditions
In the present study, we used the Gram-positive Listeria innocua (strain DSMZ 20649) provided by the Deutsche Sammlung von Mikroorganismen und Zellkulturen, Braunschweig, Germany. The bacteria strains were plated and incubated on Luria-Bertani (LB) agar plates (10 g/l NaCl, 10 g/l tryptone, 5 g/l yeast extract, and 15 g/l agar, Thermo Fisher Scientific). One day before the experiments, a single bacterial colony was picked up and cultured in LB broth medium at 37°C in a shaker incubator at 225 RPM for 16–18 h to achieve saturation conditions. A 1:5 volumetric dilution of the cell culture was then grown in LB until reaching the log phase. Then, the growth was stopped and bacteria were harvested by centrifugation at 7.200 g (Beckman Coulter tj-25 centrifuge, California, USA) for 10 min in order to separate the cells from the medium. Sterilized LB broth was measured (3 ml) into sterile tubes. The bacteria concentration was evaluated by the spectrophotometric measurement (Bio-Rad SmartSpecTM Plus Spectrophotometer, California, USA) of the suspension absorbance at 600 nm (optical density at 600 nm, i.e., OD600), considering that 8 × 108 cells/ml have an OD600 = 1.
Microtiter-Plate Test
In order to quantify the biofilm formation, we use the crystal violet assay (Stepanović et al., 2000). This technique involves fixing the bacterial film with methanol, staining with crystal violet, releasing the bound dye with 33% glacial acetic acid, and measuring the optical density (OD) of the solution at 600 nm by spectrophotometric measurement (Bio-Rad SmartSpecTM Plus Spectrophotometer, CA, USA).
The Viability Test
The viability of the bacterial strain (L. innocua) was tested through the live/dead viability/cytotoxicity assay kit (Live/Dead BacLight bacterial viability kit, Thermo Fisher Scientific, Waltham, MA, USA). The easy-to-use live/dead kit is utilized for monitoring the viability of the bacterial populations as a function of the sheet integrity of the cell. The live/dead BacLight Bacterial Viability Kits utilize mixtures of our SYTO® 9 green-fluorescent nucleic acid stain and the red fluorescent nucleic acid stain, propidium iodide. These stains differ both in their spectral characteristics and in their ability to penetrate healthy bacterial cells. Cells with a compromised sheet that are considered to be dead or dying will stain red, whereas cells with an intact sheet will stain green. The cells were incubated on each substrate for 24 h. After incubation, each substrate was immersed in 8 μl of 1,000-fold diluted live/dead kit solution and was incubated for 15 min in the dark. The fluorescence micrographs were acquired by an inverted laser scanning confocal microscope (Zeiss LSM 700, Germany), equipped with a 20 × objective.
Results and Discussion
Two PMMA sheets were subjected to PE by using a PPLN crystal (see Figure 1 for the multi-domain procedure). A faster test to verify the presence of the charges on the PE sheet is the decoration technique. The polymer solution was spun on one face of the crystal and, in the second experiment, on the other face of the PPLN. As a consequence, we produced two freestanding PS sheets with a bipolar polarization pattern following the pattern of reversed domains of the PPLN. The hexagonal regions of the PS sheets exhibited positive and negative polarity, respectively. Figures 2A,B shows the typical optical microscope images of these two PS sheets after gentle sprinkling of toner dust on the surface.
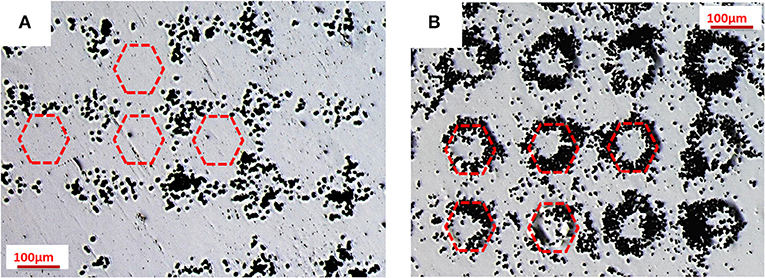
Figure 2. Optical microscope images of decoration technique: toner distribution on two polystyrene (PS) sheets after pyro-electrification (PE) by a periodically poled lithium niobate (PPLN) crystal, where the hexagons exhibit (A) positive and (B) negative charge polarity. The hexagons are indicated by the schematic drawings with the red dotted line.
These images show clearly that the particles of toner, bringing a negative charge, are attracted electrostatically by the hexagonal regions (positive charge) in the first case (see Figure 2A) and by the surrounding regions (positive charge) in the second case (see Figure 2B), thus demonstrating the non-negligible electric field generated on the surface of the pyro-electrified PS sheets.
We demonstrate here the possibility of using the permanent electric field on the PE sheet for guiding live cell adhesion in vitro. PS and PMMA labware equipment have been used for cell culture since about 1965. Many cell types adhere to and move on the surfaces of such materials and present a morphology that is very similar to that seen when the cells are grown on glass. However, it has long been known that these materials must be subjected to a surface treatment to render their surface suitable for cell attachment. Bare PS and PMMA are unsuitable for cell attachment, meaning that cells seeded and incubated onto surfaces made of such polymers cannot find adhesion cues and remain suspended. This has been attributed to the surface chemistry of the materials, and many different specific surface treatments have been made to change the chemistry involved in the non-adhesive nature of these polymers (Klemperer and Knox, 1977; Maroudas, 1977; Grinnell, 1978; Lee et al., 1994; Buttiglione et al., 2007).
Here, we demonstrate the cytophilic capability of PE polymer for cultured NIH-3T3 cells (see Materials and Methods for details) on PS and PMMA sheets pyro-electrified on a single domain crystal. Figure 3 shows the optical microscope images of the sheets after 24 h incubation with NIH-3T3 cells. In particular, the image in Figures 3a,b show the cytophobic behavior of bare PS and PMMA polymer spotted on a glass coverslip where it is clearly seen that the cells are not able to adhere and spread. These materials are often treated in a chemical or physical way in order to permit cell adhesion and spreading. Figures 3c–e show the adhesion of NIH-3T3 cells on a conventional PS Petri dish (generally treated with UV light), PS coated with fibronectin protein, and PMMA coated with fibronectin protein, respectively. Figures 3f,g show the NIH-3T3 cells grown on the pyro-electrified PS and PMMA, respectively.
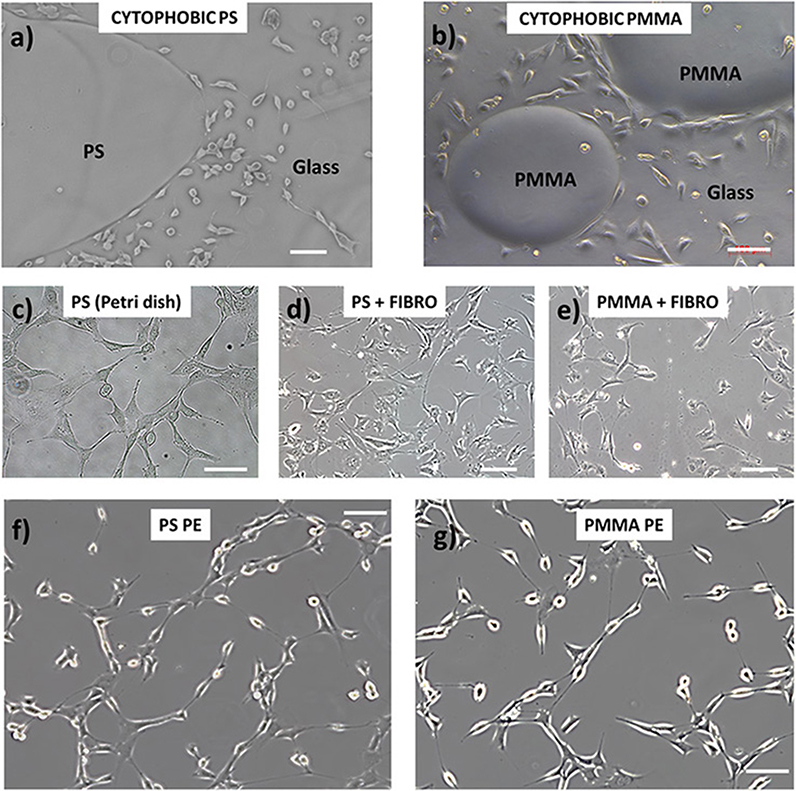
Figure 3. NIH-3T3 cell adhesion behavior on (a,b) bare PS and poly(methyl methacrylate) (PMMA) polymer (respectively) spotted on a glass coverslip where it shows the cytophobic behavior of the materials; (c–e) conventional PS Petri dish (generally treated with UV light), PS coated with fibronectin protein, and PMMA coated with fibronectin protein, respectively; (f,g) NIH-3T3 cells grown on the pyro-electrified PS and PMMA, respectively. Scale bar, 100 μm.
On substrates treated with fibronectin, the cellular conformation and their spatial distribution are characterized by a more compact organization and a more spread cellular shape, indicating a stronger cell–cell interaction than the interaction with the adhesion surface. The cellular distribution on the pyro-electrified sheets shows, on the other hand, an elongated shape of the nuclei and cellular filopodes, typical of cells polarized along one preferential direction. This characteristic is an indication of a strong interaction with the contact surface, which is predominant with respect to the cell–cell interaction.
Moreover, as already shown in our previous paper (Rega et al., 2016a,b), it is possible to have a cell pattern configuration when seeded on sheets pyro-electrified by a multi-domain crystal with a bipolar configuration of the charge distribution on the sheet surface. Here, we demonstrate the reliability of the technique with other periods and different concentrations of the polymer.
Figure 4A shows that SH-SY5Y cells adhered selectively on the positive region of PS sheet with bipolar domains having linear geometry with a 200 μm period, while Figure 4B corresponds to a PMMA (15% in anisole w/w) sheet, with bipolar domains having two-dimensional distributions at a 200 μm period. Moreover, in order to better elucidate the material–cytoskeleton cross-talk during adhesion, we fixed the cells after 24 h incubation on PS sheets pyro-electrified by PPLN crystals with hexagonal domains, and we performed immunofluorescence reactions (Figure 4C) (see Materials and Methods for details). The cells appear clearly to adhere selectively on the regions with positive polarity in the case of linear domains as well as in the case of two-dimensional domains. In fact, the cells bring a negative net charge on the external sheet (Ohgaki et al., 2001) and, as a consequence, are attracted electrostatically by the regions of the sheet exposing a net positive polarization charge.
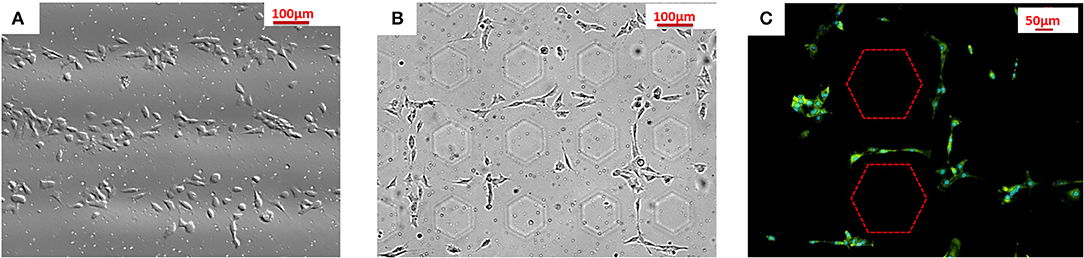
Figure 4. SH-SY5Y cell adhesion behavior on (A) PS sheet pyro-electrified linearly at 200 μm; (B) cell patterning on PMMA (15% in anisole w/w) sheet pyro-electrified by an array of hexagons at 200 μm; (C) immunofluorescence images of cells cultured after 24 h incubation on PS sheets pyro-electrified by an array of hexagons at 200 μm. The dashed lines correspond to the boundaries between regions with opposite polarities.
The PE allows us to achieve cell patterning results through a cation-free method, avoiding time-consuming and expensive lithographic-based procedures and moreover directly onto freestanding, and cheap polymer sheets. The relatively easy-to-accomplish procedure, which makes use simply of LN crystals and conventional hotplates, would be easily implementable in biology laboratories for routine cell biology experiments where selective cell adhesion configurations are of crucial importance. For example, in the case of electric-field-sensitive cells, we envisage the possibility of using PE for developing pre-defined neuronal networks for deep studies on their physiology and morphology behavior.
Recently, we demonstrated the possibility of using pyro-electrified sheets and fibers for rapid and reliable “biofilm electrostatic test” (Gennari et al., 2018) of Escherichia coli and Staphylococcus epidermidis, with potential applications in the field of biomedicine. In fact, the rapid biofilm formation promoted by our sheets would have a significant impact on health service when a fast response to an antibiogram test can save lives. Here, we show how the pyro-electrified sheets promote a rapid biofilm formation also in the case of L. innocua, a non-pathogenic species closely related to L. monocytogenes (Scifò et al., 2009; Tajkarimi et al., 2016; Jeon et al., 2018; Sheng et al., 2018). We use an SD-PE sheet as a tool for simple, rapid, and cost-effective evaluation of biofilm formation, through the electrostatic interaction of planktonic bacteria with a pyro-electrified carrier (Asadishad et al., 2011; Gennari et al., 2018). The fact that biofilms have a multidisciplinary impact that includes environmental, industrial, and clinical characteristics, and that over 60% of all human bacterial infections and up to 80% of all chronic infections are related to bacterial biofilms, is of fundamental importance in assessing how different environmental factors may affect the bacterial vitality. The cellular mechanisms underlying microbial biofilm formation and behavior are beginning to be understood and are targets for novel specific intervention strategies to control bacteria colonies formation in different fields and in particular for the food-processing environment (Dickson and Koohmaraie, 1989; Sahm et al., 1994; Linke and Goldman, 2011; Bianco et al., 2017; Bruslind, 2017; Mandracchia et al., 2019).
Here, we demonstrate that SD-PE carrier provides a polarization field able to immobilize the L. innocua bacteria and test their ability to form live biofilms within 2 h, avoiding time-consuming and laborious incubations and/or intermediate chemical treatments. PSU sheets (about 100 μm thickness and 2 × 2 cm2 sized) were produced: (Stuart et al., 2010) a bare sheet that represents the control and (Jacobs and Whitesides, 2001) polysulfone pyro-electrified (PSU-PE), where the positive side was in contact with the bacterial suspension. These sheets were incubated at different times (2 and 4 h) at 37°C in two different Petri dishes (35 mm) covered with 1 ml of L. innocua bacterial suspension (Gram-positive) and 2 ml of phosphate-buffered saline (PBS). The control and the SD-PE sheets were observed under an optical microscope, and Figure 5 shows the corresponding typical images.
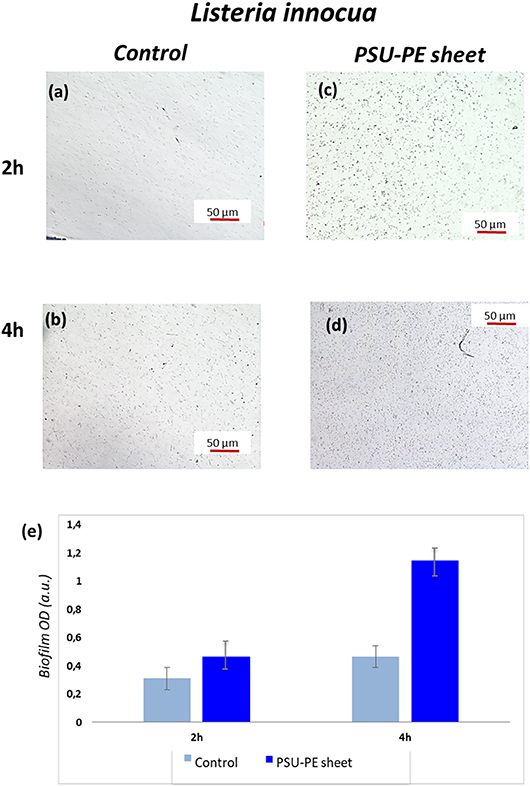
Figure 5. (a,b) Optical microscopy images of L. innocua bacterial adhesion at two different time points on control and (c,d) on the polysulfone pyro-electrified (PSU-PE) sheet; (e) histogram of bacteria biofilm OD (optical density) on the control and the PSU-PE sheet at two different time points.
These microscope images show the immobilized and biofilm forming bacteria on the control and PSU-PE sheet at different time intervals. The number of adhesion bacteria on the PSU-PE sheet appeared clearly higher than that on the control at each observation time. These results are confirmed by the quantitative evaluation of biofilm formation obtained by the microtiter-plate test (see Materials and Methods for details). The diagram in Figure 5e shows results averaged over five measures of biofilm OD (optical density) formation and shows evidence of the larger population of bacteria on the PE sheet.
To verify the viability of the biofilms, the PSU-PE sheet was mounted onto a glass slide in a well after 24 h incubation with planktonic bacteria in order to perform the reaction with the live/dead staining kit (see Material and Methods for details). After 24 h incubation, we found that the viability of the high-density biofilms formed onto the PSU-PE sheet was clearly evident (Figure 6). The PSU-PE sheet immobilized planktonic bacteria more rapidly than the control and favored biofilm formation without damaging the bacterial cytomembrane even after 24 h incubation, thus demonstrating biocompatiblity.
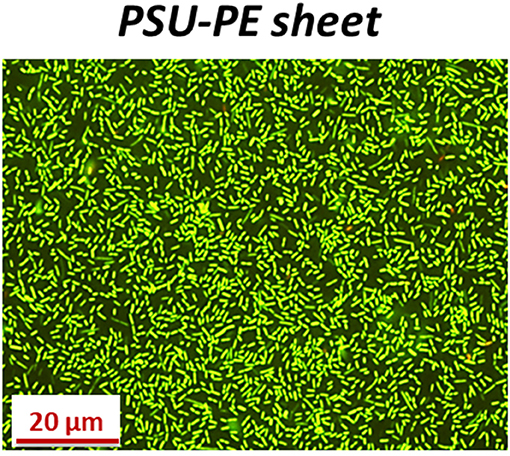
Figure 6. Fluorescence microscopy images of L. innocua forming biofilms onto the PSU-PE sheet after 24 h incubation and live/dead staining.
On the contrary, chemical coatings could immobilize planktonic bacteria, but could even destroy the bacterial cytomembrane causing their death. Cationic surfaces are obtained usually by the covalent attachment of different chemical compounds, such as quaternary ammonium organo-silanes (Isquith et al., 1972; Gottenbos et al., 2002; Li et al., 2006), antimicrobial peptides (Gabriel et al., 2006; Gao et al., 2011), polyethylenimines (PEI) (Lin et al., 2003), and many others. The mechanism of interaction, as already explained in Gennari et al. (2018), is schematized in Figure 7. The PE process provides a sheet (in this case, the PSU-PE sheet) with δ+ charge due to the permanent dipole generated during the process. Those charges attract the negative net charge onto the bacterial cytomembrane (COO− groups), thus leading to bacteria immobilization and promoting the biofilm formation. In contrast, the cationic groups NH3+, generally formed on the chemically treated surface, form an electrostatic bond with the COO− groups onto the cytomembrane of bacteria adhered, thus displacing the divalent cations forming the lipopolysaccharide network. This causes the disruption of the cytomembrane and then microorganism death.
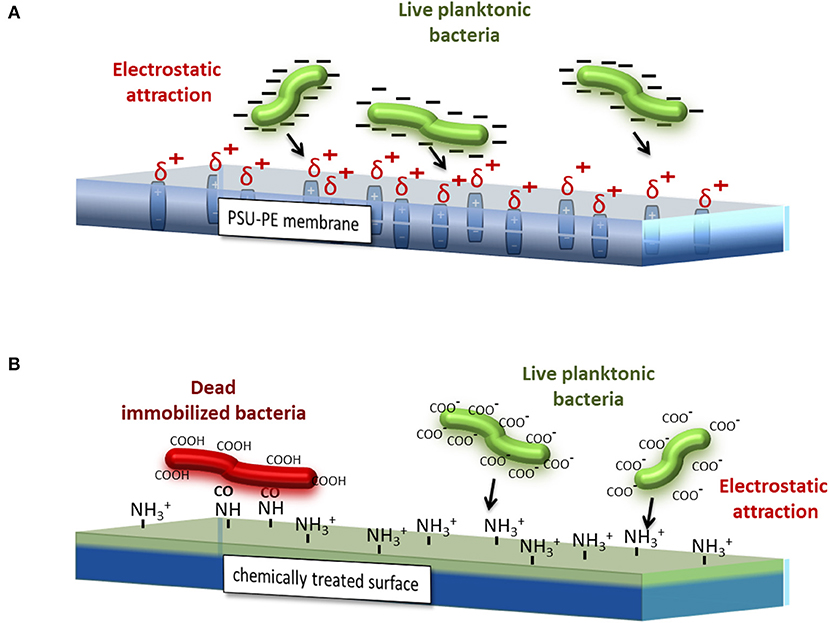
Figure 7. Simplified schematic views of the different interaction mechanisms between bacteria, the cytomembrane, and (A) the PSU-PE sheet and (B) a generic chemical-coated sheet.
The PE of freestanding polymer sheets could find applications in the food processing field as a quality check tool. The ultimate goal is the development of a rapid and easy PE assay for a quantitative analysis of a food sample, enabling food technologists to detect the contaminant agents in fresh products that represent a serious risk for all the consumers. In fact, it would be desirable having a compact and efficient system that could be used, for example, in an industry outside the laboratory. However, to develop and to design industrial applications for food processing, more data on the interaction between the process and the target organism are required. PE assay approaches will help to close this gap in the future, because they have the potential to be used as a method to control bacteria contamination and to ensure fresh food safety.
Conclusion
Here, we show an innovative PE technique capable of inducing a permanent dipole charge into freestanding polymer sheets by exploiting the pyroelectric properties of LN crystals, under both single- and multi-domain configurations. The resulting sheets can correspondingly have a mono- and bipolar charge with a magnitude able to selectively promote the adhesion of both eukaryotic and prokaryotic cells. The innovative use of the pyroelectric effect allows us to avoid expensive and time-consuming fabrication of electrodes and high-voltage circuits, since the appropriate thermal treatment of LN generates electrical potentials on the order of kilovolts. The same LN crystal can be used indefinitely for different PE cycles. The technique is free from lithography-based procedures and free from the use of cation-based chemicals, eventually detrimental for specific cell sheet structures. We show how these sheets can be used for cell patterning as well as for rapid biofilm formation. The relatively easy implementation makes PE implementable in a biology laboratory, opening the route to a novel generation of platforms for manipulating cell adhesion in vitro through non-invasive polarization charges, with the additional advantage of being flexible, freestanding, and cheap.
Author Contributions
RR planned, conducted experiments and wrote the manuscript. OG, MM, FN and EO prepared the biological cultures. VP made the PPNL samples. PF and SG supervised the project. All authors discussed the results and contributed to the manuscript.
Funding
The authors acknowledge the EU funding within the Horizon 2020 Program, under the FET-OPEN Project SensApp, Grant Agreement No. 829104.
Conflict of Interest Statement
The authors declare that the research was conducted in the absence of any commercial or financial relationships that could be construed as a potential conflict of interest.
References
Asadishad, B., Ghoshal, S., and Tufenkji, N. (2011). Method for the direct observation and quantification of survival of bacteria attached to negatively or positively charged surfaces in an aqueous medium. Environ. Sci. Technol. 45, 8345–8351. doi: 10.1021/es201496q
Bhowmick, S., Iodice, M., Gioffrè, M., Breglio, G., Irace, A., Riccio, M., et al. (2017). Investigation of pyroelectric fields generated by lithium niobate crystals through integrated microheaters. Sens. Actuators A Phys. 261, 140–150. doi: 10.1016/j.sna.2017.05.010
Bianco, V., Mandracchia, B., Nazzaro, F., Marchesano, V., Gennari, O., Paturzo, M., et al. (2017). “Food quality inspection by speckle decorrelation properties of bacteria colonies,” in Optical Methods for Inspection, Characterization, and Imaging of Biomaterials III (San Francisco, CA).
Buttiglione, M., Vitiello, F., Sardella, E., Petrone, L., Nardulli, M., Favia, P., et al. (2007). Behaviour of SH-SY5Y neuroblastoma cell line grown in different media and on different chemically modified substrates. Biomaterials 28, 2932–2945. doi: 10.1016/j.biomaterials.2007.02.022
Carrascosa, M., García-Cabañes, A., Jubera, M., Ramiro, J. B., and Agulló-López, F. (2015). LiNbO3: a photovoltaic substrate for massive parallel manipulation and patterning of nano-objects. Appl. Phys. Rev. 2:040605. doi: 10.1063/1.4929374
Chen, L., Li, S., Fan, B., Yan, W., Wang, D., Shi, L., et al. (2016). Dielectrophoretic behaviours of microdroplet sandwiched between LN substrates. Sci. Rep. 6:29166. doi: 10.1038/srep29166
Cole, J. J., Barry, C. R., Wang, X., and Jacobs, H. O. (2010). Nanocontact electrification through forced delamination of dielectric interfaces. ACS Nano 4, 7492–7498. doi: 10.1021/nn1016692
DeRose, C. T., Enami, Y., Loychik, C., Norwood, R. A., Mathine, D., Fallahi, M., et al. (2006). Pockel's coefficient enhancement of poled electro-optic polymers with a hybrid organic–inorganic sol-gel cladding layer. Appl. Phys. Lett. 89:131102. doi: 10.1063/1.2357157
Detrait, E., Lhoest, J. B., Knoops, B., Bertrand, P., and de Aguilar, P. V. D. B. (1998). Orientation of cell adhesion and growth on patterned heterogeneous polystyrene surface. J. Neurosci. Methods 84, 193–204. doi: 10.1016/S0165-0270(98)00114-9
Dickson, J. S., and Koohmaraie, M. (1989). Cell surface charge characteristics and their relationships to bacterial attachment to meat surfaces. App. Environ. Microbiol. 55, 832–836.
Esseling, M., Zaltron, A., Sada, C., and Denz, C. (2013). Charge sensor and particle trap based on z-cut lithium niobate. Appl. Phys. Lett. 103:061115. doi: 10.1063/1.4818143
Ferraro, P., Coppola, S., Grilli, S., Paturzo, M., and Vespini, V. (2010). Dispensingnano–pico droplets and liquid patterning by pyroelectrodynamic shooting. Nat. Nanotechnol. 5, 429–435. doi: 10.1038/nnano.2010.82
Gabriel, M., Nazmi, K., Veerman, E. C., Nieuw Amerongen, A. V., and Zentner, A. (2006). Preparation of LL-37-grafted titanium surfaces with bactericidal activity. Bioconjug. Chem. 17, 548–550. doi: 10.1021/bc050091v
Gao, G., Lange, D., Hilpert, K., Kindrachuk, J., Zou, Y., Cheng, J. T. J., et al. (2011). The biocompatibility and biofilm resistance of implant coatings based on hydrophilic polymer brushes conjugated with antimicrobial peptides. Biomaterials 32, 3899–3909. doi: 10.1016/j.biomaterials.2011.02.013
Gennari, O., Grilli, S., Coppola, S., Pagliarulo, V., Vespini, V., Coppola, G., et al. (2013). Spontaneous assembly of carbon-based chains in polymer matrixes through surface charge templates. Langmuir 29, 15503–15510. doi: 10.1021/la403603d
Gennari, O., Marchesano, V., Rega, R., Mecozzi, L., Nazzaro, F., Fratianni, F., et al. (2018). Pyroelectric effect enables simple and rapid evaluation of biofilm formation. ACS Appl. Mater. Interfaces 10, 15467–15476. doi: 10.1021/acsami.8b02815
Gottenbos, B., van der Mei, H. C., Klatter, F., Nieuwenhuis, P., and Busscher, H. J. (2002). In vitro and in vivo antimicrobial activity of covalently coupled quaternary ammonium silane coatings on silicone rubber. Biomaterials 23, 1417–1423. doi: 10.1016/S0142-9612(01)00263-0
Grilli, S., Paturzo, M., Miccio, L., and Ferraro, P. (2008a). In situ investigation of periodic poling in congruent LiNbO3 by quantitative interference microscopy Meas. Sci.Technol. 19:074008. doi: 10.1088/0957-0233/19/7/074008
Grilli, S., Vespini, V., and Ferraro, P. (2008b). Surface-charge lithography for direct PDMS micro-patterning. Langmuir 24, 13262–13265. doi: 10.1021/la803046j
Grinnell, F. (1978). “Cellular adhesiveness and extracellular substrata,” in International Review of Cytology, Vol. 53, eds G. H. Bourne, J. F. Danelli, and K. W. Jeon (San Francisco, CA: Academic Press, 65–144.
Hill, R. A., Knoesen, A., and Mortazavi, M. A. (1994). Corona poling of nonlinear polymer thin films for electro-optic modulators. Appl. Phys. Lett. 65, 1733–1735. doi: 10.1063/1.112899
Huang, S., Luo, J., Yip, H. L., Ayazi, A., Zhou, X. H., and Jen, A. K. Y. (2012). Efficient poling of electro-optic polymers in thin films and silicon slot waveguides by detachable pyroelectric crystals. Adv. Mater. 24:42. doi: 10.1002/adma.201102874
Isquith, A. J., Abbott, E. A., and Walters, P. A. (1972). Surface-bonded antimicrobial activity of an organosilicon quaternary ammonium chloride. Appl. Microbiol. 24, 859–863.
Jacobs, H. O., and Whitesides, G. M. (2001). Submicrometer patterning of charge in thin-film electrets. Science 291, 1763–1766. doi: 10.1126/science.1057061
Jeon, H. R., Kwon, M. J., and Yoon, K. S. (2018). Control of Listeria innocua biofilms on food contact surfaces with slightly acidic electrolyzed water and the risk of biofilm cells transfer to duck meat. J. Food Prot. 81, 582–592. doi: 10.4315/0362-028X.JFP-17-373
Klemperer, H. G., and Knox, P. (1977). Attachment and growth of BHK and liver cens on polystyrene: effect of surface groups introduced by treatment with chromic acid. Lab. Pratt. 26, 179–180.
Lee, J. H., Jung, H. W., Kang, I. K., and Lee, H. B. (1994). Cell behaviour on polymer surfaces with different functional groups. Biomaterials 15, 705–711. doi: 10.1016/0142-9612(94)90169-4
Lettieri, S., Rega, R., Pallotti, D. K., Gennari, O., Mecozzi, L., Maddalena, P., et al. (2017). Direct evidence of polar ordering and investigation on cytophilic properties of pyroelectrified polymer films by optical second harmonic generation analysis. Macromolecules 50, 7666–7671. doi: 10.1021/acs.macromol.7b00794
Li, W., Yan, Z., Ren, J., and Qu, X. (2018). Manipulating cell fate: dynamic control of cell behaviors on functional platforms. Chem. Soc. Rev. 47, 8639–8684. doi: 10.1039/C8CS00053K
Li, Z., Lee, D., Sheng, X., Cohen, R. E., and Rubner, M. F. (2006). Two-level antibacterial coating with both release-killing and contact-killing capabilities. Langmuir 22, 9820–9823. doi: 10.1021/la0622166
Lin, J., Qiu, S., Lewis, K., and Klibanov, A. M. (2003). Mechanism of bactericidal and fungicidal activities of textiles covalently modified with alkylated polyethylenimine. Biotechnol. Bioeng. 83, 168–172. doi: 10.1002/bit.10651
Liu, L., Tian, X., Ma, Y., Duan, Y., Zhao, X., and Pan, G. (2018). A versatile dynamic mussel-inspired biointerface: from specific cell behavior modulation to selective cell isolation. Angew. Chem. Int. Ed. 57, 7878–7882. doi: 10.1002/anie.201804802
Ma, Y., Tian, X., Liu, L., Pan, J., and Pan, G. (2019). Dynamic synthetic biointerfaces: from reversible chemical interactions to tunable biological effects. Acc. Chem. Res. doi: 10.1021/acs.accounts.8b00604. [Epub ahead of print]
Mandracchia, B., Palpacuer, J., Nazzaro, F., Bianco, V., Rega, R., Ferraro, P., et al. (2019). Biospeckle decorrelation quantifies the performance of alginate-encapsulated probiotic bacteria. IEEE J. Sel. To. Quantum Electron. 25, 1–6. doi: 10.1109/JSTQE.2018.2836941
Maroudas, N. G. (1977). Sulphonated polystyrene as an optimal substratum for the adhesion and spreading of mesenchymal cells in monovalent and divalent saline solutions. J. Cell. Physiol. 90, 511–519. doi: 10.1002/jcp.1040900314
Mecozzi, L., Gennari, O., Rega, R., Battista, L., Ferraro, P., and Grilli, S. (2017). Simple and rapid bioink jet printing for multiscale cell adhesion islands. Macromol. Biosci. 17:1600307. doi: 10.1002/mabi.201600307
Mecozzi, L., Gennari, O., Rega, R., Grilli, S., Bhowmick, S., Gioffrè, M. A., et al. (2016). Spiral formation at the microscale by μ-pyro-electrospinning. Soft Matter 12, 5542–5550. doi: 10.1039/C6SM00156D
Ohgaki, M., Kizuki, T., Katsura, M., and Yamashita, K. (2001). Manipulation of selective cell adhesion and growth by surface charges of electrically polarized hydroxyapatite. J. Biomed. Mater. Res A 57, 366–373. doi: 10.1002/1097-4636(20011205)57:3<366::AID-JBM1179>3.0.CO;2-X
Pagliarulo, V., Gennari, O., Rega, R., Mecozzi, L., Grilli, S., and Ferraro, P. (2018). Twice electric field poling for engineering multiperiodic Hex-PPLN microstructures. Opt. Lasers Eng. 104, 48–52. doi: 10.1016/j.optlaseng.2017.08.015
Palleau, E., Sangeetha, N. M., Viau, G., Marty, J. D., and Ressier, L. (2011). Coulomb force directed single and binary assembly of nanoparticles from aqueous dispersions by AFM nanoxerography. ACS Nano 5, 4228–4235. doi: 10.1021/nn2011893
Rega, R., Gennari, O., Mecozzi, L., Grilli, S., Pagliarulo, V., and Ferraro, P. (2016a). Bipolar patterning of polymer sheets by pyroelectrification. Adv. Mater. 28, 454–459. doi: 10.1002/adma.201503711
Rega, R., Gennari, O., Mecozzi, L., Pagliarulo, V., Bramanti, A., Ferraro, P., et al. (2019). Maskless arrayed nanofiber mats by bipolar pyroelectrospinning. ACS Appl. Mater. Interfaces 11, 3382–3387. doi: 10.1021/acsami.8b12513
Rega, R., Gennari, O., Mecozzia, L., Grilli, S., Pagliarulo, V., and Ferraro, P. (2016b). “Pyro-electrification of polymer membranes for cell patterning,” in AIP Conference Proceedings (Ischia: AIP Publishing), 020042.
Robertus, J., Browne, W. R., and Feringa, B. L. (2010). Dynamic control over cell adhesive properties using molecular-based surface engineering strategies. Chem. Soc. Rev. 39, 354–378. doi: 10.1039/B906608J
Rychkov, D., Kuznetsov, A., and Rychkov, A. (2011). “Electret properties of polyethylene and polytetrafluoroethylene films with chemically modified surface,” in Proceedings of the 14th International Symposium on Electrects (Montpellier: IEEE), 8–14.
Sahm, D. F., Weissfeld, A., and Trevino, E. A. (1994). Baily and Scott's Diagnostic Microbiology, 9th Edn. St. Louis, MO: Mosby.
Scifò, G. O., Randazzo, C. L., Restuccia, C., Fava, G., and Caggia, C. (2009). Listeria innocua growth in fresh cut mixed leafy salads packaged in modified atmosphere. Food Control 20, 611–617. doi: 10.1016/j.foodcont.2008.08.017
Seemann, L., Stemmer, A., and Naujoks, N. (2007). Local surface charges direct the deposition of carbon nanotubes and fullerenes into nanoscale patterns. Nano Lett. 7, 3007–3012. doi: 10.1021/nl0713373
Sheng, L., Hanrahan, I., Sun, X., Taylor, M. H., Mendoza, M., and Zhu, M. J. (2018). Survival of Listeria innocua on Fuji apples under commercial cold storage with or without low dose continuous ozone gaseous. Food Microbiol. 76, 21–28. doi: 10.1016/j.fm.2018.04.006
Sprave, M., Blum, R., and Eich, M. (1996). High electric field conduction mechanisms in electrode poling of electro-optic polymers. Appl. Phys. Lett. 69, 2962–2964. doi: 10.1063/1.117744
Stepanović, S., Vuković, D., Dakić, I., Savić, B., and Švabić-Vlahović, M. (2000). A modified microtiter-plate test for quantification of staphylococcal biofilm formation. J. Microbiol. Methods 40, 175–179. doi: 10.1016/S0167-7012(00)00122-6
Stuart, M. A. C., Huck, W. T., Genzer, J., Müller, M., Ober, C., Stamm, M., et al. (2010). Emerging applications of stimuli-responsive polymer materials. Nat. Mater. 9, 101–113. doi: 10.1038/nmat2614
Tajkarimi, M., Harrison, S. H., Hung, A. M., and Graves, J. L. Jr. (2016). Mechanobiology of antimicrobial resistant Escherichia coli and Listeria innocua. PLoS ONE 11:e0149769. doi: 10.1371/journal.pone.0149769
Xi, X., Zhao, D., Tong, F., and Cao, T. (2012). The self-assembly and patterning of thin polymer films on pyroelectric substrates driven by electrohydrodynamic instability. Soft Matter 8, 298–302. doi: 10.1039/C1SM06434G
Keywords: dipoles orientation, pyro-electrification, pyroelectric effect, lithium niobate, cell patterning and manipulation, bacteria biofilm
Citation: Rega R, Gennari O, Mecozzi L, Pagliarulo V, Mugnano M, Oleandro E, Nazzaro F, Ferraro P and Grilli S (2019) Pyro-Electrification of Freestanding Polymer Sheets: A New Tool for Cation-Free Manipulation of Cell Adhesion in vitro. Front. Chem. 7:429. doi: 10.3389/fchem.2019.00429
Received: 29 January 2019; Accepted: 27 May 2019;
Published: 19 June 2019.
Edited by:
Pellegrino Musto, Italian National Research Council (CNR), ItalyReviewed by:
Guoqing Pan, Jiangsu University, ChinaRocktotpal Konwarh, Addis Ababa Science and Technology University, Ethiopia
Copyright © 2019 Rega, Gennari, Mecozzi, Pagliarulo, Mugnano, Oleandro, Nazzaro, Ferraro and Grilli. This is an open-access article distributed under the terms of the Creative Commons Attribution License (CC BY). The use, distribution or reproduction in other forums is permitted, provided the original author(s) and the copyright owner(s) are credited and that the original publication in this journal is cited, in accordance with accepted academic practice. No use, distribution or reproduction is permitted which does not comply with these terms.
*Correspondence: Romina Rega, r.rega@isasi.cnr.it