- Chongqing Key Laboratory of Inorganic Functional Materials, Department of Scientific Research Management, Chongqing Normal University, Chongqing, China
In this study, novel two-dimensional (2D) g-C3N4@BiOCl/Bi12O17Cl2 composites have been fabricated through a facile deposition-precipitation process. The as-prepared photocatalysts were characterized by XRD, SEM, TEM, XPS, UV-vis DRS, PL, Photocurrent, EIS, ESR, and N2 adsorption-desorption. The photocatalytic activities were investigated through NO removal test in gas under visible light irradiation (λ > 420 nm). The g-C3N4@BiOCl/Bi12O17Cl2 composites exhibit enhanced visible light absorption and photo-induced electron-hole separation efficiency, compared with pristine g-C3N4 and BiOCl/Bi12O17Cl2. The intimated contact interfaces between g-C3N4 and BiOCl/Bi12O17Cl2 nanosheets are responsible for the more efficient photochemical interactions. The present work provides a new direction to develop a class of ternary g-C3N4-based visible-light-driven photocatalysts for environmental purification.
Introduction
In the past decades, with the rapid development of modern industrial society, a large amount of highly harmful and toxic contaminants have been discharged into environmental system, which have been the focus of world attention (Han et al., 2017; Li et al., 2018). It remains a great challenge to completely achieve the degradation of environmental contaminants through the conventional treatment process, especially for the low-level concentrations of contaminants (Jiang et al., 2017, 2018; Zhong et al., 2017).
Photocatalysis, as a novel technique, have potential application in degradation low-level concentrations of environmental contaminants under visible-light irradiation (Xiong et al., 2015; Zheng and Zhang, 2016; Guan et al., 2017; Jin et al., 2018). Up to now, although a large number of photocatalysts have been explored for environmental purification, most of them still suffer from the limited utilization of solar light, resulting in quite low visible light photocatalytic activity (Chibac et al., 2017; Zhang et al., 2017). Hence, it is desirable to develop highly efficient visible-light-driven photocatalysts for satisfying the requirements of practical applications.
Currently, graphitic carbon nitride (g-C3N4), an organic semiconductor photocatalyst, has attracted intensive research interest in energy conversion and environmental remediation fields, largely due to its typical physicochemical properties, such as suitable band gap, good chemical and thermal stability, environmental friendly, etc (Wang et al., 2011; Dong and Zhang, 2013; Dong et al., 2015). However, the photocatalytic efficiency of g-C3N4 is far below the requirement of practical applications, mainly due to its fast recombination of photo-excited electron-hole pairs. So far, various strategies have been developed to improve the photocatalytic performance of g-C3N4, including electronic structure engineering, nanostructure optimization, and heterojunction construction (Cao et al., 2015; Zhao et al., 2015; Ong et al., 2016). Furthermore, it is well-known that the g-C3N4 nanosheets can provide a good two-dimensional surface and interface platform for growth of other nanostructured semiconductors. In particular, the heterojunction with other semiconductor photocatalysts has been regarded as an attractive and effective solution in enabling the efficient separation of photo-excited electron-hole pairs, which not only helps to prolong the life-time of photo-excited charge carriers, but also endows g-C3N4-based heterojunctions with more abundant active sites, leading to the significantly enhancement of photocatalytic performance. Successful examples include g-C3N4/TiO2 (Wei et al., 2016), g-C3N4/MoS2 (Hou et al., 2013), g-C3N4/BiOBr (Sun et al., 2014), g-C3N4/WO3 (Huang et al., 2013), g-C3N4/CdS (Liu, 2015), g-C3N4/g-C3N4 (Dong et al., 2013), g-C3N4/(BiO)2CO3 (Zhang et al., 2014), g-C3N4/graphene (Kim et al., 2016), and g-C3N4/Ag (Olga et al., 2016), etc. However, little information about ternary g-C3N4@BiOCl/Bi12O17Cl2 heterojunction for visible light photocatalytic removal of NO has been reported.
Here, we report a highly cost-effective method based on the in situ self-assembly of BiOCl/Bi12O17Cl2 binary nanoplates onto the surface of g-C3N4 nanosheets at room temperature. It takes into consideration advantages of well-matched band structures among g-C3N4, BiOCl and Bi12O17Cl2, the as-prepared ternary g-C3N4@BiOCl/Bi12O17Cl2 heterojunctions exhibit apparent characteristics including larger surface area, improved visible light absorption ability, and efficient separation of photo-induced charge carries, which are extremely favorable for improving the photocatalytic activity.
Fabrication of g-C3N4@BiOCl/Bi12O17Cl2 Composites
The pure g-C3N4 nanosheets and TiO2 powders were fabricated according to the previous reports (Dong et al., 2011; Zhang et al., 2014), respectively. It's a typical synthesize that 1.33 g of BiCl3 and 0.25 g of as-obtained g-C3N4 were added to 50 mL absolute ethyl alcohol and then were ultrasonicated for 30 min. Afterwards, dripping 12.6 mL of NaOH solution (2.0 mol/L) dropwise into BiCl3 solution and then stirring vigorously for 4 h at room temperature. After that, filtering and washing the resulting precipitate for times with distilled water and ethanol. The final samples were obtained after drying under vacuum at 60°C for 24 h. Five types of samples were prepared. The mass rations of BiOCl/Bi12O17Cl2 to g-C3N4 are 1:2, 1:4, 1:1, 2:1, and 4:1, respectively. Accordingly, the final samples were labeled as BOC-CN-1-2, BOC-CN-1-4, BOC-CN-1-1, BOC-CN-2-1, and BOC-CN-4-1, respectively. The pure BiOCl/Bi12O17Cl2 samples were synthesized under the same conditions without adding g-C3N4 nanosheets, and the g-C3N4@BiOCl/Bi12O17Cl2 sample was labeled as BOC.
Materials Characterization
In a typical analysis of the crystal phase of the as-obtained samples, X-ray diffraction with Cu Kα radiation (XRD: model D/max RA, Japan) was applied. Meanwhile scanning electron microscope (SEM, JEOL model JSM-6490, Japan) and transmission electron microscopy (TEM: JEM-2010, Japan) were utilized to characterize the morphology and structure. And the surface properties were examined by X-ray photoelectron spectroscopy with Al Kα X-rays (hν = 1486.6 eV) radiation operated at 150 W (XPS: Thermo ESCALAB 250, USA). By using a Scan UV-vis spectrophotometer (UV-vis DRS: UV-2450, Shimadzu, Japan) equipped with an integrating sphere assembly and BaSO4 as reflectance sample, the UV-vis diffuse reflection spectra was gained. Nitrogen adsorption-desorption was conducted on a nitrogen adsorption apparatus (ASAP 2020, USA) to insure the specific surface areas and total pore volumes. Photoluminescence (PL: F-7000, HITACHI, Japan) was used to investigate the charge transfer properties. ESR spectrometer (FLsp920, England) was applied to detect the electron spin resonance (ESR) signals of •OH and , respectively. The photocurrent measurements (CHI 660B electrochemical system: Shanghai, China) and electrochemical impedance spectroscopy (EIS) were carried out to analyze the photo-generated charge separation properties. All the samples were degassed at 150°C prior to measurements.
Appraisement of Photocatalytic Activity
The photocatalytic activity was investigated by removal of NO at ppb (1 × 10−9) levels in a continuous flow reactor at ambient temperature. The volume of the rectangular reactor, made of stainless steel and covered with Saint-Glass, was 4.5 L (30 × 15 × 10 cm). A 100-W commercial tungsten halogen lamp (THL100, Beijing, China) was vertically placed outside the reactor, and the light spectra range (see Supplementary Figure 1) from 350 to 2,400 nm. A UV cut-off filter (Aike UBG-420, Shenzhen, China) was adopted to remove UV light in the light beam. Photocatalyst (0.2 g) was coated onto a dish with a diameter of 12.0 cm, and the irradiance received by the photocatalyst powder is about 0.66 W/cm2. The coated dish was then pretreated at 70°C to remove water in the suspension. The catalyst adhesion on the dish was firm enough to avoid the erosion (or removal) of the catalyst during air flowing. The NO gas was acquired from a compressed gas cylinder at a concentration of 100 × 10−6 NO (N2 balance, BOC gas). The initial concentration of NO was diluted to about 500 × 10−9 by the air stream. The desired relative humidity (RH) level of the NO flow was controlled at 50% by passing the zero air streams through a humidification chamber. The gas streams were premixed completely by a gas blender, and the flow rate was controlled at 2.4 L·min−1 by a mass flow controller. After the adsorption-desorption equilibrium was achieved, the lamp was turned on. The concentration of NO was continuously measured by a chemiluminescence NO analyzer (Thermo Environmental Instruments Inc., 42i-TL), which monitors NO, NO2, and NOx (NOx represents NO + NO2) with a sampling rate of 1.0 L·min−1. The removal ratio (η) of NO was calculated by η (%) = (1-C/C0) × 100%, where C and C0 are concentrations of NO in the outlet stream and the feeding stream, respectively.
Results and Discussion
The phase structures of the obtained samples were investigated by XRD patterns. As shown in Figure 1, the BOC sample exhibits typical XRD peaks of BiOCl and Bi12O17Cl2 (He et al., 2016; Huang et al., 2016), thus suggesting the formation of BiOCl/Bi12O17Cl2 heterojunctions, the result is good agreement with the previous reports (Zhang et al., 2017). For the pure CN sample, the typical diffraction peaks appeared at 27.4 and 13.1° are indexed to the g-C3N4 (002) and (100) planes (Wang et al., 2011; Cao et al., 2015), respectively. Based on further observation, the peak intensity of the CN decreased with increasing BOC content, implying that interactions exist between the BOC and CN. Finally, two peaks of CN cannot be observed in the BOC-CN composites, which can be ascribed to a good dispersion of BOC onto the surface of CN.
The morphologies and microstructures of BOC, CN, and BOC-CN were characterized by SEM and TEM. For BOC samples (Figure 2), the layered and irregular microstructures consist of smooth nanosheets with different sizes. Moreover, there are two different fringes with the lattice spacing of 0.59 and 0.25 nm (Figure 2d), which can be indexed to the (006) crystal plane of Bi12O17Cl2 and (003) crystal plane of BiOCl, respectively. For CN samples (Figure 3), it presents the lamellar morphology is composed of numerous nanosheets with a much looser pore structure. As shown in Figures 4a–d, the BOC-CN composites also consist of a large number of layered nanosheets with different shapes. Obviously, the BOC nanosheets were in situ growth on the surface of g-C3N4, resulting in the formation of closely interface in g-C3N4@BiOCl/Bi12O17Cl2 composites, which is beneficial for the separation and transfer of the photo-induced electron-hole pairs. The surface element dispersion state of BOC-CN composites are studied by EDS mapping. As shown in Figures 4e–k, the Bi, C, Cl, N, and O elements are uniformly distributed in BOC-CN samples.
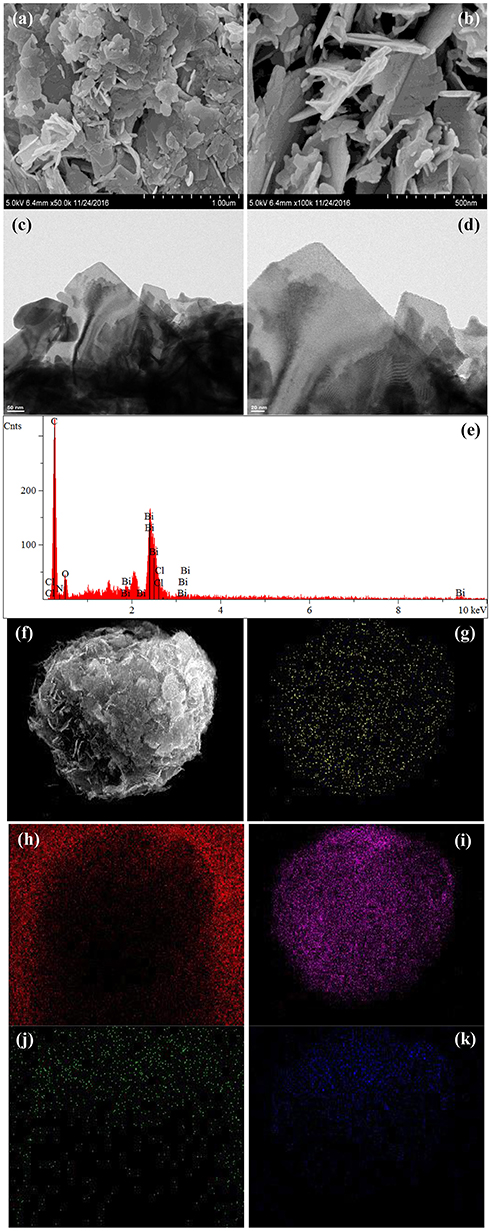
Figure 4. SEM (a,b) and TEM (c,d) images of BOC-CN. EDS elemental mapping (e–k) of the same region, indicating the spatial distribution of Bi (g), C (h), Cl (i), N (j), and O (k), respectively.
The XPS measurements were applied to verify the composition and chemical state of the elements. Two peaks at 159.1 and 164.3 eV are consistent with Bi4f7/2 and Bi4f5/2 (Figure 5A), respectively. The XPS spectrum for Cl shows two peaks at 197.8 and 199.4 eV attributed to Cl2p3/2 and Cl2p1/2 (Figure 5B), respectively. The peak centered at 530.3 eV that corresponds to the binding energy of O 1s (Figure 5C) (Bi et al., 2016; Zhang et al., 2017). The C peak at 284.8 eV can be ascribed to the adventitious carbon atom. Figure 5D shows one peak at 288.5 is identified as overlapped peaks of N-C = N and the O-C = O. Two different peaks are observed in Figure 5E, the N peak at 398.9 eV correspond to the C = N-C and the N peak at 400.8 eV is attributed to the residual amino groups (Zhang et al., 2014).
The optical absorption property of the as-obtained samples was investigated by UV-vis DRS. As can be seen from Figure 6A, BOC and CN exhibit absorbance edge around 550 and 475 nm, respectively, displaying that BOC and CN possess good visible light absorption ability. Interestingly, BOC-CN-4-1 shows relatively stronger visible light absorption ability lies in the range of 550–800 nm, because of the synergetic effect between BOC and CN. Figure 6B shows the PL spectra for BOC, CN, and BOC-CN-4-1 using the exciting light of 320 nm. Compared to CN, the PL intensity of BOC-CN-4-1 significantly decreases, demonstrating that the interface interaction between BOC and CN could inhibit the recombination rate of photo-generated electrons and holes.
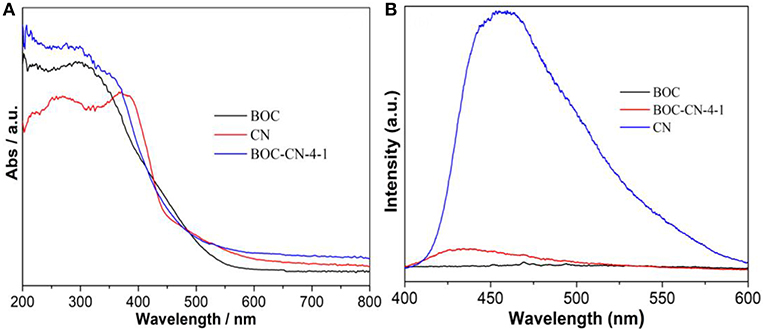
Figure 6. UV-vis diffuse reflectance spectra (A) and PL spectra (B) for the BOC, CN, and BOC-CN-4-1 samples.
The photocurrent and electrochemical impedance experiments were used to investigated the photo-generated charges separation and transfer property of BOC, CN, and BOC-CN-4-1 samples under visible light irradiation (Zhang et al., 2018a). Compared with the pure BOC and CN, it was interesting to find that BOC-CN-4-1 exhibits significantly enhanced photocurrent density (Figure 7A), suggesting that BOC-CN-4-1 possesses higher photo-generated charge separation property. As can be seen from the Figure 7B, the arc radius on the EIS Nyquist plot of BOC-CN-4-1 was smaller than that of the pure BOC and CN, demonstrating that BOC-CN-4-1 has much more efficient photo-generated charge separation and transfer property.
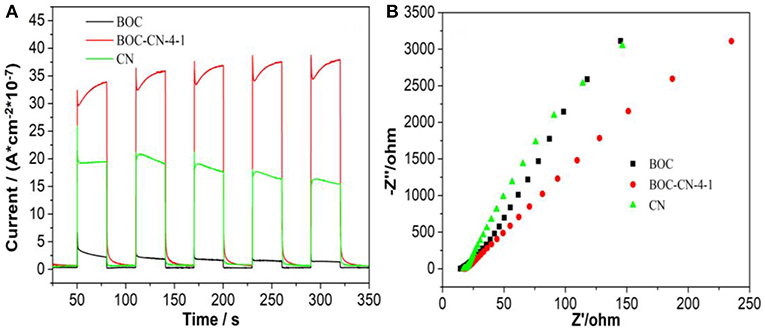
Figure 7. Photocurrent response (A) and Nyquist plots (B) for BOC, CN, and BOC-CN-4-1 samples under visible light irradiation (λ ≥ 420 nm, [Na2SO4] = 0.5 M).
Figure 8A shows that the adsorption-desorption isotherms of all the samples are type IV according to the IUPAC classification, demonstrating that all the samples have mesopores (Dong et al., 2013; Zhang et al., 2014). However, the typical H3 hysteresis loop at low pressure can be ascribed to the aggregation of nanosheets with slit-like pores. Figure 8B further confirms the presence of mesopores in BOC, CN, and BOC-CN samples. In addition, the specific surface area (SBET) and total pore volume (Vp) are 32.8 m2/g and 0.176 cm3/g for BOC-CN composites, which are higher than those of pure BOC (29.7 m2/g and 0.168 cm3/g) and CN (21.8 m2/g and 0.132 cm3/g), the enlarged SBET and Vp of BOC-CN composites can be attributed to the stack of nanosheets layered by layered. The enlarged SBET and Vp of BOC-CN composites can facilitate the reactants adsorb and transfer, and provide more active sites for the photocatalytic reaction.
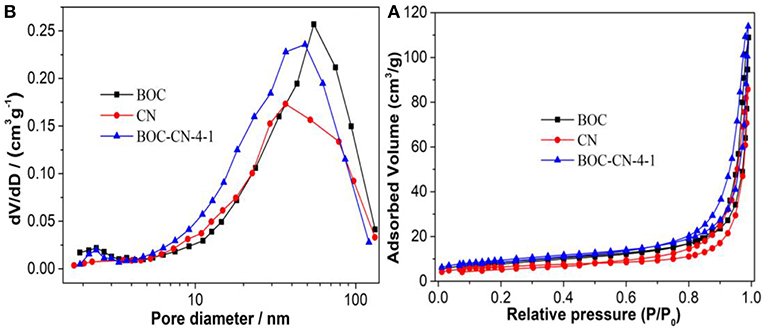
Figure 8. The adsorption-desorption isotherms (A) and pore size distribution curves (B) of BOC, CN, and BOC-CN-4-1 samples.
The electron spin resonance (ESR) experiments were further used to confirm the active species during the photocatalytic reaction process under visible light irradiation. As shown in Figures 9A,B, the superoxide () radicals and hydroxyl (•OH) radicals have been successfully detected by the ESR technique, respectively. Moreover, the intensity of all peaks increase significantly with the irradiation time, demonstrating that and •OH are continuously generated during the reaction. The result shows that both and •OH are the main photocatalytic reaction active species. The formation of and •OH from the photochemical reaction shows in Equations 1–3 (Zhang et al., 2018a).
Furthermore, the possible mechanism for the photocatalytic reaction at the BOC-CN interface are presented in Figure 10. After the visible light irradiation, the Bi12O17Cl2 and g-C3N4 can be excited and then produce electron-hole pairs (Dong et al., 2013; Zhang et al., 2018b). On the one hand, the excited electrons in CB of g-C3N4 can directly transfer to CB of BiOCl (Zhang et al., 2013). On the other hand, the excited holes in VB of Bi12O17Cl2 can transfer to VB of g-C3N4, the excited electrons in CB of g-C3N4 can transfer to CB of Bi12O17Cl2, and the electrons can further transfer to the CB of BiOCl from the CB of Bi12O17Cl2, the band structures of the three components are well-matched resulting in efficient separation and transfer of the photo-induced carriers. Hence, the suitable band structures clearly show that the efficient electron-hole pair separation plays critical role in improving the photochemical reaction (Zhang et al., 2013, 2014).
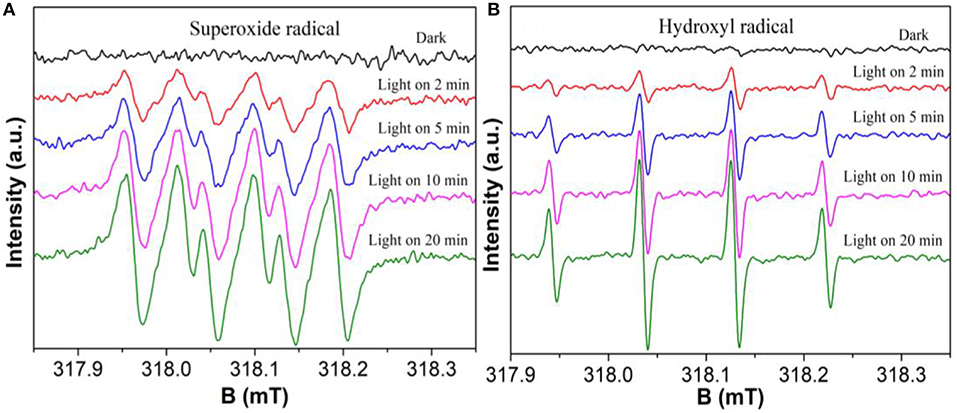
Figure 9. DMPO spin-trapping ESR spectra of BOC-CN-4-1 in methanol dispersion for DMPO- (A), and in aqueous dispersion for DMPO-•OH (B), respectively.
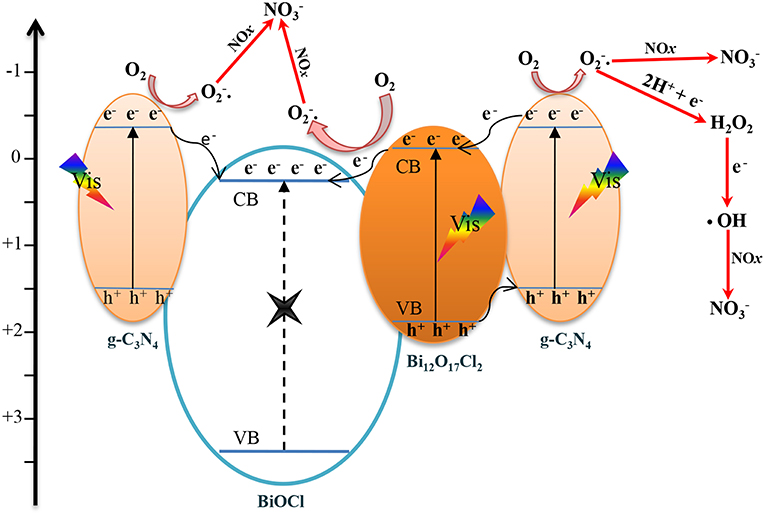
Figure 10. The proposed schematic mechanism for the photocatalytic reation at the BOC-CN heterojunction interface.
The visible-light-induced photocatalytic activities of the TiO2, BOC, CN, and BOC-CN samples toward NO were revealed in Figure 11A. The pure BOC and CN only removed 36.2 and 14.6% of NO after 30 min visible light irradiation due to its fast recombination of photo-induced carriers, respectively. However, the visible-light-induced photocatalytic activities of the TiO2 sample can be neglected under the same conditions, indicating that the activity does not result from a UV-A light induced photocatalytic activity due to some trace of UV-A light after the cut-off filter. When the heterojunction was formed, the NO removal ratio over BOC-CN composites was increased to 46.8%. For ruling out and evidencing the NO degradation, the adsorption experiment of the optimized BOC-CN-4-1was carried out under dark condition, the result shows that adsorption property of NO over the BOC-CN-4-1-dark sample can also be ignored. The photocatalytic stability experiment of the BOC-CN-4-1 sample was evaluated by repeating the reaction for five runs under visible light irradiation. As shown in in Figure 11B, the photocatalytic performance shows slightly loss after five run, indicating that BOC-CN-4-1 photocatalyst possesses good photocatalytic stability. Interestingly, the BOC-CN composites exhibit even higher visible light photocatalytic activity than that of BiOBr/C3N4 (removal rate of 32.7%) and p-doped g-C3N4 (removal rate of 42.3%) (Sun et al., 2014; Zhang et al., 2016). The enhanced photocatalytic activity of BOC-CN can be attributed to the synergistic contribution of BOC and g-C3N4 with respect to the suitable band structure, enlarged SBET and Vp, improved visible light absorption, and efficient photo-induced carrier separation at the interface of BOC and g-C3N4 (Dong et al., 2013; Hou et al., 2013; Sun et al., 2014; Liu, 2015; Wei et al., 2016).
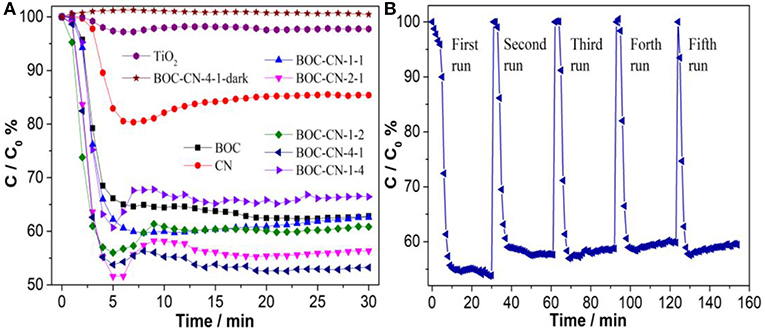
Figure 11. (A) Photocatalytic removal of NO over the as-obtained samples, (B) cycling runs of the BOC-CN-4-1 composite in air under visible light irradiation (λ > 420 nm).
Conclusion
In summary, we have synthesized ternary BOC-CN heterojunctions with outstanding visible light photocatalytic performance by self-assembly of BiOCl/Bi12O17Cl2 nanosheets on the surface of g-C3N4 nanosheets via a chemical deposition-precipitation method. The results reveal that g-C3N4, BiOCl, and Bi12O17Cl2 possess well-matched band structures, which is helpful to the separation and transport of photo-induced carriers. This work provides a new perspective for the design and fabrication of high performance and stable BiOCl/Bi12O17Cl2-based photocatalysts via a facile method at room temperature.
Author Contributions
WZ: experiment, data analysis, and paper writing. YL: paper writing.
Funding
This research is financially supported by the National Natural Science Foundation of China (No. 51708078), Natural Science Foundation of Chongqing (CSTC, 2018jcyjA1040), and the Chongqing Postdoctoral Science Foundation funded project (No. Xm2016027).
Conflict of Interest Statement
The authors declare that the research was conducted in the absence of any commercial or financial relationships that could be construed as a potential conflict of interest.
Supplementary Material
The Supplementary Material for this article can be found online at: https://www.frontiersin.org/articles/10.3389/fchem.2019.00231/full#supplementary-material
Supplementary Figure 1. The light spectra range of tungsten halogen lamp.
References
Bi, C. J., Cao, J., Lina, H. L., Wang, Y. J., and Chen, S. F. (2016). Enhanced photocatalytic activity of Bi12O17Cl2 through loading Pt quantum dots as a highly efficient electron capturer. Appl. Catal. B Environ. 195, 132–140. doi: 10.1016/j.apcatb.2016.05.011
Cao, S., Low, J., Yu, J., and Jaroniec, M. (2015). Polymeric photocatalysts based on graphitic carbon nitride. Adv. Mater. Weinheim. 27, 2150–2176. doi: 10.1002/adma.201500033
Chibac, A. L., Buruiana, T., Melinte, V., and Buruiana, E. C. (2017). Photocatalysis applications of some hybrid polymeric composites incorporating TiO2 nanoparticles and their combinations with SiO2/Fe2O3. Beilstein J. Nanotechnol. 8, 272–286. doi: 10.3762/bjnano.8.30
Dong, F., Guo, S., Wang, H. Q., Li, X. F., and Wu, Z. B. (2011). Enhancement of the visible light photocatalytic activity of c-doped TiO2 nanomaterials prepared by a green synthetic approach. J. Phys. Chem. C. 115, 13285–13292. doi: 10.1021/jp111916q
Dong, F., Zhao, Z., Xiong, T., Ni, Z., Zhang, W., and Sun, Y. (2013). In situ construction of g-C3N4/g-C3N4 metal-free heterojunction for enhanced visible-light photocatalysis. ACS Appl. Mater. Interface 5, 11392–11401. doi: 10.1021/am403653a
Dong, G. H., Ho, W. K., Li, Y. H., and Zhang, L. Z. (2015). Facile synthesis of porous graphene-like carbon nitride (C6N9H3) with excellent photocatalytic activity for NO removal. Appl. Catal. B Environ. 174–175, 477–485. doi: 10.1016/j.apcatb.2015.03.035
Dong, G. H., and Zhang, L. Z. (2013). Synthesis and enhanced Cr(VI) photoreduction property of formate anion containing graphitic carbon nitride. J. Phys. Chem. C. 117, 4062–4068. doi: 10.1021/jp3115226
Guan, W., Zhang, Z. H., Tian, S. C., and Du, J. W. (2017). Ti4O7/g-C3N4 for visible light photocatalytic oxidation of hypophosphite: effect of mass ratio of Ti4O7/g-C3N4. Front. Chem. 8:313. doi: 10.3389/fchem.2018.00313
Han, J., Zheng, X. Z., Zhang, L. W., Fu, H. B., and Chen, J. M. (2017). Removal of SO2 on a nanoporous photoelectrode with simultaneous H2 production. Environ. Sci. Nano 4, 834–842. doi: 10.1039/C6EN00638H
He, G. P., Xing, C. L., Xiao, X., Hu, R. P., Zuo, X. X., and Nan, J. M. (2016). Facile synthesis of flower-like Bi12O17Cl2/β-Bi2O3 composites with enhanced visible light photocatalytic performance for the degradation of 4-tert-butylphenol. Appl. Catal. B Environ. 170–171, 1–9. doi: 10.1016/j.apcatb.2015.01.015
Hou, Y., Lauren, A. B., Zhang, J., Zhang, G., Zhu, Y., Wang, X., et al. (2013). Layered nanojunctions for hydrogen-evolution catalysis. Angew. Chem. Int. Ed. 52, 1–6. doi: 10.1002/anie.201210294
Huang, H. W., Xiao, K., He, Y., Zhang, T. R., Dong, F., Du, X., et al. (2016). Rational design on 3D hierarchical bismuth oxyiodides via in situ self-template phase transformation and phase-junction construction for optimizing photocatalysis against diverse contaminants. Appl. Catal. B Environ. 199, 75–86. doi: 10.1016/j.apcatb.2016.10.082
Huang, L., Xu, H., Li, Y., Li, H., Cheng, X., and Xia, J. (2013). Visible-light-induced WO3/g-C3N4 composites with enhanced photocatalytic activity. Dalton. Trans. 42, 8606–8616. doi: 10.1039/C3DT00115F
Jiang, G., Lan, M., Zhang, Z., Lv, X., Lou, Z., Xu, X., et al. (2017). Identification of active hydrogen species on palladium nanoparticles for an enhanced electrocatalytic hydrodechlorination of 2,4-dichlorophenol in water. Environ. Sci. Technol. 51, 7599–7605. doi: 10.1021/acs.est.7b01128
Jiang, G. M., Wang, K. F., Li, J. Y., Fu, W. Y., Zhang, Z. Y., Johnson, G., et al. (2018). Electrocatalytic hydrodechlorination of 2,4-dichlorophenol over palladium nanoparticles and its pH-mediated tug-of-war with hydrogen evolution. Chem. Eng.J. 348, 26–34. doi: 10.1016/j.cej.2018.04.173
Jin, S., Dong, G. H., Luo, J. M., Ma, F. Y., and Wang, C. Y. (2018). Improved photocatalytic NO removal activity of SrTiO3 by using SrCO3 as a new co-catalyst. Appl. Catal. B Environ. 227, 24–34. doi: 10.1016/j.apcatb.2018.01.020
Kim, S. Y., Oh, J., Park, S., Shim, Y., and Park, S. (2016). Production of metal-free composites composed of graphite oxide and oxidized carbon nitride nanodots and their enhanced photocatalytic performances. Chem. Eur. J. 22, 5142–5145. doi: 10.1002/chem.201505100
Li, Y. H., Wu, X. F., Ho, W. K., Lv, K. L., Li, Q., Li, M., et al. (2018). Graphene-induced formation of visible-light-responsive SnO2-Zn2SnO4 Z-scheme photocatalyst with surface vacancy for the enhanced photoreactivity towards NO and acetone oxidation. Chem. Eng.J. 336, 200–210. doi: 10.1016/j.cej.2017.11.045
Liu, J. J. (2015). Origin of high photocatalytic efficiency in monolayer g-C3N4/CdS heterostructure: a hybrid DFT study. J. Phys. Chem. C 119, 28417–28423. doi: 10.1021/acs.jpcc.5b09092
Olga, F. C., Mario, J. M. B., Marcos, F. G., and Anna, K. (2016). Interface effects in sunlight-driven Ag/g-C3N4 composite catalysts: study of the toluene photodegradation quantum efficiency. ACS Appl. Mater. Interface 8, 2617–2627. doi: 10.1021/acsami.5b10434
Ong, W. J., Tan, L. L., Ng, Y. H., Yong, S. T., and Chai, S. P. (2016). Graphitic carbon nitride (g-C3N4)-based photocatalysts for artificial photosynthesis and environmental remediation: are we a step closer to achieving sustainability? Chem. Rev. 116, 7159–7329. doi: 10.1021/acs.chemrev.6b00075
Sun, Y., Zhang, W., Xiong, T., Zhao, Z., Dong, F., Wang, R., et al. (2014). Growth of BiOBr nanosheets on C3N4 nanosheets to construct two-dimensional nanojunctions with enhanced photoreactivity for NO removal. J. Colloid. Interf. Sci. 418, 317–323. doi: 10.1016/J.JCIS.2013.12.037
Wang, Y., Wang, X. C., and Antonietti, M. (2011). Polymeric graphitic carbon nitride as a heterogeneous organocatalyst: from photochemistry to multipurpose catalysis to sustainable chemistry. Angew. Chem. Int. Ed. 50, 2–24. doi: 10.1002/anie.201101182
Wei, X., Shao, C., Li, X., Lu, N., Wang, K., Zhang, Z., et al. (2016). Facile in situ synthesis of plasmonic nanoparticles-decorated g-C3N4/TiO2 heterojunction nanofibers and comparison study of their photosynergistic effects for efficient photocatalytic H2 evolution. Nanoscale 8, 11034–11043. doi: 10.1039/C6NR01491G
Xiong, T., Zhang, H. J., Zhang, Y. X., and Dong, F. (2015). Ternary Ag/AgCl/BiOIO3 composites for enhanced visible-light-driven photocatalysis. Chin. J. Catal. 8, 784–788. doi: 10.1016/S1872-2067(15)60980-9
Zhang, R. Y., Wan, W. C., Li, D. W., Dong, F., and Zhou, Y. (2017). Three-dimensional MoS2/reduced graphene oxide aerogel as a macroscopic visible-light photocatalyst. Chin. J. Catal. 2, 313–320. doi: 10.1016/S1872-2067(16)62568-8
Zhang, W., Sun, Y., Dong, F., Zhang, W., Duan, S., and Zhang, Q. (2014). Facile synthesis of organic–inorganic layered nanojunctions of g-C3N4/(BiO)2CO3 as efficient visible light photocatalyst. Dalton. Trans. 43, 12026–12036. doi: 10.1039/c4dt00513a
Zhang, W. D., Dong, X. A., Bin, J., Zhong, J. B., Sun, Y. J., and Dong, F. (2018a). 2D BiOCl/Bi12O17Cl2 nanojunction: enhanced visible light photocatalytic NO removal and in situ DRIFTS investigation. Appl. Surf. Sci. 430, 571–577. doi: 10.1016/j.apsusc.2017.06.186
Zhang, W. D., Dong, X. A., Liang, Y., Sun, Y. J., and Dong, F. (2018b). Ag/AgCl nanoparticles assembled on BiOCl/Bi12O17Cl2nanosheets: enhanced plasmonic visible light photocatalysis and in situ DRIFTS investigation. Appl. Surf. Sci. 455, 236–243. doi: 10.1016/j.apsusc.2018.05.171
Zhang, W. D., Zhang, J., Dong, F., and Zhang, Y. X. (2016). Facile synthesis of in situ phosphorus-doped g-C3N4 with enhanced visible light photocatalytic property for NO purification. RSC Adv. 6, 88085–88089. doi: 10.1039/C6RA18349B
Zhang, W. D., Zhang, Q., and Dong, F. (2013).Visible-light photocatalytic removal of NO in air over BiOX (X = Cl, Br, I) single-crystal nanoplates prepared at room temperature. Ind. Eng. Chem. Res. 52, 6740–6746. doi: 10.1021/ie400615f
Zhao, Z., Sun, Y., and Dong, F. (2015). Graphitic carbon nitride based nanocomposites: a review. Nanoscale 7, 15–37. doi: 10.1039/C4NR03008G
Zheng, X. Z., and Zhang, L. W. (2016). Photonic nanostructures for solar energy conversion. Energy Environ. Sci. 9, 2511–2532. doi: 10.1039/C6EE01182A
Keywords: facile synthesis, g-C3N4@BiOCl/Bi12O17Cl2, visible light, photocatalytic activity, nitrogen oxide (NO) removal
Citation: Zhang W and Liang Y (2019) Facile Synthesis of Ternary g-C3N4@BiOCl/Bi12O17Cl2 Composites With Excellent Visible Light Photocatalytic Activity for NO Removal. Front. Chem. 7:231. doi: 10.3389/fchem.2019.00231
Received: 19 August 2018; Accepted: 25 March 2019;
Published: 11 April 2019.
Edited by:
Nicolas Keller, l'Environnement et la Santé (ICPEES), FranceReviewed by:
Dong Guohui, Shaanxi University of Science and Technology, ChinaBitao Liu, Chongqing University of Arts and Sciences, China
Liwu Zhang, Fudan University, China
Copyright © 2019 Zhang and Liang. This is an open-access article distributed under the terms of the Creative Commons Attribution License (CC BY). The use, distribution or reproduction in other forums is permitted, provided the original author(s) and the copyright owner(s) are credited and that the original publication in this journal is cited, in accordance with accepted academic practice. No use, distribution or reproduction is permitted which does not comply with these terms.
*Correspondence: Wendong Zhang, w5i1@163.com