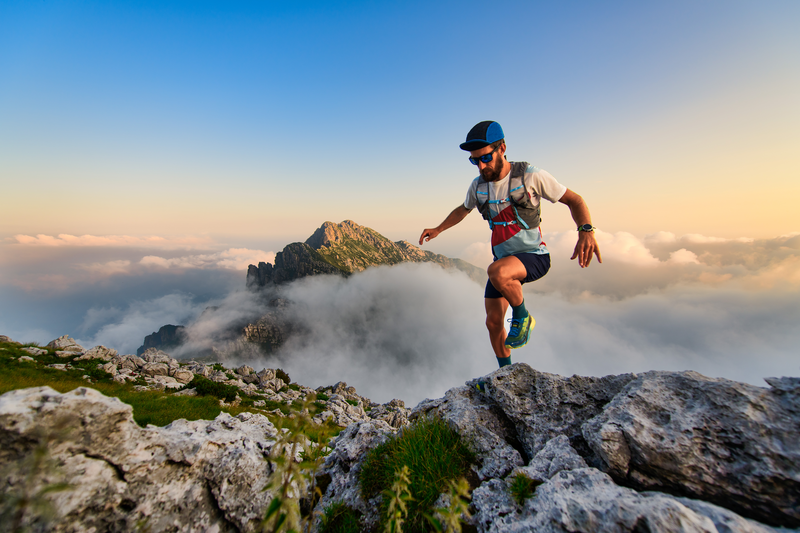
95% of researchers rate our articles as excellent or good
Learn more about the work of our research integrity team to safeguard the quality of each article we publish.
Find out more
REVIEW article
Front. Chem. , 10 December 2018
Sec. Green and Sustainable Chemistry
Volume 6 - 2018 | https://doi.org/10.3389/fchem.2018.00551
This article is part of the Research Topic Visible Light-Responsive Photocatalysts for Energy Production View all 8 articles
Graphitic carbon nitride (g-C3N4) is always a research hotspot as a metal-free visible-light-responsive photocatalyst, in the field of solar energy conversion (hydrogen-production by water splitting). This critical review summarizes the recent progress in the design and syntheses of two-dimensional (2D) g-C3N4 and g-C3N4-based nanocomposites, covering (1) the modifications of organic carbon nitrogen precursors, such as by heat treatment, metal or metal-free atoms doping, and modifications with organic functional groups, (2) the influencing factors for the formation of 2D g-C3N4 process, including the calcination temperature and protective atmosphere, etc. (3) newly 2D g-C3N4 nanosheets prepared from pristine raw materials and bulk g-C3N4, and the combination of 2D g-C3N4 with other 2D semiconductors or metal atoms as a cocatalyst, and (4) the structures and characteristics of each type of 2D g-C3N4 systems, together with their optical absorption band structures and interfacial charge transfers. In addition, the first-principles density functional theory (DFT) calculation of the g-C3N4 system has been summarized, and this review provides an insightful outlook on the development of 2D g-C3N4 photocatalysts. The comprehensive review is concluded with a summary and future perspective. Moreover, some exciting viewpoints on the challenges, and future directions of 2D g-C3N4 photocatalysts are discussed and highlighted in this review. This review can open a new research avenue for the preparation of 2D g-C3N4 photocatalysts with good performances.
The energy crisis has become a growing concern as society continues to develop, which further necessitates the development of sustainable energy sources to supersede traditional fossil fuels (Chang et al., 2017; He et al., 2017a,b, 2018; Wang et al., 2018; Zhang G. G. et al., 2018). The hydrogen produced by the photocatalytic water splitting reaction under sunlight, resulting in solar-to-chemical energy conversion, has been deemed to play a key role in resolving the solar-to-chemical energy conversion (Zhong et al., 2016; Zhang G. G. et al., 2018; Zhang S. W. et al., 2018). As a half reaction of the hydrogen production via water splitting, the reaction progress is the decrease of protons/water to hydrogen (Bard and Fox, 1995; Zou et al., 2001). Although the produced hydrogen process refers to the simple reactants, demanding only two electrons to generate a hydrogen molecule, the reaction kinetics is slow due to the large energy barriers in the multiple reaction steps (Tu et al., 2013; Wondraczek et al., 2015; Zhang N. et al., 2015; Zhang et al., 2017a). At the same time, the reduction of water to hydrogen requires many photo-induced holes with oxidant properties (Zhang et al., 2014). Therefore, photocatalysts are indispensable for these reactions, which can generate photo-induced electrons and holes under sunlight. So far, the most effective photocatalysts are still metal-based materials (Ma F. K. et al., 2016; Ma Z. et al., 2016; Ai et al., 2018). However, the high cost and heavy-metal-toxicity of these photocatalysts limit their usage.
In recent decades, abundant non-metal photocatalysts, mainly based on earth-abundant non-metals elements (P, S, N, and C), have been explored. Among them is graphitic carbon nitride (g-C3N4), which has recently been widely used in the field of photocatalytic water splitting, as a metal-free and environmentally friendly photocatalytic material, (Wang et al., 2012, 2014; Low et al., 2014; Dong and Cheng, 2015), of which the bulk and granulated g-C3N4 are the most widely used electrocatalysts for hydrogen production. This seriously inhibits the efficiency of photocatalytic water splitting (Zhang G. G. et al., 2016). Moreover, the application of g-C3N4 is restricted in the reaction because of its frequent photo-corrosion under sunlight. Therefore, continuous efforts have been made to develop more stable and efficient g-C3N4-based heterogeneous photocatalysts in recent years (Wang et al., 2011; Shi et al., 2015; Li G. et al., 2016; Yang et al., 2016). Additionally, as a new type of two-dimensional (2D) material, 2D g-C3N4 has been utilized as a photocatalyst in solar-driven water splitting. The progress in this research field is discussed in this review.
This critical review summarizes the recent progress made in the formation of 2D g-C3N4 (g-C3N4-based nanocomposites) for hydrogen production, and further elucidates the modifications of functional groups, the influencing factors of the formation process, new methods, heterojunction nanostructures, and so on. In addition, the DFT calculations for the g-C3N4 systems are also summarized to provide an insightful outlook. Finally, this review is concluded with a summary and future perspective.
As a fascinating material, 2D g-C3N4 has attracted worldwide attention (Ma et al., 2014; Liang et al., 2015), and promises access to a wide field of applications compared with other photocatalytic materials, due to its outstanding features, such as its non-metal and non-toxicity (Liu G. et al., 2015; Zhang G. G. et al., 2015; Zhang M. et al., 2016; Zhang et al., 2017b). Furthermore, g-C3N4 is a wide-band gap indirect semiconductor (Schwinghammer et al., 2013) with an appealing electronic structure. This allows its direct use as a heterogeneous photocatalyst. However, the photocatalytic effect of pure g-C3N4 is inferior to those of metal semiconductor photocatalysts. Therefore, some modifications, such as metal-free or metal atom doping, are necessary to improve the photocatalytic effects of g-C3N4.
For the doping of g-C3N4 with metal-free atoms, halogen elements are very important and effective (Groenewolt and Antonietti, 2005; Chang et al., 2015; Han et al., 2016; Ye et al., 2016; Ma et al., 2017), The ionic radii of the incorporated guests of halogen elements decrease in the order F<Cl<Br (Chong et al., 2013). Generally, by using a heating treatment (dicyandiamide) in eutectic melting salt, such as LiY and KY (Y = F, Cl or Br), bulk g-C3N4 can chemically and physically be exfoliated into thin layers (Li+, K+ or X−) (Bojdys et al., 2013; Ma et al., 2017). As early as 2010, Wang et al. reported the synthesis of a fluorinated polymeric carbon nitride, which was employed as a heterogeneous catalyst for hydrogen generation from water. In addition, it was also used for the oxygenation of benzene into phenol under visible light (Figure 1, Wang et al., 2010). Other metal-free atoms are also used to dope g-C3N4, such as O, C, N, P, S, and B (Ran et al., 2015; Feng et al., 2016; Lu et al., 2017; Zhu et al., 2017). Zhang et al. used S8 (elemental sulfur) and melamine as the raw materials to obtain g-C3N4-Sx, where x refers to the quality of S8 (Zhang J. et al., 2012), in which the absorption edges of CN-Sx samples became marginally red-shifted with adding S8 contents (Figure 2), thus decreasing the corresponding band gaps (Eg) from 2.76 to 2.58 eV (Zhang J. et al., 2012).
Figure 1. (A) XRD patterns of samples. (B) UV-Vis spectra of g-C3N4 and CNF-x (inset shows optical band gaps(E)g of g-C3N4 and sample-2.0). (C) XPS spectrum of sample-2.0. (D) Solid-state MAS-NMR spectrum of sample-2.0 (Wang et al., 2010). Copyright 2010, American Chemical Society.
Figure 2. (a) Image of the g-C3N4 and CN-S2.0. (b) TEM images of CN-S2.0 and (c) unmodified g-C3N4. (d) High resolution XPS spectra of samples. (e) EPR spectra of samples in the dark. (F) UV–Vis spectra of samples (Zhang J. et al., 2012). Copyright 2012, American Chemical Society.
The doping of g-C3N4 with metal atoms (Fe3+, Co2+, Ni2+, Cu2+, Zn2+, K+, Na+, and Li+) has also been widely used to enhance the catalytic properties of g-C3N4 (Figure 3, Pan et al., 2011; Yue et al., 2011; Ding et al., 2013; Tonda et al., 2014; Ye et al., 2014; Ong et al., 2016). For example, Wang et al have reported a g-C3N4 framework, including Zn2+ and Fe2+ for the first time, which could improve the visible-light absorption, decrease the band gap (Eg), expedite the charge mobility and extend the lifetime of charge carriers. All these characteristics are necessary to improve photocatalytic activity (Wang X. et al., 2009; Wang X. C. et al., 2009).
Figure 3. Schematic structure of g-C3N4 framework with obtaining metal ion (Ong et al., 2016). Copyright 2016, American Chemical Society.
Since Wang et al. proposed the preparation of g-C3N4 as an efficient photocatalyst (Wang X. C. et al., 2009), g-C3N4 materials have gradually become a hot topic in the field of energy and catalysis research, due to advantages such as its low-cost, sustainability and visible-light response (Martin et al., 2014a; Xu et al., 2015; Zheng et al., 2015; Kang et al., 2016; Li J. et al., 2016). In recent years, high-efficiency 2D g-C3N4 nanosheet photocatalysts have been prepared by an organic reaction. For example, phenylene groups can be part of carbon nitrides through the copolymerization of 2-aminobenzonitrile (CN-ABN0.5) with dicyandiamide (Zhang et al., 2010; Zhang J. S. et al., 2012). The optical absorption edge of carbon nitride red-shifted to 700 nm from that of the pristine carbon nitride (460 nm), as the 2-aminobenzonitrile content increased. The sample (CN-ABN0.05 with a platinum co-catalyst) showed the topmost photocatalytic evolution of hydrogen (147 μmol h−1) compared with pristine carbon nitride (18 μmol h−1 at λ > 420 nm) (Zhang et al., 2010; Zhang J. S. et al., 2012). Zhao et al. designed a 2D g-C3N4 organic material (with a thickness of about 1.5 nm), which was successfully synthesized from melamine raw materials for the first time. The synthetic method for the 2D g-C3N4 organic material was simple and efficient. Based on the organic synthesis theory, the synthetic mechanism was theoretically explored (Figure 4, Zhao G. et al., 2018). These photocatalysts have good photocatalytic hydrogen production compared to common bulk g-C3N4 (Zhao G. et al., 2018).
Figure 4. (a) SEM images of melamine, and (b) layered CN. (c,d) AFM images of layered CN, (e) Infrared spectra of melamine and synthetic layered CN, (f) XPS spectra of layered CN (C 1s) (Zhao G. et al., 2018). Copyright 2018, Wiley-VCH.
Two-dimensional g-C3N4 with atomic thickness has become a fascinating material in photocatalysis, because of the large specific surface area and efficiently photoexcited carriers, which can decrease the possibility of electron-hole recombination (Zhu et al., 2010; Shiraishi et al., 2014, 2015; Liu et al., 2015a,b; Shi et al., 2015). However, the synthesis or exfoliation of ultrathin (monolayer or bilayer) 2D g-C3N4 nanosheets with a homogeneous thickness, continues to be a large-scale challenge.
It is known that g-C3N4 has a two-dimensional laminated structure parallel to graphene and the theoretical specific surface area of the ideal monolayer g-C3N4 can reach up to 2,500 m2 g−1. Inspired by the formation of graphene from graphite exfoliation, many effective ways have been explored for the exfoliation of raw bulk g-C3N4 to obtain a 2D ultrathin structure, such as ultrasonic liquid exfoliation, chemical exfoliation, and thermal oxidation exfoliation as well as other methods (Niu et al., 2012; Yang et al., 2013; Feng et al., 2016). For example, Yang et al. prepared g-C3N4 nanosheets from bulk g-C3N4 powders with a simple and cost-effective liquid exfoliation method (Figure 5, Yang et al., 2013). These nanosheets possess the structural features of homogeneous decentralized carbon and nitrogen atoms, an infinitesimal thickness, a large specific surface area (BET) and an optimal bandgap, which can bring about good photocatalytic activity with regards to the hydrogen evolution in visible light (Yang et al., 2013).
Figure 5. Fabrication of 2D g-C3N4 nanosheets using a simple method from bulk g-C3N4 powders for hydrogen evolution under visible light (Yang et al., 2013). Copyright 2013, Wiley-VCH.
Although these methods can effectively synthesize some g-C3N4 nanosheets and improve the photocatalytic property, the recombination of the electron-hole on the surfaces of the 2D materials, remains a key issue for most single-phase photocatalysts (Dong et al., 2013; Martin et al., 2014b; Ye et al., 2015). Therefore, the concept of 2D g-C3N4-based nanocomposites was proposed. Theoretical models have predicted that the restoration of photo-generated electrons/holes could be pounding down because of their effective spatial isolation on the heterojunction interface (Dong et al., 2013). Additionally, other advantages of photocatalytic reactions can also be achieved such a: good visible-light absorption and outstanding surface reaction activity. Herein, the design of 2D g-C3N4-based nanocomposites has become a research hotspot to improve the photocatalytic performance (Iwase et al., 2011; Lin and Wang, 2014; Chen et al., 2015; Han et al., 2016; She et al., 2016, 2017). For example, She et al reported that small amounts of α-Fe2O3 nanosheets could actively promote the exfoliation of g-C3N4, preparing a 2D hybrid structure that exhibited an effective Z-scheme junction (She et al., 2017). The nanostructured hybrids presented a high H2 evolution rate >3 × 104 μmol g−1 h−1 and the quantum efficiency was about 44.35% at 420 nm, which is the uppermost value reported so far for g-C3N4 photocatalysts (Figure 6, She et al., 2017).
Figure 6. Synthesis of hybrid nanostructures. (A) Schematic diagram of synthesis of α-Fe2O3/2D g-C3N4 hybrid structure. (B) AFM image of 2D g-C3N4. (C) SEM image of α-Fe2O3 nanosheets (hexagonal structure). Scale bar: 100 nm. (D) TEM image of α-Fe2O3 nanosheet. (E) HRTEM image of α-Fe2O3 nanosheet. (F) SEM image of the 2D g-C3N4. Scale bar: 100 nm. (G) HRTEM image of α-Fe2O3/2D g-C3N4 (3.8%) hybrid structure, Scale bar: 5 nm. (H) HRTEM image of α-Fe2O3/2D g-C3N4 (39.8%) hybrid. Scale bar: 2 nm (She et al., 2017). Copyright 2017, Wiley-VCH.
A layered-structure, MoS2, is also a candidate for incorporation with g-C3N4 to construct 2D/2D nanocomposites (Hou et al., 2013; Li X. G. et al., 2016). For example, Li et al. designed a 2D g-C3N4 and MoS2 heterojunction via means of the self-assembly of 2D g-C3N4 with MoS2 nanosheets (Li X. G. et al., 2016). As shown in Figure 7, the 2D g-C3N4 and MoS2 nanosheets were prepared from the exfoliation of bulk g-C3N4 and MoS2 raw materials, through ultrasonication (Li X. G. et al., 2016). Thin g-C3N4 and MoS2 2D nanosheets were observed in 2D g-C3N4/MoS2 nanocomposites by TEM images (Figure 7). This type of g-C3N4/ MoS2 photocatalysts also showed a good photocatalytic effect.
Figure 7. (A) Schematic diagram of 2D MoS2/g-C3N4 nanocomposite preparation. (B) TEM and (C) HRTEM images of 2D MoS2/g-C3N4 nanocomposite (Li X. G. et al., 2016). Copyright 2016, Elsevier.
In a single 2D g-C3N4 system, the photo-excited electrons of the conduction band (CB) generally return to the valence band (VB) (Tian et al., 2014), while the unpopular recovery of photo-generated electrons and holes are a great disadvantage of photocatalytic reactions (Yin et al., 2016). The photocatalyst is used as a semiconductor, to intimately constitute with g-C3N4, to create a suitable band structure. The spatial isolation of photo-generated electrons and holes can be realized through an effective charge transfer on the two semiconductor interfaces (Figure 8, Jiang et al., 2013; Liu et al., 2016; Zhang X. J. et al., 2016; Fu et al., 2017). Commonly, the bandgap of pristine g-C3N4 bandgap is about 2.7 eV and their CB and VB are situated at −1.1 eV and +1.6 eV, respectively (Cao et al., 2015). g-C3N4 is therefore used as a photocatalyst for photo-reduction reactions, because of its sufficiently negative conduction band position in Figure 8. Generally, 2D g-C3N4-based hetero-junction systems are very effective in separating photo-generated electron/hole pairs, because of the component photocatalyst has this kind of Z-Z band structures (Cao et al., 2015). Therefore, an appropriate band-structure is important to consider when choosing the component photocatalyst for the structuring of 2D g-C3N4-based heterojunction photocatalysts (Fu et al., 2017).
Figure 8. (A,B) Charge transfer in the conventional type-II g-C3N4-based heterojunction systems (Fu et al., 2017). Copyright 2017, Wiley-VCH.
Additionally, the band gap requires that the oxidation of the photo-generated hole has enough strength, in order to obtain oxygen from the oxidation of water, and the photo-generated electron must restore enough, to reduce the water, in order to yield H2 (Li et al., 2012). In other words, the location of the HOMO-LUMO band must consume the water oxidation-reduction potential (Wang et al., 2012). As illustrated in Figure 9, it is able to run half of two independent reactions, by calculating the carbon nitride band positions (Thomas et al., 2008; Maeda et al., 2014). The type of containment in an organic semiconductor is a rare condition in Figure 10 (Wang et al., 2012).
Figure 9. Density functional theory of band structure (Thomas et al., 2008). Copyright 2008, Royal Society of Chemistry.
Figure 10. Photocatalytic mechanism of g-C3N4 photocatalystt (Wang et al., 2012). Copyright 2012, American Chemical Society.
In 2016, Chen et al. fabricated a 2D/2D P-doped g-C3N4/ZnIn2S4 photocatalyst by an in situ loading method, wherein ZnIn2S4 nanosheets where grown on the P-doped mesoporous g-C3N4 nanosheet surface (Chen et al., 2016). As shown in Figure 11. the 2D nanosheet structure can clearly be observed for the P-C3N4/ZnIn2S4 nanocomposites. Moreover, the EDS mapping images of the P-C3N4/ZnIn2S4 show that all the elements (Zn, In, S, C, N, and P) are evenly dispersed on the surface of the photocatalyst (Chen et al., 2016). This type of a special 2D/2D surface contact can provide more contact areas between P-C3N4 and ZnIn2S4, which is conducive to an effective charge carrier separation. Under light irradiation, the photo-generated electrons can transfer from the CB of ZnIn2S4 to the CB of P-C3N4. Similarly, the photo-generated holes can shift from the VB of P-C3N4 to the VB of ZnIn2S4, as shown in Figure 12. The spatial isolation of photo-generated charge carriers can vastly optimize the catalytic performance of the P-C3N4/ZnIn2S4 photocatalyst (Chen et al., 2016).
Figure 11. (A,B) TEM images, and (C) EDS mapping images of P-C3N4/ZnIn2S4 nanocomposites (Chen et al., 2016). Copyright 2016, Royal Society of Chemistry.
Figure 12. Water photolysis mechanism of P-C3N4/ZnIn2S4 photocatalyst under simulated solar irradiation (Chen et al., 2016). Copyright 2016, Royal Society of Chemistry.
Currently, two-dimensional g-C3N4, a metal-free and visible-light-responsive photocatalyst, in the field of hydrogen-production through water splitting, is a hot topic in research. This critical review summarizes the ultramodern progress in the design and preparation of 2D g-C3N4 and g-C3N4-based composites. Although significant advances in 2D g-C3N4-based photocatalysts have been made, photocatalytic efficiency remains too low. However, its wide application proves that 2D g-C3N4-based photocatalysts are prospective materials in the practical application of efficient sun-energy conversion in the future.
All authors listed have made a substantial, direct and intellectual contribution to the work, and approved it for publication.
The authors declare that the research was conducted in the absence of any commercial or financial relationships that could be construed as a potential conflict of interest.
This work was supported by the National Natural Science Foundation of China (Grant No. 51802117, 51672109, 21505050, 21707043), the Natural Science Foundation of Shandong Province for Excellent Young Scholars (ZR2016JL015), and Natural Science Foundation of Shandong Province (Grant No. ZR2018BEM019, ZR2017BEE005, ZR2017PB010, ZR2016EMB16).
Ai, Z. Z., Zhao, G., Zhong, Y. Y., Shao, Y. L., Huang, B. B., Wu, Y. Z., et al. (2018). Phase junction CdS: high efficient and stable photocatalyst for hydrogen generation. Appl. Catal. B Environ. 221, 179–186. doi: 10.1016/j.apcatb.2017.09.002
Bard, A. J., and Fox, M. A. (1995). Artificial photosynthesis: solar splitting of water to hydrogen and oxygen. Acc. Chem. Res. 28, 141–145. doi: 10.1021/ar00051a007
Bojdys, M. J., Severin, N., Rabe, J. P., Cooper, A. I., Thomas, A., Antonietti, M., et al. (2013). Exfoliation of crystalline 2D carbon nitride: thin sheets, scrolls and bundles via mechanical and chemical routes. Macromol. Rapid Commun. 34, 850–854. doi: 10.1002/marc.201300086
Cao, S., Low, J., Yu, J., and Jaroniec, M. (2015). Polymeric photocatalysts based on graphitic carbon nitride. Adv. Mater. 27, 2150–2176. doi: 10.1002/adma.201500033
Chang, B., Zhao, G., Shao, Y. L., Zhang, L., Huang, B. B., Wu, Y. Z., et al. (2017). Photo-enhanced electrocatalysis of sea-urchin shaped Ni3(VO4)2 for the hydrogen evolution reaction. J. Mater. Chem. A 5, 18038–18043. doi: 10.1039/c7ta05642g
Chang, K., Li, M., Wang, T., Ouyang, S., Li, P., Liu, L., et al. (2015). Drastic layer-number-dependent activity enhancement in photocatalytic H2 evolution over nMoS2/CdS (n≥1) under visible light. Adv. Energy Mater. 5:1402279. doi: 10.1002/aenm.201402279
Chen, S. S., Qi, Y., Hisatomi, T., Ding, Q., Asai, T., Li, Z., et al. (2015). Efficient visible-light-driven z-scheme overall water splitting using a MgTa2O6-xNy /TaON heterostructure photocatalyst for H2 evolution. Angew. Chem. Int. Ed. 54, 8498–8501. doi: 10.1002/anie.201502686
Chen, W., Liu, T. Y., Huang, T., Liu, X. H., and Yang, X. J. (2016). Novel mesoporous P-doped graphitic carbon nitride nanosheets coupled with ZnIn2S4 nanosheets as efficient visible light driven heterostructures with remarkably enhanced photo-reduction activity. Nanoscale 8, 3711. doi: 10.1039/C5NR07695A
Chong, S. Y., Jones, J. T. A., Khimyak, Y. Z., Cooper, A. I., Thomas, A., Antonietti, M., et al. (2013). Tuning of gallery heights in a crystalline 2D carbon nitride network. J. Mater. Chem. A 1, 1102–1107. doi: 10.1039/C2TA01068B
Ding, G., Wang, W., Jiang, T., Han, B., Fan, H., and Yang, G. (2013). Highly selective synthesis of phenol from benzene over a vanadium-doped graphitic carbon nitride catalyst. ChemCatChem 5, 192–200. doi: 10.1002/cctc.201200502
Dong, F., Zhao, Z., Xiong, T., Ni, Z., Zhang, W., Sun, Y., et al. (2013). In situ construction of g-C3N4/g-C3N4 metal-free heterojunction for enhanced visible-light photocatalysis. ACS Appl. Mater. Interfaces 5, 11392–11401. doi: 10.1021/am403653a
Dong, X., and Cheng, F. (2015). Recent development in exfoliated two-dimensional g-C3N4 nanosheets for photocatalytic applications. J. Mater. Chem. A 2015, 3, 23642–23652. doi: 10.1039/C5TA07374J
Feng, J., Chen, T., Liu, S., Zhou, Q., Ren, Y., Lv, Y., et al. (2016). Improvement of g-C3N4 photocatalytic properties using the Hummers method. J. Colloid Interface Sci. 479, 1–6. doi: 10.1016/j.jcis.2016.06.040
Fu, J. W., Yu, J. G., Jiang, C. J., and Cheng, B. (2017). g-C3N4-based heterostructured photocatalysts. Adv. Energy Mater. 8:1701503. doi: 10.1002/aenm.201701503
Groenewolt, M., and Antonietti, M. (2005). Synthesis of g-C3N4 nanoparticles in mesoporous silica host matrices. Adv. Mater. 17, 1789–1792. doi: 10.1002/adma.200401756
Han, Q., Wang, B., Gao, J., Cheng, Z. H., Zhao, Y., Zhang, Z. P., et al. (2016). Atomically thin mesoporous nanomesh of graphitic C3N4 for high-efficiency photocatalytic hydrogen evolution. ACS Nano 10, 2745–2751. doi: 10.1021/acsnano.5b07831
He, W. D., Liang, Z. F., Ji, K. Y., Sun, Q. F., Zhai, T. Y., and Xu, X. J. (2018). Hierarchical Ni-Co-S@Ni-W-O core–shell nanosheet arrays on nickel foam for high-performance asymmetric supercapacitors. Nano Res. 11, 1415–1425. doi: 10.1007/s12274-017-1757-2
He, W. D., Wang, C. G., Li, H. Q., Deng, X. L., Xu, X. J., and Zhai, T. Y. (2017a). Ultrathin and porous Ni3S2/CoNi2S4 3D-network structure for superhigh energy density asymmetric supercapacitors. Adv. Energy Mater. 7:1700983. doi: 10.1002/aenm.201700983
He, W. D., Wang, C. G., Zhuge, F. W., Deng, X. L., Xu, X. J., and Zhai, T. Y. (2017b). Flexible and high energy density asymmetrical supercapacitors based on core/shell conducting polymer nanowires/manganese dioxide nanoflakes. Nano Energy 35, 242–250. doi: 10.1016/j.nanoen.2017.03.045
Hou, Y., Laursen, A. B., Zhang, J., Zhang, G., Zhu, Y., Wang, X., et al. (2013). Layered nanojunctions for hydrogen-evolution catalysis. Angew. Chem. Int. Ed. 52, 3621. doi: 10.1002/anie.201210294
Iwase, A., Ng, Y. H., Ishiguro, Y., Kudo, A., and Amal, R. (2011). Reduced graphene oxide as a solid-state electron mediator in Z-scheme photocatalytic water splitting under visible light. J. Am. Chem. Soc. 133, 11054–11057. doi: 10.1021/ja203296z
Jiang, D., Chen, L., Zhu, J., Chen, M., Shi, W., and Xie, J. (2013). Novel p–n heterojunction photocatalyst constructed by porous graphite-like C3N4 and nanostructured BiOI: facile synthesis and enhanced photocatalytic activity. Dalton Trans. 42, 15726. doi: 10.1039/C3DT52008K
Kang, Y. Y., Yang, Y. Q., Yin, L. C., Kang, X. D., Wang, L. Z., Liu, G., et al. (2016). Selective breaking of hydrogen bonds of layered carbon nitride towards greatly enhanced visible light photocatalysis. Adv. Mater. 28, 6471–6477. doi: 10.1002/adma.201601567
Li, G., Lian, Z., Wang, W., Zhang, D., and Li, H. (2016). Nanotube confinement induced size-controllable g-C3N4 quantum dots modified single-crystalline TiO2 nanotube arrays for stable synergetic photoelectrocatalysis. Nano Energy 19, 446–454. doi: 10.1016/j.nanoen.2015.10.011
Li, J., Liu, E., Ma, Y., Hu, X., Wan, J., Sun, L., et al. (2016). Synthesis of MoS2/g-C3N4 nanosheets as 2D heterojunction photocatalysts with enhanced visible light activity. Appl. Surf. Sci. 364, 694. doi: 10.1016/j.apsusc.2015.12.236
Li, X. G., Bi, W. T., Zhang, L., Tao, S., Chu, W. S., Zhang, Q., et al. (2016). Single-atom Pt as Co-catalyst for enhanced photocatalytic H2 evolution. Adv. Mater. 28, 2427. doi: 10.1002/adma.201505281
Li, X. H., Wang, X. C., and Antonietti, M. (2012). Solvent-free and metal-free oxidation of toluene using O2 and g-C3N4 with nanopores: nanostructure boosts the catalytic selectivity. ACS Catal. 2, 2082–2086. doi: 10.1021/cs300413x
Liang, Q., Li, Z., Huang, Z.-H., Kang, F., and Yang, Q.-H. (2015). Holey graphitic carbon nitride nanosheets with carbon vacancies for highly improved photocatalytic hydrogen production. Adv. Funct. Mater. 25, 6885–6892. doi: 10.1002/adfm.201503221
Lin, Z. Z., and Wang, X. C. (2014). Ionic liquid promoted synthesis of conjugated carbon nitride photocatalysts from urea. ChemSusChem 7, 547–1550. doi: 10.1002/cssc.201400016
Liu, G., Wang, T., Zhang, H., Meng, X., Hao, D., Chang, K., et al. (2015). Nature-inspired environmental “phosphorylation” boosts photocatalytic H2 production over carbon nitride nanosheets under visible-light irradiation. Angew. Chem. Int. Ed. 54, 13561–13565. doi: 10.1002/anie.201505802
Liu, G., Zhao, G., Zhou, W., Liu, Y., Pang, H., Zhang, H., et al. (2016). In situ bond modulation of graphitic carbon nitride to construct p–n homojunctions for enhanced photocatalytic hydrogen production. Adv. Funct. Mater. 26, 6822. doi: 10.1002/adfm.201602779
Liu, J., Li, W., Duan, L., Li, X., Ji, L., Geng, Z., et al. (2015a). A Graphene-like oxygenated carbon nitride material for improved cycle-life lithium/sulfur batteries. Nano Lett. 15, 5137–5142. doi: 10.1021/acs.nanolett.5b01919
Liu, J., Wang, H., Chen, Z. P., Moehwald, H., Fiechter, S., vande Krol, R., et al. (2015b). Microcontact-printingassisted access of graphitic carbon nitride films with favorable textures toward photoelectrochemical application. Adv. Mater. 27, 712–718. doi: 10.1002/adma.201404543
Low, J., Cao, S., Yu, J., and Wageh, S. (2014). Two-dimensional layered composite photocatalysts. Chem. Commun. 50, 10768–10777. doi: 10.1039/c4cc02553a
Lu, S., Li, C., Li, H. H., Zhao, Y. F., Gong, Y. Y., Niu, L. Y., et al. (2017). The effects of nonmetal dopants on the electronic, optical and chemical performances of monolayer g–C3N4 by first-principles study. Appl. Surf. Sci. 392, 966–974. doi: 10.1016/j.apsusc.2016.09.136
Ma, F. K., Sun, C. L., Shao, Y. L., Wu, Y. Z., Huang, B. B., and Hao, X. P. (2017). One-step exfoliation and fluorination of g-C3N4 nanosheets with enhanced photocatalytic activities. New J. Chem. 41, 3061–3067. doi: 10.1039/C7NJ00035A
Ma, F. K., Wu, Y. Z., Shao, Y. L., Zhong, Y. Y., Lv, J. X., and Hao, X. P. (2016). 0D/2D nanocomposite visible light photocatalyst for highly stable and efficient hydrogen generation via recrystallization of CdS on MoS2 nanosheets. Nano Energy 27, 466–474. doi: 10.1016/j.nanoen.2016.07.014
Ma, T. Y., Tang, Y., Dai, S., and Qiao, S. Z. (2014). Proton-functionalized two-dimensional graphitic carbon nitride nanosheet: an excellent metal-/label-free biosensing platform. Small 10, 2382–2389. doi: 10.1002/smll.201303827
Ma, Z., Sa, R., Li, Q., and Wu, K. (2016). Interfacial electronic structure and charge transfer of hybrid graphene quantum dot and graphitic carbon nitride nanocomposites: insights into high efficiency for photocatalytic solar water splitting. Phys. Chem. Chem. Phys. 18, 1050–1058. doi: 10.1039/C5CP05847C
Maeda, K., Kuriki, R., Zhang, M. W., Wang, X. C., and Ishitania, O. (2014). The effect of the pore-wall structure of carbon nitride on photocatalytic CO2 reduction under visible light. J. Mater. Chem. A 2, 15146–15151. doi: 10.1039/C4TA03128H
Martin, D. J., Qiu, K., Shevlin, S. A., Handoko, A. D., Chen, X., Guo, Z., et al. (2014a). Highly efficient photocatalytic H2 evolution from water using visible light and structure-controlled graphitic carbon nitride. Angew. Chem. Int. Ed. 53, 9240. doi: 10.1002/anie.201403375
Martin, D. J., Reardon, P. J. T., Moniz, S. J. A., and Tang, J. (2014b). Visible light-driven pure water splitting by a nature-inspired organic semiconductor-based system. J. Am. Chem. Soc. 136, 12568–12571. doi: 10.1021/ja506386e
Niu, P., Zhang, L., Liu, G., and Cheng, H. M. (2012). Graphene-like carbon nitride nanosheets for improved photocatalytic activities. Adv. Funct. Mater. 22, 4763. doi: 10.1002/adfm.201200922
Ong, W. J., Tan, L. L., Ng, Y. H., Yong, S. T., and Chai, S. P. (2016). Graphitic carbon nitride (g-C3N4)-based photocatalysts for artificial photosynthesis and environmental remediation: are we a step closer to achieving sustainability? Chem. Rev. 116, 7159–7329. doi: 10.1021/acs.chemrev.6b00075
Pan, H., Zhang, Y. W., Shenoy, V. B., and Gao, H. (2011). Ab initio study on a novel photocatalyst: functionalized graphitic carbon nitride nanotube. ACS Catal. 1, 99–104. doi: 10.1021/cs100045u
Ran, J., Ma, T. Y., Gao, G., Du, X. W., and Qiao, S. Z. (2015). Porous P-doped graphitic carbon nitride nanosheets for synergistically enhanced visible-light photocatalytic H2 production. Energy Environ. Sci. 8, 3708. doi: 10.1039/C5EE02650D
Schwinghammer, K., Tuffy, B., Mesch, M. B., Wirnhier, E., Martineau, C., Taulelle, F., et al. (2013). Triazine-based carbon nitrides for visible-light-driven hydrogen evolution. Angew. Chem. Int. Ed. 52, 2435–2439. doi: 10.1002/anie.201206817
She, X. J., Wu, J. J., Xu, H., Zhong, J., Wang, Y., Song, Y. H., Nie, K. Q., et al. (2017). High efficiency photocatalytic water splitting using 2D α-Fe2O3/g-C3N4 Z-scheme catalysts. Adv. Energy Mater. 2017:1700025. doi: 10.1002/aenm.201700025
She, X. J., Wu, J. J., Zhong, J., Xu, H., Yang, Y. C., Vajtai, R., et al. (2016). Oxygenated monolayer carbon nitride for excellent photocatalytic hydrogen evolution and external quantum efficiency. Nano Energy 27, 138–146. doi: 10.1016/j.nanoen.2016.06.042
Shi, L., Wang, T., Zhang, H., Chang, K., and Ye, J. (2015). Electrostatic self-assembly of nanosized carbon nitride nanosheet onto a zirconium metal–organic framework for enhanced photocatalytic CO2 reduction. Adv. Funct. Mater. 25, 5360–5367. doi: 10.1002/adfm.201502253
Shiraishi, Y., Kanazawa, S., Sugano, Y., Tsukamoto, D., Sakamoto, H., Ichikawa, S., et al. (2014). Highly selective production of hydrogen peroxide on graphitic carbon nitride (g-C3N4) photocatalyst activated by visible light. ACS Catal. 4, 774–780. doi: 10.1021/cs401208c
Shiraishi, Y., Kofuji, Y., Sakamoto, H., Tanaka, S., Ichikawa, S., and Hirai, T. (2015). Effects of surface defects on photocatalytic H2O2 production by mesoporous graphitic carbon nitride under visible light irradiation. ACS Catal. 5, 3058–3066. doi: 10.1021/acscatal.5b00408
Thomas, A., Fischer, A., Goettmann, F., Antonietti, M., Muller, J. O., Schlogl, R., et al. (2008). Graphitic carbon nitride materials: variation of structure and morphology and their use as metal-free catalysts. J. Mater. Chem. 18, 4893–4908. doi: 10.1039/b800274f
Tian, Y., Chang, B., Fu, J., Zhou, B., Liu, J., Xi, F., et al. (2014). Graphitic carbon nitride/Cu2O heterojunctions: preparation, characterization, and enhanced photocatalytic activity under visible light. J. Solid State Chem. 212, 1–6. doi: 10.1016/j.jssc.2014.01.011
Tonda, S., Kumar, S., Kandula, S., and Shanker, V. (2014). Fe-doped and mediated graphitic carbon nitride nanosheets for enhanced photocatalytic performance under natural sunlight. J. Mater. Chem. A 2, 6772–6780. doi: 10.1039/C3TA15358D
Tu, W., Zhou, Y., and Zou, Z. (2013). Versatile graphene-promoting photocatalytic performance of semiconductors: basic principles, synthesis, solar energy conversion, and environmental applications. Adv. Funct. Mater. 23, 4996–5008. doi: 10.1002/adfm.201203547
Wang, H., Su, Y., Zhao, H., Yu, H., Chen, S., Zhang, Y., et al. (2014). Photocatalytic oxidation of aqueous ammonia using atomic single layer graphitic-C3N4. Environ. Sci. Technol. 48, 11984. doi: 10.1021/es503073z
Wang, S. Z., Ma, F. K., Jiang, H. H., Shao, Y. L., Wu, Y. Z., and Hao, X. P. (2018). Bandgap tunable porous borocarbonitrides nanosheets for high energy-density supercapacitors. ACS Appl. Mater. Interfaces 10, 19588–19597. doi: 10.1021/acsami.8b02317
Wang, X., Chen, X., Thomas, A., Fu, X., and Antonietti, M. (2009). Metal containing carbon nitride compounds: a new functional organic–metal hybrid material. Adv. Mater. 21, 1609–1612. doi: 10.1002/adma.200802627
Wang, X. C., Blechert, S., and Antonietti, M. (2012). Polymeric graphitic carbon nitride for heterogeneous photocatalysis. ACS Catal. 2, 1596–1606. doi: 10.1021/cs300240x
Wang, X. C., Maeda, K., Thomas, A., Takanabe, K., Xin, G., Carlsson, J. M., et al. (2009). A metal-free polymeric photocatalyst for hydrogen production from water under visible light. Nat. Mater. 8, 76–80. doi: 10.1038/nmat2317
Wang, Y., Di, Y., Antonietti, M., Li, H. R., Chen, X. F., and Wang, X. C. (2010). Excellent visible-light photocatalysis of fluorinated polymeric carbon nitride solids. Chem. Mater. 22, 5119–5121. doi: 10.1021/cm1019102
Wang, Y., Li, H., Yao, J., Wang, X., and Antonietti, M. (2011). Synthesis of boron doped polymeric carbon nitride solids and their use as metal-free catalysts for aliphatic C-H bond oxidation. Chem. Sci. 2, 446–450. doi: 10.1039/C0SC00475H
Wondraczek, L., Tyystjarvi, E., Mendez-Ramos, J., Muller, F. A., and Zhang, Q. Y. (2015). Shifting the sun: solar spectral conversion and extrinsic sensitization in natural and artificial photosynthesis. Adv. Sci. 2:1500218. doi: 10.1002/advs.201500218
Xu, H. Q., Hu, J. H., Wang, D. K., Li, Z. H., Zhang, Q., Luo, Y., et al. (2015). Visible-light photoreduction of CO2 in a metal-organic framework: boosting electron-hole separation via electron trap states. J. Am. Chem. Soc. 137, 13440–13443. doi: 10.1021/jacs.5b08773
Yang, D., Jiang, T., Wu, T., Zhang, P., Han, H., and Han, B. (2016). Highly selective oxidation of cyclohexene to 2-cyclohexene-1-one in water using molecular oxygen over Fe-Co-g-C3N4. Catal. Sci. Technol. 6, 193–200. doi: 10.1039/C5CY01177A
Yang, S., Gong, Y., Zhang, J., Zhan, L., Ma, L., Fang, Z., et al. (2013). Exfoliated graphitic carbon nitride nanosheets as efficient catalysts for hydrogen evolution under visible light. Adv. Mater. 25, 2452. doi: 10.1002/adma.201204453
Ye, C., Li, J. X., Li, Z. J., Li, X. B., Fan, X. B., Zhang, L. P., et al. (2015). Enhanced driving force and charge separation efficiency of protonated g-C3N4 for photocatalytic O2 evolution. ACS Catal. 5, 6973–6979. doi: 10.1021/acscatal.5b02185
Ye, L., Wang, D., and Chen, S. (2016). Fabrication and enhanced photoelectrochemical performance of MoS2/S-doped g-C3N4 heterojunction film. ACS Appl. Mater. Interfaces 8, 5280–5289. doi: 10.1021/acsami.5b11326
Ye, X., Cui, Y., Qiu, X., and Wang, X. (2014). Selective oxidation of benzene to phenol by Fe-CN/TS-1 catalysts under visible light irradiation. Appl. Catal. B 152–153, 383–389. doi: 10.1016/j.apcatb.2014.01.050
Yin, S., Di, J., Li, M., Sun, Y., Xia, J., Xu, H., et al. (2016). Ionic liquid-assisted synthesis and improved photocatalytic activity of p-n junction g-C3N4/BiOCl. J. Mater. Sci. 51, 4769–4777. doi: 10.1007/s10853-016-9746-5
Yue, B., Li, Q., Iwai, H., Kako, T., and Ye, J. (2011). Hydrogen production using zinc-doped carbon nitride catalyst irradiated with visible light. Sci. Technol. Adv. Mater. 12, 034401. doi: 10.1088/1468-6996/12/3/034401
Zhang, G. G., Lan, Z. A., and Wang, X. C. (2016). Conjugated polymers: catalysts for photocatalytic hydrogen evolution. Angew. Chem. Int. Ed. 55, 15712–15727. doi: 10.1002/anie.201607375
Zhang, G. G., Lan, Z. A., and Wang, X. C. (2017a). Surface engineering of graphitic carbon nitride polymers with cocatalysts for photocatalytic overall water splitting. Chem. Sci. 8, 5261–5274. doi: 10.1039/C7SC01747B
Zhang, G. G., Li, G. S., Lan, Z. A., Lin, L. H., Savateev, A., Heil, T., et al. (2017b). Optimizing optical absorption, exciton dissociation, and charge transfer of a polymeric carbon nitride with ultrahigh solar hydrogen production activity. Angew. Chem. Int. Ed. 56, 13445–13449. doi: 10.1002/anie.201706870
Zhang, G. G., Lin, L. H., Li, G. S., Zhang, Y. F., Savateev, A., Wang, X. C., et al. (2018). Ionothermal synthesis of triazine-heptazine based coframeworks with apparent quantum yields of 60 % at 420 nm for solar hydrogen production from “sea water”. Angew. Chem. Int. Ed. 57, 9372–9376. doi: 10.1002/anie.201804702
Zhang, G. G., Zang, S. H., and Wang, X. C. (2015). Layered Co(OH)2 Deposited polymeric carbon nitrides for photocatalytic water oxidation. ACS Catal. 5, 941–947. doi: 10.1021/cs502002u
Zhang, G. G., Zhang, M. W., Ye, X. X., Qiu, X. Q., Lin, S., and Wang, X. C. (2014). Iodine modified carbon nitride semiconductors as visible light photocatalysts for hydrogen evolution. Adv. Mater. 26, 805–809. doi: 10.1002/adma.201303611
Zhang, J., Chen, X., Takanabe, K., Maeda, K., Domen, K., Epping, J. D., et al. (2010). Synthesis of a carbon nitride structure for visible-light catalysis by copolymerization. Angew. Chem. Int. Ed. 49, 441–444. doi: 10.1002/anie.200903886
Zhang, J., Zhang, G., Chen, X., Lin, S., Möhlmann, L., Dołega, G., et al. (2012). Co-monomer control of carbon nitride semiconductors to optimize hydrogen evolution with visible light. Angew. Chem. Int. Ed. 51, 3183–3187. doi: 10.1002/anie.201106656
Zhang, J. S., Zhang, M. W., Zhang, G. G., and Wang, X. C. (2012). Synthesis of carbon nitride semiconductors in sulfur flux for water photoredox catalysis. ACS Catal. 2, 940–948. doi: 10.1021/cs300167b
Zhang, M., Jiang, W., Liu, D., Wang, J., Liu, Y., Zhu, Y., et al. (2016). Photodegradation of phenol via C3N4-agar hybrid hydrogel 3D photocatalysts with free separation. Appl. Catal. B 183, 263–268. doi: 10.1016/j.apcatb.2015.10.049
Zhang, N., Yang, M.-Q., Liu, S., Sun, Y., and Xu, Y.-J. (2015). Waltzing with the versatile platform of graphene to synthesize composite photocatalysts. Chem. Rev. 115, 10307–10377. doi: 10.1021/acs.chemrev.5b00267
Zhang, S. W., Gao, H. H., Huang, Y. S., Wang, X. X., Hayat, T., Li, J. X., et al. (2018). Ultrathin g-C3N4 nanosheets coupled with amorphous Cu-doped FeOOH nanoclusters as 2D/0D heterogeneous catalysts for water remediation. Environ. Sci. Nano 5, 1179–1190. doi: 10.1039/c8en00124
Zhang, X. J., Wang, L., Du, Q. C., Wang, Z. Y., Ma, S. G., and Yu, M. (2016). Photocatalytic CO2 reduction over B4C/C3N4 with internal electric field under visible light irradiation. J. Colloid Interface Sci. 464, 89. doi: 10.1016/j.jcis.2015.11.022
Zhao, G., Cheng, Y. L., Wu, Y. Z., Xu, X. J., and Hao, X. P. (2018). New 2D Carbon Nitride Organic Materials Synthesis with Huge-Application Prospects in CN Photocatalyst. Small 2018:1704138. doi: 10.1002/smll.201704138
Zheng, Y., Lin, L. H., Wang, B., and Wang, X. C. (2015). Graphitic carbon nitride polymers toward sustainable photoredox catalysis. Angew. Chem. Int. Ed. 54, 12868 −12884. doi: 10.1002/anie.201501788
Zhong, Y. Y., Zhao, G., Ma, F. K., Wu, Y. Z., and Hao, X. P. (2016). Utilizing photocorrosion-recrystallization to prepare a highly stable and efficient CdS/WS2 nanocomposite photocatalyst for hydrogen evolution. Appl. Catal. B Environ. 199, 466–472. doi: 10.1016/j.apcatb.2016.06.065
Zhu, B., Zhang, J., Jiang, C., Cheng, B., and Yu, J. (2017). First principle investigation of halogen-doped monolayer g-C3N4 photocatalyst. Appl. Catal. B 207, 27. doi: 10.1016/j.apcatb.2017.02.020
Zhu, J., Wei, Y., Chen, W., Zhao, Z., and Thomas, A. (2010). Graphitic carbon nitride as a metal-free catalyst for NO decomposition. Chem. Commun. 46, 6965–6967. doi: 10.1039/C0CC01432J
Keywords: two-dimensional g-C3N4, metal-free photocatalysts, atom doping, modification, heterojunction
Citation: Zhao G, Yang H, Liu M and Xu X (2018) Metal-Free Graphitic Carbon Nitride Photocatalyst Goes Into Two-Dimensional Time. Front. Chem. 6:551. doi: 10.3389/fchem.2018.00551
Received: 16 July 2018; Accepted: 25 October 2018;
Published: 10 December 2018.
Edited by:
Junguang Tao, Hebei University of Technology, ChinaReviewed by:
Chunhai Jiang, Xiamen University of Technology, ChinaCopyright © 2018 Zhao, Yang, Liu and Xu. This is an open-access article distributed under the terms of the Creative Commons Attribution License (CC BY). The use, distribution or reproduction in other forums is permitted, provided the original author(s) and the copyright owner(s) are credited and that the original publication in this journal is cited, in accordance with accepted academic practice. No use, distribution or reproduction is permitted which does not comply with these terms.
*Correspondence: Gang Zhao, c3BzX3poYW9nQHVqbi5lZHUuY24=
Xijin Xu, c3BzX3h1eGpAdWpuLmVkdS5jbg==
Disclaimer: All claims expressed in this article are solely those of the authors and do not necessarily represent those of their affiliated organizations, or those of the publisher, the editors and the reviewers. Any product that may be evaluated in this article or claim that may be made by its manufacturer is not guaranteed or endorsed by the publisher.
Research integrity at Frontiers
Learn more about the work of our research integrity team to safeguard the quality of each article we publish.