- Institute of Low Temperatures and Structure Research PAS, Wrocław, Poland
A new approach to enhance the sensitivity of transition metal ion based nanocrystalline luminescent thermometer is presented. It was shown that the increase of Cr3+ concentration in three types of garnet host namely Y3Al5O12, Y3Ga5O12, and Y3Al2Ga3O12 allows for significant enhancement of their performance in non-contact thermometry. This phenomenon is related to the weakening of the crystal field strength due to enlargement of average Cr3+-O2− distance at higher Cr3+ concentrations. By increasing Cr3+ concentration from 0.6 to 30%, the sensitivity increased by over one order of magnitude from S = 0.2%/°C to S = 2.2%/°C at 9°C in Y3Al2Ga3O12 nanocrystals. Moreover, it was found that due to the Cr3+ → Nd3+ energy transfer in the Cr3+, Nd3+ co-doped system, the usable Cr3+ concentration, for which its emission can be detected, is limited to 10% while the sensitivity at −50°C was doubled (from 1.3%/°C for Y3Al2Ga3O12:10%Cr3+ to 2.2%/°C Y3Al2Ga3O12:10%Cr3+, 1%Nd3+ nanocrystals).
Introduction
In response to the requirements imposed by technology, micro/nanoelectronics or photonics as well as by biomedical applications, new approaches to the luminescent nanothermometers (LNTs) have to be proposed to secure fast and accurate temperature sensing with submicrometer spatial resolution, and highly sensitive temperature readout (Brites et al., 2012; Jaque and Vetrone, 2012; Chen et al., 2016; del Rosal et al., 2016a,b; Dramićanin, 2016; Marciniak et al., 2016b; Suo et al., 2017; Wang et al., 2017; Gao et al., 2018; Liao et al., 2018; Liu et al., 2018; Malysa et al., 2018; Runowski et al., 2018; Zhong et al., 2018). One of the most promising one, relies on exploiting transition metal (TM) ions, whose highly temperature dependent emission is referred to emission of barely temperature dependent lanthanides ions (Marciniak et al., 2017a; Drabik et al., 2018; Elzbieciak et al., 2018; Kniec and Marciniak, 2018; Marciniak and Trejgis, 2018; Trejgis and Marciniak, 2018). Materials which could be applied as real time temperature sensors in biomedicine, must also accomplish some other important requirements like sufficient sensitivity to temperature changes, high stability, low cytotoxicity (Brites et al., 2012; Jaque and Vetrone, 2012; Benayas et al., 2015) and operation in spectral range of optical transparency windows of biological tissues (Anderson and Parrish, 1981; Jaque and Jacinto, 2016). Because the transition metal ions based luminescent nanothermometers meet abovementioned flagship demands, they can be considered as distinctively attractive research area. However, in order to be applicable, the thorough understanding of the correlation between structure of the host material and the thermal quenching of luminescence has to be studied. In our previous work (Elzbieciak et al., 2018), we have shown that by appropriate adjustment of the stoichiometry of the host matrix, relative sensitivity of Cr3+ ions-based luminescent thermometer can be intentionally modulated. The presented tuning occurred as a result of modification of the metal-to-oxygen ionic distance, which modified the strength of the crystal field (CF) (Struve and Huber, 2000; Xu et al., 2017). Therefore, taking advantage from the fact that CF influences the position of 4T2 parabola, the activation energy, responsible for determination of thermal stability of luminescence, can be deliberately reduced. Efficient thermal quenching of the luminescence intensity is beneficial for sensitive luminescent thermometry. As it was already presented (Marciniak and Bednarkiewicz, 2016; Marciniak et al., 2016a, 2017b; Azkargorta et al., 2017), significant changes of the CF strength may also result from rising the concentration of active or passive dopants. Recently, Deren et al. (2012) showed that the increase of the Cr3+ concentration in Y3Ga5O12:Cr3+ causes the diminishment of 2E → 4A2 narrow emission band and enhancement of 4T2→4A2 band's intensity. Lowering the CF strength by growing number of Cr3+ ions, which is related with the elongation of the M-O distance, should relevantly enhance the relative sensitivity to temperature changes of such Cr3+ based luminescent thermometer. These observations motivated us to verify this hypothesis through comprehensive investigations of the impact of Cr3+ concentration (0.01–50%) on the temperature sensing capability in Y3Al5O12, Y3Al2Ga3O12 and Y3Ga5O12 nanocrystals.
Experimental
Nanopowders of (0.1; 0.5; 2; 5; 10; 20; 50%) Cr3+ and (0.1; 0.5; 2; 5; 10; 20; 50%) Cr3+,1% Nd3+ doped Y3Al5O12, Y3Ga5O12 and (0.06; 0.3; 1.2; 3; 6; 9; 12; 30%) Cr3+, (0.06; 0.3; 1.2; 3; 6; 9; 12; 30%) Cr3+, 1%Nd3+ doped Y3Al2Ga3O12 garnets were synthesized by the modified Pechini method (Pechini, 1967). Whole synthesis procedure was analogous as described in our earlier work (Elzbieciak et al., 2018). In brief, in order to obtain metal nitrates, calculated amount of yttrium oxide and neodymium oxide were dissolved in deionized water with addition of ultrapure nitric acid. After triple recrystallization process, yttrium or yttrium and neodymium nitrates were mixed together with aluminum, gallium and chromium nitrates. Afterwards, aqueous solution of citric acid and PEG was added to the mixture, than it was stirred for 3 h. In order to form a resin the obtained solution was heated for 1 week at 90°C.
All the synthesized materials were annealed for 16 h at 850°C. The following starting materials were used for synthesis: yttrium oxide (Y2O3 with 99.995% purity from Stanford Materials Corporation), neodymium oxide (Nd2O3 with 99.998% purity from Stanford Materials Corporation), aluminum nitrate nonahydrate (Al(NO3)3·9H2O Puratronic 99.999% purity from Alfa Aesar), gallium(III) nitrate nonahydrate (Ga(NO3)3·9H2O Puratronic 99.999% purity from Alfa Aesar), chromium nitrate nonahydrate (Cr(NO3)3·9H2O, 99.99% purity from Alfa Aesar), citric acid (C6H8O7 with 99.5+% purity from Alfa Aesar) and poly(ethylene glycol) (PEG C2H6O2 BioUltra 200 from Sigma).
All of the obtained materials were examined by XRD (X-ray diffraction) measurements carried out on PANalitycal X'Pert diffractometer, equipped with an Anton Paar TCU 1000 N temperature control unit, using Ni-filtered Cu-Kα radiation (V = 40 kV, I = 30 mA). Transmission electron microscope (TEM) images were taken using FEI TECNAI G2 X-TWIN microscope equipped with EDS detector. Powders were dispersed in methanol solution in ultrasounds and applied for lacey type copper lattices. The studies were performed in conventional TEM microscope with 300 keV parallel beam electron energy. Images were digitally recorded using the Gatan Ultrascan 1000XP.
Excitation spectra were measured using FLS980 Fluorescence Spectrometer form Edinburgh Instruments. Temperature dependent emission spectra were measured using 450 nm excitation line from laser diode and recorded using a Silver-Nova Super Range TEC Spectrometer from Stellarnet of 1 nm spectral resolution. Temperature during the measurement was controlled using the THMS 600 heating stage from Linkam (0.1°C stability and 0.1°C set point resolution).
Results and Discussion
The yttrium aluminum/gallium garnets, crystallize in cubic structure with Ia3-d space group. As it is known, structures of these materials (A3B2C3O12) provide three types of cation sites, namely dodecahedral Y3+ site and also octahedral (B) and tetrahedral (C) Al3+/Ga3+ sites which, because of similarity in ionic radii and the same coordination number, could be occupied by lanthanide Ln3+ (A) and transition metal ions TM3+ (B, C), respectively. Representative XRD patterns of Y3Al2Ga3O12: Cr3+ nanocrystals with different chromium concentration are presented in Figure 1a. All the reflection peaks correspond to the reference patterns confirming phase purity of the synthesized materials even for high dopant concentration (see also Supporting Information, Figures S1–S5). It is worth noting that in case of YAG (Figures S2, S3) and YGG (Figures S4, S5) with doping above 20% of Cr3+ ions, additional peaks occur in the XRD pattern. The cell parameter, as it can be seen in Figure 1b, is strongly affected by the Cr3+ concentration. In the case of YAG:Cr3+, parameter a increases from 11.99 Å for 0.1%Cr3+ to 12.16 Å for 50%Cr3+. On the other hand for YGG:Cr3+ an opposite tendency can be found –the a parameter decreases from 12.09 Å for 0.1%Cr3+ to 12.05 Å for 50%Cr3+. This is due to differences in ionic radius between host ions Al3+ (67.5 pm) and Ga3+(76 pm) ions being substituted by a larger dopant Cr3+ ions (75.5 pm). When atom with shorter ionic radius is substituted by atom with longer one, as in the case of Y3Al5O12, the volume of the unit cell increases, due to the local expansion of the structure. This was also confirmed by the increase of the microstrains in the YAG structure (calculated using Rietveld refinement, Figure S7). On the other hand, in the case of substitution of larger host ion with a smaller dopant ion, the parameter a and microstrains decrease likewise in the Y3Ga5O12. The reduction of the cell parameter for increasing Cr3+ concentration observed in YAGG, is therefore a direct confirmation that Cr3+ ions substitute octahedral sites of Ga3+ ions. The average grain size of the nanocrystals, calculated using Rietveld refinement technique (around 60 nm), was in agreement with the nanoparticle size distribution determined from TEM images-70 ± 10 nm (Figures 1c,d, Figure S6). TEM images revealed good crystallization and some agglomeration of the obtained powders.
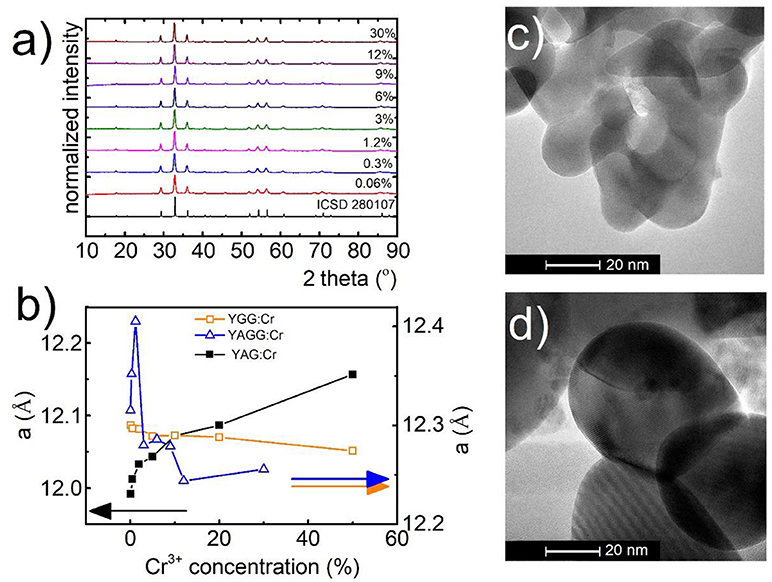
Figure 1. Structural and morphological characterization of synthesized materials: XRD spectra for Y3Al2Ga3O12 (a) with different concentrations of Cr3+ ions, dependence of unit cell parameter (a) of YGG, YAG, and YAGG on Cr3+ concentration (b); representative TEM images for Y3Al2Ga3O12:0.06%Cr3+,1%Nd3+ (c) and Y3Al2Ga3O12:30%Cr3+,1%Nd3+ (d) nanocrystals.
The simplified configurational coordinates diagram of Cr3+ is presented in Figure 2A. The luminescence of Cr3+ ions occurs through radiative depopulation of 2E and/or 4T2 states to the 4A2 ground state. Due to the fact that strength of the crystal field determines the emission of Cr3+ ions, sharp emission line corresponding to the 2E → 4A2 transition and broadband emission corresponding to the 4T2→4A2 transition can be observed in the emission spectra of Cr3+ ion in strong and weak crystal field, respectively. At higher temperatures, when the thermal energy is sufficient to reach the intersection point between the 2E parabola and 4T2 or 4A2 parabolas, the process of nonradiative, multiphonon relaxation results in lowering of their emission intensity. Analysis of the emission spectra of Y3Al2Ga3O12:Cr3+ for different dopant concentration obtained upon 450 nm excitation, clearly indicates that at higher Cr3+ concentration the broad 4T2→4A2 emission band localized at around 870 nm increase its intensity in respect to the 2E → 4A2 band at 692 nm (Figure 2B). Obviously, the total emission intensity decreases at higher dopant concentration due to the concentration quenching of luminescence. Nevertheless, it can be distinctly seen that 4T2→4A2 dominates in the spectra for 30% of Cr3+ ions. Similar observation can be done in the emission spectra of Y3Al5O12:Cr3+ and Y3Ga5O12:Cr3+ nanocrystals presented in Figures S8, S9, respectively. However, in these cases similar trends can be observed for lower Cr3+ concentrations and to a lesser extent also in Y3Al2Ga3O12. Two main consequences of the lowering of 4T2 state parabola can be found. First, at low dopant concentration the gradual reduction of its energy facilitates thermal depopulation of 2E state–lowered ΔE2 energy (the consequence of the intersection point between 2E and 4T2 parabolas). Secondly, at higher dopant concentration, when the energy 4T2 state becomes lower than 2E one, the broadband emission appears. The characteristic red-shift of the Cr3+ absorption bands localized around 400 nm and 575 nm, which can be attributed to the 4A2→4T1 and 4A2→4T2 electronic transitions, respectively, is a result of lowering of the CF strength (Figures S12–S14). In order to quantify the observed change of the crystal field strength in the examined nanocrystals, the Dq/B parameter was calculated for each of the samples as follows (Casalboni et al., 1994):
where x could be defined as (Casalboni et al., 1994):
As it can be observed in Figure 2C the Dq/B gradually decreases with Cr3+ concentration from 2.79 to 2.44 for Y3Al5O12; from 2.88 to 2.55 for Y3Ga5O12 and from 3.42 to 2.76 for Y3Al2Ga3O12 nanocrystals. In order to obtain self-referenced luminescent thermometer based of the Cr3+ emission intensity the examined nanocrystals with different Cr3+ concentration were co-doped with 1% Nd3+ ions. Emission intensity of Nd3+ is expected to be significantly less temperature dependent in respect to the chromium emission. Moreover, the Nd3+ ions emit in the first (band around 880 nm attributed to the 4F3/2→4I9/2 electronic transition) and the second (bands around 1064 nm and 1350 nm attributed to the 4F3/2→4I11/2 and 4F3/2→4I13/2 electronic transitions, respectively) optical window of the biological tissues making it well suited for biological applications.
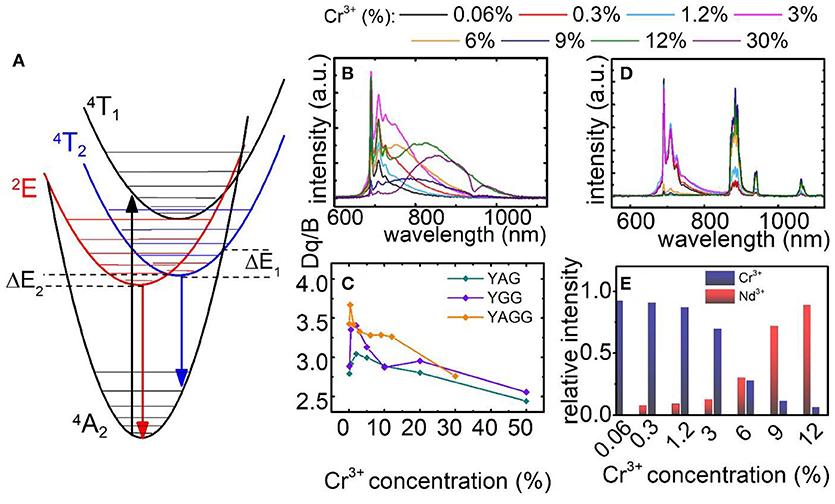
Figure 2. Configurational coordinates diagram of Cr3+ ions (A); comparison of normalized emission spectra for Y3Al2Ga3O12: xCr3+ (B) and for Y3Al2Ga3O12: xCr3+, 1%Nd3+ nanocrystals (D); dependence of Dq/B parameter (C) and Cr3+/Nd3+ ratio at T = −150°C (E) on Cr3+ concentration.
Following excitation spectra, a 450 nm excitation line was chosen, which provides the condition of direct excitation of each individual optically active ion-Cr3+ (4A2→4T1) and independently Nd3+ (4I9/2→2G5/2). As it was recently showed, this is an important condition to enhance the relative sensitivity of this kind of LTs (Marciniak et al., 2017a). In case of Y3Al2Ga3O12: Cr3+, Nd3+ (Figure 2D) nanocrystals the presence of Nd3+ ion significantly quenched the Cr3+ emission intensity due to the Cr3+ → Nd3+ energy transfer. Therefore, the 2E → 4A2 emission band can be barely seen for Y3Al2Ga3O12: 30%Cr3+, 1%Nd3+ while in the case of Y3Al5O12 and Y3Ga5O12 above 10% of Cr3+, no chromium emission was detected (Figures S10, S11). Moreover, the presence of broad Cr3+ absorption bands in the excitation spectra when monitoring Nd3+ emission (4F3/2→4I9/2 emission band), is an additional confirmation of the interionic energy transfer which takes place between dopants (Figures S12–S14). The change of contribution of the emission of particular optically active ions in the total emission intensity related with Cr3+ concentration is presented in Figure 2E. Initially, at low Cr3+ amount the 2E → 4A2 emission band dominates in the spectra. However, around 6% of Cr3+ its emission intensity equalize with Nd3+ amount. Above this value, the chromium emission intensity rapidly decreases. Therefore, this energy transfer strongly limits the usable Cr3+ concentration which can be applied for luminescent thermometry.
To understand the role of Cr3+ on the luminescence thermal quenching in the Y3Al5O12 and Y3Ga5O12 and Y3Al2Ga3O12 nanocrystals, their emission spectra were measured in a wide temperature range. The representative thermal evolution spectrum of Y3Al2Ga3O12:1.2%Cr3+ nanocrystals is presented in Figure 3A. It is clearly seen that sharp R-line of the 2E → 4A2 emission band is rapidly quenched at around 50°C in contrary to the 4T2→4A2 emission. Therefore, due to this difference in the rates of thermal quenching of these particular emission bands, their luminescence intensity ratio was chosen as a temperature sensor LIR1:
At low Cr3+ concentration the LIR1 decreases with temperature due to the fact that 2E state population feeds the 4T2 state at higher thermal energy. However, for higher Cr3+ (above 6%) different tendency can be found. Initially the LIR1 increases up to temperatures around 100°C above which saturation of its value can be found. This effect occurs because 4T2→4A2 band's intensity starts to play an important role in the total emission intensity. Due to the strong electron-phonon coupling, this emission band is expected to be efficiently reduced by the temperature. Therefore, its much higher rate of thermal quenching in respect to the 2E → 4A2 results in the enhancement of LIR1 value. To quantitative describe the observed changes, relative sensitivity (S) of LIR1-based luminescent thermometer was calculated according to the following formula:
Independently from the Cr3+ concentration, the relative intensities reach maximal value at temperatures below 100°C. Above this value, low changes of LIR1 are manifested as a minor value of S (Figure 3C). It is clearly seen that, according to our expectation, S significantly increases proportionally to Cr3+ content. The Smax at 9°C increases from 0.2%/°C for Y3Al2Ga3O12: 0.06%Cr3+ to 2.2%/°C for Y3Al2Ga3O12: 30%Cr3+ nanocrystals. Analogous tendency was found for the Y3Al5O12:Cr3+ and Y3Ga5O12:Cr3+ where for 10% Cr3+, Smax equals to 2.7%/°C at −105°C and 2%/°C at −78°C respectively (Figures S15, S16). The observed enhancement of the rate of the thermal quenching is obviously caused by the lowering of the CF strength. The optically active ions in the heavily Cr3+-doped nanocrystals are located in the lower CF sites (Figure 3D) which reduce the activation energy and facilitate luminescence thermal quenching. Therefore, the highest S were found for high Cr3+ concentration (low Dq/B values). However, above 130°C this correlation is suppressed due to the fact that above this temperature no 2E → 4A2 emission was observed. It is worth noting that the temperature range of high S overlaps with physiological temperature range (10–50°C) what indicates the importance of these LTs for biomedical applications.
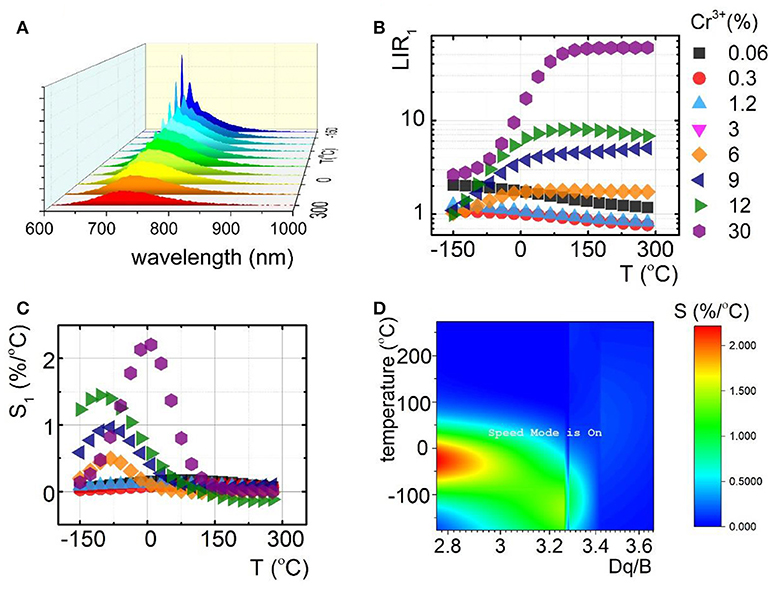
Figure 3. Comparison of thermal evolution of emission spectra for Y3Al2Ga3O12:1.2%Cr3+ (A); evolution of LIR1 (B) with the corresponding relative sensitivities (S1) (C) in the function of temperature; sensitivity map for different temperatures depending on Dq/B (D).
Due to the fact that emission intensity of both 2E → 4A2 and 4T2→4A2 bands decreases at higher temperatures, relative sensitivity of luminescent thermometer based on their intensity ratio is reduced. Therefore, Nd3+ co-dopant, whose emission intensity is expected to be less temperature dependent, were used as a luminescent reference. The luminescent properties of Nd3+, Cr3+ co-doped nanocrystals were investigated in the analogous temperature range as in the case of singly Cr3+ doped counterparts. Representative thermal evolution of Y3Al2Ga3O12: 1.2%Cr3+, 1%Nd3+ nanocrystals is presented in Figure 4a. According to the expectations the intensity of bands at 880 and 1,060 nm attributed to 4F3/2→4I9/2 and 4F3/2→4I11/2 electronic transition of Nd3+ ions, respectively, is almost independent on the temperature, while the 2E → 4A2 emission intensity is strongly thermally quenched. It is worth noting that at temperatures above 50°C, additional Nd3+ bands appears which can be attributed to the 4F5/2, 4S3/2→4I9/2 electronic transition. This band occurs at higher temperature due to the fact that population of 4F5/2, 4S3/2 states increases in respect to the 4F3/2 with temperatures according to Boltzmann population. Taking advantage from these changes of the emission spectra with temperature, two types of luminescent thermometers based on the Nd3+/Cr3+ luminescence intensity ratio have been defined as follows:
The thermal dependence of LIR2 and LIR3 for different concentration of Cr3+ ions are presented in Figures 4b,d, respectively. Obviously, due to the Cr3+ → Nd3+ energy transfer the usable concentration range of Cr3+ is strongly limited (to 12% Cr3+). Independently from dopant concentration, both LIR2 and LIR3 increase at higher temperature due to the thermal quenching of 4T2→4A2 band of Cr3+ (Figures 4b,d). However, more rapid changes can be found for LIR3 what is obviously related with the increase of the 4F5/2, 4S3/2→4I9/2 emission band of Nd3+. Both LIR2 and LIR3 are strongly modulated by the Cr3+ concentration. In agreement with the results obtained for singly Cr3+ doped systems, significant enhancement of LIR's thermal changes can be found. At heavily doped phosphors the activation energies of 4T2 level is meaningfully diminished facilitating its nonradiative depopulation. Small activation energy is beneficial for enhancement of the relative sensitivity of luminescent thermometers. Therefore, these effects substantially affect the relative sensitivity of both S2 and S3 (Figures 4c,e). The highest S2 at 150°C increases from 0.17%/°C for 0.06% of Cr3+ to 1.17%/°C for 12% of Cr3+ ions. On the other hand the S3 at −48°C increases from 0.95%/°C for 0.06% of Cr3+ to 2.16%/°C for 12% of Cr3+ ions. Comparing these results with the Figure 3, evident profitable effect of the Nd3+ ions use as a luminescent reference can be found. The relative sensitivity for 0.06% Cr3+ increases from S1 = 0.14%/°C to S2 = 0.17%/°C and S3 = 0.95%/°C, while for 12% Cr3+ from S1 = 1.37%/°C to S2 = 1.17%/°C and S3 = 2.16%/°C. Analogous beneficial effect of high Cr3+ concentration can be found for Y3Ga5O12:Cr3+, Nd3+ as well as Y3Al5O12:Cr3+, Nd3+ (Figures S10, S11). It is also worth noting that the LIR2 reveals high sensitivity at high temperature range (above 100°C) in contrast to LIR3, which unveils good performance for non-contact temperature sensing at low temperatures (below 0°C). Therefore, by simultaneous employment of both of these thermometers the temperature range where high accuracy temperature readout can be achieved, is widened.
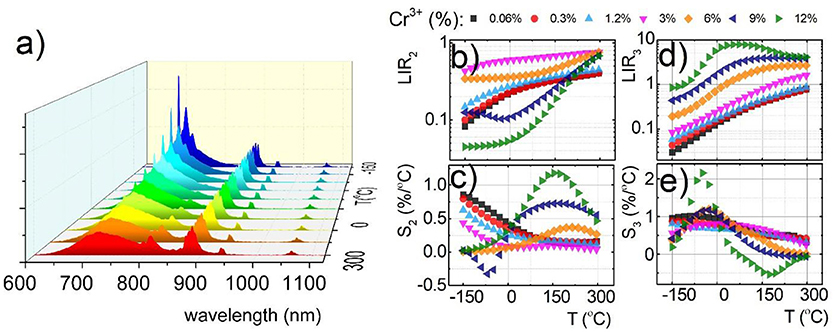
Figure 4. Comparison of thermal evolution of emission spectra for Y3Al2Ga3O12:1.2%Cr3+, 1%Nd3+ (a); thermal evolution of LIR2 (b); and LIR3 (d) and the corresponding relative sensitivities S2 (c) and S3 (e) for different Cr3+ concentration for Y3Al2Ga3O12:1.2%Cr3+, 1%Nd3+ nanocrystals.
The highest recorded sensitivity (S = 2.64%/°C) was found for YAG nanocrystals at −100°C for 10% of Cr3+ (Figure S15B) due to the fact of the lowest CF strength for this host material. Nevertheless, CF strength was tuned in the widest range for Y3Al2Ga3O12: Cr3+, Nd3+ via the Cr3+ doping the enhancement of S3 was the strongest in this case. The most important finding presented in this paper is that highly sensitive luminescent thermometers can be intentionally designed by the modification of the CF strength through elongation of Cr3+-O2− distance and enlargement of the Cr3+ concentration.
Conclusions
In this work, we proposed a new strategy to modulate the relative thermal sensitivity of Cr3+ doped nanophosphors. We considered three types of garnet matrices doped with different Cr3+ concentrations, with or without Nd3+ co-dopant. It was shown that the increase of the Cr3+ concentration causes elongation of the average Cr3+-O2− distance leading to the reduction of the crystal field strength in Y3Al2Ga3O12, Y3Ga5O12 and Y3Al5O12 nanocrystals. A gradual increase of the broad emission band attributed to the 4T2→4A2 spin allowed transition of Cr3+ is observed. Moreover, the reduction of the 4T2 state energy facilitates thermal quenching of 2E state. Therefore, the relative sensitivity of 4T2→4A2 to 2E → 4A2 emission intensity increases from S = 0.2%/°C for 0.06%Cr3+ to S = 2.2%/°C for 30%Cr3+ at 9°C in Y3Al2Ga3O12 nanocrystals, from S = 0.027%/°C for 0.5%Cr3+ to S = 2.7% for 10%Cr3+ at −105°C in Y3Al5O12 nanocrystals, and from S = 0.14%/°C for 0.5%Cr3+ to S = 2% for 10%Cr3+ at −78°C in Y3Ga5O12 nanocrystals. In the case of Nd3+ co-doped nanocrystals, due to the Cr3+ → Nd3+ energy transfer, the usable concentration range of Cr3+ dopants is strongly reduced and no evidence of Cr3+ emission was found above 10% of Cr3+. However, by taking advantage from this energy transfer and the fact that 4F5/2, 4S3/2→4I9/2 emission intensity increases proportionally to the temperature according to the Boltzmann distribution, the relative sensitivities of luminescent thermometers defined as 4F5/2, 4S3/2→4I9/2 to 4T2→4A2 luminescence intensity ratio enhances from 1.3%/°C at −50°C without Nd3+ dopant to 2.2%/°C at −50°C for nanocrystals doped with Nd3+ ions Y3Al2Ga3O12: 10%Cr3+, Nd3+). The presented results confirm that the relative sensitivity of luminescent thermometers can be effectively modulated by the dopant concentration. Moreover, it was proved that the presence of Nd3+ dopant contributes to faster quenching of Cr3+ luminescence, what is favorable for highly sensitive non-contact luminescent thermometers. Our studies may be considered as a next step toward intentional designing of nanocrystalline luminescent thermometers with fully controllable thermooptical response.
Author Contributions
All authors listed have made a substantial, direct and intellectual contribution to the work, and approved it for publication.
Conflict of Interest Statement
The authors declare that the research was conducted in the absence of any commercial or financial relationships that could be construed as a potential conflict of interest.
Acknowledgments
The High sensitive thermal imaging for biomedical and microelectronic application project is carried out within the First Team programme of the Foundation for Polish Science co-financed by the European Union under the European Regional Development Fund.
Supplementary Material
The Supplementary Material for this article can be found online at: https://www.frontiersin.org/articles/10.3389/fchem.2018.00424/full#supplementary-material
References
Anderson, R. R., and Parrish, J. A. (1981). The optics of human skin. J. Invest. Dermatol. 77, 13–19. doi: 10.1111/1523-1747.ep12479191
Azkargorta, J., Marciniak, L., Iparraguirre, I., Balda, R., Strek, W., Barredo-Zuriarrain, M., et al. (2017). Influence of grain size and Nd3+concentration on the stimulated emission of LiLa1-xNdxP4O12crystal powders. Opt. Mater. (Amst). 63, 46–50. doi: 10.1016/j.optmat.2016.07.016
Benayas, A., del Rosal, B., Pérez-Delgado, A., Santacruz-Gómez, K., Jaque, D., Alonso Hirata, G., et al. (2015). Nd:YAG Near-Infrared Luminescent Nanothermometers. Adv. Opt. Mater. 3, 687–694. doi: 10.1002/adom.201400484
Brites, C. D., Lima, P. P., Silva, N. J., Millán, A., Amaral, V. S., Palacio, F., et al. (2012). Thermometry at the nanoscale. Nanoscale. 4:4799. doi: 10.1039/c2nr30663h
Casalboni, M., Luci, A., and Grassano, U. M. (1994). Optical spectroscopy of La3Ga5SiO14: Cr3+ crystals. Phys. Rev. B. 49, 3781–3790. doi: 10.1103/PhysRevB.49.3781
Chen, D., Liu, S., Zhou, Y., Wan, Z., Huang, P., and Ji, Z. (2016). Dual-activator luminescence of RE/TM:Y3Al5O12(RE = Eu3+, Tb3+, Dy3+; TM = Mn4+, Cr3+) phosphors for self-referencing optical thermometry. J. Mater. Chem. C. 4, 9044–9051. doi: 10.1039/C6TC02934E
del Rosal, B., Carrasco, E., Ren, F., Benayas, A., Vetrone, F., Sanz-Rodríguez, F., et al. (2016a). Infrared-emitting QDs for thermal therapy with real-time subcutaneous temperature feedback. Adv. Funct. Mater. 26, 6060–6068. doi: 10.1002/adfm.201601953
del Rosal, B., Pérez-Delgado, A., Carrasco, E., Jovanović, D. J., Dramićanin, M. D., DraŽić, G., et al. (2016b). Neodymium-based stoichiometric ultrasmall nanoparticles for multifunctional deep-tissue photothermal therapy. Adv. Opt. Mater. 4, 782–789. doi: 10.1002/adom.201500726
Deren, P. J., Watras, A., Gagor, A., and Pazik, R. (2012). Weak crystal field in yttrium gallium garnet (YGG) submicrocrystals doped with Cr3+. Cryst. Growth Des. 12, 4752–4757. doi: 10.1021/cg300435t
Drabik, J., Cichy, B., and Marciniak, L. (2018). A New Type of Nanocrystalline Luminescent Thermometers Based on Ti/Ti and Ti/Ln ( Ln = Nd, Eu, Dy) Luminescence Intensity Ratio. J. Phys. Chem. C 122, 14928–14936. doi: 10.1021/acs.jpcc.8b02328
Dramićanin, M. D. (2016). Sensing temperature via downshifting emissions of lanthanide-doped metal oxides and salts. A review. Methods Appl. Fluoresc. 4:042001. doi: 10.1088/2050-6120/4/4/042001
Elzbieciak, K., Bednarkiewicz, A., and Marciniak, L. (2018). Temperature sensitivity modulation through crystal field engineering in Ga3+ co-doped Gd3Al5-xGaxO12: Cr3+, Nd3+ nanothermometers. Sens. Actuat. B Chem. 269, 96–102. doi: 10.1016/j.snb.2018.04.157
Gao, Y., Cheng, Y., Huang, F., Lin, H., Xu, J., and Wang, Y. (2018). Sn2+/Mn2+codoped strontium phosphate (Sr2P2O7) phosphor for high temperature optical thermometry. J. Alloys Compd. 735, 1546–1552. doi: 10.1016/j.jallcom.2017.11.243
Jaque, D., and Jacinto, C. (2016). Luminescent nanoprobes for thermal bio-sensing: Towards controlled photo-thermal therapies. J. Lumin. 169, 394–399. doi: 10.1016/j.jlumin.2015.03.037
Jaque, D., and Vetrone, F. (2012). Luminescence nanothermometry. Nanoscale. 4:4301. doi: 10.1039/c2nr30764b
Kniec, K., and Marciniak, L. (2018). The influence of grain size and vanadium concentration on the spectroscopic properties of YAG:V3+,V5+and YAG: V, Ln3+(Ln3+ = Eu3+, Dy3+, Nd3+) nanocrystalline luminescent thermometers. Sens. Actuat. B Chem. 264, 382–390. doi: 10.1016/j.snb.2018.02.189
Liao, J., Wang, Q., Kong, L., Ming, Z., Wang, Y., Li, Y., et al. (2018). Effect of Yb3+concentration on tunable upconversion luminescence and optically temperature sensing behavior in Gd2TiO5:Yb3+/Er3+phosphors. Opt. Mater. (Amst). 75, 841–849. doi: 10.1016/j.optmat.2017.12.009
Liu, L., Sun, Z., Ma, C., Tao, R., Zhang, J., Li, H., et al. (2018). Highly sensitive and accurate optical thermometer through Er doped tellurite glasses. Mater. Res. Bull. 105, 306–311. doi: 10.1016/j.materresbull.2018.04.053
Malysa, B., Meijerink, A., and Jüstel, T. (2018). Temperature dependent Cr3+photoluminescence in garnets of the type X3Sc2Ga3O12(X = Lu, Y, Gd, La). J. Lumin. 202, 523–531. doi: 10.1016/j.jlumin.2018.05.076
Marciniak, L., and Bednarkiewicz, A. (2016). The influence of dopant concentration on temperature dependent emission spectra in LiLa1–x–yEuxTbyP4O12 nanocrystals: toward rational design of highly-sensitive luminescent nanothermometers. Phys. Chem. Chem. Phys. 18, 15584–15592. doi: 10.1039/C6CP00898D
Marciniak, L., Bednarkiewicz, A., Drabik, J., Trejgis, K., and Strek, W. (2017a). Optimization of highly sensitive YAG:Cr3+, Nd3+ nanocrystal-based luminescent thermometer operating in an optical window of biological tissues. Phys. Chem. Chem. Phys. 19, 7343–7351. doi: 10.1039/C6CP07213E
Marciniak, Ł., Bednarkiewicz, A., Hreniak, D., and Strek, W. (2016a). The influence of Nd3+ concentration and alkali ions on the sensitivity of non-contact temperature measurements in ALaP4O12 :Nd3+ (A = Li, K, Na, Rb) nanocrystalline luminescent thermometers. J. Mater. Chem. C. 4, 11284–11290. doi: 10.1039/C6TC03396B
Marciniak, L., Bednarkiewicz, A., Kowalska, D., and Strek, W. (2016b). A new generation of highly sensitive luminescent thermometers operating in the optical window of biological tissues. J. Mater. Chem. C. 4, 5559–5563. doi: 10.1039/C6TC01484D
Marciniak, L., Pilch, A., Arabasz, S., Jin, D., and Bednarkiewicz, A. (2017b). Heterogeneously Nd3+ doped single nanoparticles for NIR-induced heat conversion, luminescence, and thermometry. Nanoscale. 9, 8288–8297. doi: 10.1039/C7NR02630G
Marciniak, L., and Trejgis, K. (2018). Luminescence lifetime thermometry with Mn3+-Mn4+ co-doped nanocrystals. J. Mater. Chem. C 6, 7092–7100. doi: 10.1039/C8TC01981A
Runowski, M., Bartkowiak, A., Majewska, M., Martín, I. R., and Lis, S. (2018). Upconverting lanthanide doped fluoride NaLuF4:Yb3+-Er3+-Ho3+- optical sensor for multi-range fluorescence intensity ratio (FIR) thermometry in visible and NIR regions. J. Lumin. 201, 104–109. doi: 10.1016/j.jlumin.2018.04.040
Struve, B., and Huber, G. (2000). The effect of the crystal field strength on the optical spectra of Cr3+ in gallium garnet laser crystals. Appl. Phys. B. 36, 195–201. doi: 10.1007/BF00704574
Suo, H., Hu, F., Zhao, X., Zhang, Z., Li, T., Duan, C., et al. (2017). All-in-one thermometer-heater up-converting platform YF3 :Yb3+, Tm3+ operating in the first biological window. J. Mater. Chem. C. 5, 1501–1507. doi: 10.1039/C6TC05449H
Trejgis, K., and Marciniak, L. (2018). The influence of manganese concentration on the sensitivity of bandshape and lifetime luminescent thermometers in Y3Al5O12:Mn3+, Mn4+, Nd3+ nanocrystals. Phys. Chem. Chem. Phys. 20, 9574–9581. doi: 10.1039/C8CP00558C
Wang, C., Wadhwa, A., Cui, S., Ma, R., Qiao, X., Fan, X., et al. (2017). Dual mode temperature sensing through luminescence lifetimes of F- and O-coordinated Cr3+sites in fluorosilicate glass-ceramics. RSC Adv. 7, 52435–52441. doi: 10.1039/C7RA10864H
Xu, J., Ueda, J., and Tanabe, S. (2017). Toward tunable and bright deep-red persistent luminescence of Cr3+ in garnets. J. Am. Ceram. Soc. 100, 4033–4044. doi: 10.1111/jace.14942
Keywords: chromium, luminescence, luminescent thermometers, Nanocrystals (NCs), garnets
Citation: Elzbieciak K and Marciniak L (2018) The Impact of Cr3+ Doping on Temperature Sensitivity Modulation in Cr3+ Doped and Cr3+, Nd3+ Co-doped Y3Al5O12, Y3Al2Ga3O12, and Y3Ga5O12 Nanothermometers. Front. Chem. 6:424. doi: 10.3389/fchem.2018.00424
Received: 21 June 2018; Accepted: 28 August 2018;
Published: 19 September 2018.
Edited by:
Luís António Dias Carlos, University of Aveiro, PortugalReviewed by:
Xiaoji Xie, Nanjing Tech University, ChinaChun Xu, The University of Queensland, Australia
Copyright © 2018 Elzbieciak and Marciniak. This is an open-access article distributed under the terms of the Creative Commons Attribution License (CC BY). The use, distribution or reproduction in other forums is permitted, provided the original author(s) and the copyright owner(s) are credited and that the original publication in this journal is cited, in accordance with accepted academic practice. No use, distribution or reproduction is permitted which does not comply with these terms.
*Correspondence: Lukasz Marciniak, bC5tYXJjaW5pYWtAaW50aWJzLnBs