- 1CAS Key Laboratory of Tropical Marine Bio-Resources and Ecology, Guangdong Key Laboratory of Marine Materia Medica, South China Sea Institute of Oceanology, Chinese Academy of Sciences, Guangzhou, China
- 2University of Chinese Academy of Sciences, Beijing, China
- 3Guangdong Provincial Key Laboratory of New Drug Screening, Guangzhou Key Laboratory of Drug Research for Emerging Virus Prevention and Treatment, School of Pharmaceutical Sciences, Southern Medical University, Guangzhou, China
- 4State Key Laboratory of Organ Failure Research, Southern Medical University, Guangzhou, China
Influenza A virus (IAV) is a severe worldwide threat to public health and economic development due to its high morbidity and mortality. Marine-derived fungi have been evidenced as a prolific source for the discovery of pharmacologically-active lead compounds. During the course of our search for novel bioactive substances from marine microorganisms, six new polyketides, including two octaketides (1–2), one chromone derivative (13), two highly substituted phthalides (17–18), and one α-pyrone derivative (21) along with 22 known congeners were isolated from a mangrove-associated fungus Diaporthe sp. SCSIO 41011. Their structures were determined by spectroscopic analysis and by comparison with literature data. And the absolute configurations were established according to the specific rotation or electron circular dichroism method. Antiviral evaluation results revealed that compounds 14, 15, 26, and 5-chloroisorotiorin displayed significant anti-IAV activities against three influenza A virus subtypes, including A/Puerto Rico/8/34 H274Y (H1N1), A/FM-1/1/47 (H1N1), and A/Aichi/2/68 (H3N2), with IC50 values in the range of 2.52–39.97 μM. The preliminary structure-activity relationships (SARs) are also discussed. These findings expand the chemical and bioactive diversity of polyketides derived from the genus Diaporthe, and also provide a basis for further development and utilization of chromone, xanthone, and chloroazaphilone derivatives as source of potential anti-viral chemotherapy agents.
Introduction
Polyketides represent an important category of secondary metabolites with great structural diversity from simple aromatics to highly modified complex architectures, such as macrolides, polyphenols, polyethers, polyenes, and enediynes (Fujii, 2010; Zheng et al., 2015). Distributing broadly in microbial origins, they are constructed by combination of iterative polyketide synthases (PKSs) and multifunctional and iterative oxygenases (Hang et al., 2016). In addition, polyketides play a vital role in modern medicine due to their diverse pharmacological features, such as lovastatin, a well-known fungal polyketide statins functioned as a cholesterol-lowering agent (Crawford and Townsend, 2010). Belonging to the family of octaketides, biosynthetically related cytosporones, dothiorelones and phomopsins are characterized with a di-/tri-hydroxybenzene lactone or a resorcinol scaffold harboring an n-heptane substituent, which were mainly encountered in endophytic fungi of several genera, such as Phomopsis (Kornsakulkarn et al., 2015; Kongprapan et al., 2017; Tan et al., 2017), Diaporthe (Brady et al., 2000; Liu et al., 2018), Cytospora (Brady et al., 2000; Abreu et al., 2010), Pestalotiopsis (Xu et al., 2009b). Of special note, cytosporone B (7) was reported as a nuclear orphan receptor Nur77 agonist as a promising therapeutic drug for cancers and hypoglycemia (Zhan et al., 2008), as well as the transcription factor NR4A1 agonist to control IAV infection and improve pulmonary function in infected mice (Xia et al., 2013; Egarnes et al., 2017), which had aroused a great interest for chemical synthesis study (Von Delius et al., 2017).
Influenza A virus (IAV), a negative sense RNA virus, is one of the main causes of human acute respiratory diseases characterized with high morbidity and mortality, posing a serious threat to public health and economic development (Liu et al., 2017). IAVs repeatedly circulate in many animal hosts, such as humans, birds, horses, dogs, and pigs, which can be subtyped to two envelope proteins: haemagglutinin (HA) and neuraminidase (NA) glycoproteins according to the antigenic properties (Medina and Garcia-Sastre, 2011). In 2009, the pandemic influenza H1N1 virus rapidly spread to 214 countries around the world, causing human infection and acute respiratory illness of more than 17,700 deaths (Bautista et al., 2010). As of 25th April 2018, there have been reported 1625 confirmed cases of human H7N9 infection and 623 deaths since 2013 according to the World Health Organization (http://www.fao.org/ag/againfo/programmes/en/empres/H7N9/situation_up-date.html). However, two families of antiviral drugs are hitherto currently used to treat human IAV infections, which are NA inhibitors, like zanamivir and oseltamivir, and inhibitors of the viral M2 protein exemplified by amantadine and rimantadine (Medina and Garcia-Sastre, 2011; Song et al., 2015). Due to the emergence of drug-resistant viral strains, there is an urgent development for novel classes of anti-IAV agents with new mode of action.
Marine-derived fungi are reported as a prodigious source of development for new antivirals against different important viruses (Moghadamtousi et al., 2015). In our continuing endeavor to discover biologically active compounds from marine microbes, a series of structurally interesting and biologically active natural products have been described (Luo et al., 2017; Tan et al., 2018). Recently, six new cytotoxic chloroazaphilone derivatives, isochromophilones A–F, have been isolated from the fungus Diaporthe sp. SCSIO 41011, an endophytic fungus obtained from the fresh tissue of the marine mangrove plant Rhizophora stylosa (Luo et al., 2018). Subsequent chemical investigations on the remaining fractions of the fungus led to the isolation of structurally diverse aromatic polyketides, including octaketides (dothiorelones or cytosporones), phthalides, chromones, xanthones, etc. (Figure 1). The structures of these compounds were determined by physicochemical properties and spectral data analysis as well as comparison with those reported in the literature. All the compounds were examined for anti-IAV activities against three influenza A virus subtypes, including A/Puerto Rico/8/34 H274Y (H1N1), A/FM-1/1/47 (H1N1), and A/Aichi/2/68 (H3N2). Details of the isolation, structure elucidation, and biological activity of these compounds, as well as preliminary SARs, are reported herein.
Materials and Methods
General Experimental Procedures
Semi-preparative HPLC was performed on a Hitachi Primaide apparatus using an ODS (octadecylsilanized silica) column (YMC-pack ODS-A, YMC Co. Ltd., 10 × 250 mm, 5 μm, 2.5 mL/min). Chiral HPLC separation was performed using CHIRALPAK IC column (250 × 4.6 mm, 5 μm). TLC and column chromatography (CC) were performed on plates precoated with silica gel GF254 (10–40 μm) and over silica gel (200–300 mesh) (Qingdao Marine Chemical Factory), and Sephadex LH-20 (Amersham Biosciences), respectively. Spots were detected on TLC (Qingdao Marine Chemical Factory) under 254 nm UV light. All solvents employed were of analytical grade (Tianjin Fuyu Chemical and Industry Factory). The NMR spectra were obtained on a Bruker Avance spectrometer (Bruker) operating at 500 and 700 MHz for 1HNMR, 125 MHz, and 175 MHz for 13CNMR, using TMS as an internal standard. Optical rotations were acquired using a Perkin Elmer MPC 500 (Waltham) polarimeter. HRESIMS and ESIMS spectra data were recorded on a MaXis quadrupole-time-of-flight mass spectrometer and an amaZon SL ion trap mass spectrometer (Bruker), respectively. UV spectra were recorded on a Shimadzu UV-2600 PC spectrometer (Shimadzu). ECD spectra were performed on a Chirascan circular dichroism spectrometer (Applied Photophysics). IR spectra were measured on an IR Affinity-1 spectrometer (Shimadzu). The artificial sea salt was a commercial product (Guangzhou Haili Aquarium Technology Company).
Fungal Material
The fungus Diaporthe sp. SCSIO 41011 had the same origination as that in our recent published paper (Luo et al., 2018). A voucher specimen was deposited in the CAS Key Laboratory of Tropical Marine Bio-resources and Ecology, South China Sea Institute of Oceanology, Chinese Academy of Sciences, Guangzhou, China.
Extraction and Isolation
The fermentation of the strain and the extraction and isolation of its extract was identical to that of our recent published paper (Luo et al., 2018). Briefly, nine fractions was isolated from the EtOAc extract (150 g) by silica gel vacuum liquid chromatography (VLC) using step gradient elution with petroleum ether/CH2Cl2 (0–100%). Compounds 23 (40 mg, tR 30 min) and 25 (4.5 mg, tR 18 min) were purified from Fr.2 (13 g) by an ODS column (MeOH/H2O: 10–100%) and followed by semiprep-HPLC with 72% (2.5 mL/min) and 50% MeCN (2.3 mL/min) elutions, respectively. Fr.3 (10 g) was purified on Sephadex LH-20 (MeOH), an ODS column (MeOH/H2O: 10–100%) and finally semiprep-HPLC (70% MeCN, 2.3 mL/min) to afford compounds 17 (4.2 mg, tR 7 min) and 24 (3.5 mg, tR 9 min). Fraction 4 (12 g) was subjected to Sephadex LH-20 (MeOH) to provide eight subfractions (Frs.4-1~4-8). Then ten subfractions (Frs.4-3-1~4-3-10) were obtained from Fr. 4-3 by an ODS column (MeOH/H2O: 10–100%). Compound 18 (150 mg) was isolated from Fr. 4-3-4 by recrystallization. Meanwhile, compounds 20 (7.1 mg, tR 24 min), 14 (4.3 mg, tR 15 min) and 6 (141 mg, tR 25 min) were isolated from Frs. 4-3-6, 4-3-7, 4-3-8 by semiprep-HPLC with 55% MeOH (2.5 mL/min), 50% MeCN (2.3 mL/min), and 68% MeCN (1.4 mL/min) elutions, respectively. Besides, compounds 27 (2 mg, tR 10 min) and 26 (2.2 mg, tR 12 min) was purified from Fr.4-4 and Fr. 4-6 by semiprep-HPLC with 80% MeOH (2.5 mL/min) and 60% MeCN (2.3 mL/min) elutions, respectively. Fraction 5 (4 g) was subjected to Sephadex LH-20 (MeOH) to provide five subfractions (Frs.5-1~5-5). Fr. 5-4 was further purified on semiprep-HPLC by 66% MeCN (2.5 mL/min) to give compounds 8 (77 mg, tR 9 min), 12 (4 mg, tR 11.5 min), and 7 (142 mg, tR 20.6 min). Seven subfractions (Frs.7-1~7-7) were obtained from Fr. 7 (4 g) by Sephadex LH-20 (MeOH). Then Fr. 7-4 was subjected to an ODS column (MeOH/H2O: 10–100%) to provide thirteen subfractions (Frs.7-4-1~7-4-13). Fr. 7-4-8 was further purified on semiprep-HPLC by 47% MeCN (2.5 mL/min) to give compounds 4 (2.3 mg, tR 12 min), 10 (2 mg, tR 16 min), and 2 (3.8 mg, tR 39 min), along with one subfraction (Fr. 7-4-8-6). Compounds 11 (4.7 mg, tR 42 min) and 1 (24 mg, tR 45 min) were purified from Fr. 7-4-8-6 by semiprep-HPLC by 56% MeOH (2.3 mL/min). Fr. 7-4-12 was subjected to semiprep-HPLC (74%MeOH, 1.8 mL/min) to give compounds 16 (2.6 mg, tR 14 min) and 15 (3.8 mg, tR 45 min). Similarly, Fr. 7-5 was subjected to an ODS column (MeOH/H2O: 10–100%) to provide eight subfractions (Frs.7-5-1~7-5-8). Compounds 19 (5.6 mg, tR 10.4 min) and 28 (5 mg, tR 8 min) were purified from Fr.7-5-5 and Fr.7-5-6 by semiprep-HPLC with 50% MeCN (2.5 mL/min) and 47% MeCN (2.5 mL/min) elutions, respectively. Fr. 7-5-7 was subjected to semiprep-HPLC (50%MeCN, 2.5 mL/min) to afford compounds 22 (5 mg, tR 11.5 min) and 21 (1.5 mg, tR 15 min). Fr.9 (7 g) was subjected to Sephadex LH-20 (MeOH) and followed by an ODS column (MeOH/H2O: 10–100%) to provide six subfractions (Frs.9-1-1~9-1-6). Fr. 9-1-4 was purified by semiprep-HPLC (55%MeCN, 2.0 mL/min) to provide compounds 9 (3 mg, tR 12 min), 5 (3 mg, tR 25 min), 3 (2 mg, tR 28 min). Compund 13 (0.8 mg, tR 10 min) was isolated from Fr.9-1-5 by semiprep-HPLC (78% MeOH, 2.0 mL/min).
Antiviral Activity
All the isolated compounds (1–28), along with recently reported co-isolated 5-chloroisorotiorin (Luo et al., 2018), were screened for their anti-IAV activities according to the previously reported 3-(4,5-dimethylthiazol-2yl)-2,5-diphenyltetrazolium bromide (MTT) colorimetric assay, using ribavirin as a positive control (Li et al., 2017; Yang et al., 2018). In brief, Madin Derby canine kidney (MDCK) cells were cultured in Dulbecco's modified Eagle's medium (DMEM) supplemented with 10% fetal bovine serum and 1% penicillin/streptomycin. Meanwhile, different influenza A virus subtypes, including A/Puerto Rico/8/34 H274Y (H1N1), A/FM-1/1/47 (H1N1), and A/Aichi/2/68 (H3N2), were multiplied in 10-day-old chick embryo at 37°C. The cytotoxicity of the compounds was also evaluated by the MTT assay. Briefly, approximately 90% confluent cells in 96-well plates were exposed to the compounds at 2-fold serial dilutions. After 48 h of incubation, 100 μL of MTT solution, which was diluted by the medium to 0.5 mg/mL, was added and retained at 37°C for 4 h. Then the supernatant was removed, followed by the addition of 150 μL of dimethyl sulfoxide (DMSO) to dissolve the formazan product. The optical density for each well was measured on the Tecan Genios Pro microplate reader (Bedford, MA, USA) at 570 nm. To determine the antiviral activities of the compounds, confluent MDCK cells were infected with the virus at multiplicity of infection (MOI) of 0.01 at 37°C for 1 h. The compounds of non-cytotoxic concentrations were then added to the cells after washing away the unabsorbed virus with phosphate-buffered saline (PBS), and the cells were cultured for another 48 h. At the end of the culture, the MTT-based assay as previously described was assessed for the antiviral activity of the isolated compounds.
Statistical Analysis
All statistical analysis of the data were processed by GraphPad Prism. The results are presented as the mean ± standard deviation (SD) from experiments in triplicate. Student's t-test was used to analyze the statistical significance between two groups, more groups by one-way ANOVA with or without Tukey–Kramer multiple comparison. A p < 0.05 was regarded as statistically significant.
Results and Discussion
Identification of Compounds
The endophytic fungus Diaporthe sp. SCSIO 41011 was cultured on solid rice medium for 60 days. The EtOAc extract (150 g) of the fermentation was separated by continuously silica gel chromatography and semi-preparative HPLC chromatography to yield 28 aromatic polyketides (1–28). Their chemical structures were determined by comprehensive spectroscopic analyses or comparison with those reported data.
Compound 1 was obtained as colorless oil and had the molecular formula C19H26O7 as determined by a deprotonated ion peak at m/z 365.1607 (calcd for C19H25O7, 365.1600) in HRESIMS data. The 1H NMR data (Table 1) along with HSQC experiment of 1 displayed two singlet methyls at δH 2.01 (3H, s) and 3.65 (3H, s), two aromatic protons at δH 6.26 (1H, d, J = 2.2 Hz) and 6.19 (1H, d, J = 2.2 Hz), along with an array of methylene signals. The 13C NMR spectrum (Table 1) showed 19 resonances that were sorted by a distortionless enhancement by polarization transfer (DEPT) experiment, assigned to three carbonyls (δC 209.0, 174.0, and 173.1), six aromatic carbons (δC 161.8, 160.1, 137.0, 120.9, 112.0, and 102.9), eight methylenes (δC 65.7, 45.0, 40.4, 30.3, 30.1, 29.6, 26.8, and 25.4) and two methyls (δC 52.3 and 20.8). The aforementioned NMR data showed 1 was closely related structurally to the co-isolated 16-acetoxydothiorelone C (3) (Liu et al., 2018). The only difference was the appearance of a methyl group (δH/C 3.65/52.3) at C-1′ in 1 instead of an ethyl group in 3, which was also verified by the HMBC correlation from H3-1′ to C-1 (Figure 2). Thus, the structure of 1 was determined as shown in Figure 1 and assigned the trivial name dothiorelone O.
Compound 2 was also acquired as colorless oil and was determined to have the molecular formula C20H28O7 from the HRESIMS data. Inspection of the comprehensive spectral data of 2, including MS, and 1D, 2D-NMR data, indicated that 2 shared the same planer structure with (15S)-acetoxydothiorelone A (Liu et al., 2018). Comparison of the specific rotations between 2 ([α] = −5.8 (c 0.10, MeOH)) and (15S)-acetoxydothiorelone A ([α] = +4.9 (c 0.25, MeOH)) suggested 2 had the 15R configuration (Izuchi et al., 2011; Beekman and Barrow, 2015; Liu et al., 2018), given that they possessed only one chiral carbon at C-15. Therefore, the structure of 2 was resolved and accordingly named (15R)-acetoxydothiorelone A.
Compound 13 was also isolated as colorless oil and gave a molecular formula of C18H22O5 relied on a deprotonated ion peak at m/z 317.1403 (calcd for C18H21O5, 317.1389) in the HRESIMS spectrum. The 1H NMR data (Table 1) together with HSQC and HMBC spectra of 13 displayed signals indicative of two doublet methyls at δH 0.93 (3H, d, J = 7.1 Hz) and 1.24 (3H, d, J = 7.1 Hz), three aromatic protons at δH 6.65 (1H, d, J = 2.2 Hz), 6.73 (1H, d, J = 2.2 Hz) and 5.98 (1H, s), along with several methylenes. The 13C NMR spectrum (Table 1) showed 18 resonances attributable to two carbonyls (δC 173.6 and 181.4), six aromatic carbons (three oxygenated ones), six methylenes (δC 61.7, 42.1, 34.5, 32.3, 27.6, and 23.4) and two methyls (δC 14.2 and 14.5). The above mentioned spectral characteristics were closely consistent to those of the co-isolated pestalotiopsone F (14) (Xu et al., 2009a), but suggested the appearance of an ethyl group (δH/C 4.13/61.7 at C-1′ and 1.24/14.5 at C-2′) in 13 rather than a methyl group (δH/C 3.66/52.2) at C-1′ in 14. These changes were also ascertained by the HMBC correlations from H3-1′ to C-1 and from H3-2′ to C-1′, as well as the 1H-1H COSY correlation of H3-2′ and H2-1′ (Figure 2). Based on the above discussion, the structure of 13 was elucidated and the trivial name pestalotiopsone H was assigned.
Compound 17 was obtained as a white solid and its molecular formula was found to be C14H18O7 on the basis of HRESIMS and NMR data. The 1H NMR data (Table 2) of 17 revealed four O-methyls at δH 3.38, 3.38, 3.54, and 3.99, two singlet methylenes at δH 4.60 and 4.65, and a hemiketal methine at δH 6.29. In addition to the above seven corresponding hydrogen-bearing carbons, seven carbons remained in the 13C NMR spectrum, including one carbonyl (δC 169.6), six aromatic carbons [(δC 111.4, 119.0, 121.4, 148.9), and two oxygenated ones at δC 160.7 and 174.3]. The foregoing spectroscopic data showed great similarity to that of microsphaerophthalide F (Sommart et al., 2012) except that a methyl group (δH/C 2.16/8.5) located at C-6 in microsphaerophthalide F was replaced by an ethoxyl group (δH/C 4.60/65.3 at C-9 and 3.38/58.1 at C-10) anchored at C-6 in 17. This deduction was also supported by the HMBC correlations from H3-10 to C-9 and from H2-9 to C-5 and C-7. The barely measurable optical rotation value and quite weak Cotton effects in the ECD spectrum suggested 17 was racemic, which was also confirmed by the chiral HPLC analysis with two peaks (peak area ratio: 1:1) in the HPLC profile (Supplementary Materials). However, the quantity of 17 was too little to perform further resolution. Hence, the structure of compound 17 was elucidated and the trivial name (±)-microsphaerophthalide H was assigned.
Compound 18 was obtained as colorless needle crystals and had the molecular formula C13H14O7, as evidenced by HRESIMS ([M+H]+, 283.0809; calcd for C13H15O7, 283.0818) and the NMR data. The highly similar NMR spectroscopic data of 18 to those of 17 indicated that their structures were closely related, except for the presence of an aldehyde group (δH/C 10.14/192.5) joined at C-4 in 18, rather than an ethoxyl group (δH/C 4.65/67.2 and 3.38/58.4) anchored at C-4 in 17. The absolute configuration of C-3 of 18 was mainly determined by comparison of the specific rotations with those reported data, as well as comparison between the computed and experimental ECD spectra. Among these reported 3-oxygenated phthalides, the 3S- and 3R- ones generally showed negative and positive specific rotations, respectively (Sommart et al., 2012). Thus, the positive sign of specific rotation [[α] = +25 (c 0.10, MeOH)] of 18 led to the deduction of 3R configuration in 18, which was also confirmed from experimental and calculated ECD spectra of 18 as shown in Figure 3. Further chiral HPLC analysis confirmed that 18 was single enantiomer. Consequently, the structure of 18 was determined as shown in Figure 1 and termed microsphaerophthalide I. Notably, the 3-oxygenated phthalides are uncommon in natural sources.
Compound 21 was obtained as a white solid and had the molecular formula C12H14O5, as determined from HRESIMS ([M+H]+, 239.0917; calcd for C12H15O5, 239.0919) and the NMR data. Detailed analyses of its NMR spectroscopic features implied that it was closely related structurally to convolvulopyrone (Tsantrizos et al., 1992), but for the presence of an additional O-methyl group (δH/C 3.76/52.0) at C-10 in 21, indicating that 21 was a methyl derivative of convolvulopyrone. Besides, compound 21 and convolvulopyrone shared the same relative configuration of 7E due to the absence of a NOESY correlation between H-7 and H3-11. As a result, the structure of 21 was determined and given the trivial name methyl convolvulopyrone. However, compound 21 was likely obtained as an artifact formed in the process of extraction and purification using MeOH as a solvent.
Besides, these co-isolated known congeners were elucidated by comparing their physicochemical properties and spectroscopic data with those reported literature values (Supplementary Materials). They were determined as 16-acetoxydothiorelone C (3) (Liu et al., 2018), dothiorelone C (4) (Liu et al., 2018), (14R)-acetoxydothiorelone B (5) (Liu et al., 2018), cytosporone N (6) (Beekman and Barrow, 2015), cytosporone B (7) (Brady et al., 2000), cytosporone A (8) (Brady et al., 2000), dothiorelone I (9) (Liu et al., 2018), (15R)-dothiorelone A (10) (Liu et al., 2018), methyl (R)-2-(2-(7-acetoxyoctanoyl)-3,5-dihydroxyphenyl)acetate (11) (Beekman and Barrow, 2015), secocurvularin (12) (Bracher and Krauss, 1998), pestalotiopsone F (14) (Xu et al., 2009a), pestalotiopsone B (15) (Xu et al., 2009a), pestalotiopsone A (16) (Xu et al., 2009a), 5-hydroxy-7-methoxy-4,6-dimethylphthalide (19) (Sommart et al., 2012), dihydrovermistatin (20) (Komai et al., 2005), sclerotinin A (22) (Lai et al., 2013), 3,5-dimethyl-8-hydroxy-3,4-dihydroisocoumarin (23) (Kokubun et al., 2003), 3,5-dimethyl-8-methoxy-3,4-dihydroisocoumarin (24) (Kokubun et al., 2003), methyl 8-hydroxy-6-methyl-9-oxo-9H-xanthene-1-carboxylate (25) (Lai et al., 2013), 3,8-dihydroxy-6-methyl-9-oxo-9H-xanthene-1-carboxylate (26) (Nguyen et al., 2017), pinselin (27) (Cui et al., 2016), 7-hydroxy-2,5-dimethylchromone (28) (Koenigs et al., 2010). Amongst, methyl (R)-2-[2-(7-acetoxyoctanoyl)-3,5-dihydroxyphenyl]acetate (11) was isolated as a naturally occurring compound for the first time. While sclerotinin A (22) was isolated as a diastereoisomeric mixture which could not be separated by RP-HPLC method (Lai et al., 2013). This research further enriched secondary metabolites in the genus Diaporthe and also expanded the chemical diversity of polyketides, such as dothiorelones, cytosporones, phthalides, chromones, etc.
Antiviral Activity
Antiviral effect of the isolated compounds 1–28 and 5-chloroisorotiorin against different IAV subtypes, including A/Puerto Rico/8/34 H274Y (H1N1), A/FM-1/1/47 (H1N1), and A/Aichi/2/68 (H3N2), were then evaluated. Those compounds showed nearly no cytotoxicity against MDCK cells (IC50 > 200 μM). Compounds 14, 15, 26, and 5-chloroisorotiorin displayed significant anti-IAV activities against the above mentioned subtypes with IC50 values in the range of 2.52–39.97 μM (Table 3). However, the remaining compounds (1–13, 16–25, 27–28) were inactive toward the three aforementioned IAV subtypes. Furthermore, pestalotiopsone F (14) and 3,8-dihydroxy-6-methyl-9-oxo-9H-xanthene-1-carboxylate (26) exhibited obvious inhibition effect on A/FM-1/1/47 (H1N1), as well as A/Aichi/2/68 (H3N2), in a dose-dependent manner (Figure 4).
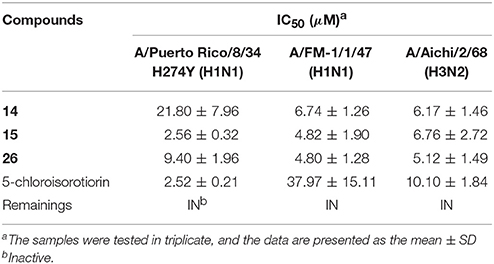
Table 3. Inhibition activity of compounds 1–28 and 5-chloroisorotiorin against Influenza A Virus strains.
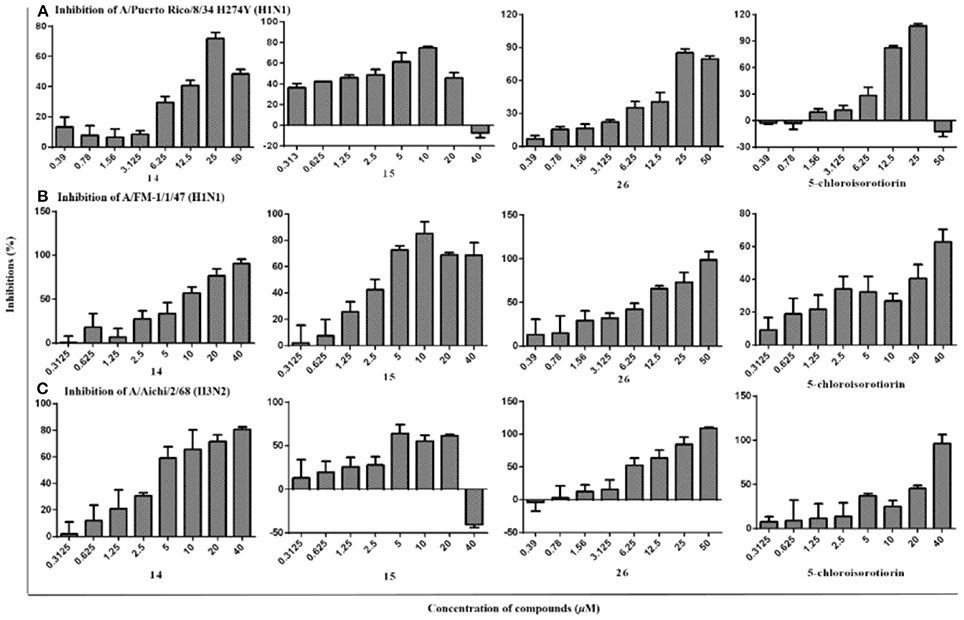
Figure 4. The inhibitory activities of compounds 14, 15, 26, and 5-chloroisorotiorin against influenza A viruses, including A/Puerto Rico/8/34 H274Y (H1N1) (A), A/FM-1/1/47 (H1N1) (B), and A/Aichi/2/68 (H3N2) (C).
Additionally, based on comparison of the structural characteristics among these analogs, a preliminary structure-activity relationship is discussed. Pestalotiopsone F (14) exhibited selective inhibitions against the two IAV subtypes of A/FM-1/1/47 (H1N1) and A/Aichi/2/68 (H3N2) with the IC50 values of 6.74 ± 1.26 and 6.17 ± 1.46 μM, respectively. Comparing with the antiviral pestalotiopsones F (14) and B (15), the co-isolated siblings pestalotiopsones H (13) and A (16) were inactive toward the three IAV subtypes, which revealed methylation of the carboxyl group at C-1 for the pestalotiopsones with a C7 aliphatic branch located at C-11, and while ethylation of the carboxyl group at C-1 for the pestalotiopsones with a C5 aliphatic branch located at C-11, were essential for anti-IAV activities. Notably, among the three xanthones (25–27), only 3,8-dihydroxy-6-methyl-9-oxo-9H-xanthene-1-carboxylate (26) demonstrated remarkable inhibitory effects against A/FM-1/1/47 (H1N1), A/Puerto Rico/8/34 H274Y (H1N1), and A/Aichi/2/68 (H3N2) with IC50 values of 4.80 ± 1.28, 9.40 ± 1.96, and 5.12 ± 1.49 μM, respectively, which indicated the hydroxyl group at C-3 probably promote the anti-IAV activities toward the three subtypes. By the way, 5-chloroisorotiorin (Luo et al., 2018), a recently reported co-isolated chloroazaphilone derivative obtained with major amount, was also screened for anti-IAV activity, which presented selective inhibition activities against the two IAV subtypes of A/Puerto Rico/8/34 H274Y (H1N1) and A/Aichi/2/68 (H3N2) with the IC50 values of 2.52 ± 0.21 and 10.10 ± 1.84 μM, respectively. The remaining ones (1–13, 16–26, 27–28) showed no obvious inhibition against A/FM-1/1/47 (H1N1), A/Puerto Rico/8/34 H274Y (H1N1), and A/Aichi/2/68 (H3N2).
Characterization of Compounds
Dothiorelone O (1): colorless oil; UV (MeOH) λmax (log ε) 302 (3.75), 269 (3.89), 220 (4.16), 204 (4.27) nm; IR (film) νmax 3,356, 2,933, 2,833, 1,732, 1,714, 1,609, 1,591, 1,462, 1,261, 1,163, and 1,028 cm−1; 1H and 13C NMR data, Table 1; HRESIMS m/z 365.1607 [M–H]− (calcd for C19H25O7, 365.1600), 401.1368 [M+Cl]− (calcd for C19H26ClO7, 401.1367).
(15R)-acetoxydothiorelone A (2): colorless oil; [α] – 5.8 (c 0.10, MeOH); UV (MeOH) λmax (log ε) 303 (3.69), 269 (3.78), 220 (4.14), 204 (4.17) nm; IR (film) νmax 3,358, 2,933, 2,858, 1,732, 1,714, 1,609, 1,558, 1,456, 1,265, 1,249, 1,159, and 1,024 cm−1; 1H and 13C NMR data, Table 1; HRESIMS m/z 403.1741 [M+Na]+ (calcd for C20H28NaO7, 403.1733), 419.1470 [M+K]+ (calcd for C20H28KO7, 419.1472), 783.3563 [2M+Na]+ (calcd for C40H56NaO14, 783.3568).
Pestalotiopsone H (13): colorless oil; UV (MeOH) λmax (log ε) 291 (4.14), 250 (4.31), 242 (4.28), 217 (4.38) nm; IR (film) νmax 3,419, 2,927, 2,856, 1,732, 1,716, 1,645, 1,558, 1,456, 1,375, 1,274, 1,180, 1,161, and 1,028 cm−1; 1H and 13C NMR data, Table 1; HRESIMS m/z 317.1403 [M–H]− (calcd for C18H21O5, 317.1389), 353.1167 [M+Cl]− (calcd for C18H22ClO5, 353.1156), 635.2860 [2M–H]− (calcd for C36H43O10, 635.2856), 671.2626 [2M+Cl]− (calcd for C36H44ClO10, 671.2623).
(±)-microsphaerophthalide H (17): a white solid; [α] – 5 (c 0.06, MeOH); UV (MeOH) λmax (log ε) 307 (4.06), 249 (4.05), 220 (4.48) nm; IR (film) νmax 3,419, 2,935, 2,846, 1,747, 1,734, 1,604, 1,558, 1,448, 1,429, 1,375, 1,276, 1,201, 1,159, 1,093, and 1,074 cm−1; 1H and 13C NMR data, Table 2; HRESIMS m/z 299.1123 [M+H]+ (calcd for C14H19O7, 299.1131), 321.0954 [M+Na]+ (calcd for C14H18NaO7, 321.0950), 619.2010 [2M+Na]+ (calcd for C28H36NaO14, 619.2003).
Microsphaerophthalide I (18): colorless needle crystals; [α] = +25 (c 0.10, MeOH); UV (MeOH) λmax (log ε) 367 (3.60), 295 (4.07), 245 (4.46), 201 (4.10) nm; ECD (0.15 mg/mL, MeOH) λmax (Δε) 203 (−2.07), 225 (+4.62), 251 (−6.00) nm; IR (film) νmax 3,419, 2,945, 2,885, 1,770, 1,749, 1,668, 1,653, 1,558, 1,489, 1,375, 1,338, 1,286, 1,205, 1,097, and 1,058 cm−1; 1H and 13C NMR data, Table 2; HRESIMS m/z 283.0809 [M+H]+ (calcd for C13H15O7, 283.0818), 305.0632 [M+Na]+ (calcd for C13H14NaO7, 305.0637).
Methyl convolvulopyrone (21): a white solid; UV (MeOH) λmax (log ε) 343 (4.05), 279 (3.73), 239 (4.51), 205 (4.12) nm; IR (film) νmax 3,446, 2,954, 2,927, 1,681, 1,635, 1,446, 1,355, 1,195, 1,182, 1,139, and 1,039 cm−1; 1H and 13C NMR data, Table 2; HRESIMS m/z 239.0917 [M+H]+ (calcd for C12H15O5, 239.0919), 261.0749 [M+Na]+ (calcd for C12H14NaO5, 261.0739), 499.1590 [2M+Na]+ (calcd for C24H28NaO10, 499.1580).
Conclusions
Twenty-eight aromatic polyketides, including two new octaketides (1–2), one new chromone derivative (13), two new highly substituted phthalides (17–18), and one new α-pyrone derivative (21) along with 22 known congeners were isolated from a mangrove-associated fungus Diaporthe sp. SCSIO 41011, while methyl (R)-2-[2-(7-acetoxyoctanoyl)-3,5-dihydroxyphenyl]acetate (11) was isolated as a new natural compound and (±)-microsphaerophthalide H (17) was obtained as a racemic mixture. Amongst, pestalotiopsone F (14), pestalotiopsone B (15), 3,8-dihydroxy-6-methyl-9-oxo-9H-xanthene-1-carboxylate (26), and 5-chloroisorotiorin displayed pronounced anti-IAV activities against three IAV virus subtypes, including A/Puerto Rico/8/34 H274Y (H1N1), A/FM-1/1/47 (H1N1), and A/Aichi/2/68 (H3N2) with IC50 values in the range of 2.52–39.97 μM. This work further enriched secondary metabolites in the genus Diaporthe and also expanded the chemical and bioactive diversity of polyketides, such as dothiorelones, cytosporones, phthalides, chromones, etc. Furthermore, our findings provide a basis for further development and utilization of pestalotiopsone, xanthone, and chloroazaphilone derivatives as source of potential anti-IAV chemotherapy agents.
Author Contributions
XiaL: designed the experiments and performed the isolation and characterization of all the compounds and wrote the manuscript; JY and FC: performed the antiviral experiment; XiuL: performed the isolation and purification of the fungal strain; CC: contributed to isolation of the compounds; XZ: designed the research work and wrote the manuscript; SL and YL: contributed in project design and manuscript preparation. All authors reviewed the manuscript and approved for submission.
Conflict of Interest Statement
The authors declare that the research was conducted in the absence of any commercial or financial relationships that could be construed as a potential conflict of interest.
Acknowledgments
This work was supported by grants from the National Natural Science Foundation of China (81741154, 41476135, 21772210), National Major Scientific and Technological Special Project for Significant New Drugs Development (2018ZX09735001-002-003), the Natural Science Foundation of Guangdong Province (2014A030313765, 2016A030313591), Science and Technology Project of Guangdong Province (2016A020222009), and the Open Project of State Key Laboratory of Organ Failure Research. We thank to Z. Xiao, C. Li and A. Sun, analytical facility center of South China Sea Institute of Oceanology, for recording NMR, and MS data.
Supplementary Material
The Supplementary Material for this article can be found online at: https://www.frontiersin.org/articles/10.3389/fchem.2018.00282/full#supplementary-material
References
Abreu, L. M., Phipps, R. K., Pfenning, L. H., Gotfredsen, C. H., Takahashi, J. A., and Larsen, T. O. (2010). Cytosporones O, P and Q from an endophytic Cytospora sp. Tetrahedron Lett. 51, 1803–1805. doi: 10.1016/j.tetlet.2010.01.110
Bautista, E., Chorpitayasunondh, T., Gao, Z., Harper, S. A., Shaw, M., Uyeki, T. M., et al. (2010). Medical progress: clinical aspects of pandemic 2009 influenza A (H1N1) virus infection. New Engl. J. Med. 362, 1708–1719. doi: 10.1056/NEJMra1000449
Beekman, A. M., and Barrow, R. A. (2015). Syntheses of cytosporones A, C, J, K, and N, metabolites from medicinal fungi. Aust. J. Chem. 68, 1583–1592. doi: 10.1071/CH15144
Bracher, F., and Krauss, J. (1998). Total synthesis of secocurvularin, curvulin, and the corresponding carboxylic acids. A convenient application of the enzymatic hydrolysis of acid and base sensitive esters. Nat. Prod. Lett. 12, 31–34. doi: 10.1080/10575639808048867
Brady, S. F., Wagenaar, M. M., Singh, M. P., Janso, J. E., and Clardy, J. (2000). The cytosporones, new octaketide antibiotics isolated from an endophytic fungus. Org. Lett. 2, 4043–4046. doi: 10.1021/ol006680s
Crawford, J. M., and Townsend, C. A. (2010). New insights into the formation of fungal aromatic polyketides. Nat. Rev. Microbiol. 8, 879–889. doi: 10.1038/nrmicro2465
Cui, X., Zhu, G., Liu, H., Jiang, G., Wang, Y., and Zhu, W. (2016). Diversity and function of the Antarctic krill microorganisms from Euphausia superba. Sci. Rep. 6:36496. doi: 10.1038/srep36496
Egarnes, B., Blanchet, M. R., and Gosselin, J. (2017). Treatment with the NR4A1 agonist cytosporone B controls influenza virus infection and improves pulmonary function in infected mice. PloS ONE 12:e0186639. doi: 10.1371/journal.pone.0186639
Fujii, I. (2010). Functional analysis of fungal polyketide biosynthesis genes. J. Antibiot. 63, 207–218. doi: 10.1038/ja.2010.17
Hang, L., Liu, N., and Tang, Y. (2016). Coordinated and iterative enzyme catalysis in fungal polyketide biosynthesis. ACS Catal. 6, 5935–5945. doi: 10.1021/acscatal.6b01559
Izuchi, Y., Koshino, H., Hongo, Y., Kanomata, N., and Takahashi, S. (2011). Synthesis and structural revision of phomopsin B, a novel polyketide carrying a 10-membered cyclic-ether ring. Org. Lett. 13, 3360–3363. doi: 10.1021/ol2011117
Koenigs, P., Rinker, B., Maus, L., Nieger, M., Rheinheimer, J., and Waldvogel, S. R. (2010). Structural revision and synthesis of altechromone A. J. Nat. Prod. 73, 2064–2066. doi: 10.1021/np1005604
Kokubun, T., Veitch, N. C., Bridge, P. D., and Simmonds, M. S. J. (2003). Dihydroisocoumarins and a tetralone from Cytospora eucalypticola. Phytochemistry 62, 779–782. doi: 10.1016/S0031-9422(02)00606-4
Komai, S., Hosoe, T., Itabashi, T., Nozawa, K., Yaguchi, T., Fukushima, K., et al. (2005). New vermistatin derivatives isolated from Penicillium simplicissimum. Heterocycles 65, 2771–2776. doi: 10.3987/COM-05-10523
Kongprapan, T., Xu, X., Rukachaisirikul, V., Phongpaichit, S., Sakayaroj, J., Chen, J., et al. (2017). Cytosporone derivatives from the endophytic fungus Phomopsis sp PSU-H188. Phytochem. Lett. 22, 219–223. doi: 10.1016/j.phytol.2017.10.002
Kornsakulkarn, J., Somyong, W., Supothina, S., Boonyuen, N., and Thongpanchang, C. (2015). Bioactive oxygen-bridged cyclooctadienes from endophytic fungus Phomopsis sp BCC 45011. Tetrahedron 71, 9112–9116. doi: 10.1016/j.tet.2015.10.015
Lai, D., Broetz-Oesterhelt, H., Mueller, W. E., Wray, V., and Proksch, P. (2013). Bioactive polyketides and alkaloids from Penicillium citrinum, a fungal endophyte isolated from Ocimum tenuiflorum. Fitoterapia 91, 100–106. doi: 10.1016/j.fitote.2013.08.017
Li, R., Liu, T., Liu, M., Chen, F., Liu, S., and Yang, J. (2017). Anti-influenza A virus activity of dendrobine and its mechanism of action. J. Agr Food Chem. 65, 3665–3674. doi: 10.1021/acs.jafc.7b00276
Liu, M., Chen, F., Liu, T., Chen, F., Liu, S., and Yang, J. (2017). The role of oxidative stress in influenza virus infection. Microbes Infect. 19, 580–586. doi: 10.1016/j.micinf.2017.08.008
Liu, Z., Zhao, J., Liang, X., Lv, X., Li, Y., Qu, J., et al. (2018). Dothiorelone derivatives from an endophyte Diaporthe pseudomangiferaea inhibit the activation of human lung fibroblasts MRC-5 cells. Fitoterapia 127, 7–14. doi: 10.1016/j.fitote.2018.04.009
Luo, X., Lin, X., Tao, H., Wang, J., Li, J., Yang, B., et al. (2018). Isochromophilones A–F, cytotoxic chloroazaphilones from the marine mangrove endophyticfungus Diaporthe sp. SCSIO 41011. J. Nat. Prod. 81, 934–941. doi: 10.1021/acs.jnatprod.7b01053
Luo, X., Zhou, X., Lin, X., Qin, X., Zhang, T., Wang, J., et al. (2017). Antituberculosis compounds from a deep-sea-derived fungus Aspergillus sp. SCSIO Ind09F01. Nat. Prod. Res. 31, 1958–1962. doi: 10.1080/14786419.2016.1266353
Medina, R. A., and Garcia-Sastre, A. (2011). Influenza A viruses: new research developments. Nat. Rev. Microbiol. 9, 590–603. doi: 10.1038/nrmicro2613
Moghadamtousi, S., Nikzad, S., Kadir, H., Abubakar, S., and Zandi, K. (2015). Potential antiviral agents from marine fungi: an overview. Mar. Drugs 13, 4520– 4538. doi: 10.3390/md13074520
Nguyen, T. T. N., Tran, H. Q., Kim, K. W., Kim, H. J., Sohn, J. H., Kang, D. G., et al. (2017). Anti-inflammatory effects of secondary metabolites isolated from the marine-derived fungal strain Penicillium sp SF-5629. Arch. Pharm. Res. 40, 328–337. doi: 10.1007/s12272-017-0890-5
Sommart, U., Rukachaisirikul, V., Tadpetch, K., Sukpondma, Y., Phongpaichit, S., Hutadilok-Towatana, N., et al. (2012). Modiolin and phthalide derivatives from the endophytic fungus Microsphaeropsis arundinis PSU-G18. Tetrahedron 68, 10005–10010. doi: 10.1016/j.tet.2012.09.043
Song, G., Shen, X., Li, S., Li, Y., Liu, Y., Zheng, Y., et al. (2015). Structure-activity relationships of 3-O-beta-chacotriosyl ursolic acid derivatives as novel H5N1 entry inhibitors. Eur. J. Med. Chem. 93, 431–442. doi: 10.1016/j.ejmech.2015.02.029
Tan, Q. W., Fang, P. H., Ni, J. C., Gao, F., and Chen, Q. J. (2017). Metabolites produced by an endophytic Phomopsis sp and their anti-TMV activity. Molecules 22:E2073. doi: 10.3390/molecules22122073
Tan, Y., Yang, B., Lin, X., Luo, X., Pang, X., Tang, L., et al. (2018). Nitrobenzoyl sesquiterpenoids with cytotoxic activities from a marine-derived Aspergillus ochraceus Fungus. J. Nat. Prod. 81, 92–97. doi: 10.1021/acs.jnatprod.7b00698
Tsantrizos, Y. S., Ogilvie, K. K., and Watson, A. K. (1992). Phytotoxic metabolites of Pomopsis onvolvulus, a host-specific pathogen of Feld bindweed. Can. J. Chem. 70, 2276–2284. doi: 10.1139/v92-286
Von Delius, M., Le, C. M., Ellinger, B., Kuzikov, M., Gul, S., and Dong, V. M. (2017). Synthesis and biological activity of octaketides from the cytosporone family. Isr. J. Chem. 57, 975–981. doi: 10.1002/ijch.201700023
Xia, Z., Cao, X., Rico-Bautista, E., Yu, J., Chen, L., Chen, J., et al. (2013). Relative impact of 3-and 5-hydroxyl groups of cytosporone B on cancer cell viability. Medchemcomm 4, 332–339. doi: 10.1039/C2MD20243C
Xu, J., Kjer, J., Sendker, J., Wray, V., Guan, H., Edrada, R., et al. (2009a). Chromones from the endophytic fungus Pestalotiopsis sp. isolated from the Chinese mangrove plant Rhizophora mucronata. J. Nat. Prod. 72, 662–665. doi: 10.1021/np800748u
Xu, J., Kjer, J., Sendker, J., Wray, V., Guan, H., Edrada, R., et al. (2009b). Cytosporones, coumarins, and an alkaloid from the endophytic fungus Pestalotiopsis sp. isolated from the Chinese mangrove plant Rhizophora mucronata. Bioorgan. Med. Chem. 17, 7362–7367. doi: 10.1016/j.bmc.2009.08.031
Yang, L. P., Gu, X. L., Chen, J. X., Yang, J., Tan, S. Y., and Duan, W. J. (2018). Chemical constituents from Canarium album Raeusch and their anti-influenza A virus activities. J. Nat. Med. 72, 808–815 doi: 10.1007/s11418-018-1208-8
Zhan, Y., Du, X., Chen, H., Liu, J., Zhao, B., Huang, D., et al. (2008). Cytosporone B is an agonist for nuclear orphan receptor Nur77. Nat. Chem. Biol. 4, 548–556. doi: 10.1038/nchembio.106
Keywords: Diaporthe sp., polyketides, cytosporones, phthalides, anti-influenza A virus
Citation: Luo X, Yang J, Chen F, Lin X, Chen C, Zhou X, Liu S and Liu Y (2018) Structurally Diverse Polyketides From the Mangrove-Derived Fungus Diaporthe sp. SCSIO 41011 With Their Anti-influenza A Virus Activities. Front. Chem. 6:282. doi: 10.3389/fchem.2018.00282
Received: 07 May 2018; Accepted: 22 June 2018;
Published: 12 July 2018.
Edited by:
Xian-Wen Yang, Third Institute of Oceanography, State Oceanic Administration, ChinaReviewed by:
Christophe Salome, SpiroChem AG, SwitzerlandRongbiao Pi, Sun Yat-sen University, China
Copyright © 2018 Luo, Yang, Chen, Lin, Chen, Zhou, Liu and Liu. This is an open-access article distributed under the terms of the Creative Commons Attribution License (CC BY). The use, distribution or reproduction in other forums is permitted, provided the original author(s) and the copyright owner(s) are credited and that the original publication in this journal is cited, in accordance with accepted academic practice. No use, distribution or reproduction is permitted which does not comply with these terms.
*Correspondence: Xuefeng Zhou, eGZ6aG91QHNjc2lvLmFjLmNu
Shuwen Liu, bGl1c3dAc211LmVkdS5jbg==
Yonghong Liu, eW9uZ2hvbmdsaXVAc2NzaW8uYWMuY24=
† These authors have contributed equally to this work.