- 1Department of Pathophysiology, School of Basic Medical Sciences, Zhengzhou University, Zhengzhou, China
- 2China-US (Henan) Hormel Cancer Institute, Zhengzhou, China
- 3Department of Neurobiology, University of Massachusetts Medical School, Worcester, MA, United States
- 4Department of Biochemistry and Molecular Pharmacology, University of Massachusetts Medical School, Worcester, MA, United States
- 5Therapeutics & Biotechnology Division, Korea Research Institute of Chemical Technology, Daejeon, South Korea
- 6The Affiliated Cancer Hospital, Zhengzhou University, Zhengzhou, China
- 7Department of Pharmacy, College of Pharmacy, Mokpo National University, Mokpo-si, South Korea
- 8College of Korean Medicine, Dongshin University, Naju, South Korea
Esophageal squamous cell carcinoma (ESCC) is a leading cause of cancer-related death worldwide. Xanthohumol is a prenylated flavonoid isolated from hops. Although xanthohumol has been reported to exert anti-obesity, hypoglycemic, anti-hyperlipidemia and anti-cancer activities, the mechanisms underlying its chemotherapeutic activity are yet to be elucidated. In the present study, we found that xanthohumol inhibited ESCC cell proliferation in vitro and in vivo by targeting keratin (KRT)-18. Xanthohumol suppressed the proliferation, foci formation, and anchorage-independent colony growth of KYSE30 cells. Using xanthohumol-sepharose conjugated bead pull-down and mass/mass analysis, we found that KRT18 is a novel target of xanthohumol in KYSE30 cells. KRT18 protein was highly expressed in patient ESCC tissues compared to adjunct tissues. Anti-proliferative activity of xanthohumol was abrogated or enhanced according to the knockdown or overexpression of KRT18 protein, respectively. Xanthohumol also induced apoptosis and cell cycle arrest at G1 phase which was associated with the modulation of expression of related makers including cyclin D1, cyclin D3, and cleaved-PARP, Bcl-2, cytochrome c and Bax. While xanthohumol attenuated KRT18 protein expression, it failed to cause any change in the KRT18 mRNA level. Furthermore, oral administration of xanthohumol decreased tumor volume and weight in patient-derived xenografts (PDXs) tumors having overexpressed KRT18. Overall these results suggest that xanthohumol acts as a KRT18 regulator to suppress the growth of ESCC.
Introduction
Esophageal cancer (EC) is the ninth leading cause of cancer-related death worldwide. EC patients always have poor prognosis with 5-year survival rate about 19% (Bray et al., 2018; Siegel et al., 2019). Off the two primary subtypes of EC, the esophageal squamous cell carcinoma (ESCC) has 70% prevalence globally with predominant distribution in the developing countries located in eastern Asia and parts of Africa (Smyth et al., 2017), and the esophageal adenocarcinoma (EAC) is mostly prevalent in Western countries (Testa et al., 2017). Cancer Statistics in China reports that EC ranks the third in men and fifth in women among all diagnosed cancer (Chen et al., 2016). Major risk factors of ESCC includes but are not limited to tobacco smoking, chronic alcohol consumption, and exposure to dietary carcinogens (Yang et al., 2016; Lagergren et al., 2017).
About 19% of patients with EC are diagnosed at early localized stage when endoscopic resection or endoscopic ablation can cure the disease (Ahmed et al., 2019). Combination of surgery with chemotherapy and/or radiotherapy is the common method for treating EC and improve the survival of patients (Mayanagi et al., 2019). The commonly used chemotherapy in clinical settings include cisplatin, 5-fluorouracil, docetaxel, carboplatin, paclitaxel, and bleomycin as well as their combinations (Nakamura et al., 2013; Ku, 2017). Targeted therapies and immunotherapies are also two emerging therapy used to treat ECs. Although Food and Drug Administration (FDA)-approved 13 drugs for EC treatment, many of these are targeted therapies such as cetuximab and lapatinib (targeting epidermal growth factor receptor, EGFR), irinotecan (inhibitor of type I topoisomerase), trastuzumab (monoclonal antibody against human epidermal growth factor receptor 2, HER2) and apitinib (inhibitor of vascular endothelial growth factor, VEGFR) (Teicher, 2008; Davidson and Starling, 2016; Huang et al., 2018; Barsouk et al., 2019). However, these drugs have failed to reach clinical success to curb EC prevalence largely due to non-responsiveness of many patients. There are also many investigational drugs targeting MET, mammalian target of rapamycin (mTOR), or poly ADP ribose polymerase (PARP), which have been failed in phase II or phase III clinical trial (Lyons and Ku, 2017; Liu X. et al., 2019). The immunotherapy agent pembrolizumab, has been approved by the FDA to treat PD-L1-positive metastatic EAC and ESCC in July 2019, but patients contained PD-L1 expression is not so high to response to this agent (Barsouk et al., 2019). Thus, there is emerging need for developing new therapies with better efficacy and tolerability by focusing on novel drug targets.
Keratin 18 (KRT18) is a member of the intermediate filament family of cytoskeletal protein, it plays an important role for tissue integrity (Lebherz-Eichinger et al., 2013). KRT18 was found to be over expressed in many different types of cancer including that of colorectal cancer and associated with tumor stage, cancer migration, and invasion (Kilic-Baygutalp et al., 2016). The relatively higher serum KRT18 levels in ESCC patients as compared to normal subjects were correlated with tumor progression (Kilic-Baygutalp et al., 2016).
Because of multiple biological activities and relatively low systemic toxicity, natural compounds gained much attention from researchers to explore its anticancer function (Song et al., 2018; Liu X. et al., 2019). Xanthohumol, a prenylated flavonoid isolated from hops (Humulus lupulus L.), has anti-obesity, hypoglycemic and anti-hyperlipidemia activities (Liu et al., 2015; Jiang et al., 2018). Numerous in vitro and in vivo studies have revealed the anticancer effect of xanthohumol (Sun et al., 2018; Wei et al., 2018; Liu W. et al., 2019; Slawinska-Brych et al., 2019). Xanthohumol exerts anti-cancer effects through inhibition of the activity of AKT, mTOR, NFκB/IKK, IL-1β and TNFα (Guo et al., 2018; Li et al., 2018; Saito et al., 2018; Lin et al., 2019; Liu X. et al., 2019). Previously we have reported that xanthohumol inhibited the growth of AKT kinase overexpressing ESCC cells (Liu X. et al., 2019). We also found that xanthohumol could inhibit the proliferation of cells with low level of AKT. This led us to continue to find additional molecular target of xamthohumol for its anti-cancer effects. Mass spectrometry analysis Revealed that xanthohumol binds to KRT18 protein. We, therefore, examined whether xanthohumol can elicit anti-cancer effects via modulation of KRT8. Here we report that xanthohumol inhibits ESCC cell proliferation and colony formation through the induction of cell cycle arrest at G1 phase and apoptosis, which was associated with decreased expression of KRT18. Moreover, xanthohumol inhibits the growth of KRT18 overexpressing ESCC patient-derived xenograft (PDX) tumors in mouse model.
Materials and Methods
Reagents
Xanthohumol (purity ≥ 97%) was purchased from Sichuan Weikeqi Biological Technology, Co., Ltd. (Chengdu, China). Antibodies to detect Keratin18 (ab668) was purchased from Abcam (Cambridge, MA, United States). Antibodies to examine Bcl-2 (CST 15071), Bax (CST 5023), cyclin D1 (CST 2922), cyclin D3 (CST 2936), COX IV (CST 4850), α-tubulin (CST 3873), and β-tubulin (CST 5346) expression was from Cell Signaling Technology (Beverly, MA, United States). Antibodies to detect β-Actin (sc-47778) and cytochrome c (sc-13156) were from Santa Cruz (Santa Cruz, CA, United States). Goat anti-rabbit antibody (ZB-2301) and goat anti-mouse antibody (ZB-2305) were obtained from ZSGB-Bio Company (Beijing, China).
Cell Culture
The human EC cell line KYSE30, KYSE70, KYSE410, KYSE450, and KYSE510 was purchased from the Type Culture Collection of the Chinese Academy of Sciences (Shanghai, China). Cells were cultured in RPMI-1640 containing penicillin (100 units/mL), streptomycin (100 μg/mL), and 10% fetal bovine serum (FBS, Biological Industries, Kibbutz Beit-Haemek, Israel). The human immortalized normal esophageal epithelial cell line, SHEE, was donated by Dr. Enmin Li from the Laboratory of Tumor Pathology (Shantou University Medical College, Shantou, China) (Shen et al., 2002). Cells were maintained in a humidified atmosphere at 37°C, contain 5% CO2. Cells were cytogenetically tested and authenticated before being frozen. Each vial of frozen cells was thawed and maintained in culture for a maximum of eight passages.
Cell Proliferation Assay
Cells (1.2 × 103 cells/well) were seeded in 96-well plates and incubated for 24 h, then treated with different doses of xanthohumol or DMSO (dimethyl sulfoxide, Sigma-Aldrich, St. Louis, MO, United States). Then measured cell proliferation using MTT [(4,5-dimethylthiazol-2-yl)-2,5-diphenyltetrazolium bromide, Ruitaibio, Beijing, China] agents at 24, 48 or 72 h. For foci formation assay, cells (1.2 × 103 cells/well) were seeded in 6-well plates and incubated for 24 h, then treated with different doses of xanthohumol or vehicle. After a week culture, stained with crystal violet (Solarbio, Beijing, China) and count the foci number. For anchorage-independent cell growth, cells (8 × 103 cells/well) suspended in complete medium and 0.3% agar with different concentration of xanthohumol or vehicle, with a base layer of 0.5% agar contain different concentration of xanthohumol or vehicle. Then cultured at 37°C in a 5% CO2 incubator for 2 weeks and visualized the colony under a microscope and counted using the Image-Pro Plus software (v.6.1) program (Media Cybernetics, Rockville, MD, United States).
Cell Cycle Analysis
KYSE30 cells (2 × 105) were seeded into 60-mm plates and 24 h later, treated with xanthohumol or vehicle for 24 h. Cells were fixed in 70% ethanol and stored at −20°C for 24 h. After staining with propidium iodide at room temperature (RT) for 15 min, analyzed cell cycle distribution using a BD FACS Calibur Flow Cytometer (BD Biosciences, San Jose, CA, United States).
Annexin V Apoptosis Assay
Cells (2 × 105) were seeded in 60-mm plates and incubated for 24 h then treated with xanthohumol or vehicle for 48 h. Cells were stained with annexin-V and propidium iodide at RT for 15 min. Apoptotic cells were analyzed using a BD FACS Calibur Flow Cytometer (BD Biosciences, San Jose, CA, United States).
Western Blot Assay
Cell pellets were lyzed in cell lysis buffer containing 50 mM Tris pH 8.0, 0.5–1% NP-40, 150 mM NaCl, protease inhibitor cocktail, and 1 mM PMSF. Supernatant fractions were collected after centrifuge at 14,000 rpm for 20 min and measured protein concentration by using BCA Quantification Kit (Solarbio, Beijing, China). Cell lysates were separated by SDS-PAGE gel and transferred to polyvinylidene fluoride membranes. Blocked the membranes with 5% milk for 1 h, were incubated with antibodies against Keratin18, Bcl-2, Bax, cyclin D1, cyclin D3, cytochrome c, COX IV (CST 4850), tubulin (ac Lys40), α-Tubulin, β-Tubulin, and β-Actin at 4°C overnight then washed three times with 1 × phosphate buffered saline with 0.05% Tween-20 (PBST) buffer. Membranes were incubated with the appropriate secondary antibody and the target protein bands detected using enhanced chemiluminescence (ECL) reagent (GE Healthcare Life Science, Little Chalfont, United Kingdom) and the Amersham Image 600 (GE, Milwaukee, WI, United States).
Ex vivo Pull-Down Assay
KYSE30 cell lysate were incubated with xanthohumol-Sepharose 4B beads (or only Sepharose 4B beads) in lysis buffer containing 50 mM Tris-HCl pH 7.5, 5 mM EDTA, 150 mM NaCl, 1 mM dithiothreitol, 0.01% NP-40 and 2 mg/ml bovine serum albumin at 4°C rotator, overnight. After incubation with gentle rocking, the beads were washed three times with washing buffer containing 50 mM Tris-HCl pH 7.5, 5 mM EDTA, 150 mM NaCl, 1 mM dithiothreitol and 0.01% NP-40. The binding was visualized by Western blot with KRT18 antibody.
Knockdown and Overexpression of KRT18
For lentiviral package, pMD2.0G, psPAX2, and shKRT18 plasmids were transfected into 293T cells using the SimpleFect transfection reagent (Signaling Dawn Biotech, Wuhan, China). The medium was changed after 12 h then cells were cultured at 37°C in a 5% CO2 incubator for 48 h. Viral particles were collected by filtration using a 0.45 μm filter, infected to KYSE30 cells together with polybrene (Millipore, Billerica, MA, United States) and incubated for 24 h. The medium was replaced freshly and selected with puromycin (2 mM). The effects of KRT18 knockdown on KYSE30 cells were examined by western blot and anchorage-independent cell growth assay. For the overexpression of KRT18, pCDNA3.1-3 × Flag-KRT18 (Youbio Biological Technology, Co., Ltd., Xi’an, China) plasmid or pCDNA3.1-3 × Flag (Youbio Biological Technology, Co., Ltd., Xi’an, China) plasmid were transfected into HEK293T cell line by using SimpleFect transfection reagent for 24 h. The expression of KRT18 on HEK293T cells were examined by western blot and its effect was evaluated with anchorage-independent cell growth assay.
Patient Derived Xenograft (PDX) Mouse Model
Female mice with severe combined immunodeficient (SCID, 6–8 weeks old) were used for these in vivo experiments. This study was approved by the Ethics Committee of Zhengzhou University (Zhengzhou, China). The cancer tissues were cut into small pieces and inoculated into SCID mice. After growing the tumor (∼100 mm3), the mice were divided into four groups as follows: (1) vehicle (n = 8); (2) 40 mg/kg of xanthohumol (n = 8); (3) 80 mg/kg of xanthohumol (n = 8). (4) 160 mg/kg of xanthohumol (n = 8). Xanthohumol was orally administered once a day for 64 days. Tumor volume was calculated from measurements of three diameters (length, width, and height) of the individual tumor base using the following formula: tumor volume (mm3) = (length × width × height × 0.52). Mice were monitored until tumors reached ∼1,000 mm3 average volume, at which time mice were euthanized and tumors extracted.
Immunohistochemical (IHC) Analysis
Paraffin embedded tumor tissues were prepared for IHC analysis. After antigen retrieval, tissues were treated with H2O2 for 5 min and blocked with 5% goat serum, then incubated with antibodies to detect Ki-67 and KRT18 at 4°C, overnight. After incubated with second antibody, used 3,30-Diaminobenzidine to visualize the target proteins and counterstained with hematoxylin. Then the sections were photographed and analyzed using the Image-Pro Plus software (v.6.0) program (Media Cybernetics, Rockville, MD, United States).
Statistical Analysis
All quantitative results are expressed as mean values ± SD. Used a two-tailed independent sample t-test to calculate the significant difference. A value of p < 0.05 was considered to be statistically significant.
Results
Xanthohumol Inhibits Proliferation of ESCC Cancer Cells
To evaluate the effect of xanthohumol on ESCC cells, we treated KYSE30 cells with xanthohumol (Figure 1). KYSE30 was derived from well-differentiated invasive ESCC resected from middle intra-thoracic esophagus of a 64-year-old Japanese man prior to treatment1 [Deutsche Sammlung von Mikroorganismen und Zellkulturen GmbH (DSMZ)]. Xanthohumol attenuated the anchorage-independent growth of KYSE30 cells in soft agar colony formation assay (Figure 1A). Treatment with xanthohumol significantly reduced the colony number and size as compared to control (Figure 1B). Foci assay also revealed that the number and size of colonies were significantly reduced in xanthohumol-treated group as compared to DMSO control group (Figure 1C). Figure 1D shows representative images of the colony formation in different treatment groups (Figure 1D). Cell proliferation was also inhibited after treat with xanthohumol in KYSE30 cells (Supplementary Figure S1). These results show that xanthohumol inhibits proliferation of ESCC cancer cells.
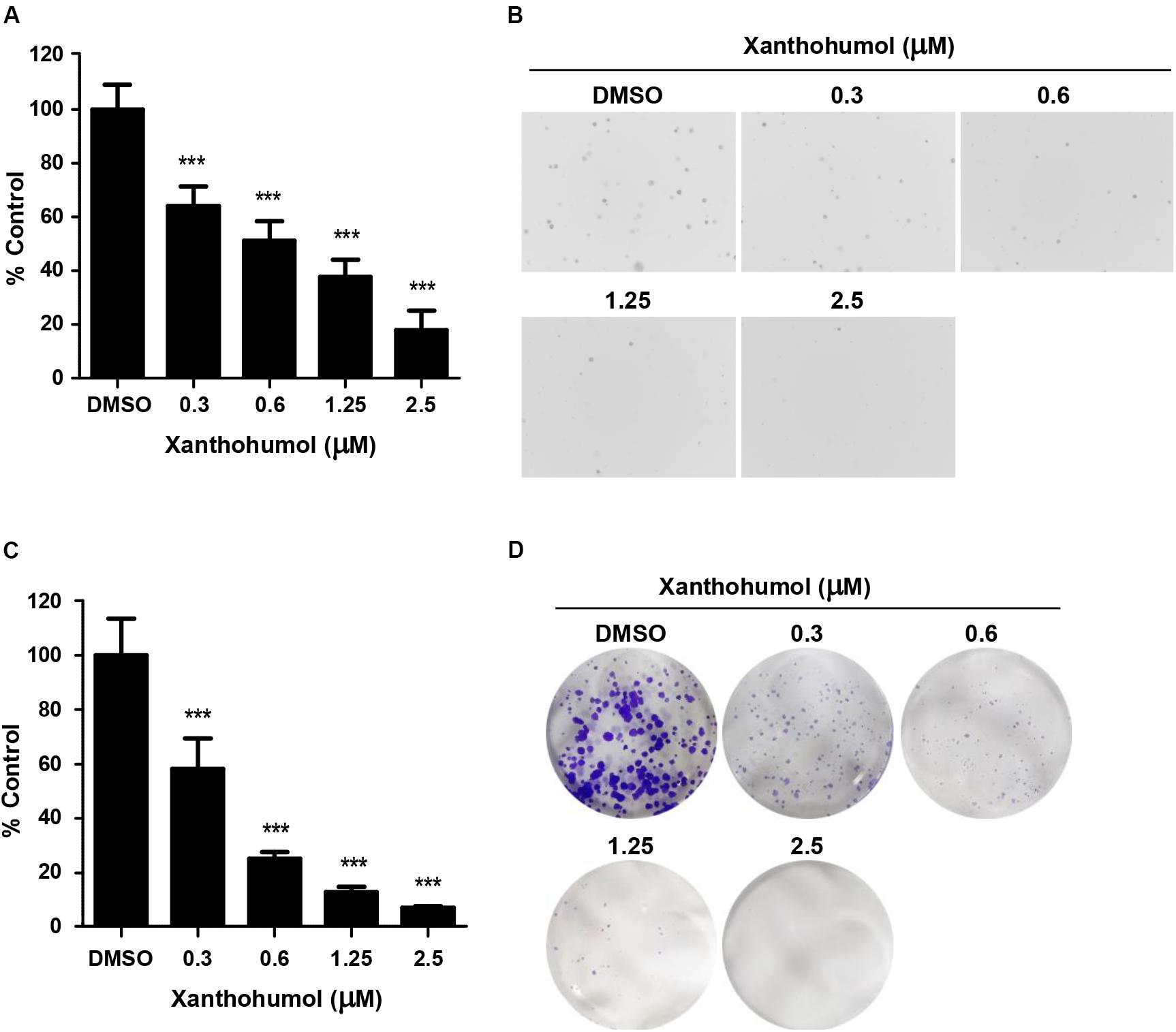
Figure 1. Xanthohumol inhibits the growth of ESCC cells. (A) Xanthohumol decreased colony formation in anchorage-independent growth of ESCC, the asterisks (*p < 0.05, **p < 0.01, ***p < 0.001) indicated a significant decrease in proliferation or colony number compared to control. (B) Representative photographs of the colony in soft agar. (C) Effects of xanthohumol on colony formation. (D) Representative photographs of the colony in Foci assay. Data were shown as means ± SD of values from triplicate samples and similar results were obtained from three independent experiments.
Xanthohumol Directly Binds With KRT18, Which Is a Potential Drug Target in ESCC
We identified a direct protein target of xanthohumol through the ex vivo pull-down assay. Incubation of KYSE30 cell lysate by adding xanthohumol-conjugated Sepharose 4B beads showed that xanthohumol was bound with KRT18 (Figure 2A). Then we evaluated the role of KRT18 in ESCC, we checked the expression of KRT18 in different ESCC cell lines and the normal SHEE esophageal cells. KRT18 was highly expressed in ESCC cell lines, especially in KYSE30 cell line (Figure 2B). To evaluate the expression of KRT18 in ESCC tissues, we checked the expression of KRT18 in an ESCC tumor microarray that included 37 pairs of adjacent tissues and cancer tissues and 40 more cancer tissues. KRT18 was significantly highly expressed in cancer tissues (Figures 2C,D).
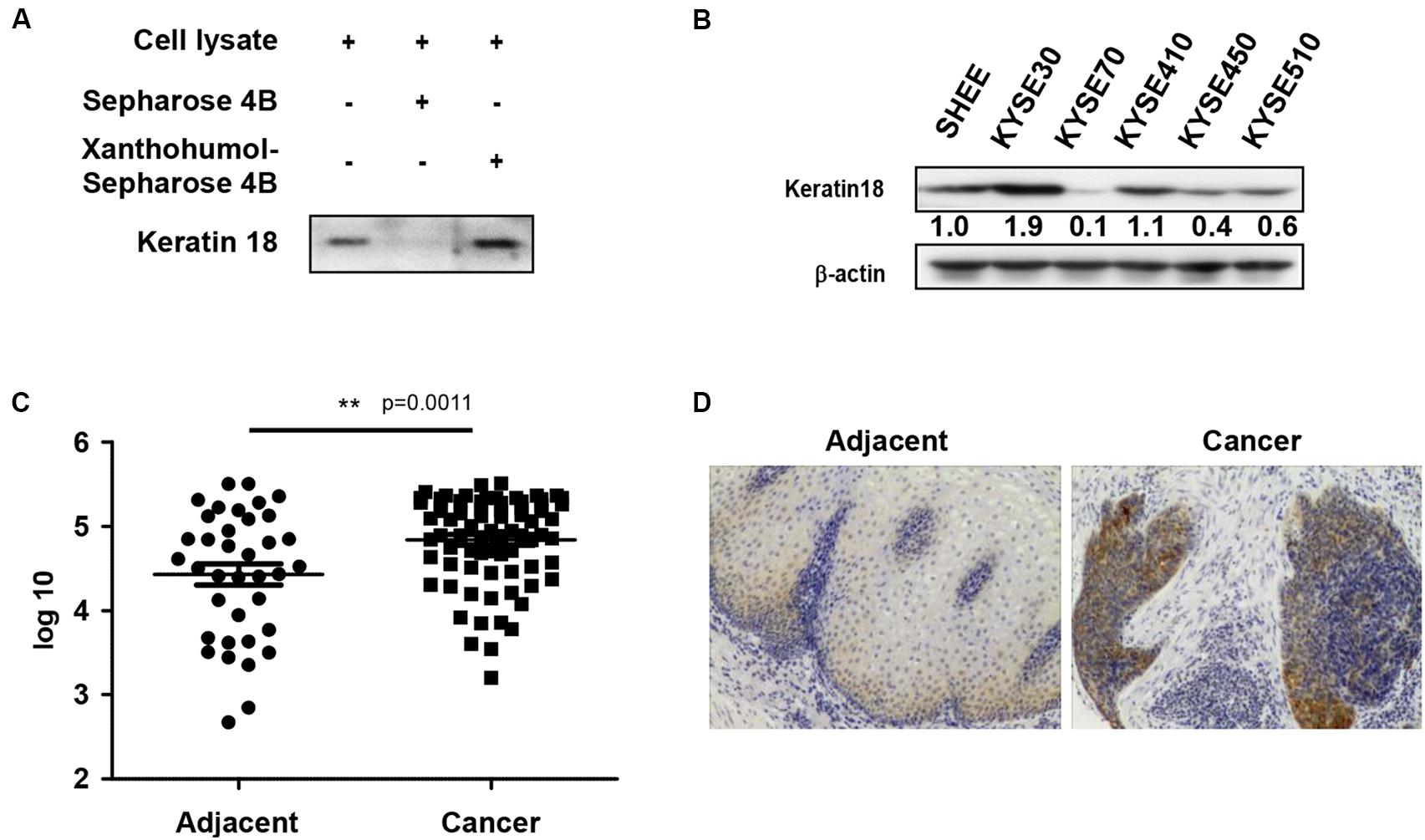
Figure 2. Xanthohumol binds with KRT18 and KRT18 is upregulated in ESCC. (A) Ex vivo pull-down assay showed xanthohumol bound to KRT18. (B) KRT18 is highly expressed in ESCC cells than that in normal cells. (C) The expression of KRT18 was examined by IHC analysis on an ESCC tumor tissue array. (D) Representative photographs of the IHC stained ESCC tumor tissues.
Xanthohumol Inhibits the Proliferation of ESCC Cells by Targeting KRT18
We knocked down KRT18 in KYSE30 cells by lentiviral shRNA silencing followed by selection of knockout colonies by puromycin treatment. Immunoblot analysis of KRT18 knockdown cells showed that number 1 and number 5 shRNA significantly decreased the expression of KRT18 (Figure 3A). The KRT18 knock down cells showed significantly reduced coloney formation in anchorage-independent growth assay and treatment with xanthohumol failed to further inhibit colony formation in KRT18 knock down cells (Figures 3B,C). On the other hand, overexpression of KRT18 in HEK293T cells restored the anchorage-independent colony formation, which was markedly reduced by treatment with xanthohumol (Figures 3D–F).
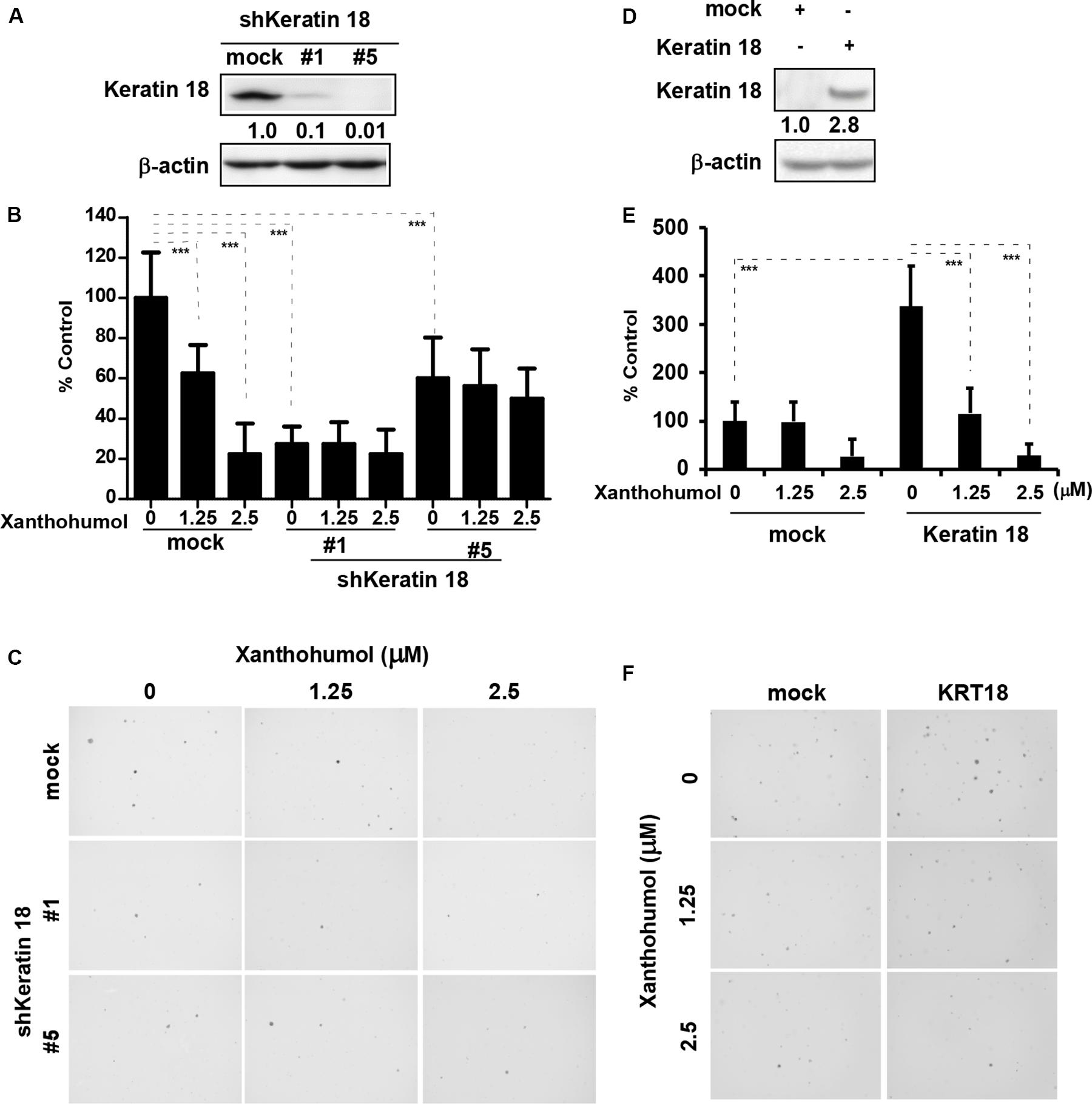
Figure 3. KRT18 is potential target of ESCC. (A) Knocking-down the expression of KRT18 in KYSE30 cell was detected by Western blotting. (B) Anchorage-independent cell growth was decreased after treated with xanthohumol in mock transfected cells or KRT18 knockdown cells but the colonies number did not further decrease after treated with xanthohumol in the KRT18 knockdown cells. (C) Representative photographs of the colonies. (D) KRT18 gene was overexpressed in HEK293T cells. Full length of KRT18 gene was transfect into HEK293T cells with simple-fect transfection reagent. Then it was confirmed the expression by Western blotting. (E) Overexpression of KRT18 promoted the colony formation in anchorage-independent cell growth, and was more sensitive to xanthohumol treatment compared to mock transfected. (F) Representative photographs of the colonies formed in soft agar. The asterisks (*p < 0.05, **p < 0.01, ***p < 0.001) indicated a significant difference between untreated control and xanthohumol-treated cells. Data were shown as means ± SD of values from triplicate samples and similar results were obtained from three independent experiments.
Xanthohumol Induces Cell Cycle G1 Phase Arrest and Cell Apoptosis in ESCC Cells
We examined the effect of xanthohumol on cell cycle and cell apoptosis. Treatment with xanthohumol for 24 h caused significant G1 phase cell cycle arrest and decreased cyclinD1, cyclinD3 expression in KYSE30 cells (Figures 4A–C). In addition, cells were treated with xanthohumol for 48 h to assess cell apoptosis. The annexinV + /PI + staining revealed that xanthohumol (2.5 μM) treatment induced apoptosis, which was supported by Figures 4D,E the increased expression of cleaved-PARP, cytochrome c and Bax, and inhibition of Bcl-2 in a concentration-dependent manner (Figure 4F). We also checked the location of cytochrome c after treat with xanthohumol, it was downregulated in mitochondrial protein lysate and upregulated in cytosolic proteins(Supplementary Figure S3)
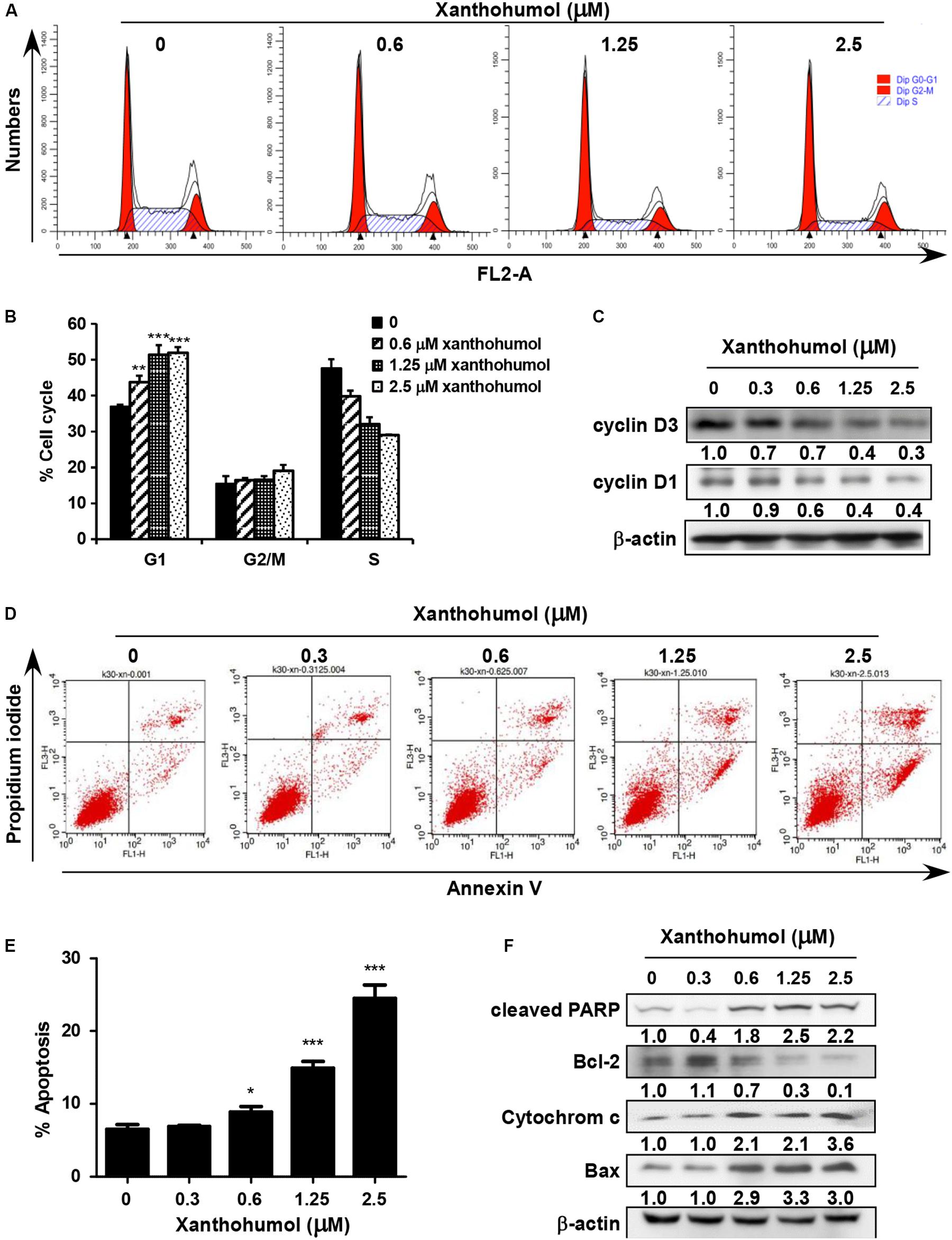
Figure 4. Xanthohumol induces cell cycle arrest at G1 phase and cell apoptosis. (A) Xanthohumol induced cell cycle arrest at G1 phase. (B) Xanthohumol induces cell cycle arrest at G1 phase in a concentration dependent manner. (C) Western blotting results showed the decreased expression of cyclinD1 and cyclinD3, G1 phase marker for cell cycle. (D) Xanthohumol treatment induced cell apoptosis. (E) Xanthohumol treatment can induce cell apoptosis in a concentration dependent manner. (F) Xanthohumol upregulated the expression of cell apoptosis markers like cleaved-PARP, Bcl-2, cytochrome c and Bax by determined with Western blotting. The asterisks (*p < 0.05, **p < 0.01, ***p < 0.001) indicated a significant difference between untreated control and xanthohumol -treated cells. Data were shown as means ± SD of values from triplicate samples and similar results were obtained from three independent experiments.
Xanthohumol Functions Through Promotion of KRT18 Degradation
We then explored the mechanisms by which xanthohumol inhibits ESCC cell proliferation through targeting KRT18. We first checked the mRNA level of KRT18 after treatment of cells with xanthohumol (Figures 5A,B). Results showed that xanthohumol did not influence the mRNA level of KRT18 (Figures 5A,B). Then we examined the KRT18 protein level after incubating cells with xanthohumol. The results revealed that xanthohumol decreased KRT18 protein expression and it was restored by MG132 pre-treatment (Figures 5C,D). These results indicated that xanthohumol may exert antiproliferative effects by enhancing KRT18 degradation.
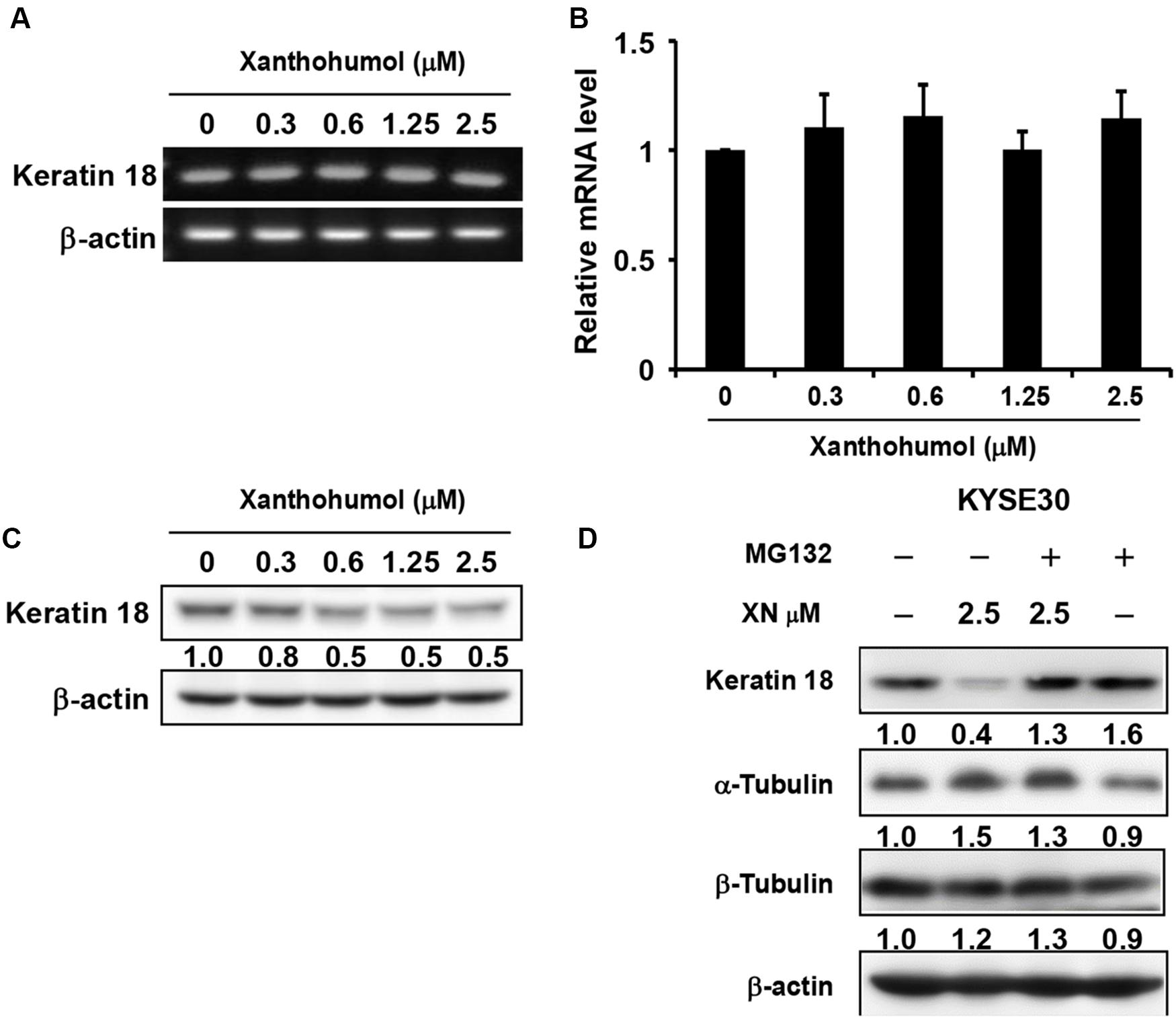
Figure 5. Xanthohumol promotes the degradation of KRT18 protein. (A) The mRNA level of KRT18 was examined after treated with xanthohumol for 48 h by RT-QPCR, AGE (agarose gel electrophoresis) results showed the intensity of KRT18 mRNA and β-Actin. (B) RT-QPCR results showed the mRNA level of KRT18 was not changed by xanthohumol treatment. (C) Western blotting results showed xanthohumol treatment decreased the protein level of KRT18. (D) MG132 treatment restored xanthohumol-induced KRT 18 degradation.
Xanthohumol Inhibits Patient-Derived Xenograft ESCC Growth in vivo
In order to explore the anti-tumor effects of xanthohumol in vivo, we used PDX tumor model in mice. We chose one patient case LEG32 to conduct experiment because KRT18 was highly expressed in LEG32 tumor tissues (Supplementary Figure S2). Cancer tissues were cut into small pieces and inoculated in to SCID mice. When the tumor volume is about 100 mm3, tumor-bearing mice were divided into four groups, each group containing eight mice. Vehicle or xanthohumol (40, 80, or 160 mg/kg body weight) was administered by gavage once a day for 64 days. Results indicated that xanthohumol significantly decreased the tumor growth relative to the control group without causing any change in mouse body weight (Figures 6A–D). Then we examined the expression of Ki67 and KRT18 in xenograft tumor sections using the IHC analysis. Xanthohumol remarkably suppressed the expression of both Ki67 and KRT18 protein in tumor tissues isolated from xanthohumol-treated group as compared to vehicle- treated group (Figures 6E,F).
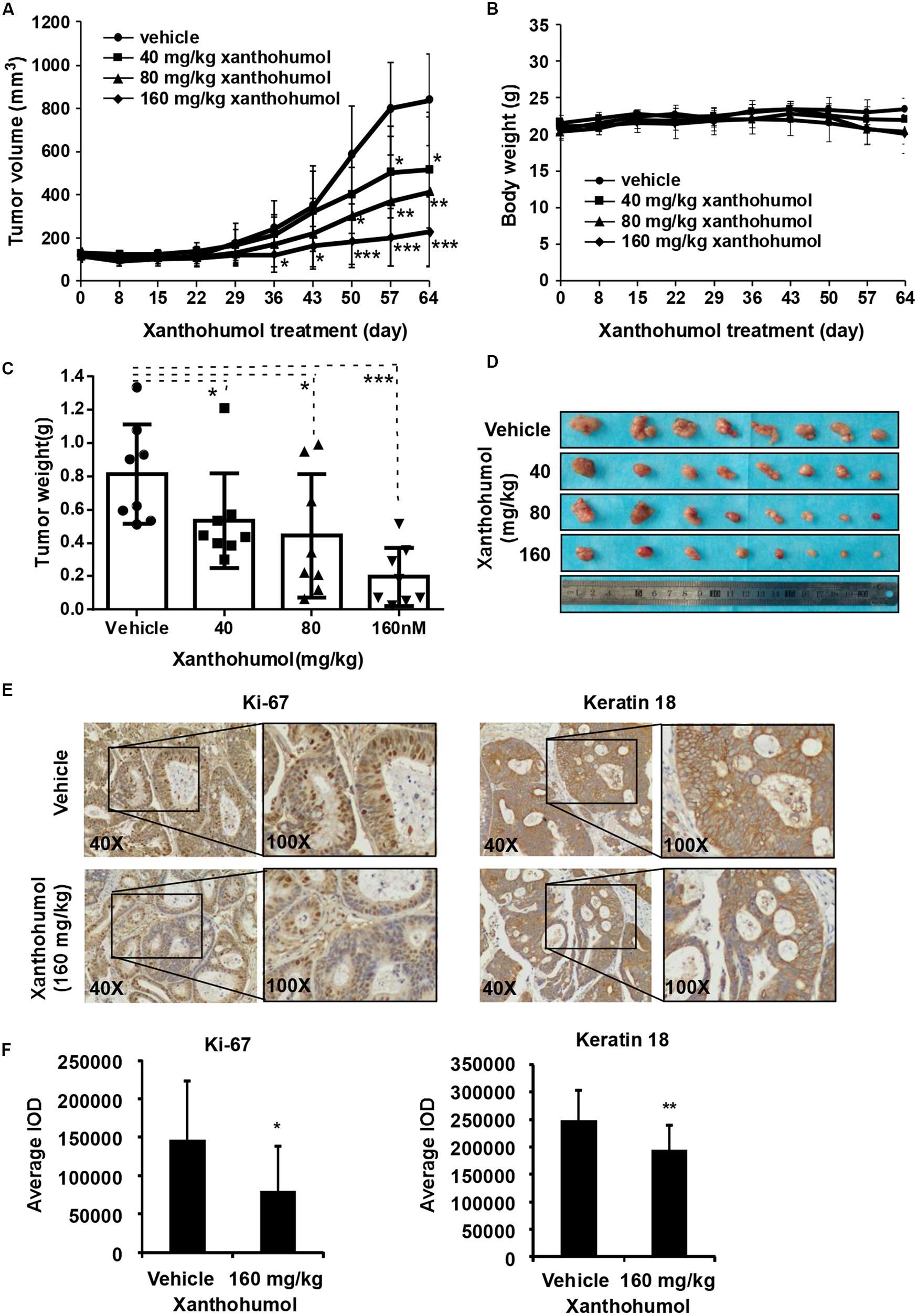
Figure 6. Effects of xanthohumol on patient-derived xenograft (PDX) tumor growth in vivo. (A) Xanthohumol treatment decreased the tumor volume compared to vehicle treatment. (B) Xanthohumol treatment had no effect on mice body weight. (C) Tumor weight was decreased after xanthohumol treatment compared to vehicle treatment. (D) Representative photographs of the tumors. (E) The expression of Ki-67 and KRT18 was examined by IHC analysis. Representative photographs showed the expression of these two proteins. (F) Quantitation of Ki-67 and KRT18 expression from the IHC results. Data are expressed as IOD values ± SD. The asterisks (*p < 0.05, **p < 0.01) indicated a significant decrease in Ki-67 and KRT18 expression in xanthohumol treated tissues compared to untreated controls.
Discussion
Esophageal cancer is a remarkable public health issue, especially in developing countries. Although a number of FDA approved therapies, such as growth factor receptor inhibitors and immunotherapies are currently used in clinical settings, none of these therapies are uniformly effective in all patient groups. Thus the hunt for novel therapies remains a constant venture to the research scientists (Shi et al., 2018; Song et al., 2018, 2019; Xu et al., 2018; Ji et al., 2019).
Because of the diverse biological activity and low systemic toxicity, many naturally occurring plant-derived chemicals have attracted considerable attention for new drug development. Xanthohumol, a prenylated flavonoid isolated from hops, has been reported to possess antibacterial, antiplatelet, anti-obesity and anticancer effects. It has been reported that xanthohumol inhibits colorectal cancer cells via downregulation of Hexokinases II-mediated glycolysis (Liu W. et al., 2019; Logan et al., 2019), exhibits anti-myeloma activity in vitro through inhibition of cell proliferation via the ERK and JNK-dependent mechanism (Slawinska-Brych et al., 2019) and exerts anticancer effects against gastric cancer (Wei et al., 2018). However, the roles of xanthohumol in ESCC were yet to be fully elucidated. Liu X. et al. (2019) reported that xanthohumol inhibits ESCC through blockade of Akt kinase activity. These authors also demonstrated that xanthohumol inhibited proliferation of ESCC cells having low Akt level thereby indicated that there might have additional mechanisms underlying anticancer effect of xanthohumol. In the present study, we have found that treatment of KYSE30 cells with xanthohumol resulted in significant inhibition of anchorage-independent colony formation (Figures 1A–D) and induction of G1 phase cell cycle arrest and apoptosis (Figures 4A–D). The alterations in the expression of apoptosis markers such as increased PARP cleavage, cytochrome c release and Bax induction along with inhibition of Bcl-2 expression further support the antitumor effect of xanthohuol on ESCC cells. We further identified KRT18 as a new target of xanthohumol, which directly binds with KRT18 (Figure 2A) and reduced the protein expression of KRT18 without affecting its mRNA transcript (Figures 5A–D). Moreover, shRNA-mediated knock down of KRT18 in KYSE30 cells reduced the colony formation and treatment of these cells with xanthohumol failed to further suppress the growth of KYSE30 cells. Conversely, overexpression of KRT18 enhances anchorage-independent growth, which was diminished by treatment with xanthohumol (Figures 3A–F). These data indicate xanthohumol is a potential chemotherapeutic compound for ESCC, and KRT18 is a primary target of xanthohumol.
Keratin 18 (KRT18) is a member of the intermediate filament family of cytoskeletal protein. Its expression is upregulated in ESCC tissues (Kilic-Baygutalp et al., 2016). However, the implication of elevated expression of KRT18 in ESCC cells is not clear. It has been reported that high Keratin18 predicts aggressive hepatocellular cancer phenotype (Golob-Schwarzl et al., 2019) and serves as a diagnostic and prognostic factor for acute alcoholic hepatitis (Vatsalya et al., 2019). In contrast, Bettermann et al. (2016) have demonstrated that KRT18 leads to the development of steatohepatitis and liver carcinogenesis in old-age mice. Thus, the role of KRT18 in hepatocellular carcinoma requires further study. Several other studies have shown that KRT18 plays an important role in gastric cancer, colorectal cancer, prostate cancer, and breast cancer (Goliwas et al., 2017; Jedroszka et al., 2017; Peduk et al., 2018; Zhang et al., 2019). In our present study, we found KRT18 is highly expressed in ESCC cell lines and ESCC tissues compared with normal tissues (Figures 2B–D). However, further studies are warranted to elucidate the mechanism underlying elevation of KRT18 during tumorigenesis.
Patient-derived tumor xenografts (PDXs) engrafted into immune-compromised rodents such as SCID mice is a useful preclinical model of human tumor growth. PDX models are used to screen for biomarkers and predict clinical trial drug response, because these models conserve original tumor characteristics such as heterogeneity, complexity, and molecular diversity (Kopetz et al., 2012; Tentler et al., 2012; Nunes et al., 2015; Park et al., 2016). Therefore, we also evaluated the effect of xanthohumol on the growth of ESCC PDX tumors in mice. Compared with control group, the tumor volume and tumor weight in the xanthohumol-treated mice were significantly decreased (Figures 6A–D). We also checked the expression of Ki67 and KRT18 in the tumors by using IHC assay, which revealed that both the markers are downregulated in the xanthohumol-treated tumors (Figures 6E,F). The PDX models can supply important information for the translation from preclinical studies to clinical studies. In summary, our study suggested that xanthohumol can inhibit the progression of ESCC, at least in part by binding with KRT18 and promoting KRT18 degradation.
Data Availability Statement
The datasets generated for this study are available on request to the corresponding author.
Ethics Statement
The animal study was reviewed and approved by Ethics Committee of Zhengzhou University (Zhengzhou, China).
Author Contributions
SY, MS, M-HL, and ZD are involved in study concept and design, acquisition of data, analysis and interpretation of data, and drafting of the manuscript. SY, MS, RZ, XL, WK, JL, and YK performed experiments analysis and interpretation of data. KL, CZ, and J-HS have supported the materials. SY, M-HL, and ZD wrote the manuscript. ZD and M-HL had supervision of all study.
Funding
This work was supported by grant funding from the National Natural Science Foundation of China NSFC81672767, NSFC81972839 and the Key Program of Henan Province, China Grant Nos. 161100510300 and Henan Provincial Government, China.
Conflict of Interest
The authors declare that the research was conducted in the absence of any commercial or financial relationships that could be construed as a potential conflict of interest.
Supplementary Material
The Supplementary Material for this article can be found online at: https://www.frontiersin.org/articles/10.3389/fcell.2020.00366/full#supplementary-material
Footnotes
References
Ahmed, O., Ajani, J. A., and Lee, J. H. (2019). Endoscopic management of esophageal cancer. World J. Gastrointest. Oncol. 11, 830–841.
Barsouk, A., Rawla, P., Hadjinicolaou, A. V., Aluru, J. S., and Barsouk, A. (2019). Targeted therapies and immunotherapies in the treatment of esophageal cancers. Med. Sci. 7:100. doi: 10.3390/medsci7100100
Bettermann, K., Mehta, A. K., Hofer, E. M., Wohlrab, C., Golob-Schwarzl, N., Svendova, V., et al. (2016). Keratin 18-deficiency results in steatohepatitis and liver tumors in old mice: a model of steatohepatitis-associated liver carcinogenesis. Oncotarget 7, 73309–73322. doi: 10.18632/oncotarget.12325
Bray, F., Ferlay, J., Soerjomataram, I., Siegel, R. L., and Torre, L. A. (2018). Global cancer statistics 2018: GLOBOCAN estimates of incidence and mortality worldwide for 36 cancers in 185 countries. CA Cancer J. Clin. 68, 394–424. doi: 10.3322/caac.21492
Chen, W., Zheng, R., Baade, P. D., Zhang, S., Zeng, H., Bray, F., et al. (2016). Cancer statistics in China, 2015. CA Cancer J. Clin. 66, 115–132.
Davidson, M., and Starling, N. (2016). Trastuzumab in the management of gastroesophageal cancer: patient selection and perspectives. Oncol. Targets Ther. 9, 7235–7245. doi: 10.2147/OTT.S100643
Goliwas, K. F., Richter, J. R., Pruitt, H. C., Araysi, L. M., Anderson, N. R., Samant, R. S., et al. (2017). Methods to evaluate cell growth, viability, and response to treatment in a tissue engineered breast cancer model. Sci. Rep. 7:14167. doi: 10.1038/s41598-017-14326-8
Golob-Schwarzl, N., Bettermann, K., Mehta, A. K., Kessler, S. M., Unterluggauer, J., Krassnig, S., et al. (2019). High Keratin 8/18 ratio predicts aggressive hepatocellular cancer phenotype. Transl. Oncol. 12, 256–268. doi: 10.1016/j.tranon.2018.10.010
Guo, D., Zhang, B., Liu, S., and Jin, M. (2018). Xanthohumol induces apoptosis via caspase activation, regulation of Bcl-2, and inhibition of PI3K/Akt/mTOR-kinase in human gastric cancer cells. Biomed. Pharmacother. 106, 1300–1306. doi: 10.1016/j.biopha.2018.06.166
Huang, Z. H., Ma, X. W., Zhang, J., Li, X., and Lai, N. L. (2018). Cetuximab for esophageal cancer: an updated meta-analysis of randomized controlled trials. BMC Cancer 18:1170. doi: 10.1186/s12885-018-5040-z
Jedroszka, D., Orzechowska, M., Hamouz, R., Gorniak, K., and Bednarek, A. K. (2017). Markers of epithelial-to-mesenchymal transition reflect tumor biology according to patient age and Gleason score in prostate cancer. PLoS One 12:e0188842. doi: 10.1371/journal.pone.0188842
Ji, Y., Liu, Y., Xue, N., Du, T., Wang, L., Huang, R., et al. (2019). Cryptotanshinone inhibits esophageal squamous-cell carcinoma in vitro and in vivo through the suppression of STAT3 activation. Oncol. Targets Ther. 12, 883–896. doi: 10.2147/OTT.S187777
Jiang, C. H., Sun, T. L., Xiang, D. X., Wei, S. S., and Li, W. Q. (2018). Anticancer activity and mechanism of xanthohumol: a prenylated flavonoid from hops (Humulus lupulus L.). Front. Pharmacol. 9:530. doi: 10.3389/fphar.2018.00530
Kilic-Baygutalp, N., Ozturk, N., Orsal-Ibisoglu, E., Gundogdu, B., Ozgeris, F. B., Bakan, N., et al. (2016). Evaluation of serum HGF and CK18 levels in patients with esophageal cancer. Genet. Mol. Res. 15:15038583. doi: 10.4238/gmr.15038583
Kopetz, S., Lemos, R., and Powis, G. (2012). The promise of patient-derived xenografts: the best laid plans of mice and men. Clin. Cancer Res. 18, 5160–5162. doi: 10.1158/1078-0432.CCR-12-2408
Ku, G. Y. (2017). Systemic therapy for esophageal cancer: chemotherapy. Chin. Clin. Oncol. 6:49. doi: 10.21037/cco.2017.07.06
Lagergren, J., Smyth, E., Cunningham, D., and Lagergren, P. (2017). Oesophageal cancer. Lancet 390, 2383–2396.
Lebherz-Eichinger, D., Krenn, C. G., and Roth, G. A. (2013). Keratin 18 and heat-shock protein in chronic kidney disease. Adv. Clin. Chem. 62, 123–149. doi: 10.1016/b978-0-12-800096-0.00003-2
Li, F., Yao, Y., Huang, H., Hao, H., and Ying, M. (2018). Xanthohumol attenuates cisplatin-induced nephrotoxicity through inhibiting NF-κB and activating Nrf2 signaling pathways. Int. Immunopharmacol. 61, 277–282. doi: 10.1016/j.intimp.2018.05.017
Lin, M., Xiang, D., Chen, X., and Huo, H. (2019). Role of characteristic components of humulus lupulus in promoting human health. J. Agric. Food Chem. 67, 8291–8302. doi: 10.1021/acs.jafc.9b03780
Liu, M., Hansen, P. E., Wang, G., Qiu, L., Dong, J., Yin, H., et al. (2015). Pharmacological profile of xanthohumol, a prenylated flavonoid from hops (Humulus lupulus). Molecules 20, 754–779. doi: 10.3390/molecules20010754
Liu, W., Li, W., Liu, H., and Yu, X. (2019). Xanthohumol inhibits colorectal cancer cells via downregulation of Hexokinases II-mediated glycolysis. Int. J. Biol. Sci. 15, 2497–2508. doi: 10.7150/ijbs.37481
Liu, X., Song, M., Wang, P., Zhao, R., Chen, H., Zhang, M., et al. (2019). Targeted therapy of the AKT kinase inhibits esophageal squamous cell carcinoma growth in vitro and in vivo. Int. J. Cancer 145, 1007–1019. doi: 10.1002/ijc.32285
Logan, I. E., Miranda, C. L., Lowry, M. B., Maier, C. S., and Stevens, J. F. (2019). Antiproliferative and cytotoxic activity of xanthohumol and its non-estrogenic derivatives in colon and hepatocellular carcinoma cell lines. Int. J. Mol. Sci. 20:1203. doi: 10.3390/ijms20051203
Lyons, T. G., and Ku, G. Y. (2017). Systemic therapy for esophagogastric cancer: targeted therapies. Chin. Clin. Oncol. 6:48. doi: 10.21037/cco.2017.07.02
Mayanagi, S., Irino, T., Kawakubo, H., and Kitagawa, Y. (2019). Neoadjuvant treatment strategy for locally advanced thoracic esophageal cancer. Ann. Gastroenterol. Surg. 3, 269–275. doi: 10.1002/ags3.12243
Nakamura, K., Kato, K., Igaki, H., Ito, Y., Mizusawa, J., Ando, N., et al. (2013). Japan esophageal oncology group/japan clinical oncology, three-arm phase III trial comparing cisplatin plus 5-FU (CF) versus docetaxel, cisplatin plus 5-FU (DCF) versus radiotherapy with CF (CF-RT) as preoperative therapy for locally advanced esophageal cancer (JCOG1109, NExT study). Jpn. J. Clin. Oncol. 43, 752–755. doi: 10.1093/jjco/hyt061
Nunes, M., Vrignaud, P., Vacher, S., Richon, S., Lievre, A., Cacheux, W., et al. (2015). Evaluating patient-derived colorectal cancer xenografts as preclinical models by comparison with patient clinical data. Cancer Res. 75, 1560–1566. doi: 10.1158/0008-5472.CAN-14-1590
Park, D., Wang, D., Chen, G., and Deng, X. (2016). Establishment of Patient-derived Xenografts in Mice. Biol. Protoc. 6, 225–230. doi: 10.21769/BioProtoc.2008
Peduk, S., Tatar, C., Dincer, M., Ozer, B., Kocakusak, A., Citlak, G., et al. (2018). The role of serum CK18, TIMP1, and MMP-9 levels in predicting R0 resection in patients with gastric cancer. Dis. Mark. 2018:5604702. doi: 10.1155/2018/5604702
Saito, K., Matsuo, Y., Imafuji, H., Okubo, T., Maeda, Y., Sato, T., et al. (2018). Xanthohumol inhibits angiogenesis by suppressing nuclear factor-κB activation in pancreatic cancer. Cancer Sci. 109, 132–140. doi: 10.1111/cas.13441
Shen, Z.-Y., Xu, L. Y., Li, E.-M., Shen, J., Zheng, R.-M., Cai, W.-J., et al. (2002). Immortal phenotype of the esophageal epithelial cells in the process of immortalization. Int. J. Mol. Med. 10, 641–646.
Shi, Y., Liu, X., Fredimoses, M., Song, M., Chen, H., Liu, K., et al. (2018). FGFR2 regulation by picrasidine Q inhibits the cell growth and induces apoptosis in esophageal squamous cell carcinoma. J. Cell. Biochem. 119, 2231–2239. doi: 10.1002/jcb.26385
Slawinska-Brych, A., Zdzisinska, B., Czerwonka, A., Mizerska-Kowalska, M., Dmoszynska-Graniczka, M., Stepulak, A., et al. (2019). Xanthohumol exhibits anti-myeloma activity in vitro through inhibition of cell proliferation, induction of apoptosis via the ERK and JNK-dependent mechanism, and suppression of sIL-6R and VEGF production. Biochim. Biophys. Acta Gen. Subj. 1863:129408. doi: 10.1016/j.bbagen.2019.08.001
Smyth, E. C., Lagergren, J., Fitzgerald, R. C., Lordick, F., Shah, M. A., Lagergren, P., et al. (2017). Oesophageal cancer. Nat. Rev. Dis. Primers 3:17048.
Song, M., Liu, X., Liu, K., Zhao, R., Huang, H., Shi, Y., et al. (2018). Targeting AKT with oridonin inhibits growth of esophageal squamous cell carcinoma in vitro and patient-derived xenografts in vivo. Mol. Cancer Ther. 17, 1540–1553. doi: 10.1158/1535-7163.MCT-17-0823
Song, M., Yin, S., Zhao, R., Liu, K., Kundu, J. K., Shim, J. H., et al. (2019). (S)-10-Hydroxycamptothecin inhibits esophageal squamous cell carcinoma growth in vitro and in vivo via decreasing topoisomerase I enzyme activity. Cancers 11:1964. doi: 10.3390/cancers11121964
Sun, Z., Zhou, C., Liu, F., Zhang, W., Chen, J., Pan, Y., et al. (2018). Inhibition of breast cancer cell survival by Xanthohumol via modulation of the Notch signaling pathway and. Oncol. Lett. 15, 908–916. doi: 10.3892/ol.2017.7434
Teicher, B. A. (2008). Next generation topoisomerase I inhibitors: rationale and biomarker strategies. Biochem. Pharmacol. 75, 1262–1271. doi: 10.1016/j.bcp.2007.10.016
Tentler, J. J., Tan, A. C., Weekes, C. D., Jimeno, A., Leong, S., Pitts, T. M., et al. (2012). Patient-derived tumour xenografts as models for oncology drug development. Nat. Rev. Clin. Oncol. 9, 338–350. doi: 10.1038/nrclinonc.2012.61
Testa, U., Castelli, G., and Pelosi, E. (2017). Esophageal cancer: genomic and molecular characterization, stem cell compartment and clonal evolution. Medicines 4:67. doi: 10.3390/medicines4030067
Vatsalya, V., Cave, M. C., Kong, M., Gobejishvili, L., Falkner, K. C., Craycroft, J., et al. (2019). Keratin 18 is a diagnostic and prognostic factor for acute alcoholic hepatitis. Clin. Gastroenterol. Hepatol. S1542–3565, 31390–31394. doi: 10.1016/j.cgh.2019.11.050
Wei, S., Sun, T., Du, J., Zhang, B., and Xiang, D. (2018). Xanthohumol, a prenylated flavonoid from Hops, exerts anticancer effects against gastric cancer in vitro. Oncol. Rep. 40, 3213–3222. doi: 10.3892/or.2018.6723
Xu, W. W., Zheng, C. C., Huang, Y. N., Chen, W. Y., Yang, Q. S., Ren, J. Y., et al. (2018). Synephrine hydrochloride suppresses esophageal cancer tumor growth and metastatic potential through inhibition of galectin-3-AKT/ERK signaling. J. Agric. Food Chem. 66, 9248–9258. doi: 10.1021/acs.jafc.8b04020
Yang, C. S., Chen, X., and Tu, S. (2016). Etiology and prevention of esophageal cancer. Gastro. Tumors 3, 3–16.
Keywords: xanthohumol, keratin18, esophageal squamous cell carcinoma (ESCC), protein stability, cell growth
Citation: Yin S, Song M, Zhao R, Liu X, Kang WK, Lee JM, Kim YE, Zhang C, Shim J-H, Liu K, Dong Z and Lee M-H (2020) Xanthohumol Inhibits the Growth of Keratin 18-Overexpressed Esophageal Squamous Cell Carcinoma in vitro and in vivo. Front. Cell Dev. Biol. 8:366. doi: 10.3389/fcell.2020.00366
Received: 12 February 2020; Accepted: 24 April 2020;
Published: 19 May 2020.
Edited by:
Venkaiah Betapudi, United States Department of Homeland Security, United StatesReviewed by:
Manoj Kumar Kashyap, Amity University Gurgaon, IndiaDhyan Chandra, University at Buffalo, United States
Copyright © 2020 Yin, Song, Zhao, Liu, Kang, Lee, Kim, Zhang, Shim, Liu, Dong and Lee. This is an open-access article distributed under the terms of the Creative Commons Attribution License (CC BY). The use, distribution or reproduction in other forums is permitted, provided the original author(s) and the copyright owner(s) are credited and that the original publication in this journal is cited, in accordance with accepted academic practice. No use, distribution or reproduction is permitted which does not comply with these terms.
*Correspondence: Zigang Dong, zgdong@hci-cn.org; Mee-Hyun Lee, mhlee@hci-cn.org; mhyun_lee@hanmail.net
†These authors have contributed equally to this work