- 1Department of Orthopedic Surgery, Baylor College of Medicine, Houston, TX, United States
- 2Biionix Cluster, Internal Medicine, College of Medicine, University of Central Florida, Orlando, FL, United States
Tissue engineered hyaline cartilage is plagued by poor mechanical properties largely due to inadequate type II collagen expression. Of note, commonly used defined chondrogenic media lack 14 vitamins and minerals, some of which are implicated in chondrogenesis. Type II collagen promoter-driven Gaussia luciferase was transfected into ATDC5 cells to create a chondrogenic cell with a secreted-reporter. The reporter cells were used in an aggregate-based chondrogenic culture model to develop a high-throughput analytic platform. This high-throughput platform was used to assess the effect of vitamins and minerals, alone and in combination with TGFβ1, on COL2A1 promoter-driven expression. Significant combinatorial effects between vitamins, minerals, and TGFβ1 in terms of COL2A1 promoter-driven expression and metabolism were discovered. An “optimal” continual supplement of copper and vitamin K in the presence of TGFβ1 gave a 2.5-fold increase in COL2A1 promoter-driven expression over TGFβ1 supplemented media alone in ATDC5 cells.
Introduction
Osteoarthritis is a leading cause of disability with annual United States healthcare costs in excess of $500 billion and is the impetus for this study (Helmick et al., 2008; Centers for disease control and prevention, 2009). This laboratory has investigated media formulations and expansion surfaces to optimize cartilage formation for multiple applications (Henderson et al., 2007, 2010; Weidenbecher et al., 2007, 2008; Kean and Dennis, 2015; Kean et al., 2016; Dennis et al., 2018). Supplements include BMP (Mounts et al., 2012) and thyroxine (Whitney et al., 2018); nevertheless, engineered cartilage invariably contained type II collagen at levels far lower than native (Whitney et al., 2014), as is the scourge of the field. Current methods used to optimize medium conditions are tedious and inefficient. The goal of this study was to streamline the method to screen for optimal culture conditions using a high-throughput assay of a Gaussia luciferase reporter system linked to a type II collagen promoter. This initial study was applied to the optimization of the base medium components used to differentiate chondrocytes, using ATDC5 cells as a model system.
Multiple laboratories have focused on methods to engineer functional cartilage for various applications. In many instances, the mechanical properties fall short of that of native cartilage tissue. One major concern is that the diminished biomechanical properties of engineered cartilage are predominantly a result of insufficient production of type II collagen, which is the most abundant molecule in cartilage after water (Sophia Fox et al., 2009). Results in our laboratory have shown type II collagen content to be approximately 20% of that in native tissue (Whitney et al., 2018). Other laboratories have reported similar results, such as those of Sato et al. (2017) who showed type II collagen levels from cultured human MSCS at 20% that of native tissue, even after enhancement by the addition of epigallocatechin-3-gallate, which doubled the type II collagen output. Similar results were shown by Gemmiti and Guldberg (2006) where collagen content of bioreactor-grown bovine chondrocytes was 25% that of native collagen content, and in a study by Shahin and Doran (2011) where type II collagen levels were 18% that of native tissue. Other studies have shown collagen levels at less than 10% of native tissue (Mahmoudifar and Doran, 2010; Tian et al., 2013).
Many researchers, including ourselves (Kean and Dennis, 2015; Kean et al., 2016; Dennis et al., 2018; Whitney et al., 2018), use serum-free chondrogenic media to engineer cartilage in vitro (Johnstone et al., 1998). Upon examination of the composition of this medium, it was noted that several vitamins and minerals potentially relevant to chondrogenesis were absent (Table 1). To address this disparity and examine their potential effects on chondrogenesis, a high-throughput assay was developed based on a COL2A1-Gaussia luciferase reporter system using ATDC5 cells formatted as aggregates in 96-well plates. The dose responses of each vitamin and mineral was assessed over time. TGFβ1 was used as a positive control, as it has previously been reported to upregulate chondrogenesis in ATDC5s (Han et al., 2005) and other mammalian cells (Puetzer et al., 2010). Combinations of vitamins and minerals which showed positive increases in type II collagen promoter activity were tested in the presence of TGFβ1. This article shows proof of concept data for a high-throughput screen and establishes an improved chondrogenic medium for ATDC5 cells.
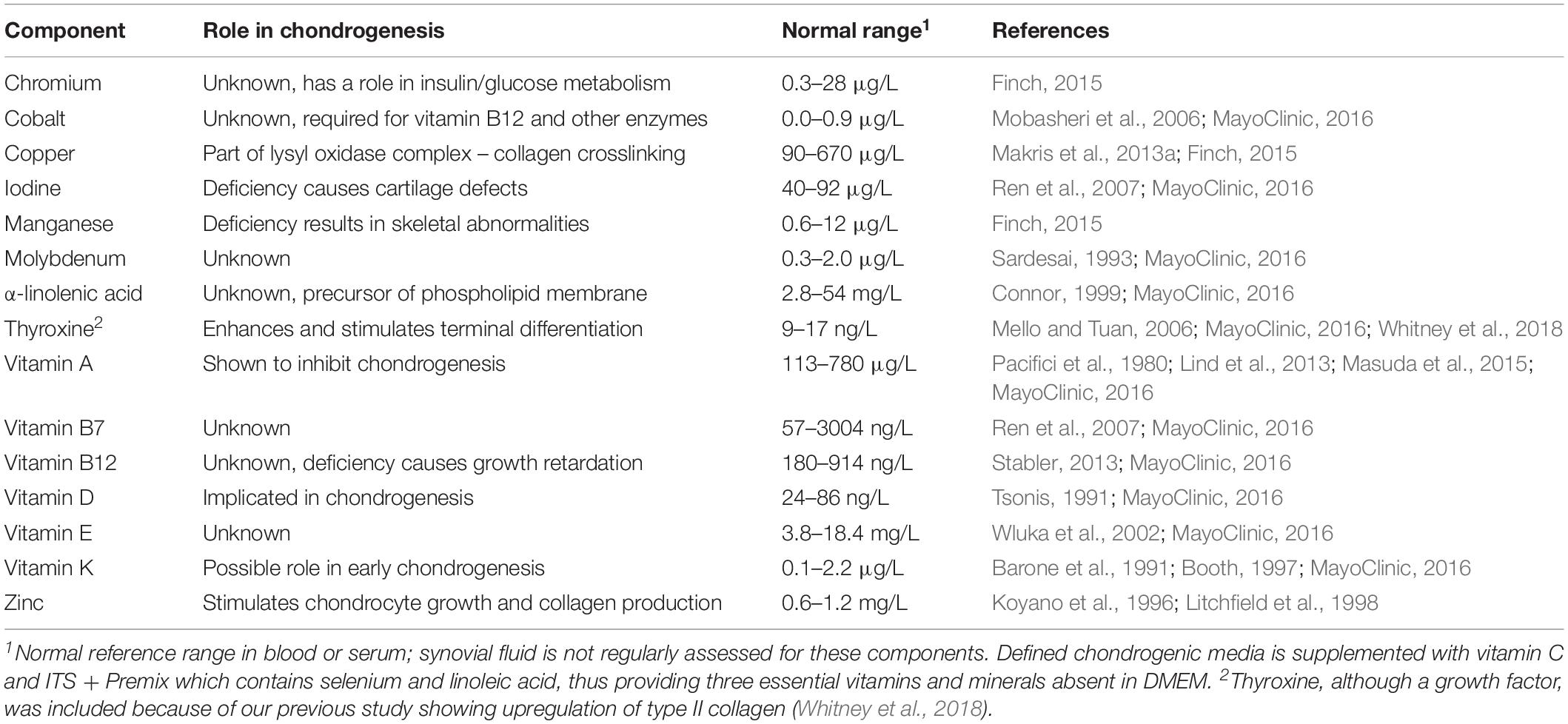
Table 1. Vitamins and minerals absent in DMEM [and therefore defined chondrogenic media (Kean and Dennis, 2015)].
Somewhat surprisingly, the common cell culture medium Dulbecco’s Modified Eagle’s Medium (DMEM) used in cell culture of chondrocytes (and other cells) lacks several vitamins and minerals that are defined as essential (see Supplementary Table S1 for common media comparison). It is likely that some or all of these essential components are provided by the supplementation of fetal bovine serum during expansion, but these components are clearly absent during chondrogenic culture using defined medium (Johnstone et al., 1998; Kean and Dennis, 2015). Chondrogenic media compositions have evolved to defined media from that originally reported by Ahrens et al. (1977) to a reduced serum medium described by Ballock and Reddi (1994), then to a serum-free defined medium published by Johnstone et al. (1998); our current chondrogenic medium is an adaptation of the last (Kean et al., 2016). Table 1 shows the essential vitamins and minerals lacking in DMEM, a base medium commonly used in chondrogenic differentiation by us and others. Indeed, Makris et al. (2013b) found that copper supplementation of this base medium enhances collagen crosslinking and mechanical properties in tissue engineered cartilage. Because synovial fluid is rarely sampled from healthy patients, tests in our studies were based on the normal range of vitamins and minerals found in serum. Yazar et al. (2005) found mean zinc and copper levels of 114.1 and 280.8 μg/L, respectively in the synovial fluid. Niedermeier et al. (1962) in a semi-quantitative assay found mean copper, chromium and manganese levels of 210, 17, and 21 μg/L, respectively from synovial fluid extracted from cadaver knees. These data support the use of the normal serum range in our studies. To investigate this and other media supplements on chondrogenic differentiation, we assayed for Gaussia luciferase levels in the conditioned medium of ATDC5 cells, a commonly used chondrocyte cell line derived from mouse teratocarcinoma AT805, transduced with lentiviral Gaussia luciferase under the control of the COL2A1 promoter (col2gLuc; HPRM22364-LvPG02, GeneCopoeia, Inc.). As a surrogate for testing RNA expression of type II collagen, a destructive assay, a secreted Gaussia luciferase assay was developed. Further, the use of the secreted form of luciferase allows for the temporal assessment of COL2A1 promoter-driven expression in the conditioned medium of aggregate cultures.
Results
Pseudolentiviral Particle Production, Infection, and Selection
Pseudolentiviral particles of control (eGFP) and col2gLuc were successfully separately produced and used to infect ATDC5 cells. Dilution titration of infected cells with eGFP determined a multiplicity of infection (MOI) of 29. Calculation of infectious particle concentration by qPCR determined this concentration to be approximately the same for col2gLuc in terms of viral particles per μL (1.9 × 107 and 1.6 × 107 for eGFP and col2gLuc, respectively), this equates to an approximate MOI of 25 for the col2gLuc studies.
Basal ATDC5 Culture Optimization
Standard cell culture conditions are generally performed at supraphysiological oxygen tensions, for chondrocytes and tissue engineered cartilage this can be particularly detrimental in terms of extracellular matrix production. In order to demonstrate that there were limited effects on Gaussia luciferase a comparison between standard (20%) oxygen tension and physiological (5%) oxygen tension experiment was performed. In initial experiments, chondrocytes were plated in high-density pellet cultures at 50,000 and 100,000 cells/pellet at both 20% oxygen and 5% oxygen [physioxia (Kean and Dennis, 2015; Kean et al., 2016)], with similar Gaussia luciferase responses to TGFβ1 in each (Figure 1). Data were averaged and the z-factor calculated according to the formula described by Zhang et al. (1999).
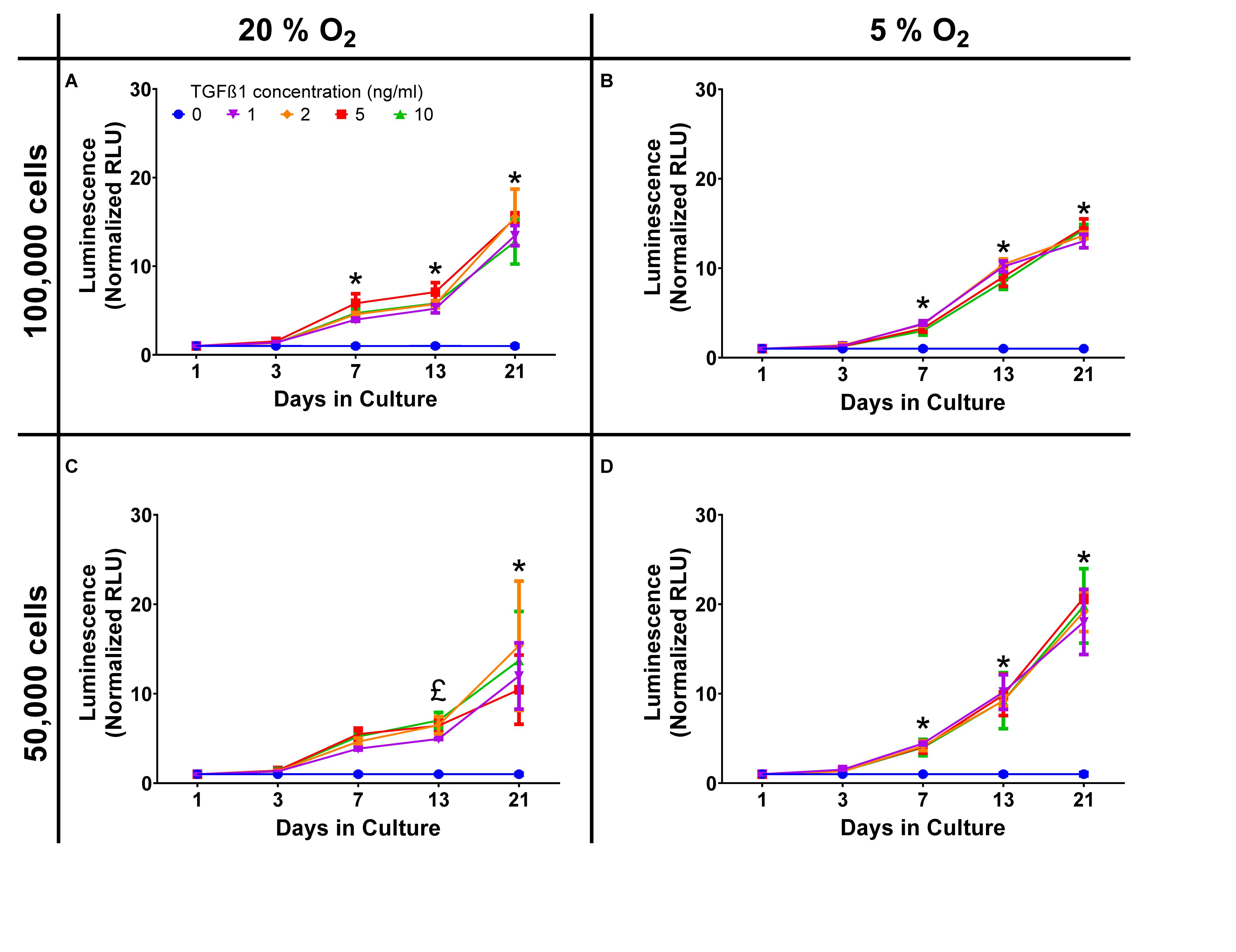
Figure 1. Oxygen and cell density response. Dose response curves, normalized to the control of 0 ng/ml TGFβ1 in chondrogenic media, were constructed and show a time dependent increase in luminescence but with similar responses in terms of both cell number and oxygen tension. (A) 20% O2, 100,000 cells; (B) 5% O2, 100,000 cells; (C) 20% O2, 50,000 cells; (D) 5% O2, 50,000 cells. * = all concentrations significantly greater than basal media, £ = concentrations ≥ 2 ng/ml TGFβ1 significantly greater than basal media (p < 0.05).
where PC, positive control and NC, negative control.
For day 21 the z′ factor for a 50,000 cell pellet was 0.89, which is regarded as very good, validating the assay as having sufficient sensitivity for high-throughput applications. Luminescent decay was assessed over 50 min and the half-life of positive (10 ng/mL) and negative (0 ng/mL) samples were similar; 27.8 and 33.1 min, respectively. As significant signal to noise was achieved using a lower number of cells, the remaining experiments were all conducted using 50,000 cell aggregates in physioxia (5% O2) and luminescence read within 10 min of adding stabilized substrate. Correlation between the expression of COL2A1 promoter-driven Gaussia luciferase and type II collagen expression was established by qPCR with an R2 value of 0.85 (Day 3, Supplementary Data S1).
TGFβ1 Dose Response
The dose response curve of TGFβ1, a known inducer of chondrogenesis, was determined along with the 50% effective concentrations (EC50) calculated as 18, 21, and 22 pg/mL for days 7, 15, and 21, respectively (Figure 2).
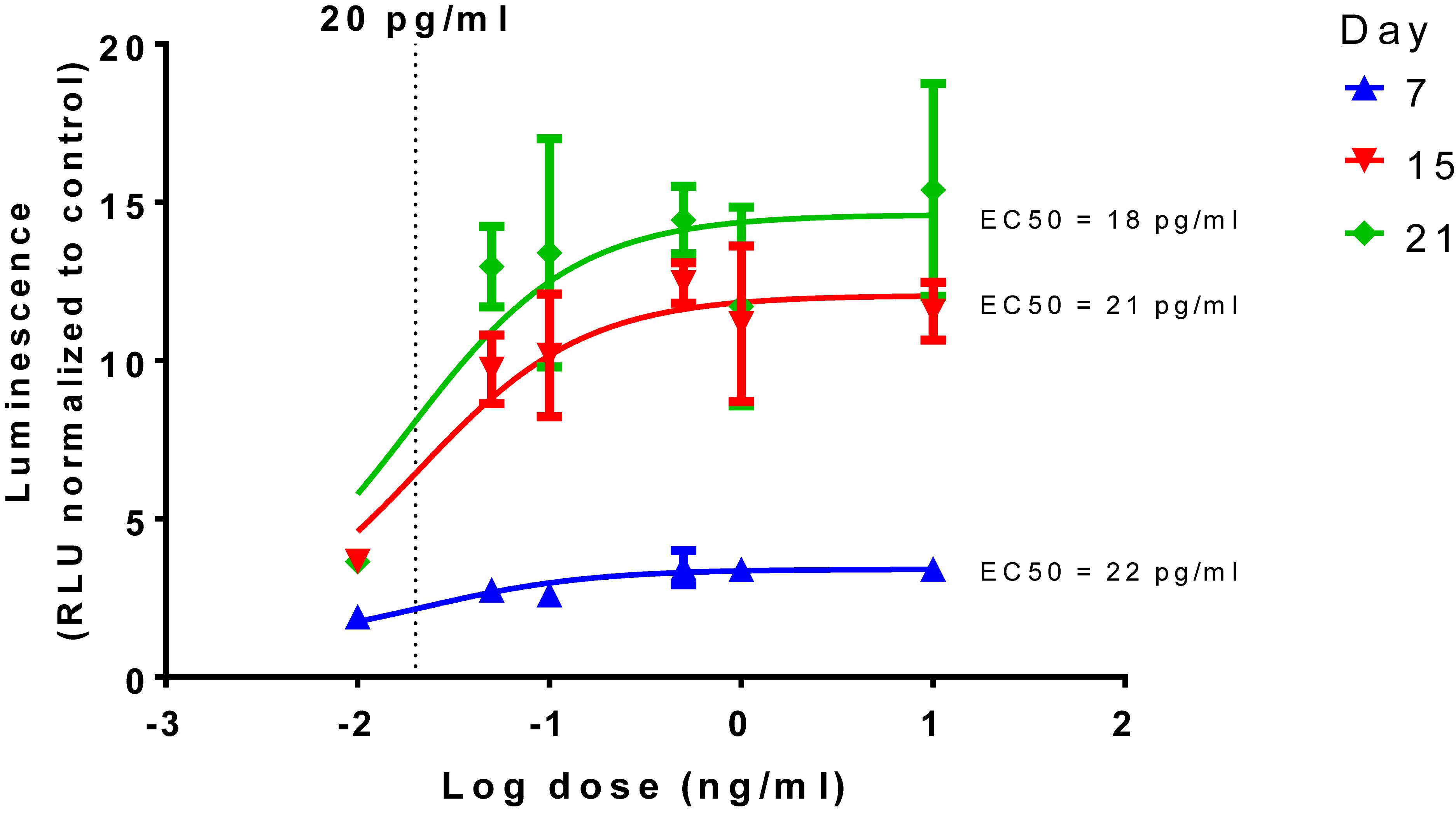
Figure 2. Time and dose dependent effect of TGFβ1 on chondrogenesis. Dose response curves, normalized to the control of 0 ng/mL in chondrogenic media, were constructed and show a time dependent increase in luminescence but with similar EC50s, a dotted line is shown to show 20 pg/mL in the log scale.
Vitamin and Mineral Dose Response in Basal Media
Vitamins and minerals were assessed at varying concentrations based on normal serum concentrations with amounts ranging from 0.01 to 1000% of the maximal serum concentration. Both dose- and time dependent effects on COL2A1 promoter-driven expression were evident (Table 2 and Supplementary Data S2). Typical dose response curves, such as that found for TGFβ1 were not evident; as might be expected for a compound acting in multiple systems as a catalyst/co-factor. All supplements except a-linolenic acid had increased expression at the higher doses at the 2 weeks time point. a-linolenic acid treatment showed increased expression at low doses during the early portion of the experiment which became detrimental at later stages. This decrease at day 21 might indicate an earlier maturation of the tissue. A small, but significant increase in promoter-driven luciferase expression was seen in most cases at one or more doses. A consistent increase over basal expression was seen with chromium (2.8 μg/L; 10% serum max) and vitamin E (1.8–9.2 mg/L; 10–50% serum max) throughout. Vitamin A, widely regarded as detrimental to chondrogenesis, was actually stimulatory to type II collagen promoter-driven expression at the lowest dose tested (78 ng/L; 0.01% serum max) except for the last time point which had returned to basal expression levels.
Vitamin and Mineral Combinatorial Effects
A selection of those factors that had overall positive effects on the relative luminescence of the conditioned media (vitamin B7, copper, iodine, thyroxine, and zinc) were tested at their optimal individual concentration in basal medium and in combination with TGFβ1 (1 ng/mL). These six factors gave 64 conditions; 53 of which were discovered to be significantly different vs. the basal medium, with 46 being increased and 7 being decreased at day 21 (Supplementary Data S3). An abbreviated selection of conditions in the presence of TGFβ1 is shown in Figure 3 (18 of 64); several conditions in combination with TGFβ1 are greater than TGFβ1-supplemented basal medium alone (15 of 31; Figure 3 and Supplementary Data S3). The results showed several interesting temporal patterns.
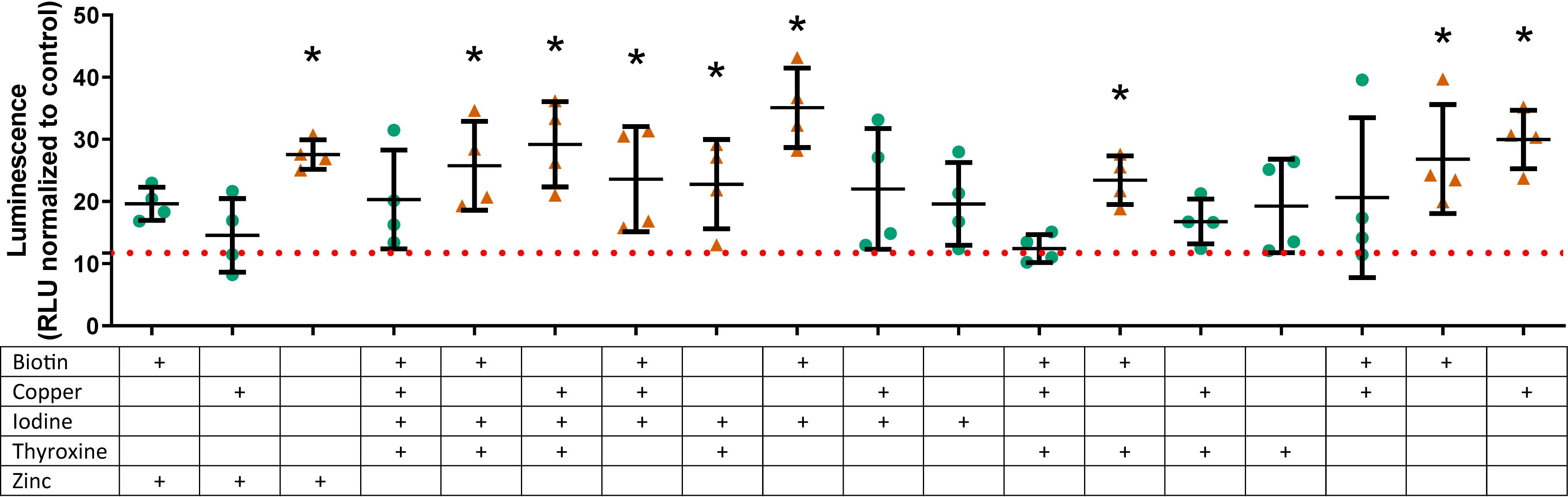
Figure 3. Type II collagen promoter-driven expression with various vitamin and mineral combinations in the presence of TGFβ1 on day 21. Vitamins and minerals (biotin, copper, iodine, thyroxine, and zinc) which had been identified as promoting COL2A1 promoter-driven expression in the initial screen were tested in combination at their individually optimized concentrations (3, 670, 0.92, 20, and 840 μg/L, respectively) in the presence of TGFβ1 at 1 ng/mL. The values are normalized to the plate control (basal media). Green circles () indicate values that are significantly greater than those for basal media alone, and the dashed red line indicates the luminescence achieved with TGFβ1 supplementation at 1 ng/mL. An orange triangle (
) and an * indicate values that were discovered different, with false discovery rate set at 1%.
Due to the prohibitively large number of combinations with the remaining 10 factors (1023 conditions), the three highest performing combinations from the first combination experiments, the results of which are shown in Figure 3, were further supplemented with combinations of vitamins B12, E, K, and chromium. Each of these vitamins and minerals had been determined to effect an increase in relative luminescence over basal media individually. Chromium, vitamins B12, K, and E were supplemented at their individually determined optimal concentrations in basal media (2.8 μg/L, 91.4 ng/L, 1.84 μg/L, and 2.2 μg/L, respectively). The three highest performing combinations from the previous experiment were used for this phase of the study and the TGFβ1 supplemented basal media. These media are termed Sup 1 (basal + TGFβ1); Sup 2 = Sup 1 + iodine + biotin; Sup 3 = Sup 1 + copper; and Sup 4 = Sup 1 + iodine + thyroxine + copper. This resulted in assessment of an additional 60 conditions. In this set of experiments, Sup 3 was higher than Sup 1, as was shown previously in Figure 4, and several combinations were lower than the supplemented media in this and Sup 4 comparisons (Figure 4 and Supplementary Data S4). Although all combinations significantly increased COL2A1 promoter-driven expression over that of basal medium, only vitamin K significantly increased luminescence above TGFβ1- and copper-supplemented basal medium (2.5-fold vs. TGFβ1 alone; Sup 3; Figure 4C and Supplementary Data S4). However, several combinations showed luminescence levels lower than baseline supplemented media (Sup 3 and Sup 4).
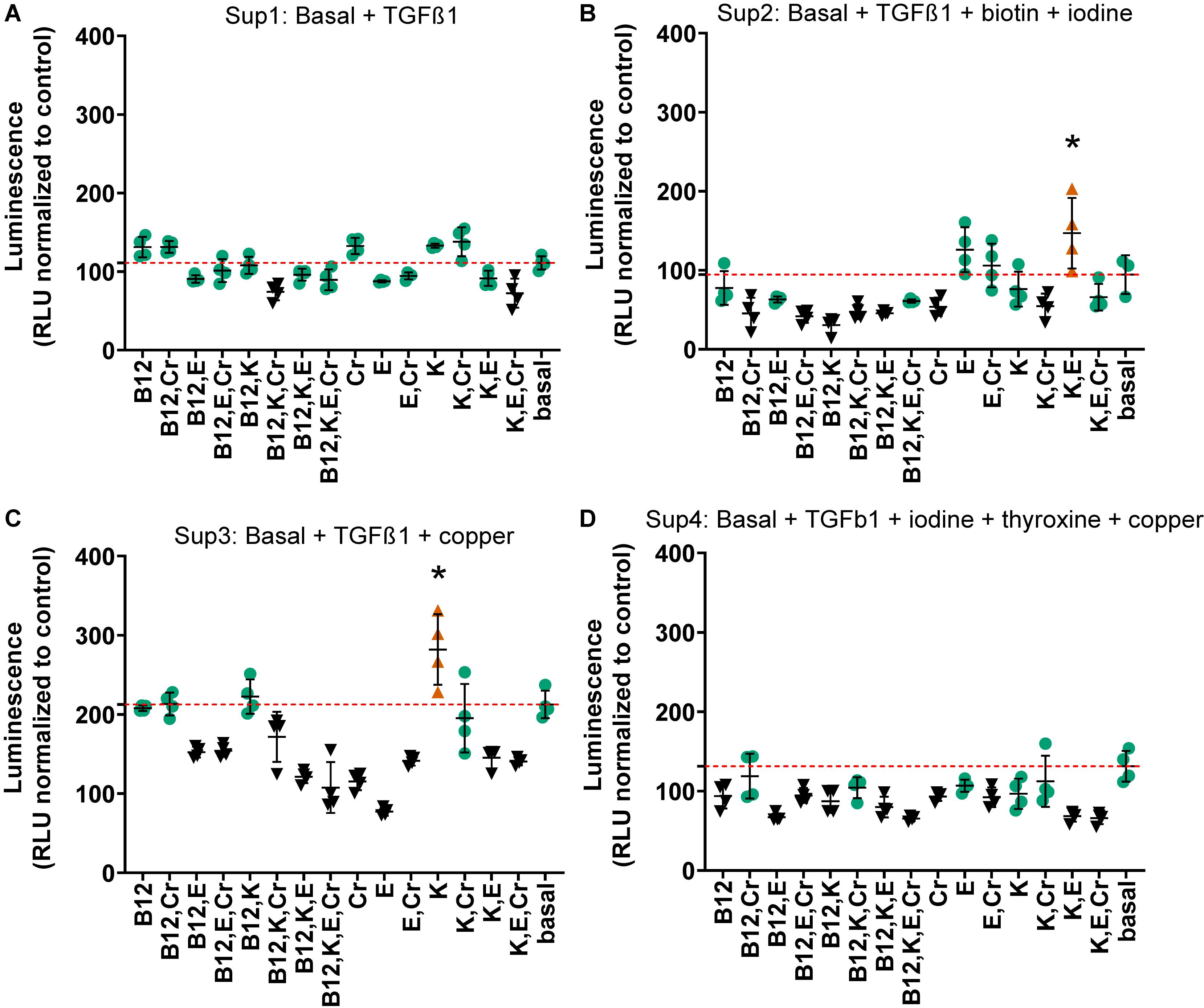
Figure 4. Combinations of chromium, vitamins B12, E, and K in supplemented media at day 21. Vitamins and minerals (chromium, vitamins B12, E, and K) which had been identified as promoting COL2A1 promoter-driven expression in the initial screen were tested alone and in combination in supplemented medias 1 (A) Basal media + TGFβ1; 2 (B) Basal + TGFβ1 + biotin + iodine; 3 (C) Basal + TGFβ1 + copper; 4 (D) Basal + TGFβ1 + iodine + thyroxine + copper. Vitamins and minerals were supplemented at their individually optimized concentration in basal media (2.8 μg/L, 91.4 ng/L, 1.84 μg/L, and 2.2 μg/L, respectively). The values are normalized to the plate control (basal media); the red dashed red line indicates the luminescence achieved with that particular supplemented media alone. Green circles indicate values that are not significantly different to supplemented media (red dashed line), all are greater than basal media. Conditions that were statistically greater than their respective supplemented media are represented by an orange triangle () and an *, black symbols (▼) indicate that those values were lower than the respective supplemented media. n ≥ 3 ± S.D.
Dose Response to Vitamin and Mineral Supplementation in the Presence of TGFβ1
Given the complex responses seen with combinations in the presence of TGFβ1, vitamin and mineral dose responses (0.01–500% serum max) were further tested in the presence of TGFβ1 (1 ng/mL). Both time and dose responses were evident (Table 3, Figure 5 and Supplementary Data S5). Conditioned medium was collected on days 3, 7, 15, and 21 and assayed for Gaussia luciferase expression to evaluate the temporal effects of different additives. The results were expressed as the fold change relative to time-matched basal medium controls (Table 3).
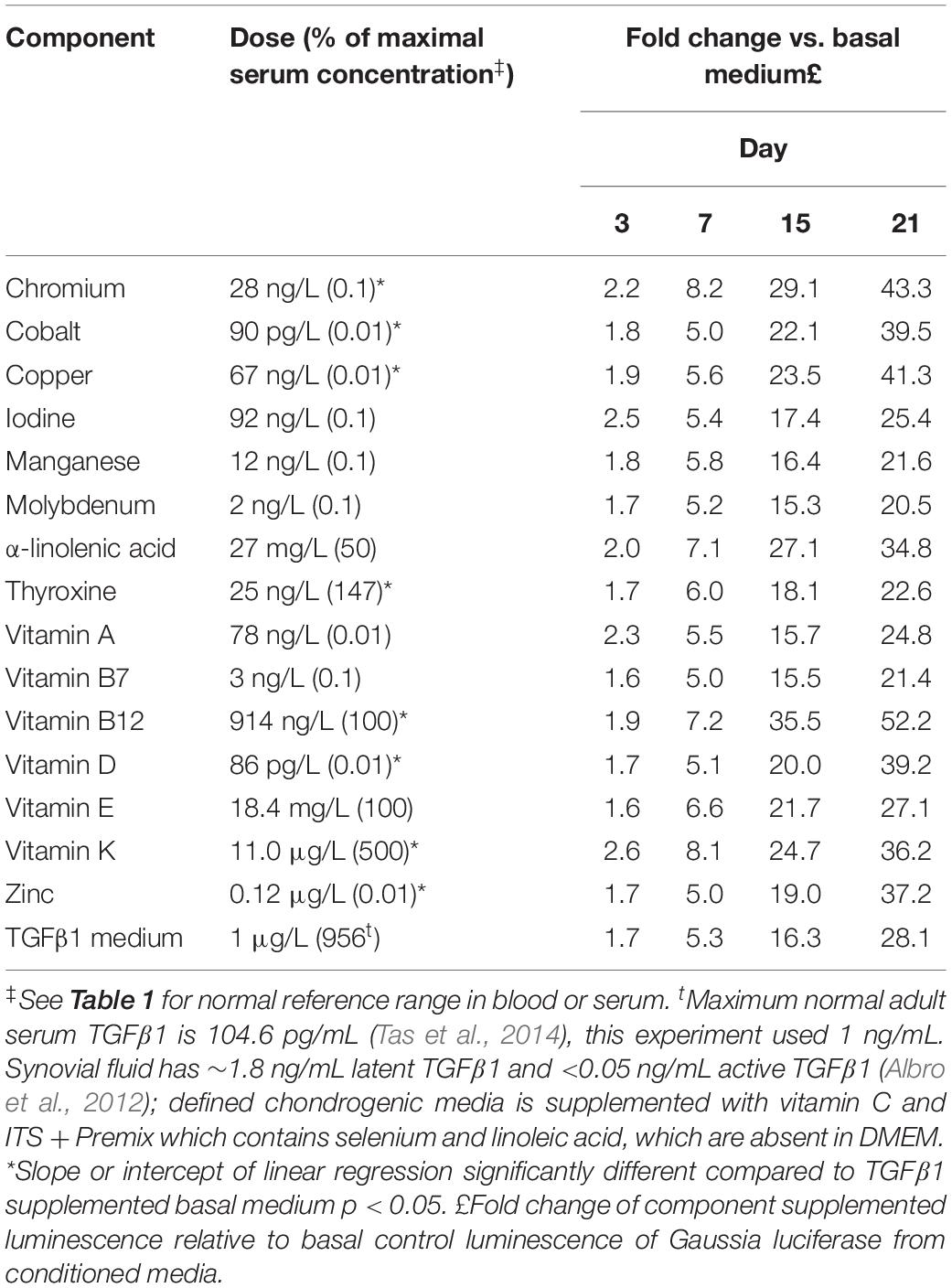
Table 3. Dose and temporal effect of vitamins and minerals in the presence of TGFβ1 on chondrogenesis.
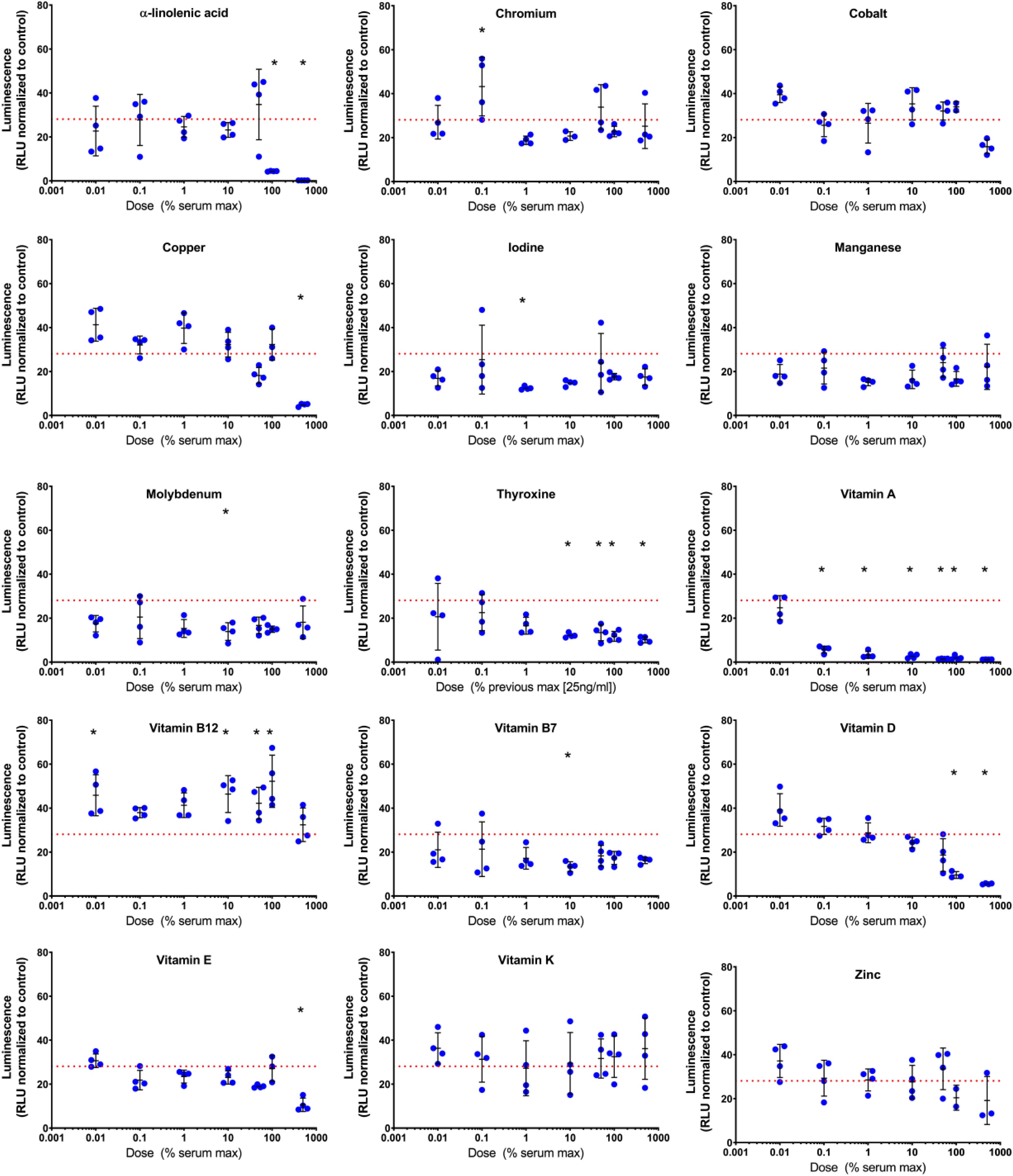
Figure 5. Day 21 evaluation of COL2A1 promoter-driven expression in vitamin and mineral supplemented TGFβ1 supplemented basal medium. Conditioned media was sampled at day 21 and assessed for luminescence, and normalized against the plate control basal medium. The red dashed line indicates the luminescence of the TGFβ1 supplemented basal medium. The symbol “*” significantly different vs. TGFβ1 supplemented basal medium, two-way ANOVA p < 0.05 Sidak’s multiple comparison test. Blue circles represent each data point.
Summation of the fold change across the whole experiment to give area under the curve analysis identified 40 conditions that were greater than the TGFβ1 supplemented medium. The top 10 conditions that gave greater expression over the whole experiment are shown in Table 4 with an example plot in Figure 6 (the remaining comparisons are shown in Supplementary Data S7).
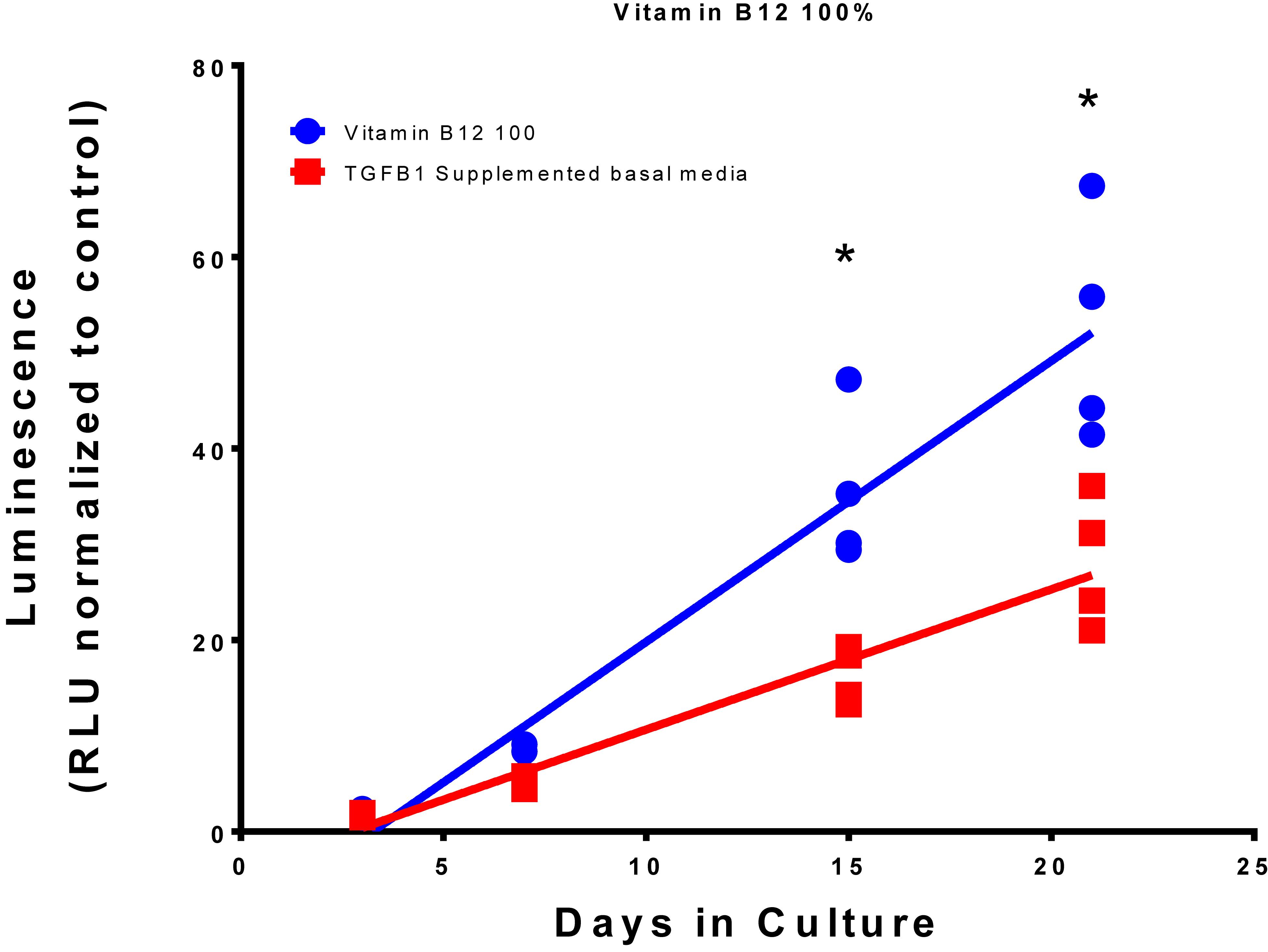
Figure 6. Comparison of Vitamin B12 and TGFβ1 supplemented medium with TGFβ1 supplemented basal medium. Type II collagen promoter-driven luminescence was assessed from the conditioned media and normalized to the plate basal medium control. Lines indicate a linear regression; symbols indicate individual data points. The symbol “*” significant difference vs. TGFβ1 supplemented basal medium; two-way ANOVA p < 0.05 Sidak’s multiple comparison test.
To assess the effects of supplementation on metabolic activity, resazurin assays were performed at day 21. At day 21, increased metabolic activity was detected at low doses of cobalt, copper, vitamin D, vitamin E, and zinc (Figure 7 and Supplementary Data S6). Decreased metabolic activity was seen at high doses of copper and vitamin D. Vitamin A was predominantly inhibitory and vitamin B12 predominantly stimulatory. When type II collagen promoter-driven luciferase expression was normalized against resazurin metabolism, none of the components tested showed increased expression at day 21 compared to TGFβ1 supplemented control except iodine at 92 μg/L. Indicating that, at this time point, the effect on type II collagen promoter-driven luciferase was predominantly or exclusively linked to metabolic activity (Supplementary Data S10).
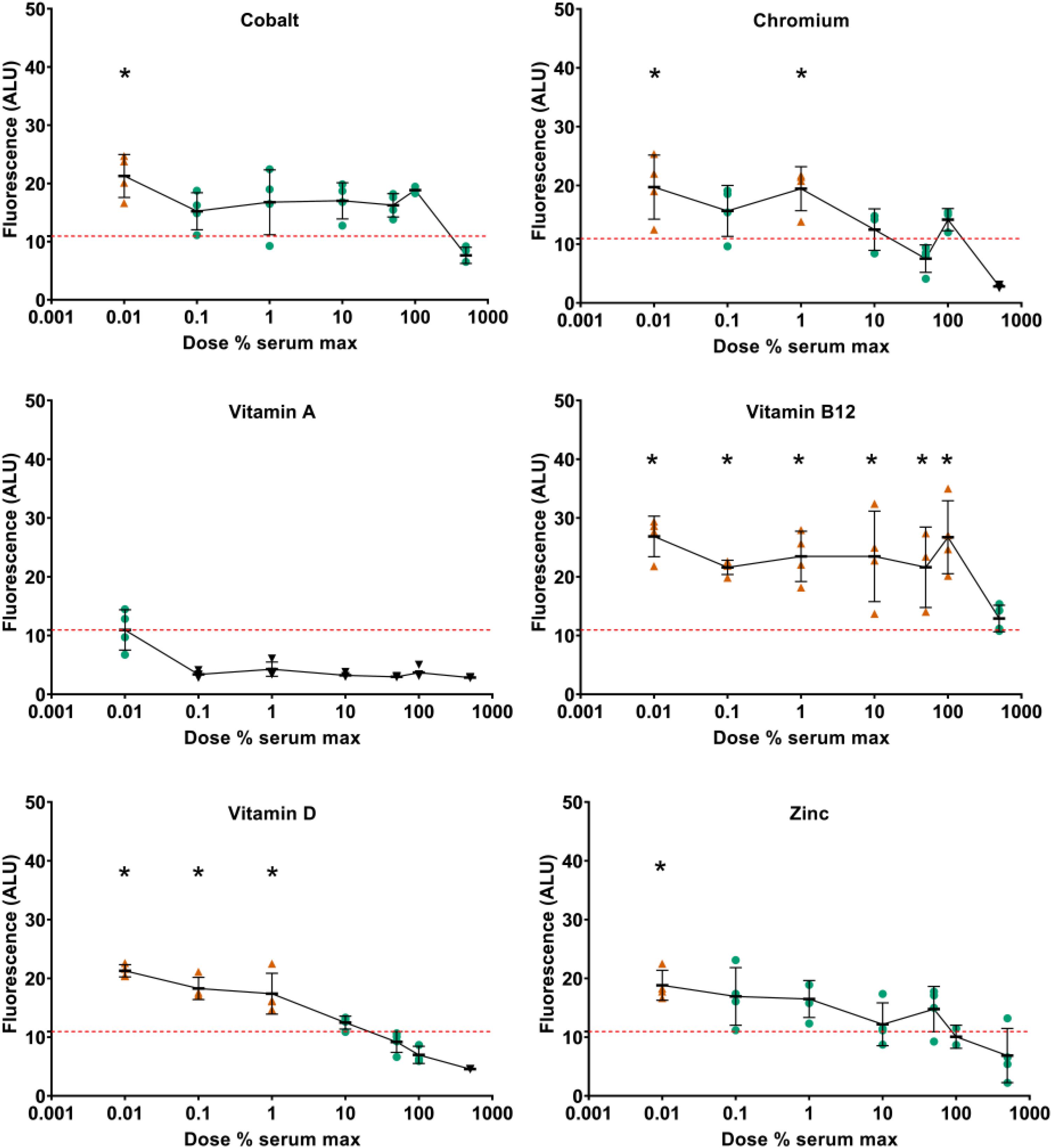
Figure 7. Effect of vitamin and mineral addition to TGFβ1 supplemented medium on metabolic activity. Cells were treated with resazurin, media was sampled at day 21 and assessed for fluorescence. All data were normalized against the conditioned media from pellets treated with basal chondrogenic media. The red dashed line indicates the fluorescence of the TGFβ1 supplemented basal medium. An orange triangle () and an * indicate greater than TGFβ1 supplemented basal medium; black triangles (▼) indicate lower vs. TGFβ1 supplemented basal medium, one-way ANOVA p < 0.05 Dunnett’s multiple comparison test. Conditions that were similar to TGFβ1 supplemented basal media are represented by a green circle (
).
Materials and Methods
ATDC5 Cell Culture and Transformation
ATDC5 cells (a kind gift of Dr. Florent Elefteriou, Baylor College of Medicine) were grown in DMEM-LG (HyClone) containing 5% FBS (Atlanta Biologicals) and 1% penicillin/streptomycin (Gibco) at 37°C in a humidified atmosphere with 20% O2, 5% CO2. Cells were transformed with pseudolentiviral particles during log expansion phase at approximately 30% confluence. Concentrated lentivirus was diluted with growth medium and mixed 1:1 with polybrene (8 μg/mL; EMD Millipore) in Opti-MEM (Gibco), then applied to the cells. This concentration of polybrene was determined to have minimal effects on proliferation and significantly improved infection. Plates were incubated at 4°C for 10 min before transfer to 37°C (humidified atmosphere with 20% O2, 5% CO2) for 12 h. Media were then exchanged for growth medium and cells were grown to ∼90% confluence. Cells were then trypsinized and re-plated at 6000 cells/cm2. After allowing cells to adhere for 24 h, infected cells were selected by incubation in growth medium containing puromycin (2 μg/mL; Alfa Aesar) for 1 week. This concentration was determined to be the lowest concentration with >95% uninfected cell death on human mesenchymal stromal cells and chondrocytes (data not shown). Puromycin-selected cells were then grown in normal growth medium for no more than four passages before use in differentiation experiments.
Lentiviral Construct
The collagen type II promoter (COL2A1)-driven, secreted Gaussia luciferase was custom ordered (HIV-based lentiviral third generation; HPRM22364-LvPG02, GeneCopoeia, Inc.). The promoter length is 1426 bp. This plasmid (col2gLuc; HPRM22364-LvPG02), the eGFP control (EX-EGFP-Lv105, GeneCopoeia, Inc.) the packaging plasmid (psPAX2) and the envelope plasmid (pMD2.G) were amplified in Escherichia coli (GCI-L3, GeneCopoeia, Inc.), and purified with a silica column (Qiagen Maxiprep). Plasmids (Supplementary Data S9) were confirmed by restriction digest and agarose gel electrophoresis. Pseudolentiviral particles were made in HEK-293Ta cells (GeneCopoeia, Inc.) by co-transfection of psPAX2, pMD2.G, and HPRM22364-LvPG02 using calcium phosphate precipitation (Dull et al., 1998). Pseudolentiviral particles were harvested from conditioned medium at 48 h and concentrated by centrifugation (10,000 RCF, 4°C, overnight). Infectious particle concentration was estimated by serial dilution of pseudolentiviral particles and flow cytometry assessment of eGFP positive cells produced in the same batch (Cellecta, 2018). The concentration of lentiviral particles used in these experiments was the most dilute concentration that resulted in >95% eGFP positive cells. Lentiviral particle concentration was further determined by qPCR assessment of lentiviral RNA according to the manufacturer’s instructions (Lenti-Pac Titration Kit; LT005, GeneCopoeia, Inc.).
qPCR Correlation of COL2A1 Promoter-Driven Gaussia Luciferase With Type II Collagen Expression
Cells were lysed and RNA purified with guanidine based extraction RNA according to the manufacturer directions (Direct-zol RNA Miniprep, Zymo Research). To create cDNA, 750 ng of purified RNA was reverse transcribed according to the manufacturer directions (SuperScriptTM III Platinum, Invitrogen) using oligoDT primers. Quantification of gene expression was performed by qPCR using SYBR green (Power SYBR, Invitrogen) detection with the following primers: Gaussia luciferase F: 5′–ACG CTG CCA CAC CTA CGA–3′, R: 5′–CCT TGA ACC CAG GAA TCT CAG GAA–3′; murine type II collagen F: 5′–CCA ATG ATG TAG AGA TGA GGG C–3′, R: 5′–TGT GTT GTT TCA GGG TTC GGG–3′. Gaussia luciferase primer efficiency was calculated from a cDNA dilution curve at 100.4%, with an amplicon length of 92 bp and a melt temp of 81.5°C (CFX96 real time, Bio-Rad Laboratories, Inc.), no cross-reactivity was found with the mouse genome [Primer-Blast (Ye et al., 2012)]. Murine Col2a1 primers amplify both variants [NM 031163.3 (variant 1) and NM 001113515.2 (variant 2)]; primer efficiency was calculated from a cDNA dilution curve at 93.6%, with an amplicon length of 243 bp and a melt temp of 81.5°C (CFX96 real time, Bio-Rad Laboratories, Inc.).
Chondrogenic Differentiation
Cells were differentiated in pellet culture at 37°C in a humidified atmosphere with 5% O2, 5% CO2 (50,000–250,000 cells/pellet) in polypropylene 96-well plates (Phenix) in defined basal chondrogenic medium: DMEM-HG supplemented with 1% insulin, transferrin, selenium + premix; 130 mM ascorbate-2-phosphate, 2 mM GlutaMax, 1% sodium pyruvate, 1% MEM non-essential amino acids, 100 nM dexamethasone, 1.25 μg/mL fungizone, and 1% penicillin/streptomycin. This medium was further supplemented with vitamins and minerals (Table 5), thyroxine (Whitney et al., 2018) and recombinant human TGFβ1 (PeproTech). Medium sampling, mixing and exchange were conducted with a Tecan Freedom Evo fitted with a 96-head multi-channel aspirator (MCA) and 4-channel dispensing tips (DiTi). The pipetting profile for the MCA was set to aspirate at 10 μL/s and dispense at 500 μL/s. The pipetting profile for the DiTi was set to aspirate at 20, 100, and 150 μL/s for <15, 15–200, and 200–1000 μL respectively and to dispense at 600 μL/s for all volumes.
Luciferase Assessment
Conditioned cell culture medium was sampled from plates (20 μl/well; MCA) at feeding and pipetted into a white 96-well plate (Greiner Bio-One) and assessed for luminescence using the stabilized Gaussia Luciferase Kit (New England Biolabs, Inc.; 50 μl of a 1:1 dilution with 18 MΩ water) and read in a plate reader (Tecan M200 PRO; 5 s 1 mm orbital mix, 45 s wait, then read 2 s/well, 20°C, no attenuation). All data were normalized against the conditioned media from pellets treated with basal chondrogenic media. Basal conditioned medium from cells was supplemented with vitamins and minerals to determine if there was any effect on the luciferase assay, none was seen (Supplementary Data S8).
Metabolic Assay
Metabolic activity was assessed on day 21 using the resazurin assay (O’Brien et al., 2000; Schreiber-Brynzak et al., 2015). Resazurin (Sigma) was dissolved in phosphate buffered saline to give a 10× stock solution (500 μM), sterile filtered (0.2 μm) and aliquots made and frozen (−80°C). Frozen aliquots were thawed and diluted in phosphate buffered saline to 50 μM, 20 μL of this solution was then added to each well and incubated at 37°C for 6 h. Fluorescence was read (Tecan Infinite® 200 PRO) with excitation at 530 nm and emission at 560 nm.
Statistics
Statistics were performed using GraphPad Prism (GraphPad Software, Inc.). Comparisons of dose response over time were made using a two-way ANOVA with Sidak’s multiple comparison post hoc test. Comparisons between supplemented media (Sup 1–4) and additives were made using a two-way ANOVA with Sidak’s multiple comparison post hoc test. Comparisons between basal or TGFβ1 supplemented media with combinations were made using multiple t-tests with a false discovery rate method of Benjamini and Hochberg set at 1%. Comparisons of the metabolic dose response due to supplementation with vitamins and minerals were made using ANOVA with Dunnett’s multiple comparison post hoc test.
Discussion
Tissue engineering of cartilage has the potential to ameliorate disability due to arthritis by (a) providing a better in vitro model for drug discovery and (b) providing a biological replacement eliminating the need for total joint arthroplasty. Without a permissive environment, i.e., one that contains all the vitamins and minerals that have been deemed essential, reflecting in vivo conditions, drug discovery and tissue engineering efforts are potentially hampered by inappropriate responses. This is exemplified in this study through the synergistic effect of vitamins, minerals, and TGFβ1 on COL2A1 promoter activity (Figure 3). It is perhaps unsurprising that vitamins and minerals which are known to be essential for proper development have significant effects on COL2A1 promoter-driven expression in this in vitro system. It is therefore surprising that they are omitted from common basal media; this work establishes that, while not all components promote COL2A1 promoter-driven expression, they can still have an effect on chondrogenesis, such as through the stimulation or inhibition of metabolism. These complex temporal effects are to be expected given the broad range of enzymes, signaling pathways, and physiological processes that require these vitamins and minerals for their activity.
The goal of the present study was to streamline the methodology for optimizing COL2A1 promoter-driven expression and, at the same time, to use the examination of vitamins and minerals missing from conventional defined media both as a proof of concept and as a much needed inquiry. To accomplish that goal, an aggregate chondrogenesis assay (Yoo et al., 1998) that had been modified for a 96-well format (Penick et al., 2005) was applied as a means to use a high-throughput cartilage tissue-based assay to screen chondrocytes transduced with a Gaussia luciferase reporter driven by a type II collagen promoter. This has resulted in significant insights into the role of vitamins and minerals on chondrocyte metabolism. There is a clear interaction between TGFβ1 and some vitamins and minerals (copper, vitamin A). However, even at our reduced TGFβ1 concentration of 1 ng/mL, one-tenth of that commonly used but still ∼500-times the EC50 and ∼10-times normal serum concentration, we may have masked some potential stimulators.
To date, only a few attempts at developing high-throughput assays for chondrogenesis have been reported. Greco et al. (2011) developed what they termed a “medium-throughput” assay that used the human C-28/12 chondrocyte cell line where cells were seeded as 1.0 × 104 micromasses in 24-well plates; terminal assays were used to determine gene expression and GAG content. Another high-throughput assay, using only 1.0 × 104 cells per well, was used to screen for factors influencing mesenchymal stem cell chondrogenesis (Huang et al., 2008) where, again, terminal assays were used to assess gene expression and GAG content. A similar aggregate assay was also described for adipose-derived stem cells where 2.0–5.0 × 105 cells per well of a 96-well plates were cultured for several weeks and then assayed for GAG content as a terminal assay (Abu-Hakmeh and Wan, 2014). Another study used ATDC5 cells in a high-throughput assay to test a library of factors for their effect on total collagen production (Le et al., 2015). In their study, ATDC5 cells were plated at 5.0 × 103 cells per well and allowed to expand for 3 days and, after 6 days, were assayed for collagen content using a fluorescent collagen-binding probe (CN35-AF488) to measure collagen content. In all of these cases, the assays were conducted as terminal assays, that is, at the end of the incubation period. One of the strengths of the methodology shown here is that the assays are conducted on conditioned medium, allowing for a complete temporal assessment of the different factors, and combinations of factors, over time. Temporal chondrogenesis and osteogenesis have been studied by others with Correa et al. (2018) investigating Sox9, aggrecan, and osteocalcin in mesenchymal stem cells.
While the addition of components to media formulations makes their composition more complex, we would argue that, in order to model the effect of any in vivo manipulation, a permissive environment, i.e., one that contains factors essential for normal cell metabolism is necessary. Given that: (1) cells are relatively efficient in recycling many of their components, (2) in vitro systems are an imperfect model, and (3) the conditions that exist within a developing joint are relatively unknown, our current suggested “optimal” medium for chondrogenesis is: DMEM-HG supplemented with 1% insulin, transferrin, selenium + premix; 130 mM ascorbate-2-phosphate, 2 mM GlutaMax, 1% sodium pyruvate, 1% MEM non-essential amino acids, 100 nM dexamethasone, 1.25 μg/mL fungizone, 1% penicillin/streptomycin, 1 ng/mL TGFβ1, 670 ng/mL copper, 2.2 ng/mL vitamin K (highest yield, Figure 4). However, based on the apparent shift around day 15 both in this work and that shown by Huynh et al. (2018) there is potential for a media switch around this time. In addition, we consider that a permissive medium solution would contain other vitamins and minerals at 1/100th–1/1000th of their serum max, potentially allowing somewhat of a “normal” response to other manipulations such as mechanical stimulation (Nazempour et al., 2016). The proposed supplements to chondrogenic medium are shown in Table 6 for the two time periods 0–15 and 16–21 days based on their ability to consistently upregulate type II collagen promoter-driven Gaussia luciferase expression without compromising metabolic activity. Select vitamins and minerals were removed from the latter stage due to their correlation with decreased COL2A1 promoter-driven expression after 15 days; this may be because the tissue is more mature, and they are recycled more efficiently and/or used less. The fat soluble vitamins A, D, E, K, and a-linolenic acid should be combined with ITS + premix as a stock solution as they will bind to the bovine serum albumin in that solution. These suggested media need further investigation in this and other systems, current work is focusing on the application of this system to primary chondrocytes.
Conclusion
A high-throughput amenable, temporal surrogate chondrogenesis assay has been developed in a model chondrocyte cell line, ATDC5. Medium supplementation with vitamins and minerals resulted in changes in COL2A1 promoter-driven expression and metabolic activity dependent on both time and concentration.
Summary
Current defined chondrogenic culture media lack several vitamins and minerals. Type II collagen is the quintessential marker of articular hyaline cartilage, and is commonly deficient in engineered tissue. A type II collagen promoter-driven secreted luciferase construct has been transduced into ATDC5 cells and used to assess vitamin and mineral effects on chondrogenesis in a high-throughput format.
Data Availability Statement
All datasets generated for this study are included in the article/Supplementary Material.
Author Contributions
TK: concept and manuscript writing. TK and JD: design, data analysis and interpretation, financial support, and manuscript editing. TK and TS: collection of the data. TK, JD, and TS: final approval of the manuscript.
Funding
Funding for the study was made through Baylor College of Medicine and the Bone Disease Program of Texas. Publication costs are covered by the College of Medicine, University of Central Florida.
Conflict of Interest
The authors declare that the research was conducted in the absence of any commercial or financial relationships that could be construed as a potential conflict of interest.
Acknowledgments
We are extremely grateful to Dr. Ken Scott (1974–2017) for his permitting us the use of his Tecan Evo Freedom, without which we could not have pursued this research. We would also like to acknowledge Dr. D. MacKay for proofreading and funding from the Baylor College of Medicine (JD) and the Bone Disease Program of Texas (TK). This manuscript has been released as a Pre-Print (Dennis et al., 2019). The plasmids, pMD2.G, and psPAX2, were a gift from Didier Trono (Addgene plasmid # 12259; http://n2t.net/addgene:12259; RRID:Addgene_12259) and (Addgene plasmid # 12260; http://n2t.net/addgene:12260; RRID:Addgene_12260) respectively.
Supplementary Material
The Supplementary Material for this article can be found online at: https://www.frontiersin.org/articles/10.3389/fcell.2020.00092/full#supplementary-material
References
Abu-Hakmeh, A. E., and Wan, L. Q. (2014). “High-throughput cell aggregate culture for stem cell chondrogenesis,” in Biomimetics and Stem Cells: Methods and Protocols, eds G. Vunjak-Novakovic, and K. Turksen, (New York, NY: Springer), 11–19. doi: 10.1007/7651_2014_75
Ahrens, P. B., Solursh, M., and Reiter, R. S. (1977). Stage-related capacity for limb chondrogenesis in cell culture. Dev. Biol. 60, 69–82. doi: 10.1016/0012-1606(77)90110-5
Albro, M. B., Cigan, A. D., Nims, R. J., Yeroushalmi, K. J., Oungoulian, S. R., Hung, C. T., et al. (2012). Shearing of synovial fluid activates latent TGF-β. Osteoarthr. Cartil. 20, 1374–1382. doi: 10.1016/j.joca.2012.07.006
Ballock, R. T., and Reddi, A. H. (1994). Thyroxine is the serum factor that regulates morphogenesis of columnar cartilage from isolated chondrocytes in chemically defined medium. J. Cell Biol. 126, 1311–1318. doi: 10.1083/jcb.126.5.1311
Barone, L. M., Owen, T. A., Tassinari, M. S., Bortell, R., Stein, G. S., and Lian, J. B. (1991). Developmental expression and hormonal regulation of the rat matrix Gla protein (MGP) gene in chondrogenesis and osteogenesis. J. Cell. Biochem. 46, 351–365. doi: 10.1002/jcb.240460410
Booth, S. L. (1997). Skeletal functions of vitamin K-dependent proteins: not just for clotting anymore. Nutr. Rev. 55, 282–284. doi: 10.1111/j.1753-4887.1997.tb01619.x
Centers for disease control and prevention (2009). Prevalence and most common causes of disability among adults–United States, 2005. MMWR Morb. Mortal. Wkly. Rep. 58, 421–426.
Correa, D., Somoza, R. A., and Caplan, A. I. (2018). Nondestructive/Noninvasive imaging evaluation of cellular differentiation progression during in vitro mesenchymal stem cell-derived chondrogenesis. Tissue Eng. Part A 24, 662–671. doi: 10.1089/ten.TEA.2017.0125
Dennis, J. E., Bernardi, K. G., Kean, T. J., Liou, N. E., and Meyer, T. K. (2018). Tissue engineering of a composite trachea construct using autologous rabbit chondrocytes. J. Tissue Eng. Regen. Med. 12, e1383–e1391. doi: 10.1002/term.2523
Dennis, J. E., Splawn, T., and Kean, T. J. (2019). High-throughput, temporal and dose dependent, effect of vitamins and minerals on chondrogenesis. bioRxiv [Preptint] bioRxiv:811877.
Dull, T., Zufferey, R., Kelly, M., Mandel, R. J., Nguyen, M., Trono, D., et al. (1998). A third-generation lentivirus vector with a conditional packaging system. J. Virol. 72, 8463–8471. doi: 10.1128/jvi.72.11.8463-8471.1998
Finch, C. W. (2015). Review of trace mineral requirements for preterm infants: what are the current recommendations for clinical practice? Nutr. Clin. Pract. 30, 44–58. doi: 10.1177/0884533614563353
Gemmiti, C. V., and Guldberg, R. E. (2006). Fluid flow increases type II collagen deposition and tensile mechanical properties in bioreactor-grown tissue-engineered cartilage. Tissue Eng. 12, 469–479. doi: 10.1089/ten.2006.12.469
Greco, K. V., Iqbal, A. J., Rattazzi, L., Nalesso, G., Moradi-Bidhendi, N., Moore, A. R., et al. (2011). High density micromass cultures of a human chondrocyte cell line: a reliable assay system to reveal the modulatory functions of pharmacological agents. Biochem. Pharmacol. 82, 1919–1929. doi: 10.1016/j.bcp.2011.09.009
Han, F., Adams, C. S., Tao, Z., Williams, C. J., Zaka, R., Tuan, R. S., et al. (2005). Transforming growth factor-beta1 (TGF-beta1) regulates ATDC5 chondrogenic differentiation and fibronectin isoform expression. J. Cell. Biochem. 95, 750–762. doi: 10.1002/jcb.20427
Helmick, C. G., Felson, D. T., Lawrence, R. C., Gabriel, S., Hirsch, R., Kwoh, C. K., et al. (2008). Estimates of the prevalence of arthritis and other rheumatic conditions in the United States. Part I. Arthritis Rheum. 58, 15–25. doi: 10.1002/art.23177
Henderson, J. H., Ginley, N. M., Caplan, A. I., Niyibizi, C., and Dennis, J. E. (2010). Low oxygen tension during incubation periods of chondrocyte expansion is sufficient to enhance postexpansion chondrogenesis. Tissue Eng. Part A 16, 1585–1593. doi: 10.1089/ten.TEA.2009.0411
Henderson, J. H., Welter, J. F., Mansour, J. M., Niyibizi, C., Caplan, A. I., and Dennis, J. E. (2007). Cartilage tissue engineering for laryngotracheal reconstruction: comparison of chondrocytes from three anatomic locations in the rabbit. Tissue Eng. 13, 843–853. doi: 10.1089/ten.2006.0256
Huang, A. H., Motlekar, N. A., Stein, A., Diamond, S. L., Shore, E. M., and Mauck, R. L. (2008). High-throughput screening for modulators of mesenchymal stem cell chondrogenesis. Ann. Biomed. Eng. 36, 1909–1921. doi: 10.1007/s10439-008-9562-4
Huynh, N. P. T., Zhang, B., and Guilak, F. (2018). High-depth transcriptomic profiling reveals the temporal gene signature of human mesenchymal stem cells during chondrogenesis. FASEB J. 33, 358–372. doi: 10.1096/fj.201800534R
Johnstone, B., Hering, T. M., Caplan, A. I., Goldberg, V. M., and Yoo, J. U. (1998). In vitro chondrogenesis of bone marrow-derived mesenchymal progenitor cells. Exp Cell Res. 238, 265–272.
Kean, T. J., and Dennis, J. E. (2015). Synoviocyte derived-extracellular matrix enhances human articular chondrocyte proliferation and maintains re-differentiation capacity at both low and atmospheric oxygen tensions. PLoS One 10:e0129961. doi: 10.1371/journal.pone.0129961
Kean, T. J., Mera, H., Whitney, G. A., MacKay, D. L., Awadallah, A., Fernandes, R. J., et al. (2016). Disparate response of articular- and auricular-derived chondrocytes to oxygen tension. Connect. Tissue Res. 57, 319–333. doi: 10.1080/03008207.2016.1182996
Koyano, Y., Hejna, M., Flechtenmacher, J., Schmid, T. M., Thonar, E. J., and Mollenhauer, J. (1996). Collagen and proteoglycan production by bovine fetal and adult chondrocytes under low levels of calcium and zinc ions. Connect. Tissue Res 34, 213–225. doi: 10.3109/03008209609000700
Le, B. Q., Fernandes, H., Bouten, C. V., Karperien, M., van Blitterswijk, C., and de Boer, J. (2015). High-throughput screening assay for the identification of compounds enhancing collagenous extracellular Matrix Production by ATDC5 cells. Tissue Eng. Part C Methods 21, 726–736. doi: 10.1089/ten.TEC.2014.0088
Lind, T., Sundqvist, A., Hu, L., Pejler, G., Andersson, G., Jacobson, A., et al. (2013). Vitamin a is a negative regulator of osteoblast mineralization. PLoS One 8:e82388. doi: 10.1371/journal.pone.0082388
Litchfield, T. M., Ishikawa, Y., Wu, L. N., Wuthier, R. E., and Sauer, G. R. (1998). Effect of metal ions on calcifying growth plate cartilage chondrocytes. Calcif. Tissue Int. 62, 341–349. doi: 10.1007/s002239900442
Mahmoudifar, N., and Doran, P. M. (2010). Chondrogenic differentiation of human adipose-derived stem cells in polyglycolic acid mesh scaffolds under dynamic culture conditions. Biomaterials 31, 3858–3867. doi: 10.1016/j.biomaterials.2010.01.090
Makris, E. A., Hu, J. C., and Athanasiou, K. A. (2013a). Hypoxia-induced collagen crosslinking as a mechanism for enhancing mechanical properties of engineered articular cartilage. Osteoarthritis Cartilage 21, 634–641. doi: 10.1016/j.joca.2013.01.007
Makris, E. A., MacBarb, R. F., Responte, D. J., Hu, J. C., and Athanasiou, K. A. (2013b). A copper sulfate and hydroxylysine treatment regimen for enhancing collagen cross-linking and biomechanical properties in engineered neocartilage. FASEB J. 27, 2421–2430. doi: 10.1096/fj.12-224030
Masuda, E., Shirai, K., Maekubo, K., and Hirai, Y. (2015). A newly established culture method highlights regulatory roles of retinoic acid on morphogenesis and calcification of mammalian limb cartilage. Biotechniques 58, 318–324. doi: 10.2144/000114300
MayoClinic (2016). Mayo Medical Laboratories Rochester Test Catalog. Available at: https://www.mayocliniclabs.com/test-catalog/index.html (accessed May 20, 2016).
Mello, M. A., and Tuan, R. S. (2006). Effects of TGF-beta1 and triiodothyronine on cartilage maturation: in vitro analysis using long-term high-density micromass cultures of chick embryonic limb mesenchymal cells. J. Orthop. Res. 24, 2095–2105. doi: 10.1002/jor.20233
Mobasheri, A., Platt, N., Thorpe, C., and Shakibaei, M. (2006). Regulation of 2-deoxy-D-glucose transport, lactate metabolism, and MMP-2 secretion by the hypoxia mimetic cobalt chloride in articular chondrocytes. Ann. N. Y. Acad. Sci. 1091, 83–93. doi: 10.1196/annals.1378.057
Mounts, T., Ginley, N., Schluchter, M., and Dennis, J. E. (2012). Optimization of the expansion and differentiation of Rabbit Chondrocytes in vitro. Cartilage 3, 181–187. doi: 10.1177/1947603511420999
Nazempour, A., Quisenberry, C. R., Van Wie, B. J., and Abu-Lail, N. I. (2016). Nanomechanics of engineered articular cartilage: synergistic influences of transforming growth factor-beta3 and oscillating pressure. J. Nanosci. Nanotechnol. 16, 3136–3145. doi: 10.1166/jnn.2016.12564
Niedermeier, W., Creitz, E. E., and Holley, H. L. (1962). Trace metal composition of synovial fluid from patients with rheumatoid arthritis. Arthritis Rheum. 5, 439–444. doi: 10.1002/art.1780050502
O’Brien, J., Wilson, I., Orton, T., and Pognan, F. (2000). Investigation of the Alamar Blue (resazurin) fluorescent dye for the assessment of mammalian cell cytotoxicity. Eur. J. Biochem. 267, 5421–5426. doi: 10.1046/j.1432-1327.2000.01606.x
Pacifici, M., Cossu, G., Molinaro, M., and Tato, F. (1980). Vitamin A inhibits chondrogenesis but not myogenesis. Exp. Cell Res. 129, 469–474. doi: 10.1016/0014-4827(80)90517-0
Penick, K. J., Solchaga, L. A., and Welter, J. F. (2005). High-throughput aggregate culture system to assess the chondrogenic potential of mesenchymal stem cells. Biotechniques 39, 687–691. doi: 10.2144/000112009
Puetzer, J. L., Petitte, J. N., and Loboa, E. G. (2010). Comparative review of growth factors for induction of three-dimensional in vitro chondrogenesis in human mesenchymal stem cells isolated from bone marrow and adipose tissue. Tissue Eng. Part B Rev. 16, 435–444. doi: 10.1089/ten.TEB.2009.0705
Ren, F. L., Guo, X., Zhang, R. J., Zuo, H., Zhang, Z. T., Geng, D., et al. (2007). Effects of selenium and iodine deficiency on bone, cartilage growth plate and chondrocyte differentiation in two generations of rats. Osteoarthritis Cartilage 15, 1171–1177. doi: 10.1016/j.joca.2007.03.013
Sardesai, V. M. (1993). Molybdenum: an essential trace element. Nutr. Clin. Pract. 8, 277–281. doi: 10.1177/0115426593008006277
Sato, K., Mera, H., Wakitani, S., and Takagi, M. (2017). Effect of epigallocatechin-3-gallate on the increase in type II collagen accumulation in cartilage-like MSC sheets. Biosci. Biotechnol. Biochem. 81, 1241–1245. doi: 10.1080/09168451.2017.1282809
Schreiber-Brynzak, E., Klapproth, E., Unger, C., Goschl, S., Schweighofer, S., Trondl, R., et al. (2015). Three-dimensional and co-culture models for preclinical evaluation of metal-based anticancer drugs. Invest. New Drugs 33, 835–847. doi: 10.1007/s10637-015-0260-4
Shahin, K., and Doran, P. M. (2011). Strategies for enhancing the accumulation and retention of extracellular matrix in tissue-engineered cartilage cultured in bioreactors. PLoS One 6:e23119. doi: 10.1371/journal.pone.0023119
Sophia Fox, A. J., Bedi, A., and Rodeo, S. A. (2009). The basic science of articular cartilage: structure, composition, and function. Sports Health 1, 461–468. doi: 10.1177/1941738109350438
Tas, F., Karabulut, S., Yasasever, C. T., and Duranyildiz, D. (2014). Serum transforming growth factor-beta 1 (TGF-β1) levels have diagnostic, predictive, and possible prognostic roles in patients with melanoma. Tumor Biol. 35, 7233–7237. doi: 10.1007/s13277-014-1984-z
Tian, H. T., Zhang, B., Tian, Q., Liu, Y., Yang, S. H., and Shao, Z. W. (2013). Construction of self-assembled cartilage tissue from bone marrow mesenchymal stem cells induced by hypoxia combined with GDF-5. J. Huazhong Univ. Sci. Technolog. Med. Sci. 33, 700–706. doi: 10.1007/s11596-013-1183-y
Tsonis, P. A. (1991). 1,25-Dihydroxyvitamin D3 stimulates chondrogenesis of the chick limb bud mesenchymal cells. Dev. Biol. 143, 130–134. doi: 10.1016/0012-1606(91)90060-g
Weidenbecher, M., Henderson, J. H., Tucker, H. M., Baskin, J. Z., Awadallah, A., and Dennis, J. E. (2007). Hyaluronan-based scaffolds to tissue-engineer cartilage implants for laryngotracheal reconstruction. Laryngoscope 117, 1745–1749. doi: 10.1097/mlg.0b013e31811434ae
Weidenbecher, M., Tucker, H. M., Awadallah, A., and Dennis, J. E. (2008). Fabrication of a neotrachea using engineered cartilage. Laryngoscope 118, 593–598. doi: 10.1097/MLG.0b013e318161f9f8
Whitney, G. A., Jayaraman, K., Dennis, J. E., and Mansour, J. M. (2014). Scaffold-free cartilage subjected to frictional shear stress demonstrates damage by cracking and surface peeling. J. Tissue Eng. Regen. Med. 11, 412–424. doi: 10.1002/term.1925
Whitney, G. A., Kean, T. J., Fernandes, R. J., Waldman, S., Tse, M. Y., Pang, S. C., et al. (2018). Thyroxine increases collagen type II expression and accumulation in scaffold-free tissue-engineered articular cartilage. Tissue Eng. Part A 24, 369–381. doi: 10.1089/ten.TEA.2016.0533
Wluka, A. E., Stuckey, S., Brand, C., and Cicuttini, F. M. (2002). Supplementary vitamin E does not affect the loss of cartilage volume in knee osteoarthritis: a 2 year double blind randomized placebo controlled study. J. Rheumatol. 29, 2585–2591.
Yazar, M., Sarban, S., Kocyigit, A., and Isikan, U. E. (2005). Synovial fluid and plasma selenium, copper, zinc, and iron concentrations in patients with rheumatoid arthritis and osteoarthritis. Biol. Trace Elem. Res. 106, 123–132. doi: 10.1385/bter:106:2:123
Ye, J., Coulouris, G., Zaretskaya, I., Cutcutache, I., Rozen, S., and Madden, T. L. (2012). Primer-BLAST: a tool to design target-specific primers for polymerase chain reaction. BMC Bioinformatics 13:134. doi: 10.1186/1471-2105-13-134
Yoo, J. U., Barthel, T. S., Nishimura, K., Solchaga, L., Caplan, A. I., Goldberg, V. M., et al. (1998). The chondrogenic potential of human bone-marrow-derived mesenchymal progenitor cells. J. Bone Joint Surg. Am. 80, 1745–1757.
Keywords: cartilage tissue engineering, defined chondrogenic media, high-throughput chondrogenesis, high-throughput organoid, in vitro chondrogenesis, defined media
Citation: Dennis JE, Splawn T and Kean TJ (2020) High-Throughput, Temporal and Dose Dependent, Effect of Vitamins and Minerals on Chondrogenesis. Front. Cell Dev. Biol. 8:92. doi: 10.3389/fcell.2020.00092
Received: 01 November 2019; Accepted: 04 February 2020;
Published: 25 February 2020.
Edited by:
Dominic C. Voon, Kanazawa University, JapanReviewed by:
Jim Y. Tiao, Murdoch University, AustraliaRicky R. Lareu, Curtin University, Australia
Roberto Narcisi, Erasmus University Rotterdam, Netherlands
Copyright © 2020 Dennis, Splawn and Kean. This is an open-access article distributed under the terms of the Creative Commons Attribution License (CC BY). The use, distribution or reproduction in other forums is permitted, provided the original author(s) and the copyright owner(s) are credited and that the original publication in this journal is cited, in accordance with accepted academic practice. No use, distribution or reproduction is permitted which does not comply with these terms.
*Correspondence: Thomas J. Kean, dGhvbWFzLmtlYW5AdWNmLmVkdQ==