- 1Centre for Biomaterials and Tissue Engineering (CBIT), Universitat Politècnica de València, Valencia, Spain
- 2Biomedical Research Networking Centre in Bioengineering, Biomaterials and Nanomedicine (CIBER-BBN), Madrid, Spain
- 3Division of Biomedical Engineering, Centre for the Cellular Microenvironment, School of Engineering, University of Glasgow, Glasgow, United Kingdom
Embryonic stem cells (ESCs) possess remarkable abilities, as they can differentiate into all cell types (pluripotency) and be self-renewing, giving rise to two identical cells. These characteristics make ESCs a powerful research tool in fundamental embryogenesis as well as candidates for use in regenerative medicine. Significant efforts have been devoted to developing protocols to control ESC fate, including soluble and complex cocktails of growth factors and small molecules seeking to activate/inhibit key signaling pathways for the maintenance of pluripotency states or activate differentiation. Here we describe a novel method for the effective maintenance of mouse ESCs, avoiding the supplementation of complex inhibitory cocktails or cytokines, e.g., LIF. We show that the addition of zinc to ESC cultures leads to a stable pluripotent state that shares biochemical, transcriptional and karyotypic features with the classical LIF treatment. We demonstrate for the first time that ESCs maintained in long-term cultures with added zinc, are capable of sustaining a stable ESCs pluripotent phenotype, as well as differentiating efficiently upon external stimulation. We show that zinc promotes long-term ESC self-renewal (>30 days) via activation of ZIP7 and AKT signaling pathways. Furthermore, the combination of zinc with LIF results in a synergistic effect that enhances LIF effects, increases AKT and STAT3 activity, promotes the expression of pluripotency regulators and avoids the expression of differentiation markers.
Introduction
Embryonic stem cells (ESCs) are the main cellular source during embryogenesis in multicellular organisms, giving rise to the three embryonic germ layers (mesoderm, endoderm and ectoderm) which are the precursors required for the development of functional tissues (Niwa, 2007; Ohtsuka and Dalton, 2008; Evans, 2011). ESCs present a pluripotent phenotype, being able to self-renew and propagate indefinitely in appropriate conditions or differentiate into all embryonic cell lineages spontaneously (Tremml et al., 2008) or in a controlled way (Wichterle et al., 2002; West et al., 2006; Schugar et al., 2008; Evseenko et al., 2010; Kattman et al., 2011). This multilineage differentiation potential, makes them a key tool in research on biological processes, developing new prospects for the medical treatment of different pathologies and as a cell source for regenerative medicine strategies (Clevers, 2016; Yiangou et al., 2018).
ESCs can be obtained from the inner cell mass of the preimplantation blastocyst stage (Tremml et al., 2008; Czechanski et al., 2014). In vitro ESC expansion is challenging due to its marked tendency to spontaneously differentiate into all primary germ layers (Heo et al., 2005; Nair et al., 2015). They thus need to be cultured in very specific conditions that mimic the in vivo niche to inhibit the activation of differentiation mechanisms and promote self-renewal (Stewart et al., 2008; Llames et al., 2015). The most common method of maintaining undifferentiated ESC phenotypes in vitro is their co-culture onto a feeder layer of inactivated mouse embryonic fibroblasts (MEF) (Tamm et al., 2013; Llames et al., 2015), which provides ESC paracrine factors for stemness maintenance (Llames et al., 2015). However, these feeder cells exhibit a heterogeneous population with different surface markers and phenotypes (Singhal et al., 2016) which entail a source of variability during ESC culture.
Among the pool of molecules released by the feeder layer of cells, some growth factors and cytokines involved in the inhibition of ESC differentiation have been identified, such as the Leukemia inhibitory factor (LIF) (Llames et al., 2015). LIF binds to the glycoprotein 130/LIF-receptor and activates multiple signaling pathways, including the tyrosine kinase Janus (JAK) (Ohtsuka and Dalton, 2008; Pera and Tam, 2010; Oshimori and Fuchs, 2012). When JAK phosphorylates, it downstream activates both the PI3K/AKT cascade and the transcription factor STAT3, regulating the expression of self-renewal associated genes (Niwa et al., 1998). Other factors identified are the fibroblast growth factor-2 (FGF2), Activin A, Gremlin 1 or transforming growth factor β1 (TGFβ1), which inhibit human ESC differentiation (Stewart et al., 2008). It is, however, often necessary to combine more than one molecule to achieve strong inhibition of ESC differentiation (Stewart et al., 2008), particularly for human ESCs. Besides the cell-released factors, there is a growing trend toward the study of small molecules to drive ESC fate (Li and Ding, 2010). Among these small molecules, it has been shown that GSK3β inhibitors (such as CHIR 99021; Bechard and Dalton, 2009; Tamm et al., 2013) or ERK1/2 inhibitors (such as SC1, Chen et al., 2006 or PD184352, Kunath et al., 2007; Hamilton and Brickman, 2014), directly or indirectly target the POU5F1, SOX2, NANOG, and KLF4 (Jaenisch and Young, 2008; Li and Ding, 2010), group of transcription factors or protein kinases, such as PI3K/AKT, which form pluripotency associated regulators (Yu and Cui, 2016).
PI3K/AKT signaling is crucial to promote ESC survival by inhibiting apoptosis through phosphorylation and inactivation of BAD or Caspase-9 (Kennedy et al., 1997; Cardone, 1998). In addition, AKT is able to downstream regulate the activity of protein kinases and transcription factors such as GSK3β, NFκβ, MEK/ERK (Armstrong et al., 2006; Yu and Cui, 2016), or POU5F1 (Lin et al., 2012), which control the activity and expression of proliferation and pluripotency-associated genes. GSK3β and ERK activity are commonly associated with loss of pluripotency. GSK3β binds and phosphorylates β-Catenin, triggering its proteasomal degradation (Doble, 2003), and consequently disrupts the Wnt/β-Catenin signaling, necessary for preserving ESC integrity and somatic cell reprogramming (Berge et al., 2011; Xu et al., 2016). In the same way, ERK activity is closely related to the suppression of ESC self-renewal and specification to endoderm lineage (Hamilton and Brickman, 2014). Inhibition of both protein kinases (GSK3β and ERK), known as the 2i condition, enhances ESC self-renewal, avoiding differentiation (Morgani et al., 2013). On the other hand, NFκβ and POU5F1 transcriptional activity are absolutely necessary for pluripotency gene expression (Armstrong et al., 2006). The role of the PI3K/AKT pathway in the regulatory process of ESC survival and pluripotency makes it an interesting target to promote ESC self-renewal. Among the molecules that activate the PI3K/AKT cascade, growth factors such as FGF2 (Johnson-Farley et al., 2007; Eiselleova et al., 2009), IGF1/2, Insulin (Johnson-Farley et al., 2007; Franke, 2008) or small molecules such as SC79 (Jo et al., 2012) or zinc have been reported (Taylor et al., 2008).
Zinc is a transition metal involved in the activity of several proteins, such as transcription factors or enzymes, and may act as a neurotransmitter (Murakami and Hirano, 2008). In addition, the role of Zn2+ has been established as an intracellular second messenger down regulating phosphatase activity (Yamasaki et al., 2007), which upgrades the phosphorylation of several protein kinases such as ERK, JNK, or AKT (Yamasaki et al., 2007; Taylor et al., 2012), promoting their activity. Several authors have reported that zinc ions are involved in the activation of the PI3K/AKT cascade through phosphorylation of AKT (Taylor et al., 2012; Cohen et al., 2014; Shao et al., 2017). After addition of extracellular concentrations of zinc, intracellular Zn2+ concentration increases, a phenomenon that is controlled by the ZIP7 transporter in the endoplasmic reticulum (Yamasaki et al., 2007; Taylor et al., 2008, 2012). Subsequently ZIP7 inhibits phosphatase activity and triggers Zn-associated AKT phosphorylation, similarly to growth factor stimuli (Taylor et al., 2012). The role of Zn2+ in the transient inhibition of murine ESC differentiation has only recently been described, associating this behavior with the activation of the STAT3 transcription factor (Hu et al., 2016). However, it is noteworthy that STAT3 activity is regulated by the protein EZH2, which in fact is a substrate for AKT-mediated phosphorylation (Zhou et al., 2007; Kim et al., 2013), opening up the possibility of STAT3 activity being a consequence of upstream activation of the PI3K/AKT pathway.
Here we unravel the mechanism by which zinc ions controlled ESCs and set up new strategies to control the pluripotency of ESCs. We demonstrate the role of extracellular Zn2+ on the fate of mouse ESC through the PI3K/AKT signaling cascade. We show that the addition of Zn2+ only, induces self-renewal mechanisms and maintains pluripotency after long-term cultures. We also show that Zn2+ successfully reinforces the effect of LIF, and avoids spontaneous ESC differentiation. We thus have identified zinc as an alternative to the various and complex cocktails of small molecules and growth factors classically used for stable ESC maintenance in vitro.
Materials and Methods
ESCs Culture and Embryoid Body Formation
Murine embryonic stem cells D3 (ESCs, ATCC) were cultured in feeder-free conditions in basal medium (BM) composed of DMEM high glucose (Lonza) supplemented with 10% Knockout Serum Replacement (KSR, Thermo Fisher Scientific), 1% Fetal Bovine Serum (FBS, Gibco), 1% 100X Nucleosides (Millipore), 1% L-Glutamine (Sigma-Aldrich), 1% Non-essential Amino Acids (Sigma-Aldrich), 1% Penicillin/Streptomycin (P/S, Gibco) and 10 mM 2-Mercatoethanol (Gibco) at 37°C in a humidified incubator containing 5% CO2. 1,000 U/ml Leukemia inhibitory factor (LIF, Millipore) was used to inhibit ESC differentiation (growth medium). Before seeding, culture dishes were coated with 0.2% gelatin (Sigma-Aldrich). Zinc chloride (Sigma-Aldrich) was used as source of Zn2+ for in vitro experiments.
For embryoid bodies (EBs), ESCs previously grown for 30 days (30d-ESC) in BM (30d-BM) BM supplemented with Zn 100 μM (30d-Zn) or LIF (30d-LIF) were cultured in hanging drops (1,000 cells/20 μL drop). EBs for histological cuts were collected after 5 days of culture, transferred to non-adhesive flasks, and cultured for 10 additional days prior to histological analysis. EBs used for spontaneous ESC differentiation were cultured in DMEM/F12 medium (Sigma-Aldrich) supplemented with 10% FBS, 1% L-Glutamine, 1% P/S.
ESCs Intracellular Zn2+ Homeostasis
For analysis of intracellular Zn2+ wave induction, cells were incubated with FluoZin3-AM (2 μM) for 40 min and washed with PBS, before adding medium at different zinc concentrations (40, 100, and 140 μM). Fluorescence emission was measured every 40 s for approximately 60 min. BM and BM supplemented with LIF were used as control. Fluorescence emission was analyzed by a Victor III plate reader (Perkin Elmer).
Immunofluorescence, Staining, and Histology Methods
For immunofluorescence protein detection, cells were fixed with 4% formaldehyde for 20 min at room temperature. Cells were then washed with TBS and blocked with TBS/Triton × 100 0.1%/BSA 2% for 1 h at room temperature and incubated with primary antibody overnight at 4°C (Supplementary Table 1). The samples were subsequently washed and incubated with secondary antibodies for 1 h at room temperature (Supplementary Table 1). Hoechst (dil: 1/7,000, Sigma-Aldrich) was used for nuclear staining. Samples were mounted with 85% glycerol and imaged by a Nikon Eclipse i80 fluorescence microscope. The percentage of protein staining was quantified by image analysis with imageJ software.
To analyze the number of alkaline phosphatase-positive (AP) colonies, ESCs were fixed with 4% formaldehyde and stained with Naphthol AS-MX/Fast Red TR (Sigma-Aldrich) for 30 min at room temperature, as previously described (Ziomek et al., 1990). After staining, cells were rinsed and nuclei were labeled with Hoechst. The samples were imaged by a Nikon Eclipse i80 fluorescence microscope. The percentage of AP stained colonies was evaluated by imageJ software.
For histological sections, EBs were fixed with 4% formaldehyde overnight at 4°C. EBs were embedded into low gelling temperature agarose (Sigma-Aldrich) and paraffin (Polyester Wax, Electron Microscopy) for 6 μm histological sections obtained with HM 350 S (Thermo Fisher Scientific). Paraffin sections were histochemically stained with Haematoxylin/Eosin (Sigma-Aldrich), as previously described (Fischer et al., 2008).
RNA Interference (RNAi)
ESCs were seeded at high density (40,000 cells/cm2) in growth medium (+ LIF). After 24 h, cells were transfected with MISSION esiRNA (Sigma-Aldrich) against ZIP7 (Slc39a7) transporter in X-tremeGENE siRNA Transfection Reagent (Roche), following the manufacturer’s instructions. Cell transfection was carried out in Opti-MEM Reduced Serum medium (Thermo Fisher Scientific) supplemented with LIF. MISSION siRNA Fluorescent Universal Negative Control 1, Cyanine 3 (NC, Sigma-Aldrich) was used as negative control. Transfected ESCs were cultured for 24 h, after which transfection medium was changed for basal medium supplemented with 100 μM Zn2+, 1,000 U/mL LIF or BM only and cultured for an additional 7 days.
AKT Inhibition Experiments
For PI3K/AKT specific inhibition, we used 2-(4-Morpholinyl)-8-phenyl-1(4H)-benzopyran-4-one hydrochloride (LY-294002, Sigma-Aldrich), at a concentration of 10 μM. ESCs were seeded at 20,000 cells/cm2 in growth medium. After 24 h, growth medium was replaced for BM supplemented with 100 μM Zn2+, LIF or BM only and LY-294002. After 2 days, LY-294002 depleted medium was replaced in the different conditions for 7 days.
Western Blot Analysis
For protein expression analysis, total protein extraction was performed with RIPA buffer supplemented with protease inhibitor cocktail tablets (Roche). Proteins were separated in 10% SDS-PAGE with Mini-PROTEAN Electrophoresis System (Bio-Rad) and transferred to a PVDF (GE-Healthcare) membrane by Trans-Blot Semi-Dry Transfer Cell (Bio-Rad). Membranes were then blocked with 5% BSA (Sigma-Aldrich) in TBS/0.1% Tween 20 (Sigma-Aldrich). For subsequent incubation with primary antibodies (Supplementary Table 2) they were diluted on TBS 0.1% Tween 20 and 3% BSA and incubated overnight at 4°C. Membranes were washed and incubated with HRP-linked secondary antibody (Supplementary Table 2) for 1 h at room temperature for chemiluminiscence band detection by ECL-Plus reactive (Thermo Fisher Scientific). A Fujifilm Las-3000 imager device was used for protein band visualization.
For detection of phosphorylated proteins (pAKT and pGSK3β), PVDF membranes were first incubated with specific antibodies against phosphorylated forms of the proteins (pAKT, pGSK3β) following the standard procedure explained before. After that, membranes were stripped using a detergent-containing buffer Western Blot Stripping Buffer (Thermo Fisher Scientific) for anti-phospho antibodies removal, and afterward reprobed again with the antibodies against the total protein (non-phosphorylated AKT and GSK3β) as a loading control.
Gene Expression Analysis by Quantitative Real Time PCR
Total RNA was extracted from ESCs using Quick RNA Miniprep kit (ZYMO Research); quantity and integrity were measured on a Q3000 micro volume spectrophotometer (Quawell). RNAs were reverse transcribed by a Maxima First Strand cDNA Synthesis Kit with Thermolabile dsDNase (Thermo Fisher Scientific). Real-time qPCR was carried out on a PowerUp SYBR Master Mix (Thermo Fisher Scientific) and ViiA 7 Real-Time PCR System (Thermo Fisher Scientific). The reactions were run four times (independent biological experiments). The primers used for amplification are indicated in Supplementary Table 3. The fractional cycle number at which fluorescence passed the threshold (Ct values) was used for gene expression quantification by the comparative ΔΔCt method. Sample values were normalized to the threshold value of Gapdh housekeeping gene.
Statistical Analyses
Each experiment was performed at least four times unless otherwise noted. Data were reported as mean ± standard deviation. The D’Agostino-Pearson omnibus test was used to determine whether the data obtained followed a normal distribution. Results were analyzed by one-way ANOVA on GraphPad Prism 6.0. When differences were found to be significant, Tukey pairwise comparisons were performed on normal data distributions or a Dunn’s test in the opposite case. A 95% confidence level was considered significant. The linear regression of immunofluorescence image quantification and Pearson’s r correlation values was obtained on R software.
Results
Zn2+ Influences ESC Viability and Proliferation
To determine zinc concentrations that are toxic for ESCs, cell viability was analyzed using concentrations of Zn2+ from 40 to 240 μM at 1, 4, and 7 days (Supplementary Figure 1). ESC viability was maintained with Zn2+ concentrations of up to 140 μM, and decreased significantly at concentrations of 160 and 240 μM (Supplementary Figure 1A).
For proliferation experiments, Zn2+ concentrations that compromised ESC viability (over 160 μM) were discarded. Total DNA was measured after supplementing cells with 40, 100, and 140 μM Zn2+ for 1, 3, 5 or 7 days. In Supplementary Figure 1B no differences can be seen in cell proliferation for the different Zn2+ concentrations, which remained similar to those of the LIF and basal medium conditions.
Zn2+ Promotes ESCs Self-Renewal
To study the mechanisms induced by soluble Zn2+ in ESCs, we first measured the Zn2+ cytosolic intake. The intracellular Zn2+ concentration was quantified for different concentrations of supplemented extracellular Zn2+ after 1, 3, and 5 days of culture. Free intracellular Zn2+ was labeled with FluoZin3-AM (Supplementary Figure 1C). Intracellular Zn2+ rose monotonically with extracellular Zn2+ concentration. The culture was maintained for 5 days, after which Zn2+ was removed from the medium and the culture left for 1 h, which led to a drastic drop in intracellular Zn2+ concentration [reaching levels similar to the basal medium (BM)]. This demonstrates that intracellular ESC zinc concentration is regulated by the concentration of extracellular Zn2+ in the culture medium.
As Zn2+ is an intracellular secondary messenger involved in multiple cellular events (Yamasaki et al., 2007), we then measured intracellular “zinc waves” in ESCs after adding Zn2+ to the culture medium. Figure 1A shows that intracellular Zn2+ rose rapidly on the addition of 100 and 140 μM and reached a plateau after 10 min. However, supplementing with 40 μM Zn2+ led to a steady uptake of intracellular Zn2+, which was still increasing after 60 min. Intracellular Zn2+ did not rise in the basal medium (BM) and LIF supplemented medium (LIF) used as controls.
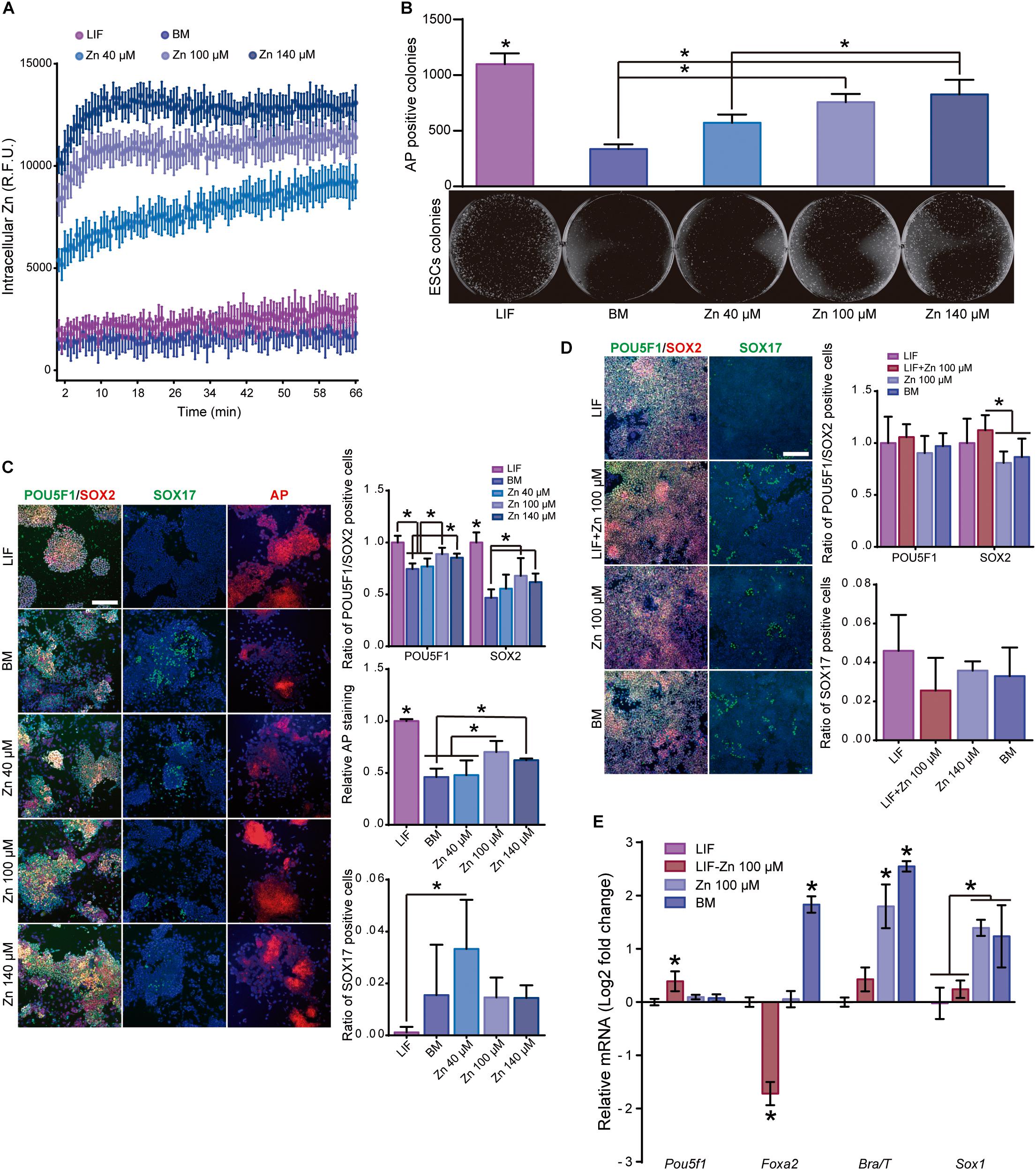
Figure 1. Role of zinc in ESC self-renewal. (A) Intracellular Zn2+ wave determined by FluoZin3-AM labeling (data points were taken every 40 s). Cells were kept at 37°C throughout the analysis (n = 6). (B) AP staining of ESCs after 5 days of culture in BM, medium supplemented with LIF or medium supplemented with different concentrations of Zn2+ (n = 3). (C) Immunofluorescence detection of pluripotency markers (POU5F1 and SOX2) and staining of AP in ESCs after 5 days of culture in BM, medium supplemented with LIF or medium supplemented with different concentrations of Zn2+ (n = 5). (D) Immunofluorescence detection of pluripotency (POU5F1 and SOX2) and differentiation markers (endoderm: SOX17) determined after 5 days of culture in accelerated differentiation conditions (medium supplemented with 5% KSR) (n = 5). (E) qPCR detection of pluripotency markers (Pou5f1) and differentiation markers (endoderm: Foxa2, mesoderm: Brachyury/T and ectoderm: Sox1) determined after 5 days of culture in accelerated differentiation conditions (medium supplemented with 5% KSR). Gapdh was used as housekeeping gene (n = 4). Significant differences were determined by ANOVA test; ∗p < 0.05. Scale bar: 200 μm.
We next assessed the effect of Zn2+ on ESC self-renewal using basal medium (BM) and media supplemented with 40, 100, or 140 μM Zn2+ (Figures 1B,C). LIF-supplemented medium was used as a positive control for stemness maintenance. To determine the potential of ESCs to form alkaline phosphatase (AP) positive colonies in the presence of Zn2+, cells were seeded at 150 cells/cm2 and cultured for 5 days. The quantification of the AP expressing colonies indicated that the number of ESCs colonies increased monotonically as the extracellular concentration of Zn2+ does (Figure 1B). Then, ESCs were seeded at low density (10,000 cells/cm2) until sub-confluence (3 days) and subsequently sub-cultured for a total of 6 days. We used POU5F1, SOX2, and AP as pluripotency markers, and SOX17 as spontaneous differentiation marker. Figure 1C shows that only 100 and 140 μM Zn2+ concentrations produced high levels of POU5F1 and SOX2 expression as well as larger AP positive colonies than in the ESCs cultured in BM. Using 40 μM Zn2+-treated cultured cells was not enough to maintain pluripotency markers that remain similar to the BM control. Accordingly, 40 μM Zn2+-treated ESCs expressed the highest SOX17 values. These results show that adding sufficiently high concentrations of Zn2+ to the culture medium maintains ESCs stemness for more than 6 days.
To show that Zn2+ promotes ESC self-renewal, we performed additional experiments to induce cell differentiation using defined media in the presence of Zn2+. ESCs were seeded at 10,000 cells/cm2 in BM supplemented with LIF. After 24 h of culture, the medium was replaced by BM supplemented with 5% KSR (to stimulate ESC differentiation; Hamatake et al., 2000) and 100 μM Zn2+, LIF, or a combination of both LIF and 100 μM Zn2+. Figures 1D,E show immunofluorescence and qPCR pluripotency expression values (POU5F1 and SOX2) and primary germ layer differentiation markers (endoderm: Foxa2; mesoderm: Brachyury/T; ectoderm: Sox1) after 5 days of culture. ESCs treated with either LIF or Zn2+ had lower expressions of differentiation markers than the BM control. Endoderm marker expression (Foxa2 and Sox17) were similar in the Zn2+ and LIF-treated cells. However, Zn2+-treated ESCs showed an upregulated expression of ectoderm and mesoderm markers (Brachyury/T and Sox1). However, the best results in terms of pluripotency were obtained with a combination of LIF and 100 μM Zn2+. In addition to downregulating the expression of differentiation markers, ESCs treated with LIF-100 and μM Zn2+ also displayed a slight overexpression of Pou5f1. These results further demonstrate that zinc maintains ESC self-renewal potential even under accelerated differentiation conditions.
Zn2+ Maintains ESCs Stemness Activating ZIP7 Transporter and AKT Signaling Transduction Pathway
Zn2+ transporter ZIP7 is found in different organelles and cytoplasmic vesicles associated with Zn2+ storage (Huang et al., 2005; Hogstrand et al., 2009), and drives Zn2+ influx from intracellular storages to cytoplasm. ZIP7 activation has been extensively associated with AKT phosphorylation (Taylor et al., 2008), which is a key protein kinase regulator of the ESC self-renewal pathway (Watanabe et al., 2006). To determine the role of ZIP7 and AKT in ESCs we designed two experiments to evaluate the effect of zinc: (i) AKT inhibition with the PI3K/AKT chemical inhibitor LY-294002, and (ii) transient down regulation of ZIP7 transporter using siRNAs.
For the first approach, ESCs were cultured in BM, in a medium supplemented with 100 μM Zn2+ or LIF and incubated for 2 days with LY-294002. ESCs were then cultured 5 more days prior to measurement of the pluripotency expression markers (POU5F1 and AP). After 24 h of treatment with LY-294002, AKT phosphorylation levels were reduced in all conditions, regardless of the presence of Zn2+ or LIF in the culture medium (Figure 2A). After 7 days of culture and PI3K/AKT inhibition, POU5F1 and AP levels dropped significantly in ESCs cultured with Zn2+ and in BM conditions, while their levels remained unaltered in the LIF-supplemented cells, presenting similar values to the control without LY-294002 inhibitor (Figures 2C,D). These results demonstrate that AKT signaling is involved in maintaining ESC self-renewal by Zn2+ but not by LIF.
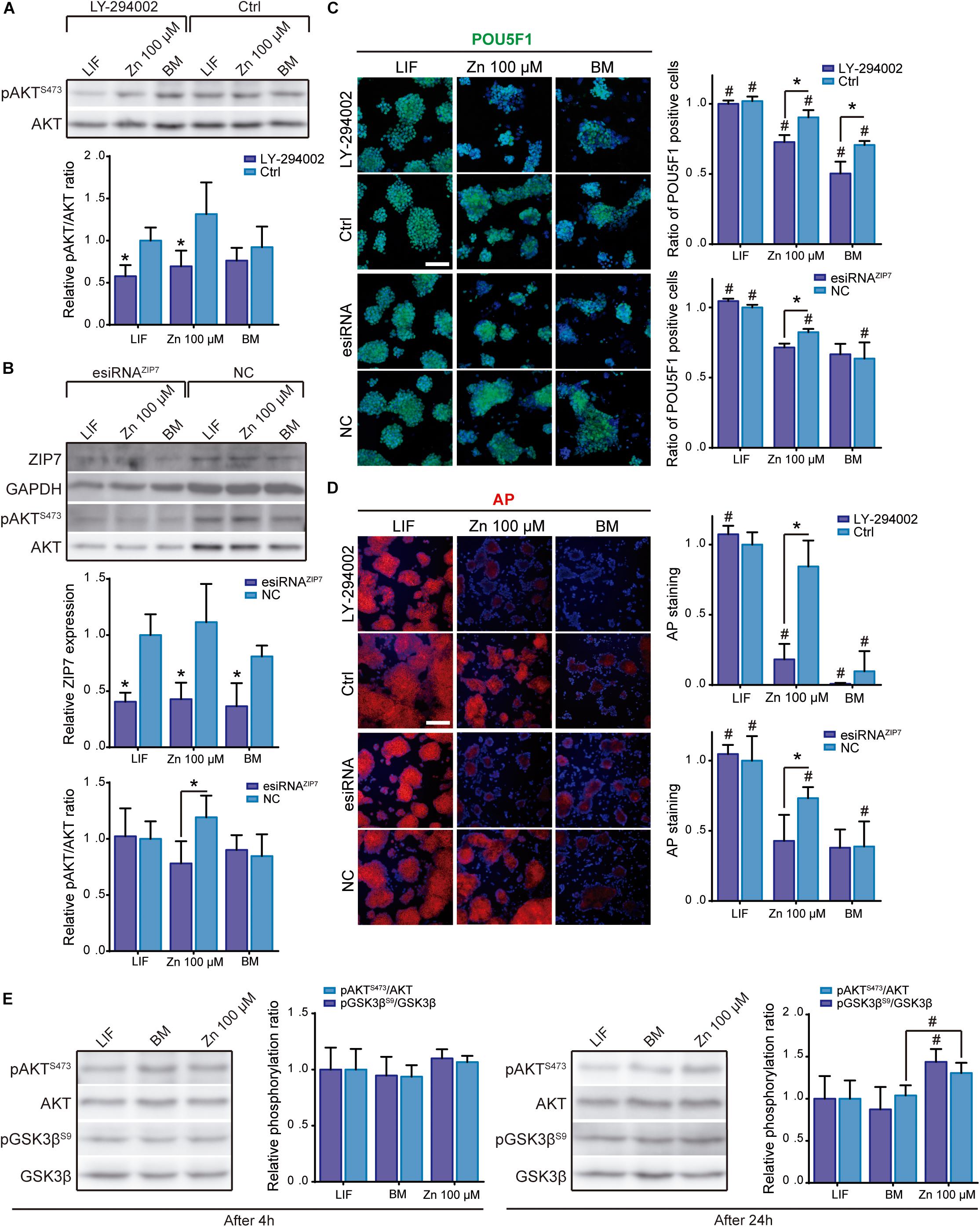
Figure 2. Role of ZIP7 and AKT in ESCs stemness maintenance. (A) Analysis of pAKT/AKT ratio by western blot after AKT inhibition with LY-294002 10 μM during 24 h in cells cultured in BM, medium supplemented with LIF or medium supplemented with 100 μM of Zn2+. GAPDH was used as loading control protein (n = 4). (B) Analysis of ZIP7 expression and pAKT/AKT ratio by western blot after ZIP7 silencing with RNAi after 3 days of culture. GAPDH was used as loading control protein (n = 4). Cells were cultured in BM and a medium supplemented with LIF or medium supplemented with 100 μM of Zn2+. (C,D) Immunofluorescence detection of pluripotency markers (POU5F1-green and alkaline phosphatase-red, AP) determined after 7 days of culture in BM, medium supplemented with LIF or medium supplemented with 100 μM of Zn2+, and treated 24 h with AKT inhibitor LY-294002, or ZIP7 silenced with RNAi during 3 days. Scale bar: 100 μm. (E) Analysis of pAKT/AKT (S473) and pGSK3β/GSK3β (S9) ratios by western blot in ESCs cultured in BM, medium supplemented with LIF or medium supplemented with 100 μM of Zn2+ for 4 or 24 h. GAPDH was used as loading control protein (n = 4). Significant differences were determined by ANOVA test; between groups: ∗p < 0.05; within group: #p < 0.05.
In the second approach, we treated ESCs with RNAi against ZIP7 transporter. After 3 days of ZIP7 silencing, ESCs were cultured in BM and a medium supplemented with 100 μM Zn2+ or LIF. Figure 2B shows the western blot experiments of ZIP7 expression after silencing by RNAi. ZIP7 expression was found to drop significantly more in all conditions than with the RNAi negative control (NC).
AKT phosphorylation after ZIP7 silencing was only significantly reduced in ESCs supplemented with Zn2+ (Figure 2B), suggesting that zinc transport inhibition greatly affects AKT phosphorylation. Note that both ZIP7 expression and pAKT/AKT ratio followed a similar trend in the control conditions; the highest levels were found in cells supplemented with 100 μM Zn2+, reinforcing the hypothesis that ZIP7 activation promotes AKT phosphorylation.
We further analyzed the expression of POU5F1 and AP markers after knocking down ZIP7. In Figure 2A significant reduction can be seen in the self-renewal effect induced by Zn2+, which reduced the expression of POU5F1 and AP to the BM levels. It is interesting to note that ZIP7 knock down is transient, and after 6 days of culture both, the ZIP7 expression and pAKT/AKT ratio fully recovered (Supplementary Figure 2). However, silencing ZIP7 impairs the maintenance of stemness markers, even after 7 days of culture and in the presence of Zn2+, showing that the effect of zinc on self-renewal maintenance is dependent of the Zn2+ transporter ZIP7.
We then evaluated GSK3β phosphorylation to determine whether Zn2+-mediated AKT activation further stimulated downstream phosphorylation of AKT target substrates. ESCs were cultured in BM and a medium supplemented with 100 μM Zn2+or LIF. In Figure 2E no differences can be seen in the pAKT/AKT or pGSK3β/GSK3β ratios after 4 h of culture in the different conditions used. However, after 24 h both AKT and GSK3β phosphorylation increased in the Zn2+- supplemented cells, whilst the LIF and BM conditions showed similar lower values. These results further support the hypothesis that zinc maintains ESC self-renewal by inducing AKT phosphorylation, which then promotes phosphorylation of downstream target proteins such as GSK3β, neutralizing their activity and promoting stemness maintenance.
Zn2+–Mediated AKT Activation Is Closely Associated With Intra-Nuclear Presence of Transcription Factor POU5F1
To determine how AKT interacts with the key POU5F1 and SOX2 pluripotency regulators, we studied their intracellular location. Immunostaining for POU5F1, SOX2, and pAKT (S473) showed that they are found in the cell nuclei, whether or not exogenous Zn2+ is added. We also observed that cell nuclei were surrounded by the Zn2+ transporter ZIP7, which is anchored to the endoplasmic reticulum (Figure 3A). Like GSK3β, both SOX2 and POU5F1 possess the RXRXXS/T AKT recognition motif (Manning and Cantley, 2007; Figure 3B and Supplementary Figure 3), proteins which have been reported to be substrates for AKT phosphorylation activity (Doble, 2003; Jeong et al., 2010; Lin et al., 2012). Phosphorylation of both POU5F1 and SOX2 is critical to complete the pluripotency-associated gene expression programme (Saxe et al., 2009; Jeong et al., 2010; Lin et al., 2012). To determine whether the nuclear presence of POU5F1 and SOX2 depends on nuclear pAKT, ESCs were cultured for 3 days in BM and a medium supplemented with either LIF or 100 μM Zn2+. In addition, we inhibited AKT phosphorylation by adding 10 μM LY-294002 in the same experimental conditions and then analyzed the nuclear expression of POU5F1, SOX2, and pAKT by immunostaining (Figure 3C). Zn2+ treated cells displayed the highest correlation values between the nuclear presence of POU5F1 and SOX2 transcription factors with pAKT. Adding PI3K inhibitor produced a negative effect in pAKT, which in the cells grown in BM and Zn2+ was also transferred to the POU5F1 levels, while the SOX2 levels only varied slightly. These results suggest that alterations in AKT signaling strongly affect POU5F1 nuclear presence, in the absence of any additional stimuli such as STAT3 activation via LIF.
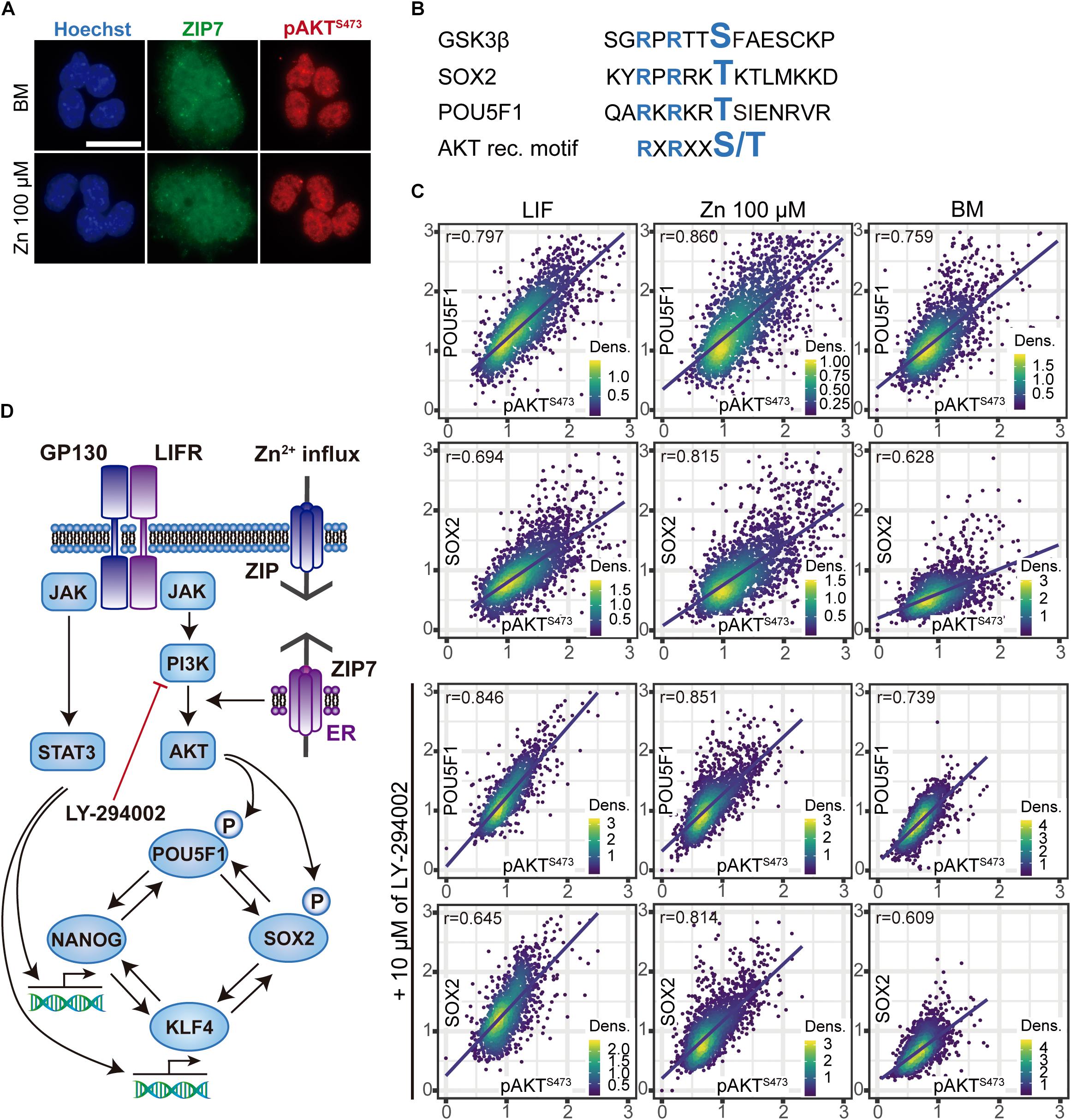
Figure 3. Relationship between nuclear pAKT and the core transcriptional network for ESC self-renewal. (A) Immunostaining of Zn2+ transporter ZIP7 and pAKT (S473) in ESCs cultured for 24 h in BM only or BM supplemented with 100 μM of Zn2+. Scale bar: 50 μm. (B) Alignments of aminoacid sequences of mouse GSK3β, POU5F1, and SOX2 showing the target motifs for AKT-mediated phosphorilation. NetPhos 3.1 Server was used to identify these sequences, which then were aligned with the minimal recognition motif of AKT. (C) Immunofluorescence detection and quantification of pluripotency markers POU5F1, SOX2, and pAKT (S473) in ESCs cultured for 3 days in normal medium and medium supplemented with 10 μM of PI3K inhibitor LY-294002. Obtained data were represented and Pearson’s correlation r-values were obtained using R. (D) Proposed mechanism illustrating the effects of Zn2+ in AKT phosphorylation and ESCs self-renewal. Addition of extracellular Zn2+ in the culture medium, activate ZIP7 and AKT downstream pathways. Note that these results have been determined experimentally in this work and the illustrated steps activated by AKT to maintain ESCs pluripotency have been previously reported in the literature (Saxe et al., 2009; Jeong et al., 2010; Lin et al., 2012).
The mechanism that emerges from these experiments is represented in Figure 3D and explains how Zn2+ interacts with AKT and the core transcriptional network that regulates ESC pluripotency.
Zn2+ Maintains ESC Pluripotency in Long-Term Cultures
After showing that Zn2+ promotes ESC stemness maintenance, we then studied whether ESCs maintain pluripotency after long-term cultures in a medium supplemented with Zn2+. Cells were cultured in expansion conditions (seeding density: 30,000 cells/cm2, passaged every 2–3 days at sub-confluence) in order to reduce spontaneous differentiation (Tremml et al., 2008). After 30 days of culture in BM, LIF and 100 μM Zn2+, pluripotency and differentiation markers were analyzed by immunofluorescence and qPCR (Figures 4A,B). Immunofluorescence showed high POU5F1 and SOX2 expressions after 30 days of culture in the presence of Zn2+, with values similar to LIF and higher than the BM control (Figure 4A). Indeed, the ratio of AP positive colonies was similar for Zn2+ and LIF-treated cells and approximately 50% higher than for the BM condition.
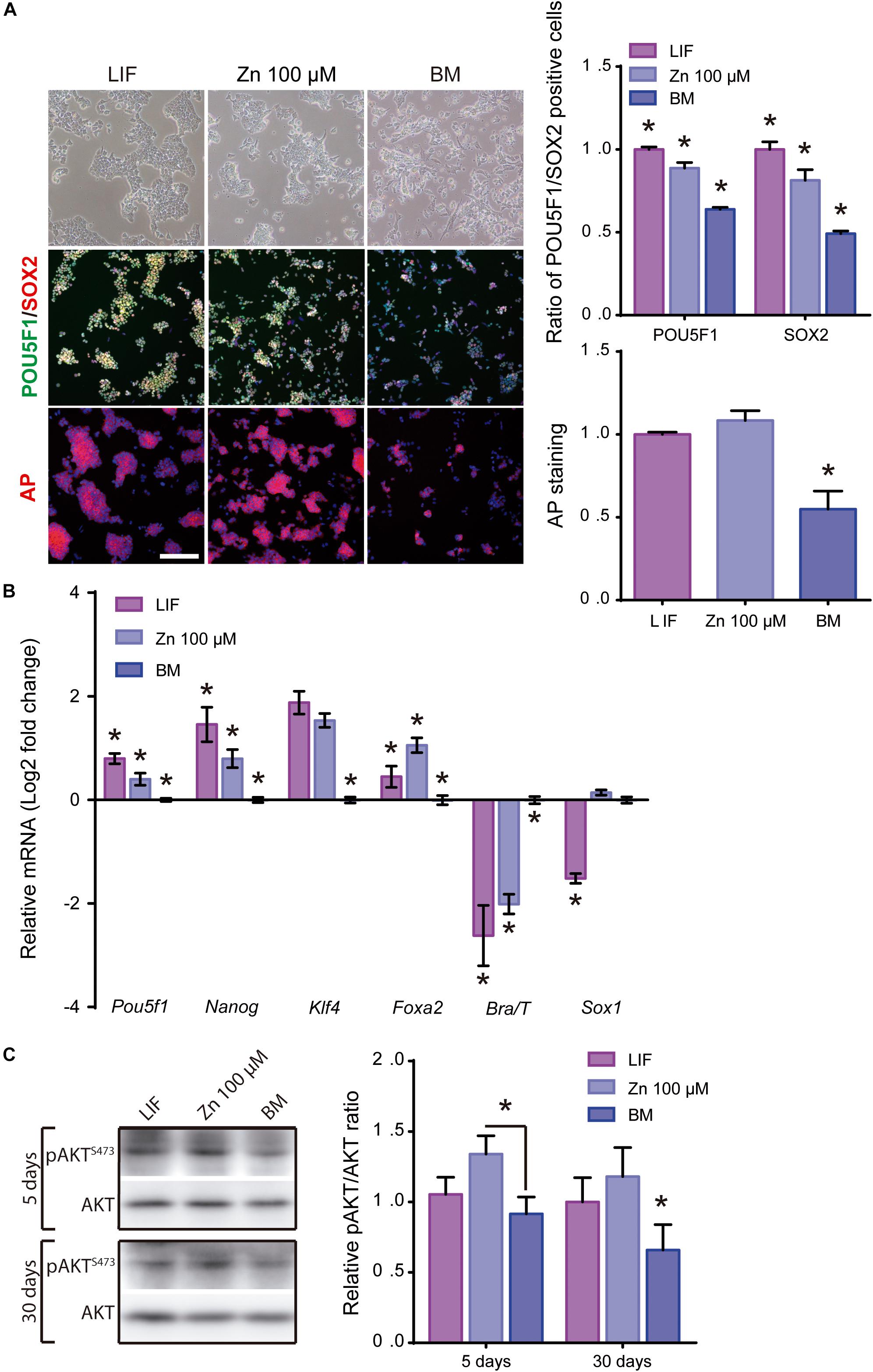
Figure 4. Role of zinc in ESCs pluripotency maintenance after 30 days of culture. (A) Immunofluorescence detection of pluripotency markers: POU5F1-green, SOX2-red and alkaline phosphatase-red, determined after 30 days of ESCs culture, in BM and a medium supplemented with LIF or medium supplemented with 100 μM of Zn2+ (n = 5). Scale bar: 200 μm. (B) qPCR detection of pluripotency markers (Pou5f1, Nanog, Klf4) and differentiation markers (endoderm: Foxa2; mesoderm: Brachyury/T and ectoderm: Sox1). Gapdh was used as housekeeping gene (n = 4). (C) Western blot detection of AKT and pAKT (S473) on ESCs cultured in BM and a medium supplemented with LIF or medium supplemented with 100 μM of Zn2+after 1, 5, and 30 days of culture (n = 4). Significant differences were determined by ANOVA test; ∗p < 0.05.
Gene expression analysis of pluripotency (Pou5f1, Nanog, KIf4) markers showed that Zn2+ provided similar expression levels to LIF (Figure 4B), confirming the immunofluorescence images (Figure 4A). In contrast, for specific genes related to differentiation, Foxa2 expression was higher for Zn2+ than LIF-treated cells. Brachyury/T expression was higher for BM cultured cells than those treated with Zn2+ or LIF, which displayed similar values, while Sox1 expression was similar for Zn2+ and BM cultured cells (Figure 4B).
We further evaluated AKT activity after culturing ESCs for 5 and 30 days in the different conditions. Figure 4C shows that the pAKT/AKT ratio remained higher for ESCs supplemented with Zn2+ further supporting the hypothesis that long-term Zn2+-mediated stemness is mediated by AKT phosphorylation.
To disregard the aberrant chromosome content in ESCs due to long-term culture times, we analyzed ESCs karyotype after 30 days of culture (Supplementary Figure 5). No differences were found between the different conditions and all showed a modal distribution of 40 chromosomes.
Zn2+ Maintains ESC Differentiation Capacity After Long-Term Cultures
Having shown that Zn2+ maintains ESC self-renewal potential rather than differentiating into specific primary germ layers, we next evaluated whether ESC differentiation potential into different lineages was maintained after long-term cultures. ESC spontaneous differentiation capacity after 30 days of culture (30d-ESC) in BM (30d-BM), medium supplemented with 100 μM Zn2+ (30d-Zn) or LIF (30d-LIF) was assessed after embryoid body (EBs) formation. EBs from low passage ESCs were included as a control (1d-LIF). EBs were formed in hanging drops for 5 days and subsequently transferred to non-adhesive plates for 10 additional days. Five days old EBs were collected to analyze gene expression of primary germ layer markers. Fifteen days old EBs were collected and fixed for histological sections. No appreciable morphological differences were found between EBs obtained from 30d-Zn or 30d-LIF EBs and control EBs (1d-LIF) (Figure 5A). Nevertheless, EBs generated from 30d-BM cells had smaller diameters, which suggests less growth from the third day of culture for the different times analyzed. Gene expression related to primary germ layers rose significantly in Sox1 (ectoderm) levels and the Brachyury/T (mesoderm) levels dropped sharply (Figure 5B).
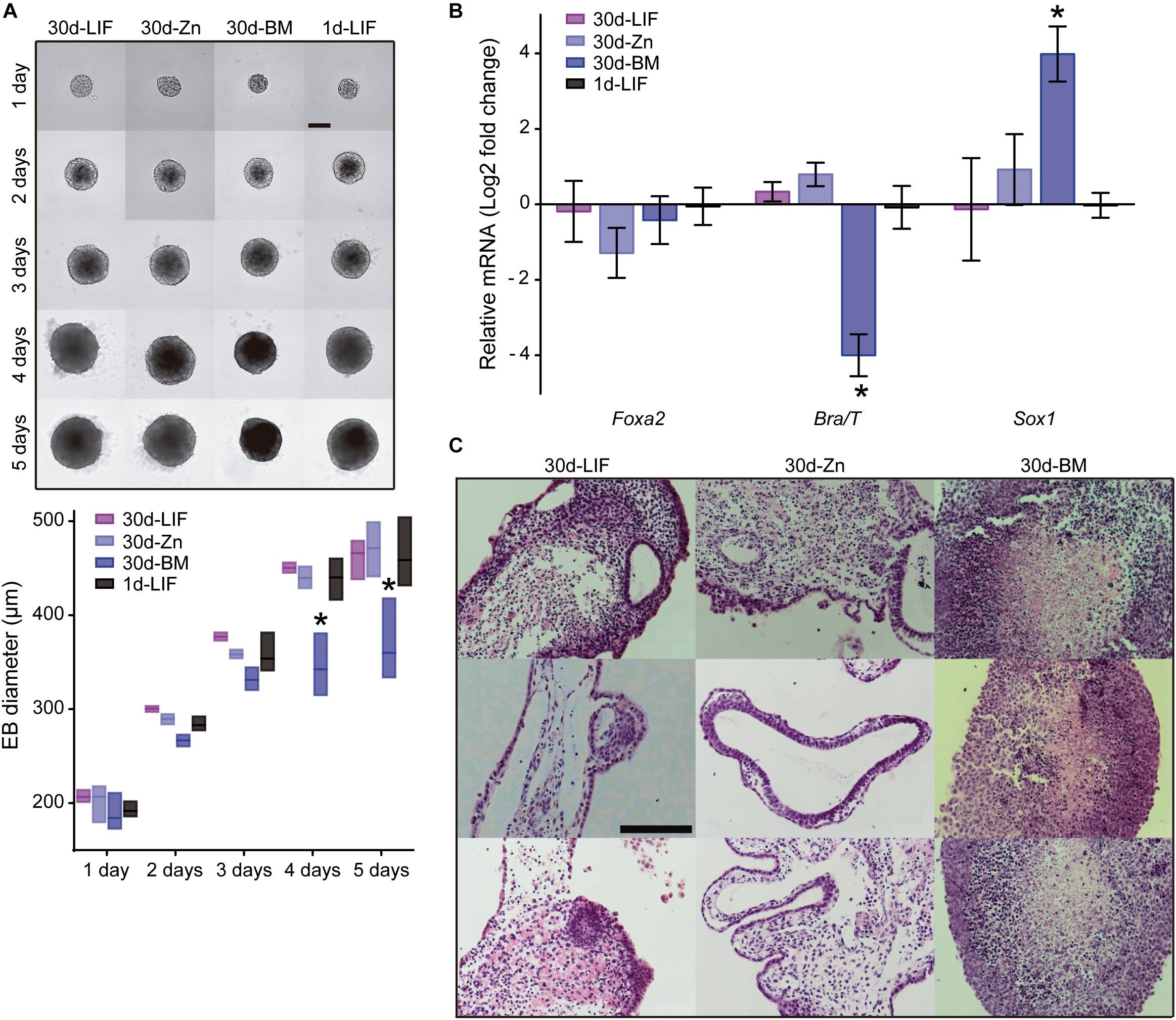
Figure 5. Role of zinc in spontaneous embryoid body differentiation after 30 days of culture. (A) Embryoid body formation of ESCs cultured for 30 days in BM and a medium supplemented with LIF or 100 μM Zn2+. New EBs formed from low passage ESCs (not previously cultured for 30 days under different conditions) were included as a control of embryoid body formation (1d-LIF). Measurements of EB diameters (n = 9). Scale bar: 200 μm. (B) Evaluation of spontaneous differentiation of EBs formed after culture of ESCs for 30 days in BM and a medium supplemented with LIF or 100 μM Zn2+. qPCR detection of primary germ layer markers: endoderm (Foxa2), mesoderm (Brachyury/T) and ectoderm (Sox1). Gapdh was used as housekeeping gene (n = 4). (C) Hematoxylin/eosin staining of histological sections of EBs after 15 days of culture. EBs were formed after culture of ESCs for 30 days in BM, medium supplemented with LIF or 100 μM Zn2+. Scale bar: 100 μm. Significant differences were determined by ANOVA test: ∗p < 0.05.
We also examined the differentiation capacity of ESCs previously cultured for 30 days in BM and a medium supplemented with LIF or 100 μM Zn2+ under defined media conditions (Supplementary Figure 6). Similar results were found for all the induced lineages using 30d-LIF cells and 1d-LIF cells, although the 30d-Zn cells were quite different to the other conditions. For mesoderm differentiation, 30d-Zn presented lower cell density and a substantially reduced expression of CD31 and CD34 markers, together with neural-like morphology (Supplementary Figure 6B). For endoderm differentiation, 30d-Zn cells expressed SOX17, but appeared more clustered than 30d-LIF and 1d-LIF cells. Finally, for neuroectoderm differentiation, similar SOX10 and βIII-TUB images were obtained in all conditions, with 30d-BM cells showing the highest levels.
Overall, these results indicated that, as in the case of LIF-treated cells, addition of zinc to the culture medium maintains the long-term ESCs’ ability to differentiate into the three lineages.
Zn2+ Maintains a Stable ESCs Pluripotent State After Long-Term Cultures
As the ESC population is highly heterogeneous (Nair et al., 2015), it contains naïve cells together with other cells primed for differentiation states, with transcriptome variations concerning the pluripotency associated gene expression (Lanner and Rossant, 2010). Our results show that 30d-Zn cells maintained their self-renewal capacity and also spontaneous differentiation toward all primary germ layers in EBs, but with lower levels of mesoderm-directed differentiation (Supplementary Figure 5B). An additional experiment was carried out to determine whether this behavior is related to ESC heterogeneity after long-term cultures. ESCs previously cultured for 30 days in BM (30d-BM) and a medium supplemented with LIF (30d-LIF) or 100 μM Zn+2 (30d-Zn) were then treated with LIF for 3 more days in an attempt to revert ESC priming to the naïve state. Figure 6 shows immunofluorescence and qPCR results for pluripotency (Pou5f1, Nanog, SOX2) and differentiation (Gata6, Fgf5, Brachyury) markers. When 30d-Zn cells were exposed to LIF, the ratio of POU5F1 positive cells increased by ca. 12%, while 30d-LIF and 30d-BM levels remained constant. SOX2 values decreased for 30d-BM cells, whilst 30d-Zn and 30d-LIF cells levels did not vary significantly. In parallel, ESCs previously cultured for 30 days in BM (30d-BM) and a medium supplemented with LIF (30d-LIF) or 100 μM Zn2+ (30d-Zn) were treated for 3 days in BM as a control for LIF-mediated signaling. We found that 30d-LIF and 30d-BM conditions reduced the POU5F1 and SOX2 levels from the initial values, whilst the 30d-Zn levels remained almost the same (Figure 6A).
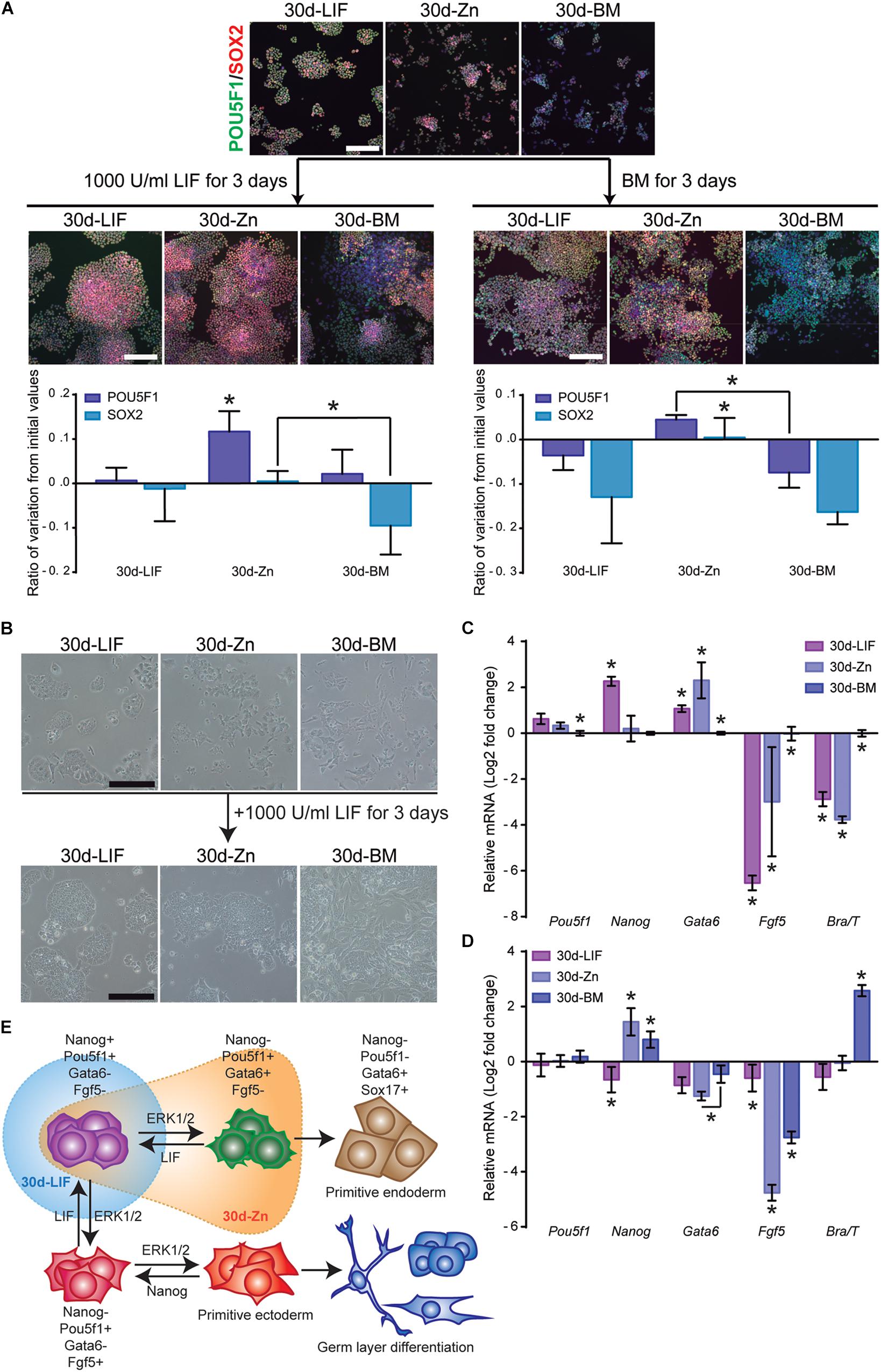
Figure 6. Zinc maintains naïve ESC state after long-term cultures. (A) ESCs previously cultured for 30 days in BM (30d-BM) and a medium supplemented with LIF (30d-LIF) or 100 μM Zn2+ (30d-Zn) were treated with LIF or BM for 3 more days. Pluripotency markers (POU5F1-green and SOX2-red) were determined by immunofluorescence (n = 5). Scale bar: 200 μm. (B) Bright field pictures of 30d-ESC cultured in BM (30d-BM) and a medium supplemented with LIF (30d-LIF) or 100 μM Zn2+ (30d-Zn) after 3 days of culture in the presence of LIF. Scale bar: 200 μm. (C) qPCR evaluation of stemness-related markers of pluripotency (Pou5f1, Nanog) indicative of naïve state, and differentiation markers (Gata6, Brachyury/T, Fgf5) indicative of primed state, of ESCs previously cultured for 30 days in BM (30d-BM), medium supplemented with LIF (30d-LIF) or 100 μM Zn2+(30d-Zn). Gapdh was used as housekeeping gene (n = 4). (D) qPCR evaluation of stemness related markers of pluripotency (Pou5f1, Nanog) indicative of naïve state, and differentiation markers (Gata6, Brachyury/T, Fgf5) indicative of primed state, of ESCs previously cultured for 30 days in BM (30d-BM), medium supplemented with LIF (30d-LIF) or 100 μM Zn2+ (30d-Zn) and treated with LIF for 3 more days in order to reverse primed to naïve state. Gapdh was used as housekeeping gene (n = 4). (E) Scheme of ESC pluripotency dynamics, indicating the state of the ESC population cultured with LIF (blue) and Zn (orange) after 30 days of culture. Significant differences were determined by ANOVA test; ∗p < 0.05.
Before the 3-day LIF treatment (Figure 6C), similar results were obtained after gene expression analysis. Nanog expression was significantly higher for 30d-LIF cells, while 30d-Zn and 30d-BM cells showed a similar expression. Furthermore, Gata6 was more expressed in 30d-LIF and 30d-Zn cells, while other differentiation markers (Fgf5, Brachyury) appeared to be less than the 30d-BM cells. After 3 days of LIF treatment (Figure 6D), the 30d-Zn cells rescued Nanog expression, with similar values to 30d-LIF cells, and the expression of Gata6 and Fgf5 differentiation markers decreased substantially, suggesting reversion from the primed to naïve state. Although 30d-BM cells exhibited a similar trend for Nanog, Gata6 and Fgf5 levels, after 3 days of LIF treatment the differences were significantly higher in the 30d-Zn cells. Note that Brachyury expression increased substantially for 30d-BM cells despite the LIF treatment (Figure 6D), suggesting incomplete transition from primed to naïve state for this condition.
The Combination of LIF and Zn2+ Work Synergistically to Maintain Pluripotency
Our results show that Zn2+ successfully sustains ESC pluripotency. Nevertheless, although 30d-Zn ESCs share many features with 30d-LIF ESCs, these cells possess particular traits, which suggest that 30d-Zn cells are more differentiated. As previous observations had indicated that the combination of LIF and Zn2+ strongly inhibited ESC differentiation (Figure 1D), we therefore carried out additional experiments combining different concentrations of LIF (LIF: 1,000 U/ml and 1/4LIF: 250 U/ml) and Zn2+ (100 μM) to enhance pluripotency maintenance. ESCs were cultured for 15 days (5 passages) in the presence of LIF, 1/4LIF, LIF-Zn, and 1/4LIF-Zn. In each passage, cells were fixed and the ratio of AP positive colonies determined as well as SOX2 and SOX17 positive cells. After 15 days, the expression of both pluripotency (Nanog) and differentiation (Brachyury/T, Gata6, and Fgf5) markers was analyzed by qPCR and protein expression was analyzed by Western Blot. Results indicate that ESCs grown with 1/4LIF gradually lost pluripotency after 15 days (Figure 7A). These cells had lower ratios of AP positive colonies and SOX2 positive cells in addition to higher Brachyury and Fgf5 expressions (Figure 7B). In addition, cells cultured with 1/4LIF also had the lowest AKT activity and STAT3 phosphorylation. Interestingly, adding 100 μM of Zn2+ to this condition (1/4LIF-Zn), reinforced pluripotency to levels comparable to ESCs cultured with 1,000 U/ml LIF (LIF) (Figure 7C). On the other hand, the combination of LIF with 100 μM Zn2+ led to a higher Nanog expression than other conditions, and a significantly higher amount of pSTAT3 than ESCs in the presence of 1/4LIF only. However, the conditions that contained Zn2+ also expressed slightly higher Gata6 values.
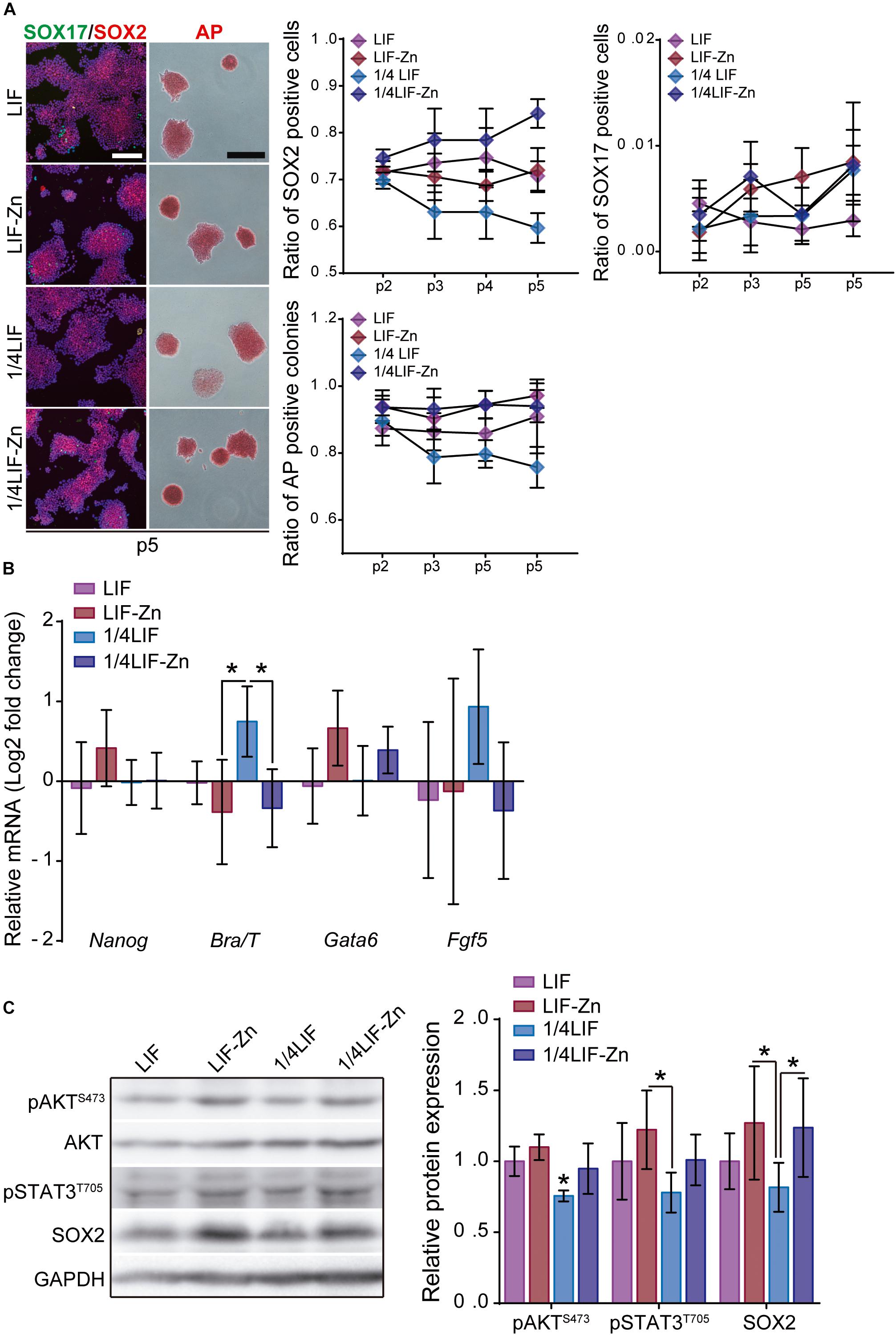
Figure 7. The combination of LIF with Zn2+ sustains ESC pluripotency with greater efficiency. (A) ESCs were cultured for 15 days, passaging cells each 3 days, in presence of either 1,000 U/ml of LIF (LIF), 1,000 U/ml LIF + 100 μM Zn2+ (LIF-Zn), 250 U/ml of LIF (1/4 LIF), and 250 U/ml LIF + 100 μM Zn2+ (1/4LIF-Zn). After each passage, ESCs were fixed and stained for alkaline phosphatase (AP) (n = 4) and immunostained for SOX2 and SOX17 (n = 5). Scale bar: 200 μm. (B) qPCR evaluation of stemness-related markers of pluripotency (Nanog) indicative of naïve state, and differentiation markers (Gata6, Brachyury/T, Fgf5) indicative of primed state, of ESCs previously cultured for 15 days. Gapdh was used as housekeeping gene (n = 4). (C) Western blot detection of AKT, pAKT (S473), pSTAT3 (T705), and SOX2 on ESCs cultured for 15 days. GAPDH was used as a housekeeping gene (n = 4). Significant differences were determined by ANOVA test; ∗p < 0.05.
Discussion
Although the need for zinc in biological processes has often been associated with its function as a cofactor in metalloproteins (Anzellotti and Farrell, 2008), its role in signaling processes is becoming increasingly important. Describing Zn2+ as a second messenger (Yamasaki et al., 2007) helps to explain the mechanisms associated with Zn2+ signaling as a useful tool for controlling cell fate. However, our results indicate that adding 40, 100, and 140 μM Zn2+ affected neither cell viability nor proliferation as compared to LIF or BM conditions (Supplementary Figure 1). Furthermore, zinc dynamics studies indicate that intracellular zinc uptake depends on the presence of extracellular zinc (Figure 1A) and is controlled by the ZIP7 transporter.
We have shown that Zn2+–mediated signaling promotes ESC self-renewal (Figure 1). The expression pattern of the pluripotency markers was similar after 100 μM Zn2+ and LIF supplementation, indicating that zinc inhibited spontaneous ESC differentiation and maintained the self-renewal potential rather than differentiating into specific germ layers, even under accelerated differentiation conditions (Figure 1D).
To better understand the underlying mechanisms involved in Zn2+–dependent ESCs stemness maintenance, we focused on PI3K/AKT activation as a consequence of the Zn2+ wave (Taylor et al., 2012). Inhibition of PI3K/AKT activity by LY-294002 significantly increased ESC differentiation marker levels in zinc and BM conditions, whereas the LIF condition remained unaltered (Figure 2). Although more subtly, similar results were obtained for Zn2+ treated cells after ZIP7 silencing (Figure 2). These findings are supported by previous works describing the link between ZIP7 and Zn2+–mediated AKT activation (Taylor et al., 2008, 2012). Our results suggest that ZIP7 plays a key role in Zn2+ mediated ESC self-renewal. It must be emphasized that LIF does not act exclusively via AKT activation to inhibit spontaneous ESC differentiation, as neither AKT inhibition nor ZIP7 silencing affected LIF–treated cells (Figure 2). The observed differences could be related to the mechanism whereby these molecules (Zn2+ or LIF) inhibit ESC differentiation. It has been reported that LIF promotes pluripotency gene expression by activating the transcription factor STAT3 and PI3K/AKT phosphorylation via JAK (Murray and Edgar, 2001; Rose-John, 2002). Instead the Zn2+ mechanism would be associated only with PI3K/AKT signaling, downstream activated after stimulating the ZIP7 transporter with extracellular Zn2+as we have shown in Figures 2, 3 (Taylor et al., 2008, 2012). Activating the protein kinase AKT entails the phosphorylation of its target substrates, such as GSK3β, which is inactivated after phosphorylation and stops promoting ESC differentiation (Fang et al., 2000; Bechard and Dalton, 2009; Figure 2E).
The role of AKT in ESC self-renewal has already been demonstrated by several authors (Watanabe et al., 2006; Yu and Cui, 2016). In addition, we have also observed that phospho-AKT (S473) as well as ZIP7 are essentially located in the ESC nuclei (Figure 3), which, in response to the increased extracellular Zn2+ concentration promotes AKT phosphorylation. It is likely that the AKT subcellular location makes it more available for substrates involved in ESC self-renewal in the same subcellular compartment such as POU5F1 (Lin et al., 2012) or SOX2 (Jeong et al., 2010; Supplementary Figure 3). Furthermore, we also observed that the co-localization of POU5F1 and SOX2 increased in Zn2+-treated ESCs (Supplementary Figure 4), supporting the hypothesis that the development of POU5F1-SOX2 heterodimers activates pluripotency related gene expression. Moreover, AKT is also a strong anti-apoptotic agent, targeting and inactivating pro-apoptotic proteins such as BAX (Yamaguchi and Wang, 2001). It was recently reported that ESCs lacking BAX and BAK are unable to exit from pluripotency (Wang et al., 2015), adding more relevance to AKT activity in ESC self-renewal.
Importantly, we have also shown that Zn2+ signaling is strong enough to promote ESC self-renewal even after 30 days of culture, maintaining both ESC–associated pluripotency markers and also AKT phosphorylation (Figure 4). Further, 30d-Zn treated ESCs spontaneously differentiate after EB formation, similarly to 30d-LIF and 1d-LIF conditions (Figure 5). When ESC differentiation was triggered by external stimuli, 30d-Zn cells differentiated toward all primary germ layers in a monolayer culture, notwithstanding the lack of mesoderm-directed differentiation (Supplementary Figure 6). We hypothesize that this lack of mesoderm differentiation is due to the pluripotency state of Zn2+ maintained ESC (Weinberger et al., 2016). As we have also observed, after treatment of 30d-Zn cells with 1,000 U/ml LIF, seeking to revert ESC priming to the naïve state by counterbalancing pro-differentiation signals (Lanner and Rossant, 2010; Weinberger et al., 2016), 30d-Zn cells rescued Nanog expression, whereas differentiation-associated genes expression (Gata6, Fgf5, Brachyury/T) dropped significantly, indicating an efficient transition from the primed to naïve state (Figure 6). Even though these 30d-Zn cells are in a more differentiated stage than LIF supplemented ESCs, their phenotype is highly reversible. The heterogeneity of the 30d-Zn cells and their being more primed for the differentiation state would explain their different response to differentiation signals than the LIF cultured cells. Furthermore, the higher expression of Gata6 suggests a considerable population of ESCs primed for endoderm linage (Lanner and Rossant, 2010; Hamilton and Brickman, 2014).
The incorporation of Zn2+ has been shown to be enough to maintain ESC pluripotency. Further, the combination of Zn2+ with LIF showed a synergistic effect, which successfully increased ESC self-renewal, even in differentiation conditions (Figure 1D). The combination of Zn2+ and LIF not only prevented ESC differentiation significantly more than just LIF or Zn2+, but also enhanced the effect of LIF after long-term cultures (Figure 7). Although we have demonstrated that LIF and Zn2+ maintain pluripotency throughout different mechanisms, the combination of both reinforced AKT and STAT3 activity. This synergistic effect allows reduced concentration of LIF from 1,000 U/ml to 250 U/ml without loss of ESC self-renewal, maintaining ESCs pluripotent, without reducing the expression of pluripotency markers even after 15 days. By contrasts, the monotonically reduction in the number of AP positive colonies and SOX2 positive cells, together with the higher expression of Brachyury, demonstrated that just 250 U/ml of LIF is not enough to maintain ESC pluripotency.
Conclusion
In conclusion, we found Zn2+ to be a small molecule capable of sustaining a stable ESC state as well as increasing the LIF effect in both expansion and differentiation conditions. The Zn2+-dependent activity of ESC self-renewal is mediated via ZIP7 and AKT activation and is maintained over a long time (more than 30 days) without additional growth factors or soluble molecules. Further, Zn2+-treated ESCs maintained pluripotency after long-term cultures and successfully differentiated to all primary germ layers. We therefore propose Zn2+ as a novel approach to control ESC fate regulation in cellular therapies.
Data Availability
The datasets generated for this study are available on request to the corresponding author.
Author Contributions
HM, MS-S, and PR contributed to conception and design of the study. HM wrote the first version of the manuscript, performed the experiments, and made figures. PR and MS-S supervised the research and wrote the final version of the manuscript. All authors contributed to manuscript revision, read and approved the submitted version.
Funding
PR acknowledges support from the Spanish Ministry of Science, Innovation and Universities (RTI2018-096794), and Fondo Europeo de Desarrollo Regional (FEDER). CIBER-BBN was an initiative funded by the VI National R&D&I Plan 2008-2011, Iniciativa Ingenio 2010, Consolider Program, CIBER Actions and financed by the Instituto de Salud Carlos III with assistance from the European Regional Development Fund. MS-S acknowledges support from the UK Engineering and Physical Sciences Research Council (EPSRC - EP/P001114/1).
Conflict of Interest Statement
The authors declare that the research was conducted in the absence of any commercial or financial relationships that could be construed as a potential conflict of interest.
Supplementary Material
The Supplementary Material for this article can be found online at: https://www.frontiersin.org/articles/10.3389/fcell.2019.00180/full#supplementary-material
References
Anzellotti, A. I., and Farrell, N. P. (2008). Zinc metalloproteins as medicinal targets. Chem. Soc. Rev. 37, 1629–1651. doi: 10.1039/b617121b
Armstrong, L., Hughes, O., Yung, S., Hyslop, L., Stewart, R., Wappler, I., et al. (2006). The role of PI3K/AKT, MAPK/ERK and NFκβ signalling in the maintenance of human embryonic stem cell pluripotency and viability highlighted by transcriptional profiling and functional analysis. Hum. Mol. Genet. 15, 1894–1913. doi: 10.1093/hmg/ddl112
Bechard, M., and Dalton, S. (2009). Subcellular localization of glycogen synthase kinase 3 controls embryonic stem cell self-renewal. Mol. Cell. Biol. 29, 2092–2104. doi: 10.1128/MCB.01405-08
Berge, D. T., Kurek, D., Blauwkamp, T., Koole, W., Maas, A., Eroglu, E., et al. (2011). Embryonic stem cells require Wnt proteins to prevent differentiation to epiblast stem cells. Nat. Cell Biol. 13, 1070–1077. doi: 10.1038/ncb2314
Cardone, M. H. (1998). Regulation of cell death protease caspase-9 by phosphorylation. Science 282, 1318–1321. doi: 10.1126/science.282.5392.1318
Chen, S., Do, J. T., Zhang, Q., Yao, S., Yan, F., Peters, E. C., et al. (2006). Self-renewal of embryonic stem cells by a small molecule. Proc. Natl. Acad. Sci. U.S.A. 103, 17266–17271. doi: 10.1073/pnas.0608156103
Clevers, H. (2016). Modeling development and disease with organoids. Cell 165, 1586–1597. doi: 10.1016/j.cell.2016.05.082
Cohen, L., Sekler, I., and Hershfinkel, M. (2014). The zinc sensing receptor, ZnR/GPR39, controls proliferation and differentiation of colonocytes and thereby tight junction formation in the colon. Cell Death Dis. 5, e1307–e1312. doi: 10.1038/cddis.2014.262
Czechanski, A., Byers, C., Greenstein, I., Schrode, N., Donahue, L. R., Hadjantonakis, A.-K., et al. (2014). Derivation and characterization of mouse embryonic stem cells from permissive and nonpermissive strains. Nat. Protoc. 9, 559–574. doi: 10.1038/nprot.2014.030
Doble, B. W. (2003). GSK-3: tricks of the trade for a multi-tasking kinase. J. Cell Sci. 116, 1175–1186. doi: 10.1242/jcs.00384
Eiselleova, L., Matulka, K., Kriz, V., Kunova, M., Schmidtova, Z., Neradil, J., et al. (2009). A complex role for FGF-2 in self-renewal, survival, and adhesion of human embryonic stem cells. Stem Cells 27, 1847–1857. doi: 10.1002/stem.128
Evans, M. (2011). Discovering pluripotency: 30 years of mouse embryonic stem cells. Nat. Rev. Mol. Cell Biol. 12, 680–686. doi: 10.1038/nrm3190
Evseenko, D., Zhu, Y., Schenke-Layland, K., Kuo, J., Latour, B., Ge, S., et al. (2010). Mapping the first stages of mesoderm commitment during differentiation of human embryonic stem cells. Proc. Natl. Acad. Sci. U.S.A. 107, 13742–13747. doi: 10.1073/pnas.1002077107
Fang, X., Yu, S. X., Lu, Y., Bast, R. C., Woodgett, J. R., and Mills, G. B. (2000). Phosphorylation and inactivation of glycogen synthase kinase 3 by protein kinase A. Proc. Natl. Acad. Sci. U.S.A. 97, 11960–11965. doi: 10.1073/pnas.220413597
Fischer, A. H., Jacobson, K. A., Rose, J., and Zeller, R. (2008). Hematoxylin and eosin staining of tissue and cell sections. Cold Spring Harb. Protoc. 2008:pdb.prot4986. doi: 10.1101/pdb.prot4986
Franke, T. F. (2008). PI3K/Akt: getting it right matters. Oncogene 27, 6473–6488. doi: 10.1038/onc.2008.313
Hamatake, M., Iguchi, K., Hirano, K., and Ishida, R. (2000). Zinc induces mixed types of cell death, necrosis and apoptosis, in Molt-4 cells. J. Biochem. 128, 933–939. doi: 10.1093/oxfordjournals.jbchem.a022844
Hamilton, W. B., and Brickman, J. M. (2014). Erk signaling suppresses embryonic stem cell self-renewal to specify endoderm. Cell Rep. 9, 2056–2070. doi: 10.1016/j.celrep.2014.11.032
Heo, J., Lee, J. S., Chu, I. S., Takahama, Y., and Thorgeirsson, S. S. (2005). Spontaneous differentiation of mouse embryonic stem cells in vitro: characterization by global gene expression profiles. Biochem. Biophys. Res. Commun. 332, 1061–1069. doi: 10.1016/j.bbrc.2005.04.173
Hogstrand, C., Kille, P., Nicholson, R. I., and Taylor, K. M. (2009). Zinc transporters and cancer: a potential role for ZIP7 as a hub for tyrosine kinase activation. Trends Mol. Med. 15, 101–111. doi: 10.1016/j.molmed.2009.01.004
Hu, J., Yang, Z., Wang, J., Yu, J., Guo, J., Liu, S., et al. (2016). Zinc chloride transiently maintains mouse embryonic stem cell pluripotency by activating Stat3 signaling. PLoS One 11:e0148994. doi: 10.1371/journal.pone.0148994
Huang, L., Kirschke, C. P., Zhang, Y., and Yan, Y. Y. (2005). The ZIP7 gene (Slc39a7) encodes a zinc transporter involved in zinc homeostasis of the Golgi apparatus. J. Biol. Chem. 280, 15456–15463. doi: 10.1074/jbc.M412188200
Jaenisch, R., and Young, R. (2008). Stem cells, the molecular circuitry of pluripotency and nuclear reprogramming. Cell 132, 567–582. doi: 10.1016/j.cell.2008.01.015
Jeong, C. H., Cho, Y. Y., Kim, M. O., Kim, S. H., Cho, E. J., Lee, S. Y., et al. (2010). Phosphorylation of Sox2 cooperates in reprogramming to pluripotent stem cells. Stem Cells 28, 2141–2150. doi: 10.1002/stem.540
Jo, H., Mondal, S., Tan, D., Nagata, E., Takizawa, S., Sharma, A. K., et al. (2012). Small molecule-induced cytosolic activation of protein kinase Akt rescues ischemia-elicited neuronal death. Proc. Natl. Acad. Sci. U.S.A. 109, 10581–10586. doi: 10.1073/pnas.1202810109
Johnson-Farley, N. N., Patel, K., Kim, D., and Cowen, D. S. (2007). Interaction of FGF-2 with IGF-1 and BDNF in stimulating Akt, ERK, and neuronal survival in hippocampal cultures. Brain Res. 1154, 40–49. doi: 10.1016/j.brainres.2007.04.026
Kattman, S. J., Witty, A. D., Gagliardi, M., Dubois, N. C., Niapour, M., Hotta, A., et al. (2011). Stage-specific optimization of activin/nodal and BMP signaling promotes cardiac differentiation of mouse and human pluripotent stem cell lines. Cell Stem Cell 8, 228–240. doi: 10.1016/j.stem.2010.12.008
Kennedy, S. G., Wagner, A. J., Conzen, S. D., Jordan, J., Bellacosa, A., Tsichlis, P. N., et al. (1997). The PI 3-kinase/Akt signaling pathway delivers an anti-apoptotic signal. Genes Dev. 11, 701–713. doi: 10.1101/gad.11.6.701
Kim, E., Kim, M., Woo, D. H., Shin, Y., Shin, J., Chang, N., et al. (2013). Phosphorylation of EZH2 activates STAT3 signaling via STAT3 methylation and promotes tumorigenicity of glioblastoma stem-like cells. Cancer Cell 23, 839–852. doi: 10.1016/j.ccr.2013.04.008
Kunath, T., Saba-El-Leil, M. K., Almousailleakh, M., Wray, J., Meloche, S., and Smith, A. (2007). FGF stimulation of the Erk1/2 signalling cascade triggers transition of pluripotent embryonic stem cells from self-renewal to lineage commitment. Development 134, 2895–2902. doi: 10.1242/dev.02880
Lanner, F., and Rossant, J. (2010). The role of FGF/Erk signaling in pluripotent cells. Development 137, 3351–3360. doi: 10.1242/dev.050146
Li, W., and Ding, S. (2010). Small molecules that modulate embryonic stem cell fate and somatic cell reprogramming. Trends Pharmacol. Sci. 31, 36–45. doi: 10.1016/j.tips.2009.10.002
Lin, Y., Yang, Y., Li, W., Chen, Q., Li, J., Pan, X., et al. (2012). Reciprocal regulation of akt and Oct4 promotes the self-renewal and survival of embryonal carcinoma cells. Mol. Cell 48, 627–640. doi: 10.1016/j.molcel.2012.08.030
Llames, S., García-Pérez, E., Meana, Á., Larcher, F., and del Río, M. (2015). Feeder layer cell actions and applications. Tissue Eng. Part B Rev. 21, 345–353. doi: 10.1089/ten.TEB.2014.0547
Manning, B. D., and Cantley, L. C. (2007). AKT/PKB signaling: navigating downstream. Cell 129, 1261–1274. doi: 10.1016/j.cell.2007.06.009
Morgani, S. M., Canham, M. A., Nichols, J., Sharov, A. A., Migueles, R. P., Ko, M. S. H., et al. (2013). Totipotent embryonic stem cells arise in ground-state culture conditions. Cell Rep. 3, 1945–1957. doi: 10.1016/j.celrep.2013.04.034
Murakami, M., and Hirano, T. (2008). Intracellular zinc homeostasis and zinc signaling. Cancer Sci. 99, 1515–1522. doi: 10.1111/j.1349-7006.2008.00854.x
Murray, P., and Edgar, D. (2001). The regulation of embryonic stem cell differentiation by leukaemia inhibitory factor (LIF). Differentiation 68, 227–234. doi: 10.1046/j.1432-0436.2001.680410.x
Nair, G., Abranches, E., Guedes, A. M. V., Henrique, D., and Raj, A. (2015). Heterogeneous lineage marker expression in naive embryonic stem cells is mostly due to spontaneous differentiation. Sci. Rep. 5:13339. doi: 10.1038/srep13339
Niwa, H. (2007). How is pluripotency determined and maintained? Development 134, 635–646. doi: 10.1242/dev.02787
Niwa, H., Burdon, T., Chambers, I., and Smith, A. (1998). Self-renewal of pluripotent embryonic stem cells is mediated via activation of STAT3. Genes Dev. 12, 2048–2060. doi: 10.1101/gad.12.13.2048
Ohtsuka, S., and Dalton, S. (2008). Molecular and biological properties of pluripotent embryonic stem cells. Gene Ther. 15, 74–81. doi: 10.1038/sj.gt.3303065
Oshimori, N., and Fuchs, E. (2012). The harmonies played by TGF-β in stem cell biology. Cell Stem Cell 11, 751–764. doi: 10.1016/j.stem.2012.11.001
Pera, M. F., and Tam, P. P. L. (2010). Extrinsic regulation of pluripotent stem cells. Nature 465, 713–720. doi: 10.1038/nature09228
Rose-John, S. (2002). GP130 stimulation and the maintenance of stem cells. Trends Biotechnol. 20, 417–419. doi: 10.1016/S0167-7799(02)02056-5
Saxe, J. P., Tomilin, A., Schöler, H. R., Plath, K., and Huang, J. (2009). Post-translational regulation of Oct4 transcriptional activity. PLoS One 4:e4467. doi: 10.1371/journal.pone.0004467
Schugar, R. C., Robbins, P. D., and Deasy, B. M. (2008). Small molecules in stem cell self-renewal and differentiation. Gene Ther. 15, 126–135. doi: 10.1038/sj.gt.3303062
Shao, Y., Wolf, P. G., Guo, S., Guo, Y., Rex Gaskins, H., and Zhang, B. (2017). Zinc enhances intestinal epithelial barrier function through the PI3K/AKT/mTOR signaling pathway in Caco-2 cells. J. Nutr. Biochem. 43, 18–26. doi: 10.1016/j.jnutbio.2017.01.013
Singhal, P. K., Sassi, S., Lan, L., Au, P., Halvorsen, S. C., Fukumura, D., et al. (2016). Mouse embryonic fibroblasts exhibit extensive developmental and phenotypic diversity. Proc. Natl. Acad. Sci. U.S.A. 113, 122–127. doi: 10.1073/pnas.1522401112
Stewart, M. H., Bendall, S. C., and Bhatia, M. (2008). Deconstructing human embryonic stem cell cultures: niche regulation of self-renewal and pluripotency. J. Mol. Med. 86, 875–886. doi: 10.1007/s00109-008-0356-9
Tamm, C., Pijuan Galitó, S., and Annerén, C. (2013). A comparative study of protocols for mouse embryonic stem cell culturing. PLoS One 8:e81156. doi: 10.1371/journal.pone.0081156
Taylor, K. M., Hiscox, S., Nicholson, R. I., Hogstrand, C., and Kille, P. (2012). Protein kinase CK2 triggers cytosolic zinc signaling pathways by phosphorylation of zinc channel ZIP7. Sci. Signal. 5:ra11. doi: 10.1126/scisignal.2002585
Taylor, K. M., Vichova, P., Jordan, N., Hiscox, S., Hendley, R., and Nicholson, R. I. (2008). ZIP7-mediated intracellular zinc transport contributes to aberrant growth factor signaling in antihormone-resistant breast cancer cells. Endocrinology 149, 4912–4920. doi: 10.1210/en.2008-0351
Tremml, G., Singer, M., and Malavarca, R. (2008). Culture of mouse embryonic stem cells. Curr. Protoc. Stem Cell Biol. 5, 1C.4.1–1C.4.19. doi: 10.1002/9780470151808.sc01c04s5
Wang, E. S., Reyes, N. A., Melton, C., Huskey, N. E., Momcilovic, O., Goga, A., et al. (2015). Fas-activated mitochondrial apoptosis culls stalled embryonic stem cells to promote differentiation. Curr. Biol. 25, 3110–3118. doi: 10.1016/j.cub.2015.10.020
Watanabe, S., Umehara, H., Murayama, K., Okabe, M., Kimura, T., and Nakano, T. (2006). Activation of Akt signaling is sufficient to maintain pluripotency in mouse and primate embryonic stem cells. Oncogene 25, 2697–2707. doi: 10.1038/sj.onc.1209307
Weinberger, L., Ayyash, M., Novershtern, N., and Hanna, J. H. (2016). Dynamic stem cell states: naive to primed pluripotency in rodents and humans. Nat. Rev. Mol. Cell Biol. 17, 155–169. doi: 10.1101/030676
West, J. A., Park, I.-H., Daley, G. Q., and Geijsen, N. (2006). In vitro generation of germ cells from murine embryonic stem cells. Nat. Protoc. 1, 2026–2036. doi: 10.1038/nprot.2006.303
Wichterle, H., Lieberam, I., Porter, J. A., and Jessell, T. M. (2002). Directed differentiation of embryonic stem cells into motor neurons. Cell 110, 385–397. doi: 10.1016/s0092-8674(02)00835-8
Xu, Z., Robitaille, A. M., Berndt, J. D., Davidson, K. C., Fischer, K. A., Mathieu, J., et al. (2016). Wnt/β-catenin signaling promotes self-renewal and inhibits the primed state transition in naïve human embryonic stem cells. Proc. Natl. Acad. Sci. U.S.A. 113, E6382–E6390. doi: 10.1073/pnas.1613849113
Yamaguchi, H., and Wang, H. G. (2001). The protein kinase PKB/Akt regulates cell survival and apoptosis by inhibiting Bax conformational change. Oncogene 20, 7779–7786. doi: 10.1038/sj.onc.1204984
Yamasaki, S., Sakata-Sogawa, K., Hasegawa, A., Suzuki, T., Kabu, K., Sato, E., et al. (2007). Zinc is a novel intracellular second messenger. J. Cell Biol. 177, 637–645. doi: 10.1083/jcb.200702081
Yiangou, L., Ross, A. D. B., Goh, K. J., and Vallier, L. (2018). Human pluripotent stem cell-derived endoderm for modeling development and clinical applications. Cell Stem Cell 22, 485–499. doi: 10.1016/j.stem.2018.03.016
Yu, J. S. L., and Cui, W. (2016). Proliferation, survival and metabolism: the role of PI3K/AKT/mTOR signalling in pluripotency and cell fate determination. Development 143, 3050–3060. doi: 10.1242/dev.137075
Zhou, J., Wulfkuhle, J., Zhang, H., Gu, P., Yang, Y., Deng, J., et al. (2007). Activation of the PTEN/mTOR/STAT3 pathway in breast cancer stem-like cells is required for viability and maintenance. Proc. Natl. Acad. Sci. U.S.A. 104, 16158–16163. doi: 10.1073/pnas.0702596104
Keywords: zinc, ZIP7, stemness maintenance, embryonic stem cells (ESC), AKT
Citation: Mnatsakanyan H, Sabater i Serra R, Salmeron-Sanchez M and and Rico P (2019) Zinc Maintains Embryonic Stem Cell Pluripotency and Multilineage Differentiation Potential via AKT Activation. Front. Cell Dev. Biol. 7:180. doi: 10.3389/fcell.2019.00180
Received: 20 June 2019; Accepted: 19 August 2019;
Published: 30 August 2019.
Edited by:
Emmanuel S. Tzanakakis, Tufts University, United StatesReviewed by:
Stavros Taraviras, University of Patras, GreeceChunliang Li, St. Jude Children’s Research Hospital, United States
Copyright © 2019 Mnatsakanyan, Sabater i Serra, Salmeron-Sanchez and Rico. This is an open-access article distributed under the terms of the Creative Commons Attribution License (CC BY). The use, distribution or reproduction in other forums is permitted, provided the original author(s) and the copyright owner(s) are credited and that the original publication in this journal is cited, in accordance with accepted academic practice. No use, distribution or reproduction is permitted which does not comply with these terms.
*Correspondence: Manuel Salmeron-Sanchez, manuel.salmeron-sanchez@glasgow.ac.uk; Patricia Rico, parico@upvnet.upv.es