- 1CNRS UMR 5305, Laboratoire de Biologie Tissulaire et Ingénierie Thérapeutique, Lyon, France
- 2Claude Bernard University Lyon 1, Villeurbanne, France
- 3Infinitus Company Ltd., Guangzhou, China
The aim of the present study was to evaluate the impact of the microenvironment produced by dermal microvascular endothelial cells, secondary to a pro-inflammatory challenge, on 2D culture models using dermal fibroblasts and in 3D reconstructed skin model using dermal fibroblasts and keratinocytes from healthy donors. We hypothesized that specific microvascular endothelial low grade inflammation could change fibroblasts phenotype and be involved in extracellular matrix (ECM) modification and skin alteration. Following IFNγ, TNFα, IL-1β pro-inflammatory stress on Human Dermal Endothelial Cells (HDMEC) we observed the increased release of Chemokine ligand 2 (CCL2), IL-6 and IL-8 but not VEGF-A in the conditioned medium (CM). The subsequent addition of this endothelial pro-inflammatory CM in dermal fibroblasts revealed an upregulation of IL6, IL8 and CCL2 but no NF-κB gene expression. The resulting ECM formation was impaired with a reduction of the collagen 1 network and a decrease in COL1A1 gene expression in 2D and 3D models. Collagen 1 and pro-LOX protein expression were significantly reduced confirming an impairment of the collagen network related to endothelial inflammation secretion. To conclude, this work showed that, without any immune cells, the endothelial secretion in response to a pro-inflammatory stress is able to activate the fibroblasts that will maintain the pro-inflammatory environment and exacerbate ECM degradation.
Introduction
Skin microcirculation is located deep in the dermis layer up to the reticular dermis close to the epidermis. Skin microcirculation plays a significant role in regulating skin homeostasis, thermoregulation, blood pressure and inflammatory response (Charkoudian, 2003; Gutterman et al., 2016). It also allows nutrients and systemic factors to reach the whole skin tissue throughout the skin microvascular network from arteries to small capillaries close to the epidermis. Microvascular endothelial cells are the major components of dermal blood vessels and are clearly involved in skin inflammatory process. Endothelial cells participate to synthesize and secrete chemokine and cytokine and are implicated in recruiting immune cells in response to dysfunctions due to different factors (e.g., UV, heat, pathological and physiological processes) (Swerlick and Lawley, 1993).
Specific inflammatory mediators are described as endothelium activators like IL-1β or TNF-α, permitting vessels hyper-permeability allowing leucocyte infiltration. These vascular modulations are involved in skin diseases linked to a chronic inflammation (McGee et al., 1995; Huggenberger and Detmar, 2011). In vitro, TNF-α, IL-1β, and IFN-γ may alter vascular processes and cells behaviors, inducing inflammation stress. A mix of these cytokines induces synergistic effect producing inflammatory responses, which could induce at low concentration expression of chemokine like CCL2 or RANTES implicated in vessels permeability on endothelial cells (Goebeler et al., 1997).
Thus, inflammation is a common condition that affects the vasculature, but is also a skin feature that occurs in long-term pathologies, as well as in short-term conditions at high or low-grade level (Huggenberger and Detmar, 2011). Dermal fibroblasts are the key cells to synthetize the ECM composed by many components including collagen and ELN fibers. Fibroblasts are also known to stimulate vascular inflammation through cytokines and chemokines production (Singer and Clark, 1999), leading to endothelial activation and leukocytes attraction (Buckley et al., 2001; Enzerink and Vaheri, 2011).
Interestingly, the impact of microvascular endothelial cells microenvironment on skin integrity in a low-grade inflammatory context is poorly understood. The aim of the present study was to evaluate the impact of the microenvironment produced by dermal microvascular endothelial cells, secondary to a pro-inflammatory challenge, on 2D culture models using dermal fibroblast from healthy donors. We hypothesized that specific endothelial low grade inflammation could change fibroblasts phenotype and be involved in ECM modification and skin alteration. We validated this hypothesis in 2D culture model and then confirmed these results in a 3D full-thickness reconstructed human skin.
Materials and Methods
Cell Culture
Human dermal microvascular endothelial cells (HDMEC, Promocell Heidelberg, Germany) were cultured in Endothelial cell medium from Promocell composed by DMEM containing heparin 90 μg/ml, hydrocortisone 1 μg/ml supplemented with 2% Fetal Bovine Serum (FBS), 0.4% Endothelial Cell Growth Supplement (Promocell). Complete medium was supplemented with 1% penicillin/streptomycin (Gibco, Life Technologies, Carlsbad, CA, United States) at 37°c and 5% CO2. Human dermal fibroblasts were kindly provided by the Cell and Tissue Bank (Hôpital Edouard-Herriot, Lyon, France). Normal abdominal human skin tissue explants were obtained from the surgical discard of anonymous healthy patients with informed consent of adult donors in accordance with ethical guidelines (French Bioethics law of 2004) and declared to the French research ministry (Declaration no. DC-2008-162 delivered to the Cell and Tissue Bank of Hospices Civils de Lyon). Exclusion criteria were related to positive test result for hepatitis B or C, or HIV as well as obesity history. Human dermal fibroblasts were cultured in a medium consisted in a ratio 1 :1 of Dulbecco’s Modified Eagle’s Medium with Glutamax and F-12 medium (Invitrogen, Cergy Pontoise, France) supplemented with 10% FBS (Sigma-Aldrich, Saint-Quentin-Fallavier, France) and 1% penicillin/streptomycin (Gibco Life Technologies). HDMEC were used below 10 population doubling level and dermal fibroblasts below 20.
Antibodies and Reagents
The following antibodies were used: Rabbit polyclonal anti-collagen I (Novotec, Bron, France), Rabbit polyclonal anti-elastin antibody (Abcam, Cambridge, Royaume-Uni), Rabbit monoclonal anti-pro-LOX antibody (D8F2K, Cell Signaling), Rabbit polyclonal anti-β-actin antibody (ab8227, Abcam Cambridge, Royaume-Uni). As secondary antibody, Goat anti-rabbit IgG Alexa Fluor 488 (Life Technologies) was used for immunofluorescence and Goat anti-rabbit IgG, HRP-linked antibody for western blot (Cell signaling). Nuclear counterstaining using DAPI was purchased from Sigma-Aldrich. rhIFN-γ (c-60724), rhTNF-α (c-63719), and IL-1β (c-61120) were purchased from Promokine (Heidelberg, Germany).
Inflammatory Conditioned Medium From HDMEC
To produce a pro-inflammatory CM from HDMEC, cells were seeded in a 75 cm2 flask (4 × 105 cells/flask) 24 h before stimulation. HDMEC were stimulated with 0 IU/mL as control, 10 IU/mL or 100 IU/mL (for 3D skin models experiments) of a mix of cytokines composed by rhIFN-γ, rhTNF-α, and rhIL-1β during 24 h at 37°C 5% CO2 (CMØ, control; CM10, conditioned medium produce with 10 IU/mL cytokines mix; CM100, conditioned medium produce with 100 IU/mL cytokines mix). Conditioned media were then collected and centrifuged at 10000 ×g for 10 min at 4°C. Supernatant of each stimulation experiment was then collected and stored at -80°C until used.
Effect of the Conditioned Medium on Human Dermal Fibroblasts
Human dermal fibroblasts extracted from abdominal skin biopsies from adult donors were seeded in 6 well plates (200 000 cells/well). 24 h after cell adhesion, fibroblasts medium was changed by endothelial medium during 24 h. Dermal fibroblasts were then washed twice with PBS and stimulated with the different CM obtained (CMØ or CM10) during 48 h at 37°C 5% CO2. Results were obtained from 3 independent experiments from 3 donors of fibroblasts.
Effect of the Conditioned Medium on 3D Human Full Thickness Skin
Full-thickness human 3D skin models (from Lab Skin Creations, Lyon, France) were cultured in 6 well plates using an insert membrane of 0.4 μm of pore size (Millicell, Burlington, United States). Reconstructed skins were cultured in skin medium provided by Lab Skin Creations. 24 h before stimulation, skins were cultured in endothelial cells medium and then stimulated with the different CMs (CMØ or CM100). From preliminary tests it has been established on human biopsies that CM100 and 5 days of stimulation were necessary to observe modifications on skin integrity in presence of CM. 3D Skin models were then stimulated with CM100 during 5 days at 37°C under 5% of CO2. Results were obtained from 7 independent experiments using 3D skin models.
RNA Isolation and Real-Time Quantitative PCR
Total RNA was isolated using RNeasy Kit for 2D in vitro models and RNeasy Fibrous Tissue Mini Kit for 3D skin models (Qiagen, Courtaboeuf, France) according to the manufacturer’s instructions. 500 ng of total RNA was reverse-transcribed into cDNA using PrimeScriptTM RT reagent kit (Takara, Shiga, Japan) and analyzed by Real-Time QPCR using SYBR® Premix Ex Taq II (Takara) on an AriaMx Realtime PCR system (Agilent Genomics, Santa Clara, CA, United States). All primer (Supplementary Table S1) were provided by Eurogentec. Results were normalized to the GAPDH expression level for HDMEC, and dermal fibroblast samples.
Results were obtained from 3 independent experiments and 3 donors of fibroblasts have been used for 2D experiments. Relative quantification was calculated using the 2ΔΔCt quantification method. The primers are listed in the Supplementary Table S1.
Western Blotting
Dermal fibroblasts were lysed in RIPA buffer from Sigma and supplemented with protease and phosphatase inhibitor at 4°C. Cells lysate was centrifuged 10 min at 10000 g and 4°C. Supernatant were collected. Proteins were denatured 5 min at 95°C, separated on 10% polyacrylamide gel according to protein size and transferred to polyvinylidene difluoride (PVDF) membranes (Merck Millipore, Ref. IPVH00010). Membranes were blocked with 1× tris–buffered saline (TBS) 0.1% Tween and 5% non-fat milk for 1 h and blotted at 4°C overnight with primary antibodies diluted as recommended by the manufacturers in 1× TBS, 0.1% Tween and 5% non-fat milk. Membranes were incubated for 1 h at room temperature with secondary antibodies diluted as recommended by the manufacturers in 1× TBS, 0.1% Tween. Then membranes were incubated 5 min with ECL (Thermo Fisher, Ref. 34080) and were revealed by chemiluminescence. Western blot results were analyzed using GelAnalyzer software.
Immunofluorescence Microscopy
For immunofluorescence, conditioned media (CMØ or CM10) were added when fibroblasts were at confluence on coverslip. Eight-day post confluence, cells were fixed in cold methanol (-20°C) for 20 min to investigate ELN network or were fixed in PBS supplemented with 4% paraformaldehyde at room temperature for 10 min to study collagen I network. Cells were incubated overnight at 4°C with a rabbit polyclonal anti-collagen I (Novotec, Bron, France) antibody diluted at 1:400 in PBS – goat serum 0.1% or a rabbit polyclonal anti-elastin antibody (Abcam, Cambridge, Royaume-Uni) diluted at 1:250 in PBS – goat serum 0.1%, This was followed by a second incubation with a goat anti-rabbit IgG Alexa Fluor 488 (Life Technologies) diluted in 1:1000 PBS – goat serum 0.1% (Life Technologies) at room temperature for 1 h. Nuclear counterstaining using DAPI (Sigma) was carried out with a dilution 1:500 in PBS – goat serum 0.1%. Negative controls were performed by omitting the primary antibody. Image acquisition was carried out using a Nikon microscope (Nikon TE300, Champigny-sur-Marne, France) with a coolsnap fx CCD camera (Photometrics, Tucson, AZ, United States) with MetaVue software (Universal Imaging Corporation, West Chester, PA, United States). Image analysis and semi-quantification were performed using ImageJ software. Results were obtained from 4 independent experiments from 3 donors of fibroblasts. Two images were acquired per condition.
Luminex Assay
The determination of the amount of multiple cytokines in CMØ and CM10 was carried out using Human Magnetic Luminex Assay from R&D Systems (Minneapolis, MN, United States) according to the manufacturer’s instructions. Levels of CCL2 (MCP-1), IL-6, IL-8 (CXCL8), VEGF-A, IFN-γ, TNF-α, and IL-1β were analyzed using Bio-Plex MAGPIX Multiplex Reader and Bio-Plex Manager software from Bio-Rad (Bio-Rad Laboratory, Hercules, CA, United States).
Statistical Analysis
All statistical analyses were performed using Prism (version 7.0, GraphPad Software Inc.). All results are expressed as mean ± SD. Statistically significant differences were calculated by one-way analysis of variance (followed by Dunnett’s test post-test) or unpaired Student’s t-test. p < 0.05 was considered statistically significant. ∗P < 0.05, ∗∗P < 0.01, ∗∗∗P < 0.001, ∗∗∗∗P < 0.0001.
Results
HDMEC Activation and Secretion Under Low-Grade Inflammatory Stimulation
Human Dermal Endothelial Cells were treated with low-grade inflammatory mix (rhIFN-γ, rhTNF-α, and rhIL-1β) and we observed an upregulation of ICAM-1 and VCAM-1 (Figure 1A) demonstrating that the low-grade inflammatory mix activated the dermal microvascular endothelial cells, as confirmed by 2.5-fold increase in VEGF-A gene expression (Figure 1B).
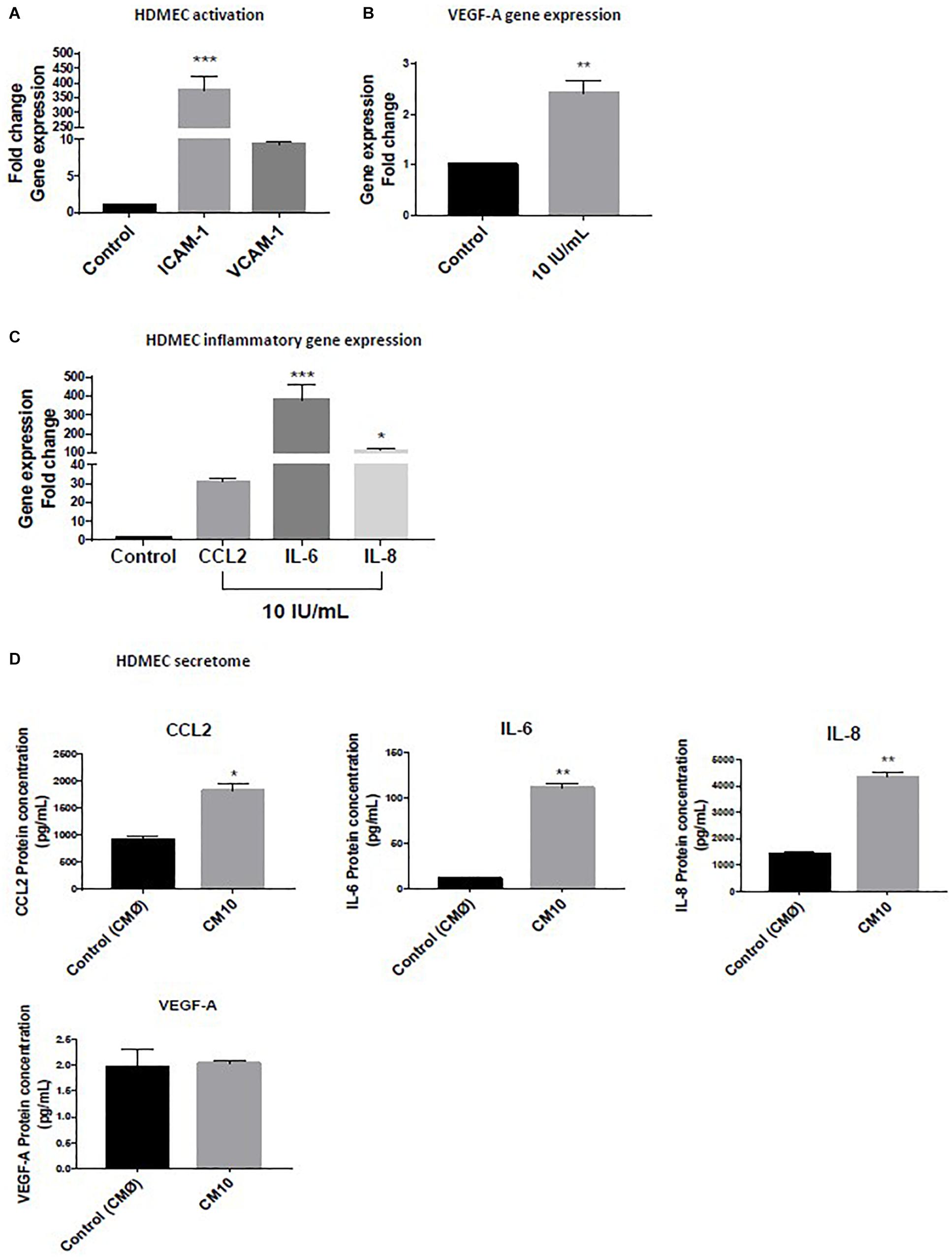
Figure 1. Effect of low grade inflammatory stimulation on HDMEC Gene expression of (A) markers of vascular activation of (B) VEGF-A (C) pro-inflammatory cytokines. (D) Expression of proteins secreted in the conditioned medium. Luminex assay for CCL2, IL-6, and IL-8 levels and VEGF-A protein expression. Gene expression was normalized with GAPDH. Data are representative of at least three independent experiments. Analysis of cytokine secretion was performed in duplicate for each sample using t-test. For vascular inflammation results were compared and analyzed to control condition using ANOVA Dunnett’s test. ∗P < 0.05, ∗∗P < 0.01, ∗∗∗P < 0.001.
We observed a significant HDMEC transcriptional activation of the pro-inflammatory gene expression markers, CCL2, IL-6, and IL-8 (Figure 1C) in response to the initial inflammatory cocktail that resulted of an increased in HDMEC protein secretion of CCL2, Il-6, and IL-8 in the CM10 compared to the control condition CMØ (Figure 1D). The protein fold increases were 1.5, 12, and 2.8, respectively (Figure 1D). In contrast, protein expression of TNF-α, IL-1β, and IFNγ were not detectable in CM10, revealing absence or non-significant levels of these cytokines in HDMEC secretome. VEGF-A protein expression was not significantly different between the two groups (Figure 1D).
Pro-inflammatory Endothelial Conditioned Medium Stimulates Further Fibroblast Inflammatory Gene Expression
The secretion of increased inflammatory cytokines released from HDMEC under low-grade inflammation could influence dermal fibroblasts function. To investigate this possibility in a cell culture system, dermal fibroblasts were stimulated with CMØ or CM10 for 48 h. It appeared that CCL2, IL-6, and IL-8 gene expression were significantly up-regulated in dermal fibroblasts in presence of CM10 compared to control condition CMØ (Figure 2A). In contrast, no significant differences were observed in pro-inflammatory NF-κB pathway, NF-κB sub-unit NFκB1 and RELA gene expression in fibroblasts challenged by CM10 compared to CMØ.
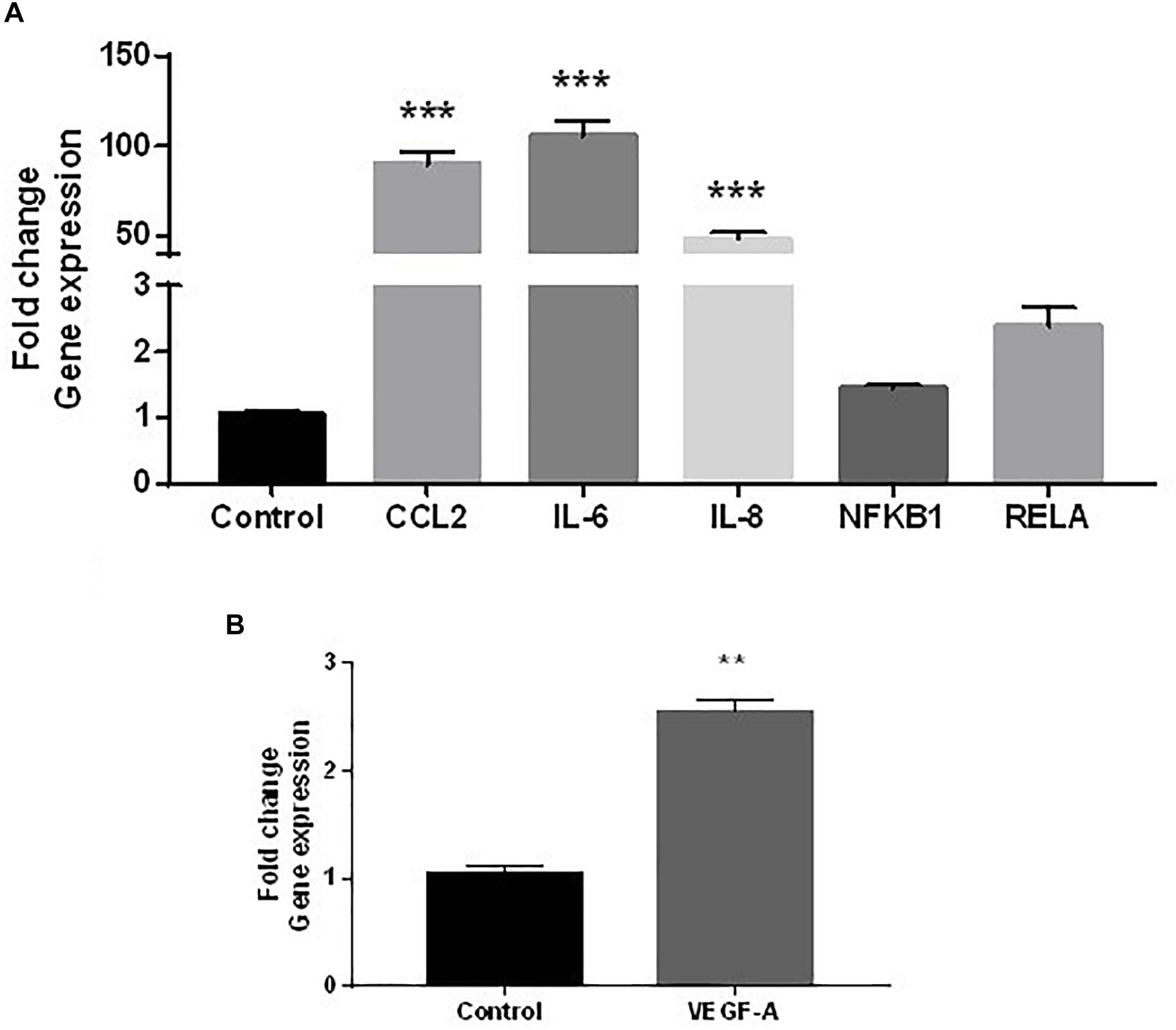
Figure 2. Effect of CM10 on fibroblast gene expression. RT-qPCR expression analysis of genes coding (A) CCL2, IL-6, and IL-8, NFκB1, RELA and (B) VEGF-A following CM10 stimulation versus control CMØ. Gene expression was normalized with GAPDH. (A) Results were compared and analyzed to control conditions using ANOVA Dunnett’s test, except for VEGF-A where gene expression data were analyzed using a t-test. ∗∗P < 0.01, ∗∗∗P < 0.001.
VEGF-A gene expression was also 2.5-fold increased by dermal fibroblasts under CM10 compared to CMØ (Figure 2B).
Pro-inflammatory Endothelial Conditioned Medium Altered ECM
In order to investigate collagen type I and ELN ECM formation in contact with CMØ or CM10, we used immunofluorescence to analyze their production within the ECM. We confirmed that dermal fibroblasts treated with CM10, 8-day post confluence, synthesized less collagen I than cells treated with CMØ (Figure 3A). Collagen I network appeared to be less organized in presence of CM10 compared to CMØ. Semi-quantification of collagen I labeling was significantly decreased in presence of CM10 compared to CMØ while ELN labeling semi-quantification tended to be reduced but did not reach significant differences (Figure 3B).
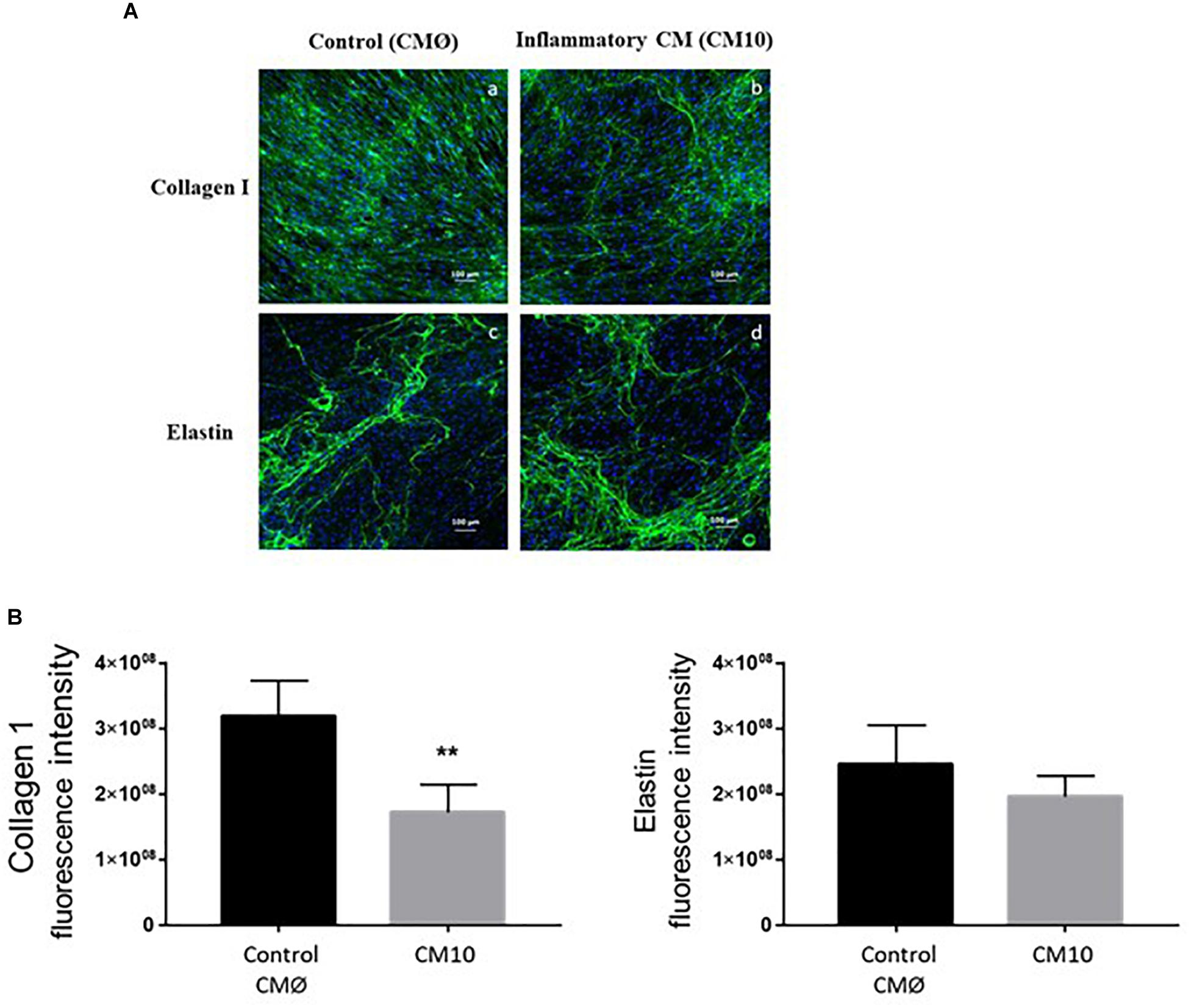
Figure 3. Effect of CM10 on ECM formation. (A) Using immunofluorescence assay for ECM formation, collagen I (a,b) and ELN (c,d) were analyzed 8-day post confluence following CM0 or CM10 stimulation. ECM components were stained with anti-collagen1 antibody (a,b) or anti-Elastin antibody (c,d) and fluorescent signals specific to the antibodies were visualized as green. (B) Semi-quantification of collagen 1 and ELN positive staining in each condition. Integrated density was used to quantify immunofluorescence. Nuclei were counter-stained with DAPI (blue). Scale bars indicate 100 μm. ∗∗p < 0.01 vs control.
We then analyzed the expression of genes encoding proteins involved in ECM rearrangement in fibroblasts treated with CM10 or CMØ (Figure 4A). MMP1 and MMP2 gene expression were significantly increased by CM10 while ELN gene expression was unchanged and COL1A1 gene expression was significantly reduced. In addition, collagen I protein expression was reduced in presence of CM10 compared to CMØ (Figure 4B) confirming the reduction of the collagen I network as observed by immunofluorescence (Figure 3A). Pro-LOX protein expression, the pro-enzyme form of LOX responsible for collagen and/or ELN cross-link, was also reduced by CM10 compared to CMØ (Figure 4B).
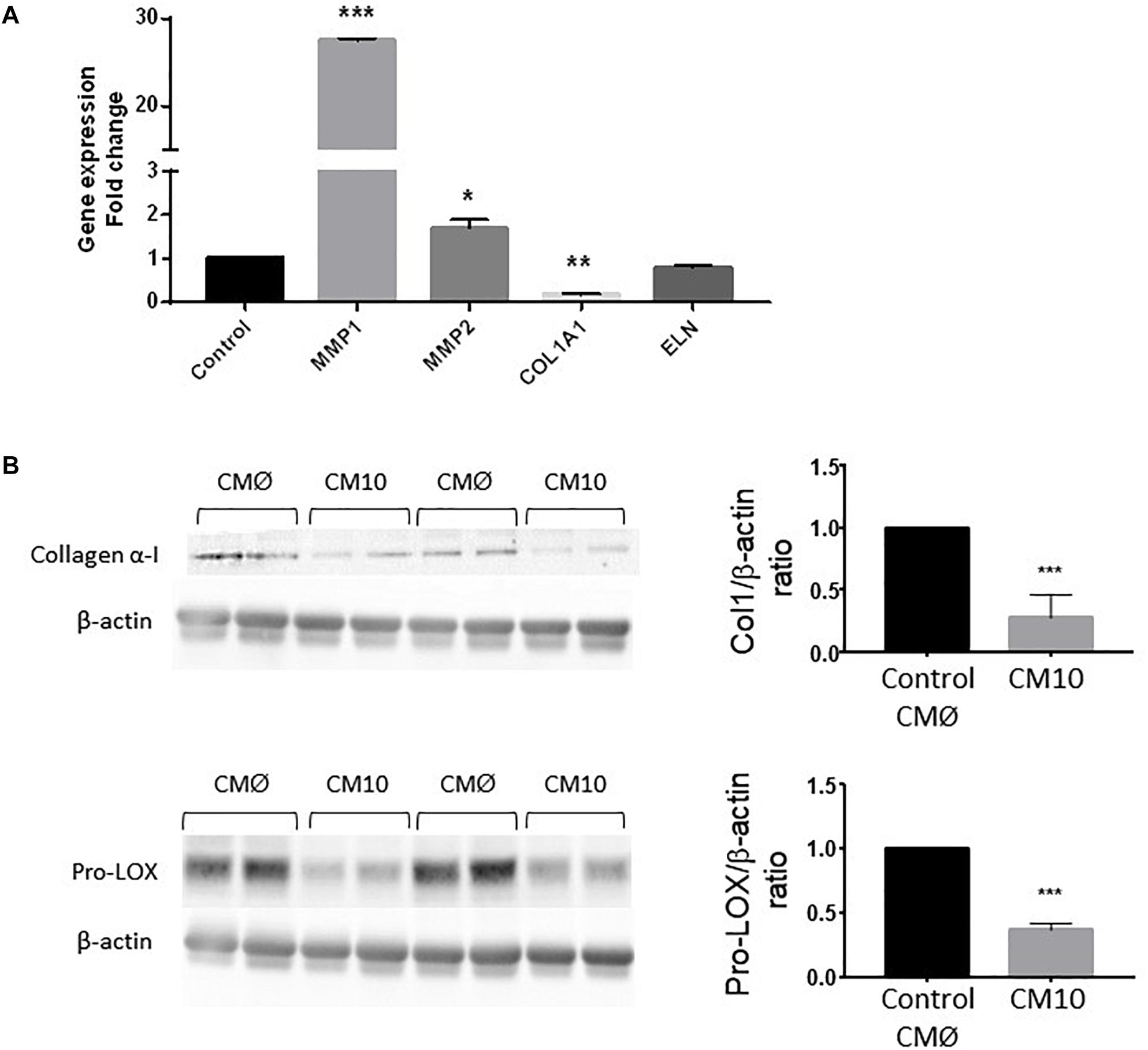
Figure 4. Effect of CM10 on fibroblast ECM gene expression. (A) RT-qPCR expression analysis of genes encoding MMP1, COL1A1, MMP2, and ELN and (B) collagen 1 and pro-LOX protein expression in dermal fibroblasts ECM cultured following CM10 stimulation versus control. Gene expression was normalized with GAPDH. Protein expression was normalized using β-actin and quantification was performed using Gel analyzer software. Results were compared and analyzed to control conditions using ANOVA Dunnett’s test, ∗P < 0.05, ∗∗P < 0.01, ∗∗∗P < 0.001.
Effect of CM100 on 3D Human Full Thickness Skin Gene Expression
To go further into the comprehension of the effect of vascular pro-inflammatory CM on ECM, we used 3D reconstructed skin including keratinocytes and fibroblasts that were challenged with CMØ or CM100 for 5 days. Gene expression of cellular senescence marker p16 and the stress marker p53 were both not different between CMØ and CM100 (Figure 5A).
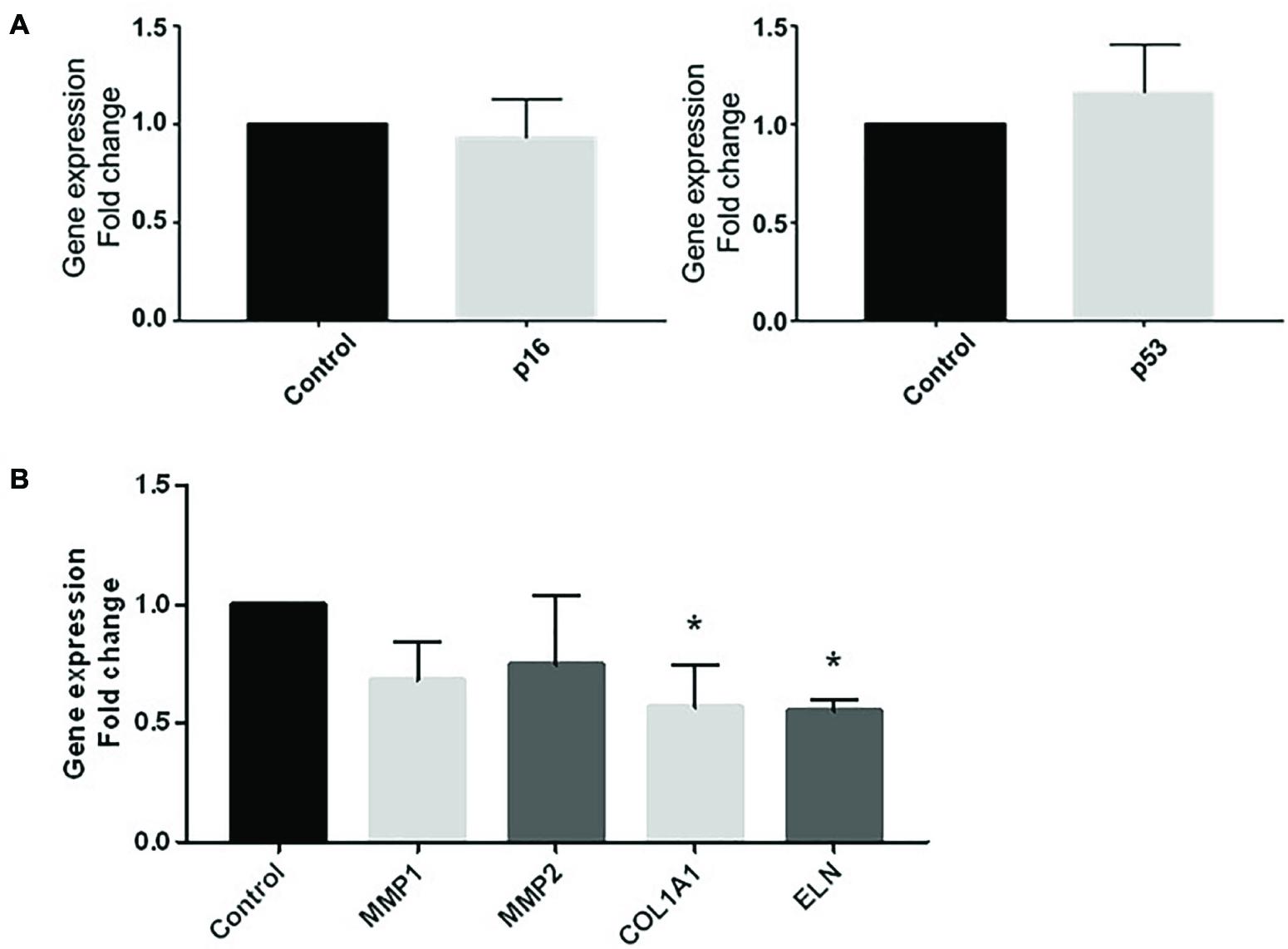
Figure 5. Effect of CM100 on 3D human full-thickness reconstructed skin. RT-qPCR expression analysis of (A) stress markers p16 and p53 and (B) ECM gene MMP1, MMP2, COL1A1, and ELN expression after 5-day stimulation of 3D skin models with CM100 stimulation versus control CMØ. Gene expression was normalized with GAPDH. Results were compared and analyzed to control condition using ANOVA Dunnett’s test, ∗P < 0.05.
In 3D skin model, COL1A1 and ELN gene expression were significantly downregulated in presence of CM100 (Figure 5B). Nevertheless, in this model there was no upregulation of MMPs in presence of CM100 compared to CMØ.
Discussion
In the present study, we showed that the activated endothelial cell secretome released following pro-inflammatory stress promoted a pro-inflammatory fibroblast phenotype that could be self-sustained without any immune cells. Indeed, CCL2, IL-6, and IL-8 released in the endothelial secretome following IFNγ, TNFα, and IL-1β stress, are responsible for increasing CCL2, IL-6, and IL-8 fibroblast gene expression generating a low-grade inflammatory fibroblast environment. The pro-inflammatory cocktail could thus maintain a perpetual fibroblast inflammatory phenotype that could be responsible for an exacerbation in ECM degradation with an increase in MMP1 gene expression and unstable collagen 1 network.
Dermal microcirculation is the conduit in which the stressors will affect the surrounding environment. In homeostasis, fibroblasts and endothelial cells are both involved in the angiogenic process and in ECM formation (Slany et al., 2016). In a pro-inflammatory microenvironment, dermal microvascular endothelial cells play an important role in homeostasis and in inflammatory responses. HDMECs synthesize and secrete chemokine and cytokines which are implicated in recruiting immune cells in response to inflammatory disorders (Qazi et al., 2011). This inside-out inflammatory response has been supplemented with an outside-in signaling involving activated pro-inflammatory fibroblasts interacting with immune cells to direct endothelial activation (Enzerink and Vaheri, 2011). In this context, fibroblasts play a critical role in modulating immune cells such as leukocytes and could be responsible for the establishment of chronic inflammation (Buckley et al., 2001).
In the present study, we focused on activated endothelial cell secretion without any immune cell interaction that could impact dermal cell behaviors such as dermal fibroblast and the ECM formation. To play their roles, endothelial cells have to be activated in order to interact with their environment. In an inflammatory context, endothelial cells release different inflammatory factors like IL-1β and TNF-α or by an upregulation of leucocyte adhesion molecule as ICAM-1 and VCAM-1, which are two markers used to confirm the endothelial cells activation (Detmar et al., 1992; Videm and Albrigtsen, 2008). Endothelial cell activation permits the interaction with the environment, playing a key role in inflammatory development (Swerlick et al., 1992). In our models, we showed that HDMEC were activated in presence of low-grade inflammation with an upregulation of ICAM-1 and VCAM-1. These activation are responsible for the loss of vascular integrity allowing secretion of cytokines and chemokines that could interact with tissues like skin structure (Adams and Shaw, 1994). HDMECs are described as critical elements for releasing proteins like VEGF-A or other growth factors. Moreover, HDMECs are also implicated in the cutaneous inflammation, secreting cytokines and chemokines that could directly interact with components of the dermis (Swerlick and Lawley, 1993). It appeared that low-grade inflammatory mix (CM10) composed of 10 IU/mL of IL-1β, TNF-α, and IFN-γ induced upregulation of cytokines and chemokines on dermal microvascular endothelial cells as CCL2, IL-6, and IL-8 which are known to be chemoattractant for T cells, dendritic cells and monocytes. The addition of the activated endothelial secretome onto dermal fibroblasts induced a switch from normal to pro-inflammatory phenotype with an upregulation of CCL2, IL-6, and IL-8 gene expression perpetuating fibroblasts pro-inflammatory environment.
The crosstalk between endothelial cells and fibroblasts has been studied through fibroblasts influencing endothelial cells, since fibroblasts provide ECM support as well as the production of several molecules like VEGF for angiogenesis (Villaschi and Nicosia, 1994; Martin et al., 2001; Berthod et al., 2006; Stunova and Vistejnova, 2018). In addition, (Villaschi and Nicosia, 1994) suggested that endothelial cells might also affect fibroblasts since cytokines, chemokines and other molecules present in the microenvironment could affect skin integrity altering ECM components (Van Linthout et al., 2014). In skin aging, it has been shown that IL-8 and IL-6 are clearly linked to MMPs modulation and COL1A1 gene and protein expression (Dasu et al., 2003; Kolar et al., 2012; Quan and Fisher, 2015). We showed that the low-grade inflammatory microenvironment produced by activated endothelial cells induced a strong up-regulation of MMP1 in dermal fibroblasts suggesting collagen I degradation associated to a reduction in collagen I synthesis due to COL1A1 gene downregulation. In addition, the significant decrease in Pro-LOX protein expression strengthens the reduction of collagen cross-linking. Indeed, the primary function of the LOX family is the covalent cross-linking of collagens and/or ELN into a stable, insoluble ECM, which is important for the maintenance of tensile strength and structural integrity of most tissues (Noblesse et al., 2004). The collagenous matrix deposited during LOX reduction should be more easily degradable (Trackman, 2005). In contrast, ELN gene expression was not modulated by the vascular pro-inflammatory microenvironment. Only a few studies have evaluated skin ELN production in an inflammatory context apart from skin aging (Ganceviciene et al., 2012) and wound healing (Almine et al., 2013) studies. We confirmed the 2D results with a downregulation of COL1A1 and the 3D skin model revealed a significant reduction in ELN gene expression, suggesting that specific inflammatory vascular environment could alter skin integrity, inducing modification of gene implicated in ECM components. These results show the significant role of skin microvessels on skin integrity, which may vary depending on inflammatory conditions.
Indeed, the loss of vascular integrity is involved in the development of skin or autoimmune diseases, inducing inflammatory processes as chronic inflammatory conditions like psoriasis or lupus (Heidenreich et al., 2009; Achtman and Werth, 2015). Endothelial cells play a significant role in inflammatory responses and link to pro angiogenic and pro-inflammatory protein secretion as described. Among these pro-inflammatory proteins, VEGF-A is described as a predominant protein implicated in immune response. In inflammatory conditions, it appeared that VEGF is not only secreted by endothelial cells but also by macrophages, T cells and fibroblasts, which are involved in angiogenesis and inflammatory processes (Mor et al., 2004; Enzerink and Vaheri, 2011). In our study, it appeared that HDMEC up-regulated VEGF-A gene expression, but no differences were observed in VEGF-A protein secretion in the CM, after 24 h of stimulation with the low-grade inflammatory cytokines mix. This result is probably linked to the short biological half-life, and the rapid metabolic degradation of VEGF-A in the medium (Lee et al., 2000).
To conclude, this study showed that the secretory microenvironment related to endothelial inflammation could trigger a perpetual fibroblast inflammatory phenotype that could be responsible for an exacerbation in ECM degradation with an increase in MMP1 and unstable collagen 1 network. In contact with endothelial inflammatory secretion, and no immune cells, it appears that fibroblasts switch from normal to pro-inflammatory phenotype and are less involved in ECM synthesis.
Author Contributions
LL, GA, JL, GL, and DS-R conceived and designed the study. BS and JD acquired, analyzed, and interpreted the data. BS, LL, JD, GA, JL, GL, and DS-R critically revised the manuscript for important intellectual content. DS-R obtained funding. BS, LL, JD, GA, JL, GL, and DS-R provided the administrative, technical, or material support. DS-R is the guarantor of this work and, as such, had full access to all of the data in the study and takes responsibility for the integrity of the data and the accuracy of the data analysis.
Funding
This work was supported by a grant from Infinitus and bike Guangzhou (China).
Conflict of Interest Statement
The authors declare that the research was conducted in the absence of any commercial or financial relationships that could be construed as a potential conflict of interest.
Acknowledgments
We are very grateful to Song Ximing and Li Jialin for their help in work management.
Supplementary Material
The Supplementary Material for this article can be found online at: https://www.frontiersin.org/articles/10.3389/fcell.2019.00044/full#supplementary-material
TABLE S1 | Details of specific primers and targets used in real-time qPCR experiments.
Abbreviations
CCL2, chemokine ligand 2; CM, conditioned medium; COL1A1, collagen 1 alpha 1; ECM, extracellular matrix; ELN, elastin; HDMEC, Human Dermal Microvascular Endothelial Cell; ICAM-1, InterCellular Adhesion Molecule 1; IFN-γ, Interferon gamma; IL-1β, Interleukin 1 Beta; IL-6, Interleukin 6; IL-8, Interleukin 8; LOX, Lysyl Oxydase; MMP, Matrix metalloproteinase; pro-LOX, pro-Lysyl Oxydase; TNF-α, Tumor Necrosis Factor alpha; VCAM-1, Vascular cell adhesion protein 1; VEGF-A, Vascular endothelial growth factor A.
References
Achtman, J. C., and Werth, V. P. (2015). Pathophysiology of cutaneous lupus erythematosus. Arthritis Res. Ther. 17:182. doi: 10.1186/s13075-015-0706-2
Adams, D. H., and Shaw, S. (1994). Leucocyte-endothelial interactions and regulation of leucocyte migration. Lancet 343, 831–836. doi: 10.1016/S0140-6736(94)92029-X
Almine, J. F., Wise, S. G., Hiob, M., Singh, N. K., Tiwari, K. K., Vali, S., et al. (2013). Elastin sequences trigger transient proinflammatory responses by human dermal fibroblasts. FASEB J. 27, 3455–3465. doi: 10.1096/fj.13-231787
Berthod, F., Germain, L., Tremblay, N., and Auger, F. A. (2006). Extracellular matrix deposition by fibroblasts is necessary to promote capillary-like tube formation in vitro. J. Cell Physiol. 207, 491–498. doi: 10.1002/jcp.20584
Buckley, C. D., Pilling, D., Lord, J. M., Akbar, A. N., Scheel-Toellner, D., and Salmon, M. (2001). Fibroblasts regulate the switch from acute resolving to chronic persistent inflammation. Trends Immunol. 22, 199–204. doi: 10.1016/S1471-4906(01)01863-4
Charkoudian, N. (2003). Skin blood flow in adult human thermoregulation: how it works, when it does not, and why. Mayo Clin. Proc. 78, 603–612. doi: 10.4065/78.5.603
Dasu, M. R., Barrow, R. E., Spies, M., and Herndon, D. N. (2003). Matrix metalloproteinase expression in cytokine stimulated human dermal fibroblasts. Burns 29, 527–531. doi: 10.1016/S0305-4179(03)00154-2
Detmar, M., Tenorio, S., Hettmannsperger, U., Ruszczak, Z., and Orfanos, C. E. (1992). Cytokine regulation of proliferation and ICAM-1 expression of human dermal microvascular endothelial cells in vitro. J. Invest. Dermatol. 98, 147–153. doi: 10.1111/1523-1747.ep12555746
Enzerink, A., and Vaheri, A. (2011). Fibroblast activation in vascular inflammation. J. Thromb Haemost. 9, 619–626. doi: 10.1111/j.1538-7836.2011.04209.x
Ganceviciene, R., Liakou, A. I., Theodoridis, A., Makrantonaki, E., and Zouboulis, C. C. (2012). Skin anti-aging strategies. Dermatoendocrinology 4, 308–319. doi: 10.4161/derm.22804
Goebeler, M., Yoshimura, T., Toksoy, A., Ritter, U., Brocker, E. B., and Gillitzer, R. (1997). The chemokine repertoire of human dermal microvascular endothelial cells and its regulation by inflammatory cytokines. J. Invest. Dermatol. 108, 445–451. doi: 10.1111/1523-1747.ep12289711
Gutterman, D. D., Chabowski, D. S., Kadlec, A. O., Durand, M. J., Freed, J. K., Ait-Aissa, K., et al. (2016). The human microcirculation: regulation of flow and beyond. Circ. Res. 118, 157–172. doi: 10.1161/CIRCRESAHA.115.305364
Heidenreich, R., Rocken, M., and Ghoreschi, K. (2009). Angiogenesis drives psoriasis pathogenesis. Int. J. Exp. Pathol. 90, 232–248. doi: 10.1111/j.1365-2613.2009.00669.x
Huggenberger, R., and Detmar, M. (2011). The cutaneous vascular system in chronic skin inflammation. J. Invest. Dermatol. Symp. Proc. 15, 24–32. doi: 10.1038/jidsymp.2011.5
Kolar, M., Szabo, P., Dvorankova, B., Lacina, L., Gabius, H. J., Strnad, H., et al. (2012). Upregulation of IL-6, IL-8 and CXCL-1 production in dermal fibroblasts by normal/malignant epithelial cells in vitro: immunohistochemical and transcriptomic analyses. Biol. Cell 104, 738–751. doi: 10.1111/boc.201200018
Lee, R. J., Springer, M. L., Blanco-Bose, W. E., Shaw, R., Ursell, P. C., and Blau, H. M. (2000). VEGF gene delivery to myocardium: deleterious effects of unregulated expression. Circulation 102, 898–901. doi: 10.1161/01.CIR.102.8.898
Martin, T. A., Harding, K., and Jiang, W. G. (2001). Matrix-bound fibroblasts regulate angiogenesis by modulation of VE-cadherin. Eur. J. Clin. Invest. 31, 931–938. doi: 10.1046/j.1365-2362.2001.00914.x
McGee, D. W., Bamberg, T., Vitkus, S. J., and McGhee, J. R. (1995). A synergistic relationship between TNF-alpha, IL-1 beta, and TGF-beta 1 on IL-6 secretion by the IEC-6 intestinal epithelial cell line. Immunology 86, 6–11.
Mor, F., Quintana, F. J., and Cohen, I. R. (2004). Angiogenesis-inflammation cross-talk: vascular endothelial growth factor is secreted by activated T cells and induces Th1 polarization. J. Immunol. 172, 4618–4623. doi: 10.4049/jimmunol.172.7.4618
Noblesse, E., Cenizo, V., Bouez, C., Borel, A., Gleyzal, C., Peyrol, S., et al. (2004). Lysyl oxidase-like and lysyl oxidase are present in the dermis and epidermis of a skin equivalent and in human skin and are associated to elastic fibers. J. Invest. Dermatol. 122, 621–630. doi: 10.1111/j.0022-202X.2004.22330.x
Qazi, B. S., Tang, K., and Qazi, A. (2011). Recent advances in underlying pathologies provide insight into interleukin-8 expression-mediated inflammation and angiogenesis. Int. J. Inflam. 2011:908468. doi: 10.4061/2011/908468
Quan, T., and Fisher, G. J. (2015). Role of age-associated alterations of the dermal extracellular matrix microenvironment in human skin aging: a mini-review. Gerontology 61, 427–434. doi: 10.1159/000371708
Singer, A. J., and Clark, R. A. (1999). Cutaneous wound healing. N. Engl. J. Med. 341, 738–746. doi: 10.1056/NEJM199909023411006
Slany, A., Bileck, A., Kreutz, D., Mayer, R. L., Muqaku, B., and Gerner, C. (2016). Contribution of human fibroblasts and endothelial cells to the hallmarks of inflammation as determined by proteome profiling. Mol. Cell Proteomics 15, 1982–1997. doi: 10.1074/mcp.M116.058099
Stunova, A., and Vistejnova, L. (2018). Dermal fibroblasts-A heterogeneous population with regulatory function in wound healing. Cytokine Growth Factor Rev. 39, 137–150. doi: 10.1016/j.cytogfr.2018.01.003
Swerlick, R. A., and Lawley, T. J. (1993). Role of microvascular endothelial cells in inflammation. J. Invest. Dermatol. 100, 111S–115S. doi: 10.1038/jid.1993.33
Swerlick, R. A., Lee, K. H., Li, L. J., Sepp, N. T., Caughman, S. W., and Lawley, T. J. (1992). Regulation of vascular cell adhesion molecule 1 on human dermal microvascular endothelial cells. J. Immunol. 149, 698–705.
Trackman, P. C. (2005). Diverse biological functions of extracellular collagen processing enzymes. J. Cell Biochem. 96, 927–937. doi: 10.1002/jcb.20605
Van Linthout, S., Miteva, K., and Tschope, C. (2014). Crosstalk between fibroblasts and inflammatory cells. Cardiovasc. Res. 102, 258–269. doi: 10.1093/cvr/cvu062
Videm, V., and Albrigtsen, M. (2008). Soluble ICAM-1 and VCAM-1 as markers of endothelial activation. Scand. J. Immunol. 67, 523–531. doi: 10.1111/j.1365-3083.2008.02029.x
Keywords: fibroblasts, endothelial cells, inflammation, extracellular matrix, collagen, elastin
Citation: Sanchez B, Li L, Dulong J, Aimond G, Lamartine J, Liu G and Sigaudo-Roussel D (2019) Impact of Human Dermal Microvascular Endothelial Cells on Primary Dermal Fibroblasts in Response to Inflammatory Stress. Front. Cell Dev. Biol. 7:44. doi: 10.3389/fcell.2019.00044
Received: 04 November 2018; Accepted: 13 March 2019;
Published: 03 April 2019.
Edited by:
Venkaiah Betapudi, U.S. Army Medical Research Institute of Chemical Defense, United StatesReviewed by:
Elia Ranzato, University of Eastern Piedmont, ItalyAnshu Malhotra, Emory University, United States
Lokesh Bhatt, Dr. Bhanuben Nanavati College of Pharmacy, India
Copyright © 2019 Sanchez, Li, Dulong, Aimond, Lamartine, Liu and Sigaudo-Roussel. This is an open-access article distributed under the terms of the Creative Commons Attribution License (CC BY). The use, distribution or reproduction in other forums is permitted, provided the original author(s) and the copyright owner(s) are credited and that the original publication in this journal is cited, in accordance with accepted academic practice. No use, distribution or reproduction is permitted which does not comply with these terms.
*Correspondence: Dominique Sigaudo-Roussel, dominique.sigaudo-roussel@univ-lyon1.fr
†Co-first authors
‡Co-last authors