- 1Department of Biomedicine, Aarhus University, Aarhus, Denmark
- 2The Fertility Clinic, Horsens Hospital, Horsens, Denmark
- 3The Fertility Clinic, Aarhus University Hospital, Aarhus, Denmark
- 4Department of Clinical Medicine, Aarhus University, Aarhus, Denmark
- 5Department of Clinical Genetics, Aarhus University Hospital, Aarhus, Denmark
Bidirectional cross talk between granulosa cells and oocytes is known to be important in all stages of mammalian follicular development. Insulin-like growth factor (IGF) signaling is a prominent candidate to be involved in the activation of primordial follicles, and may be be connected to androgen-signaling. In this study, we interrogated transcriptome dynamics in granulosa cells isolated from human primordial and primary follicles to reveal information of growth factors and androgens involved in the physiology of ovarian follicular activation. Toward this, a transcriptome comparison study on primordial follicles (n = 539 follicles) and primary follicles (n = 261 follicles) donated by three women having ovarian tissue cryopreserved before chemotherapy was performed. The granulosa cell contribution in whole follicle isolates was extracted in silico. Modeling of complex biological systems was performed using IPA® software. We found the granulosa cell compartment of the human primordial and primary follicles to be extensively enriched in genes encoding IGF-related factors, and the Androgen Receptor (AR) enriched in granulosa cells of primordial follicles. Our study hints the possibility that primordial follicles may indeed be androgen responsive, and that the action of androgens represents a connection to the expression of key players in the IGF-signaling pathway including IGF1R, IGF2, and IGFBP3, and that this interaction could be important for early follicular activation. In line with this, several androgen-responsive genes were noted to be expressed in both oocytes and granulosa cells from human primordial and primary follicle. We present a detailed description of AR and IGF gene activities in the human granulosa cell compartment of primordial and primary follicles, suggesting that these cells may be or prepare to be responsive toward androgens and IGFs.
Introduction
Female fertility is dependent on continuous (monthly) activation of primordial follicles from the resting dormant follicle pool. A primordial follicle is made up by an oocyte surrounded partly by flattened granulosa cells. As the primordial follicle is activated, it transforms into a primary follicle, where the oocyte is now surrounded by a complete layer of cubical granulosa cells. The primordial to primary follicle transition is a delicate and tightly regulated balance between activating and inhibiting factors with contribution from numerous different molecular pathways, but the mechanisms are not yet completely understood (Wandji et al., 1996). Bidirectional communication between the somatic granulosa cells and the oocyte is a fundamental part of both dormancy and activation, as well as the establishment of an optimal intrafollicular microenvironment (Eppig, 2001).
The essential role of androgens in normal ovarian function has been recognized for decades. Androgens play a key role by being the precursor of estradiol, however increasing evidence emphasize that the direct actions of androgens likewise are central for normal follicular development (Lebbe and Woodruff, 2013). It has been suggested that androgen sensitivity in early pre-antral follicles influence the primordial follicle recruitment (Vendola et al., 1999a; Stubbs et al., 2005; Yang et al., 2010). In the intraovarian communication, androgens may play a necessary role (Lebbe and Woodruff, 2013; Gervasio et al., 2014). Androgens bind the androgen receptor (AR) and exert the classical androgen response by genomic induction of transcription of several genes including AR itself, creating an autocrine loop between ligand and receptor (Weil et al., 1998; Gelmann, 2002). Besides the direct genomic effects, androgen signaling is also known to induce rapid non-genomic pathways via cytosolic AR and the mitogen-activated protein kinase extracellular signal-related kinase (MAPK/ERK) pathway (Kousteni et al., 2001). A balanced androgen level is however crucial, and exposure to excess androgens is associated with ovarian dysfunction. A large group of women suffering from ovarian dysfunction is women suffering from polycystic ovary syndrome (PCOS), a common endocrine disorder, in which hyperandrogenism is a key feature (Franks, 1995). Morphologically, polycystic ovaries have an increased percentage of growing follicles and “stockpiling” of the primary follicles compared to controls (Webber et al., 2003; Maciel et al., 2004). Moreover, clinical evidence from women exposed to androgen excess due to congenital adrenal hyperplasia (Hague et al., 1990) or exogenous testosterone treatment in female-to-male transsexuals (Spinder et al., 1989; Becerra-Fernández et al., 2014) underlines this picture by increased prevalence of morphologically polycystic ovaries compared to controls. Polycystic ovaries are also a common trait in prenatally androgenized sheep, an animal model for PCOS (Padmanabhan and Veiga-Lopez, 2013). Lambs born to dihydrotestosterone (DHT) or testosterone treated ewes showed the same pattern of dysfunctional early follicular development as the women suffering from PCOS. These examples emphasize the involvement of androgens in the early follicular development. In this follicular-phase gonadotropins are not obligatory, while local growth factors may play an important role. Insulin-like growth factor (IGF) signaling is a prominent candidate and may be connected to androgen-signaling. In the human ovary both IGF1 and IGF2 act as ligands for IGF receptor 1 (IGF1R) (Willis et al., 1998), and IGF2 expression is more prominent compared to other species (Mazerbourg et al., 2003). Rhesus monkeys treated with testosterone showed an increase in the fraction of activated primary follicles and a 5-fold increase in IGF1R mRNA in the oocytes of primordial follicles, as well as an elevation in the intra-oocyte IGF1 signaling (Vendola et al., 1999a,b). Likewise, pigs treated with the anti-androgen Flutamide reduced the mRNA and protein expression of IGF1R in the oocyte, and showed delayed primordial follicle activation (Knapczyk-Stwora et al., 2013). In preantral follicles isolated from women suffering from PCOS, an enhanced expression of IGF1R mRNA and protein was noted compared to controls (Stubbs et al., 2013). In the IGF-signaling system IGF binding proteins (IGFBPs) have in recent years received increased attention, because of their potential active modulating role of IGF-bioavailability. This is in contrast to the conventional idea about IGFBPs as simple carrier proteins. The IGFBPs bind IGF and sequester the binding of IGF to its receptors. This modulating role might be important in terms of shifting from the dormant to the activated follicular stage (Hu et al., 2017).
We hypothesize that primordial follicles may be androgen responsive based on the presents of components supporting androgen signaling, and that the action of androgens could be closely connected to the expression of key players in the IGF-signaling such as IGF1R, IGF2, and IGFBP3.
Results
The global RNA transcriptomes representative for granulosa cells from primordial and primary follicles (http://users-birc.au.dk/biopv/published_data/ernst_et_al_GC_2017/) (Ernst et al., 2018) revealed 12.872 and 11.898 transcripts in granulosa cells from primordial and primary follicles, respectively (Ernst et al., 2018). The lists were further processed to exclude transcripts that were not consistently expressed in all patients and lists representative of stage-specific consistently expressed genes (SSCEGs) were generated. We applied this strict filter to only include analysis of genes that were consistent between patients included in this study, but certainly does not rule out that additional genes could be relevant. The SSCEGs analysis of the granulosa cell transcriptome revealed 1695 transcripts in primordial follicles and 815 transcripts in primary follicles. We further applied strict bioinformatic filters, and quality control to ensure specificity in output transcriptomes, and confirmed the presence of known granulosa cell-specific factors, as well as the absence of oocyte-specific factors. The SSCEGs lists in granulosa cells from primordial and primary follicles (Ernst et al., 2018) were used to extract genes differentially expressed genes (DEG) between the two cell populations.
The “Androgen Signaling” Pathway
We identified the most enriched and significant Canonical Pathways in granulosa cells from primordial and primary follicles (Ernst et al., 2018). To further analyze the ‘Androgen Signaling’ Pathways, we used the Ingenuity Pathway Analysis (IPA®) analysis software, which can be used to determine the most significant pathways and the genes allocated with each pathway. We found “Androgen Signaling” from Canonical Pathways significantly and differentially enriched in granulosa cells from both primordial and primary follicles (Table 1).
In granulosa cells from primordial follicles, the “Androgen Signaling” was highly enriched (p = 3,97E-02) with 15 genes assigned (POLR2D, GNA12, GNAQ, POLR2J, GTF2H2, MNAT1, GNAI2, GNG11, PRKCI, AR, PRKCH, GNG5, PRKD3, PRKCB, PRKCA), including the androgen receptor (AR) (Table 1; Figure 1A). The AR transcript is low-to-moderately expressed (mean FPKM value of 3.13) with a p-value of p = 0.012, indicating that the AR transcript is consistently expressed in the samples tested.
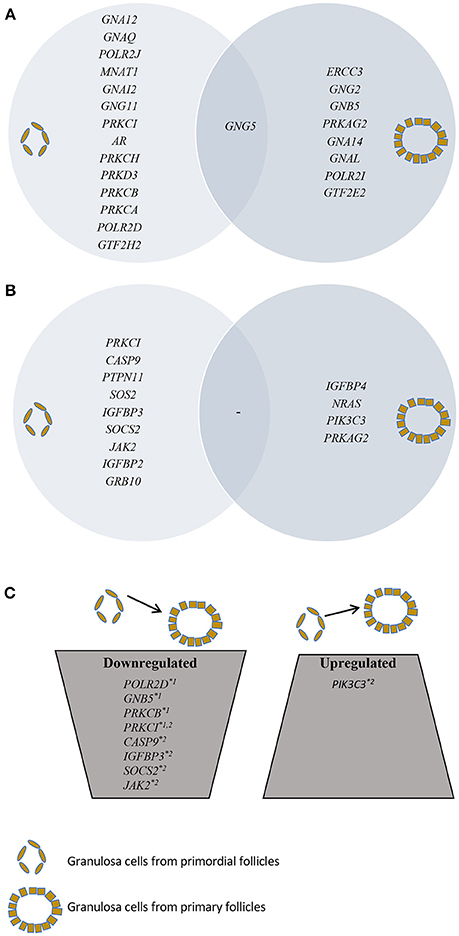
Figure 1. Presentation of SSCEGs and DEGs in granulosa cells from primordial and primary follicles. (A) Presentation of SSCEGs from the “AR Signaling” Pathway that are significantly expressed in granulosa cell from primordial and primordial follicles, as indicated, and the number in the middle representing SSCEG in both stages. (B) Presentation of SSCEGs from the “IGF1 Signaling” Pathway that are significantly expressed in granulosa cell from primordial and primary follicles, as indicated, and number in the middle representing SSCEG in both stages. (C) Presentation of DEGs from both “AR Signaling” (Noted *1) and “IGF1 Signaling” (Noted *2) pathways.
The “Androgen Signaling” was also enriched in granulosa cells from primary follicles (p = 3,42E-02) with nine genes (POLR2I, ERCC3, GNG2, GNB5, PRKAG2, GTF2E2, GNA14, GNG5, GNAL) assigned (Table 2; Figure 1A). In granulosa cells from primary follicles, the AR transcript levels is very low (mean FPKM value is 1.65) and was not consistently expressed in our samples (p = 0.39).
Differentially Expressed Genes in the “Androgen Signaling” Pathway
During the primordial to primary follicle transition, “Androgen Signaling” was non-significantly down-regulated (p = 5,65E-01). However, four genes (PRKC1, POLR2D, GNB5, and PRKCB) from the “Androgen Signaling” pathway were significantly down-regulated in the granulosa cells (Table 3; Figure 1C). As noted above, the AR transcript was down-regulated, however not significantly. The four genes were down-regulated by 2-fold change, indicating a rapid chance in expression during the primordial-to-primary transition. Interestingly, no genes from the androgen signaling pathway was significantly up-regulated during the primordial to primary follicle transition. The Androgen Signaling Pathway and the molecular network associated with this pathway is illustrated in Figure 2.
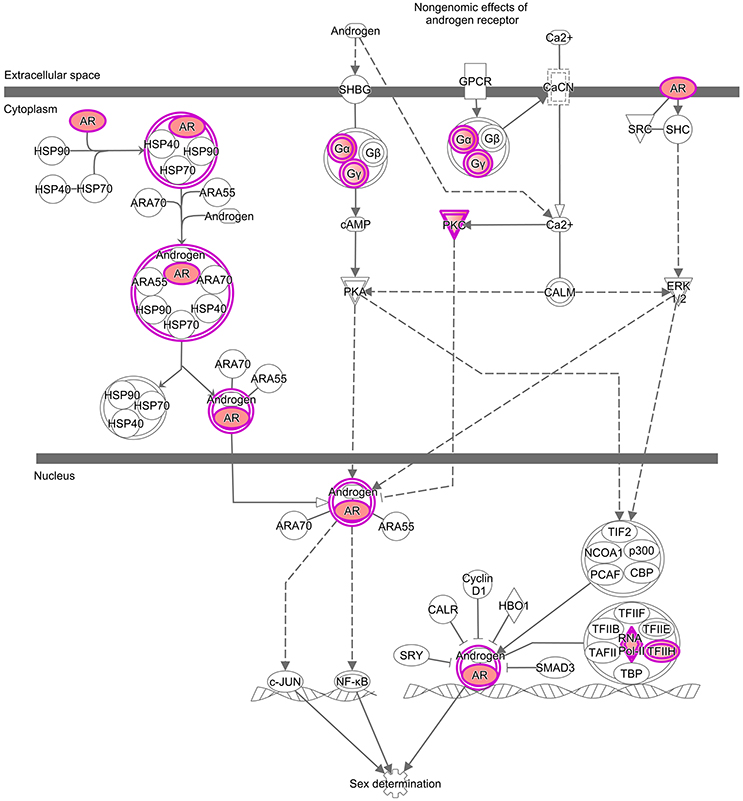
Figure 2. Enrichments analysis and IPA® pathways highlighting trends in granulosa cells from primordial and primary follicles, respectively. Pathway analysis (IPA®) of the “Androgen Signaling” pathway in granulosa cells of the primordial follicle. Color intensities are based on FPKM values where high significance is most intensive in color (red).
Differentially Expressed Genes in “IGF1 Signaling” Pathway
We interrogated the presence of IGF1, IGF2, IGF1R, and IGFR2 as well as the IGFBP1-6 transcripts in human oocytes and granulosa cells from primordial and primary follicles (Ernst et al., 2017, 2018) and found that some were significantly expressed, whilst close to be significant across the triplicates of samples (Table 4). It is noteworthy that the expression levels of IGF2 and IGF1 in granulosa cells from primordial cells to primary follicles decreased, while IGF1R and IGF2R expression levels remained. In oocytes from primordial and primary follicles, IGF2, and IGF1R transcripts increased, while IGF1 and IGF2R transcript were not significantly altered. Interestingly, expression of IGFBP1-6 varied significantly, with IGFBP-5 being highly expressed in all cells from both primordial and primary follicles. The IGFBP3 transcript appears to be upregulated in oocytes from primordial and primary follicles, but down regulated from granulosa cells from primordial cells to primary follicles.
Although the “IGF1 signaling” pathway was not significantly enriched in granulosa cells from primordial (p = 4,25E-01) or primary follicles (p = 5,56E-01), it was selected for further analysis, as IGFs are important for ovarian physiology (Adashi et al., 1985, 1991; Mondschein et al., 1989; Armstrong et al., 1996; Baumgarten et al., 2014). The IGF Signaling Pathway from the IPA® analysis contains factors directly associated with the IGF system (e.g., IGF1 and IGF2 and their respective receptors, IGF1 receptor and IGF2 receptor, as well as six binding proteins, IGFBP1-6) as well as the signal tranducting factors requires to conduct the IGF signaling (Laviola et al., 2007; Kuijjer et al., 2013; Lodhia et al., 2015), including PKC, Caspase9, JAK2, PIK3C3, and PRKCI. Consequently, it is noteworthy that many of the signal transducing components assigned to IGF Signaling Pathways are also found in other Signal transducing pathways, such as EGFR signaling. The “IGF-1 Signaling” pathway in the granulosa cells from primordial follicles contained nine genes (PRKCI, CASP9, PTPN11, SOS2, IGFBP3, SOCS2, JAK2, IGFBP2, GRB10) (Table 5; Figure 1B), and from primary follicles, we noted four genes (IGFBP4, NRAS, PIK3C3, PRKAG2 (Table 6; Figure 1B), suggesting a dynamic change of “IGF1 signaling”-related genes during the primordial to primary transition. During the primordial to primary follicle transition, the ‘IGF1 Signaling’ pathway was non-significantly downregulated (p = 5,64E-01). Interestingly, during the primordial to primary follicle transition, several members of the IGF1 Signaling family were significantly down- or up-regulated. Five genes (PRKC1, CASP9, IGFBP3, SOC2, JAK2) (Figure 1C) were significantly downregulated during the transition, and one gene (PIK3C3) (Figure 1C) was significantly up-regulated (Table 7).
IGF2 Protein Localizes to Human Oocytes and Granulosa Cells in Primordial and Primary Follicles
Transcriptomic data represents RNA profiling and thus, does not necessarily represents protein expression profiles. Human oocytes are loaded with maternal mRNA, of which many are packed into a protein complex preventing translation at this stage. The IGF2 protein was selected for immunofluorescent staining since the IGF2 gene was highly expressed in both oocytes and granulosa cells in primordial and primary follicles (Ernst et al., 2017, 2018) (Table 4). The immunofluorescence revealed a strong staining of the IGF2 protein in both oocytes and granulosa cells in primordial and primary follicles (Figure 3). The staining of IGF2 apeared as membranous and cytoplasmic staining in both oocytes and granulosa cells in primordial and primary follicles (Figure 3). The nuclear counter stain is Hoechst (blue) (Figure 3). Quantification of the IGF2 staining in primordial (pixel intensity = 16,1) and primary (pixel intensity = 20,7) follicles supports overall the RNA sequencing FPKM values noted for IGF2 (Table 4), although distinction between cell compartments could not be precisely measured. As such, it is not possible to note of the upregulation of IGF2 is more strong in the oocytes compeared to the granulosa cells in the same follicels stages, although we observed that IGF2 is uregulated on oocytes during the primordial to primary transition, in contrast to downregulation of IGF2 in granulosa cells in the primordial to primary transition (Table 4).
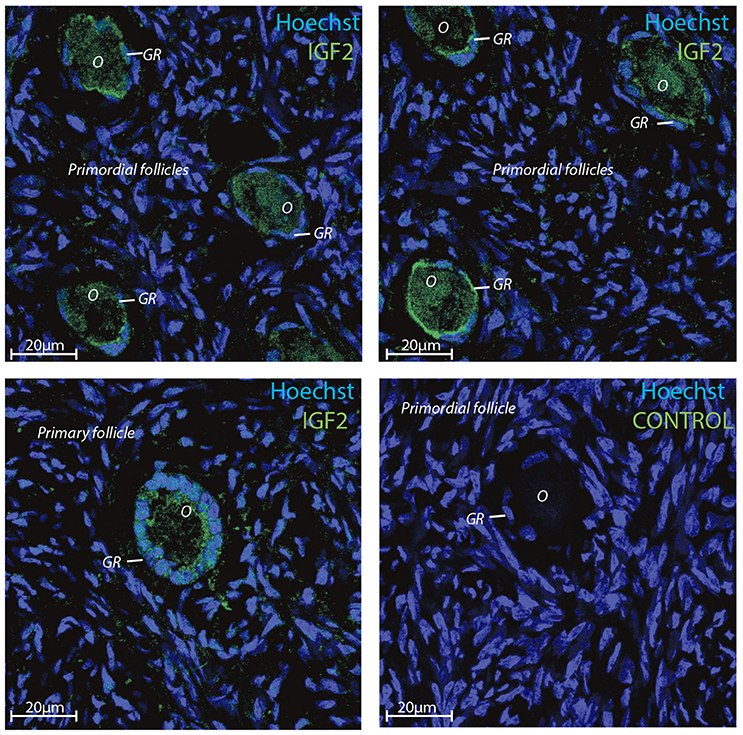
Figure 3. Intra-ovarian distribution of IGF2 in human granulosa cells from primordial and primary follicles. Images show that IGF2 localized to oocytes and granulosa cells in primordial and primary follicles. A control without primary IGF2 antibody was included and reveals no staining. Hoechst staining identifies the nucleus of cells. Scale bars; 20 μm.
Several Androgen-Responsive Genes Appear to Be Expressed During the Primordial to Primary Follicle Transition
To further reveal a potential effect of androgen signaling in primordial and primary follicles, we interrogated the presence of known androgen response genes (Romanuik et al., 2009) in the global transcriptomes of oocytes (Ernst et al., 2017) and granulosa cells (Ernst et al., 2018) from primordial and primary follicles. Of the known androgen-responsive genes (87 genes), 62 genes were found present in the transcriptome data (Table 8). Several of the androgen-responsive genes were very highly expressed (ABHD2, ATP1A1, B2M, FDFT1, GOLPH3, NDRG1, ODC1, PAK2, RPL15, SOD1, TCP1, TPD52, and TSC22D1), and several moderately expressed (such as ACSL3, ADAM28, CNBD1, DHCR24, MANEA, PIK3R3, TMEFF2, and USP33).
Discussion
Ovarian follicles are subjected to to strict control of hormones and growth factors. In human granulosa cells, IGF1 is permissive for the positive feedback toward the FHS-induced expression of aromatase (CYP19A1) through AKT signaling (Baumgarten et al., 2014). This present study performed an in silico analysis of the transcriptomes representing granulosa cells from primordial and primary follicles, respectively. This provides a unique insight into the gene expression and perhaps actions of androgen-signaling and IGF-signaling in the two earliest stages of follicular development in the normal human ovary. Thus, we explored the potential of the earlist human follicles to be able to respond toward signals mediated by IGF- and androgen-signaling.
We applied strict filters in the bioinformatic management, and quality control to ensure the most precise outcome from the global transcriptome analysis. Therefore, the data presented must be evaluated with the fact that there is a fine balance between significant and non-significant outcomes. In some instances, variations between the data from the three patients is noted non-significant. Including more samples might even out this difference, and as many af of statistical analysis are close to a value for significance, it is most likely that most of the non-significant values indeed would be significant. The quantification of the IGF2 immunofluoresence on primordial and primary follicles aligned overall with the FPKM value, however, noteworthy, it is difficult to quantify immunofluorescent on slices performed on human follicles. Additionally, although maternally contributed mRNA are subjected to degradation, the turnover time for the corresponding protein may be differentially regulated and it is not known how much IGF2 protein that might be maternally supplied as well. Previous studies performed qPCR analysis to confirm the expression profiles of selected genes (Ernst et al., 2017, 2018) supporting that the FPKM values obtained reflects the intracellular levels. The analysis contains several DEG-lists based on both SSCEGs and non-SSCEGs. Therefore, caution in the analysis of fold of change for DEG transcripts is recommended. Importantly, this study interrogated the presence of transcripts, which do not necessarily reflect the corresponding protein product. Using single cell techniques, we are able to confirm the presence of proteins using immunohistochemistry. Interestingly, we found androgen signaling highly enriched in granulosa cells from primordial follicles and also enriched in granulosa cells from primary follicles, however less than in the granulosa cells from primordial follicles. Transcripts encoding for AR were significantly expressed in the granulosa cells from primordial follicles, and a non-significant downregulation of the AR gene expression in the granulosa cells during the primordial to primary follicle transition was detected, suggesting a dynamic expression of AR in the granulosa cells. This study is the first to show transcripts of AR expressed in the primordial follicle stage, which indicates an early responsiveness to androgens. Previously the AR transcript has been demonstrated in granulosa cells of rodent, primate and human from transitional follicles (oocyte, surrounded by one layer of mixed flattened and cuboidal granulosa cells) and onwards, but not in earlier follicular stages (Weil et al., 1999; Rice et al., 2007; Sen and Hammes, 2010). Interestingly, the androgen-responsive gene (FDFT1) encoding the Farnesyl diphosphate farnesyltransferase catalyzes the conversion of trans-farnesyl diphosphate to squalene, the first specific step in the cholesterol biosynthetic pathway, suggesting that already at the earliest stages of follicle development, the cells prepare to initiate steroidogenesis. The protein encoded by another androgen-responsive gene (NDRG1) appears to play a role in growth arrest and cell differentiation, possibly as a signaling protein shuttling between the cytoplasm and the nucleus. It is highly expressed during the primordial and primary transition, suggesting this candidate to be important for the activation of dormant oocytes. It is interesting that the androgen-responsive gene, the SOD1 gene, encoding the superoxide dismutase-1 was highly expressed in both primordial and primary follicles. SOD1 is a major cytoplasmic antioxidant enzyme that metabolizes superoxide radicals to molecular oxygen and hydrogen peroxide, thus providing a defense against oxygen toxicity (Niwa et al., 2007). Intriguingly, the androgen-responsive gene, PIK3R3, encodes the phosphoinositide-3-Kinase Regulatory Subunit 3, a lipid kinases capable of phosphorylating the 3'OH of the inositol ring of phosphoinositide, and it has been demonstrated that IGF1R, INSR, and INSR substrate-1 (IRS1) bind to PIK3R3 in vitro (Dey et al., 1998). The study suggested that the interaction of PIK3R3 with IGFIR and INSR provides an alternative pathway for the activation of PI3-kinase.
This study interrogated the presence of the AR transcript through RNA sequencing during the human primordial to primary transition and suggests that at least parts of the AR responsive network, might be relevant during the first ovarian follicle activation step. Although a previous study did not detect the AR transcript in human primordial follicles (Suzuki et al., 1994; Rice et al., 2007), we believe this is attributable to technical limitations since that study used earlier version of mRNA preparations and RT-PCR analysis. The study further highlight the importance of interpretation of RT-PCR and point that its findings do not exclude the presence of a functionally active protein, and the possibility that androgens exert an effect from the earliest growing phase onwards (Rice et al., 2007). In human ovaries, AR was immunohistochemically localized to preantral, antral follicles, theca, and stroma (Chadha et al., 1994; Takayama et al., 1996; Saunders et al., 2000). However, the stage of preantral follicle development could not be specified due to the inherent insensitivity of these techniques. Interestingly in this regard, a study cultured porcine primordial follicles in the absence or presence of testosterone, and found that testosterone increased the activation of primordial follicles (Magamage et al., 2011). The study further utilized cyproterone acetate, an AR antagonist, which inhibited the stimulatory effect of testosterone on primordial follicle activation. In addition, the results from Western blot and immunohistochemistry also showed that the AR was present in porcine primordial follicles. The results from porcine primordial follicles suggest to the possibility that human early follicles may also contain AR protein, however, this remains to be established through immunohistochemistry and protein analysis. In oocytes from primordial follicles, our group has recently demonstrated low, inconsisten expression of AR, and no detectable expression in the oocyte of primary follicles (Ernst et al., 2017). The mechanism of action of androgens is primarily the direct activation of gene transcription, by binding of the ligand-receptor complex to androgen-response elements in the nucleus, but androgens are also known to induce more rapid non-genomic pathways via cytosolic AR and the MAPK/ERK pathway (Kousteni et al., 2001), and influence the IGF-signaling (Vendola et al., 1999a). In the transcriptomic data from granulosa cells from primordial follicles (Ernst et al., 2018), “IGF1 signaling” and “ERK signaling” were, however not significantly enriched, which suggests that the possible androgen signaling mechanisms in the granulosa cells from primordial follicles is based on binding of androgens to nuclear AR, and the direct genomic transcriptional induction. This is in contrast to the results from oocytes from primordial and primary follicles, where the “IGF1 Signaling” and “ERK signaling” pathways were both enriched (Ernst et al., 2017), demonstrating that the non-genomic cytosolic pathway might be the molecular mechanism of action of the androgens in the oocyte-compartment. Androgen signaling has besides the above-mentioned pathways also been linked to the canonical PI3K/PTEN/Akt pathway, which is known to regulate primordial follicle activation in human and rodent (Adhikari et al., 2012; Novella-Maestre et al., 2015). In neonatal mice, activation of the PI3K/PTEN/Akt pathway has been detected, with phosphorylation and translocation of FOXO3a, shortly after testosterone administration, as well as an increased percentage of growing follicles compared to controls (Yang et al., 2010). The link between androgen signaling and IGF1 signaling has been highly argued, as androgens were found to induce upregulation of IGF1 and IGF1R in oocytes of primordial follicles, which is positively correlated to follicular recruitment and activation (Vendola et al., 1999a). In the granulosa cells from primordial and primary follicles, we found that the “IGF1 Signaling” pathway as a group was not significantly enriched, but transcripts of several members of the IGF1 signaling family were detected including IGFBP3 and IGFBP2 in the primordial stage and IGFBP4 in the primary follicle stage. During the primordial to primary follicle transition IGFBP3 was significantly down-regulated. We find this down-regulation of IGFBP3 interesting, given IGFBP3's role as a modulator and antagonist of the IGF-IGFR interaction (Hu et al., 2017), which we speculate to be central in the fine-tuned regulation of IGF2-IGF1R interaction and thus downstream PI3K/PTEN/Akt activity in the activation of primordial follicles. Also, in the oocyte compartment, different interesting members of this pathway were detected including IGF2 and IGF1R, both with a high expression (Ernst et al., 2017). Both the transcripts of IGF1R and IGF2 were upregulated during the primordial to primary follicle transition in the oocyte, the latter of the two with a 2-fold increase. In contrast to the high expression of IGF2 in oocytes from primordial and primary follicles, IGF1 was only inconsistently expressed in a moderate level, which is consistent with previous studies on human ovarian tissue, showing that IGF2 seems more important than IGF1 in the normal ovarian physiology (Mazerbourg et al., 2003; Stubbs et al., 2013). However, our immunohistochemistry results clearly show that IGF2 protein is present in both oocytes and granulosa cell from primordial follicles, suggesting that it may also be maternally contributed as a protein. IGF1R is of particular interest, as it is a known upstream activator of the PI3K/PTEN/Akt pathway (Makker et al., 2014), which is known to be involved in the regulation of primordial follicles.
In the IGF signaling system, several other receptors besides IGF1R are also noteworthy; IGF2R and INSR. Transcripts of IGF2R and INSR were both detected in the oocytes, however the expression was low and inconsistent (Ernst et al., 2017). A higher expression of IGF2R was however detected in the whole follicle isolate compared to the oocyte only isolate, suggesting that IGF2R most likely is expressed in the granulosa cell compartment. Based on our collective results from the transcriptomic analysis of the primordial and primary granulosa cells and oocytes, and the existing literature, we pose the following hypothesis concerning the bidirectional communication in the primordial follicle activation: (1) Based on high AR expression, granulosa cells of primordial follicles may be androgen responsive through direct genomic action, (2) This responsiveness may induce transcription of paracrine factors, which in turn could stimulate the oocyte to express transcripts encoding IGF2 and IGF1R, (3) The regulation is the IGF signaling is tightly regulated, and the IGFBP1s are significant regulators of IGF signaling (Allard and Duan, 2018; Mazerbourg and Monget, 2018; Spitschak and Hoeflich, 2018). Therefore, the androgen responsiveness and its potential induction of IGF2 and IGF1R transcription could be mediated through the activation of IGFBPs and IGF ligands in the grnaulosa cells during the primodial to primary transition, which through paracrine actions stimulate transcription of specific genes in the oocytes. In line with this, as mentioned above, androgen can mediate non-genomic signaling, which may also be relevant for IGF signaling. Activated AR in the cytoplasm can interact with several signaling molecules inclu ing the PI3K/Akt, Src, Ras-Raf-1, and PKC, which in turn converge on MAPK/ERK activation, leading to cell proliferation (Kamanga-Sollo et al., 2008). Cell signaling through androgen can also occur without ERK activation. Non-ERK pathways involve activation of mammalian target of rapamycin (mTOR) via the PI3K/Akt pathway or involvement of plasma membrane, G protein coupled receptors (GPCRs) and the sex hormone binding globulin receptor (SHBGR) that modulate intracellular Ca2+ concentration and cyclic adenosine monophosphate (cAMP) levels, respectively (Mellström and Naranjo, 2001; Heinlein and Chang, 2002). IGFBPs display higher binding affinities toward IGF than IGFR1/2, and IGFs are therefore regulated by IGFBPs (Firth and Baxter, 2002; Duan and Xu, 2005). During dormancy, granulosa cell-produced IGFBP3 could sequester IGF2 in the extracellular space, thus antagonizing ligand-receptor interaction. Upon IGFBP3-decrease in granulosa cells during the primordial to primary follicle transition, oocyte-derived IGF2 might be free to exert its local effect and to bind IGF1R on the oocyte thus stimulating growth in an autocrine manner, and at the same time bind to IGF2R on the granulosa cells to paracrinally stimulate cell growth, proliferation, differentiation, and/or survival. The scenario of IGF-mediated functions in ovarian physiology is intriguing and becomes very complex considering the pattern of IGFBP expression profiles (Mazerbourg and Monget, 2018). It has previously been reported that IGFBP1 is expressed in granulosa cells of mature follicles (el-Roeiy et al., 1994; Kwon et al., 2010), and it is likely that the expression of IGFBP1 increases during follicles development, as we note very low expression (if any) in both oocytes and granulosa cells from primordial and primary follicles. Previously, it was reported that IGFBP2 decreases in the granulosa cells in an cAMP-dependent but FSH-independent manner, (Cataldo et al., 1993), suggesting perhaps an early role of this IGFBP in the early non-FSH responsive phase of follicle development. IGFBP4 mRNA is expressed at low levels in both oocytes and granulosa cells from primordial and primary follicles, and is in line with a previous study that noted IGFBP4 mRNA expression as decreasing during human follicle development (Kwon et al., 2010). While IGFBP5 is higly expressed in all cells in both primordial and primary follicles, IGPBP6 appears to be specific to granulosa cells in primordial follicles. Finally it is worthnoty that many IGF-independent functions have been reported for the IGFBPs (Allard and Duan, 2018), adding another layer of complexity to ovarian functions for this family, which hopefully will be addressed in future functional studies. Further studies are, however, needed to support this link between androgens and IGF-driven primordial follicle activation in the human ovary.
Androgens have received increased attention as a key-player in the early follicular development, as the hyperandrogenic microenvironment in the ovaries from women suffering from PCOS, is thought to be central in the anovulation phenotype (Franks and Hardy, 2010). Patients suffering from anovulatory PCOS is shown to have an increased percentage of growing follicles and stockpiling of the primary follicles compared to controls (Webber et al., 2003; Maciel et al., 2004). In a recent study, it was shown that oocytes from women suffering from hyperandrogenism have an increased expression of IGF2 (Tian et al., 2017). In a future study comparing transcriptomic data from PCOS granulosa cells and oocytes, it would be interesting to investigate if also the IGF1R expression is increased in these patients. According to our hypothesis a potential pathogenic mechanism of PCOS could be that androgen-driven overexpression of IGF1R, would make the oocyte hypersensitive to growth factors such as IGF2, which is found in a high level, and thus trigger hyperactivity in the PI3K/PTEN/Akt pathway, resulting in a hyperactivation of primordial follicles.
Materials and Methods
Tissue Collection and Follicle Isolation
Normal ovarian cortex tissue was donated from three women undergoing oophorectomy followed by cryopreservation before gonadotoxic treatment of non-gynecological cancer. The patients were aged 26, 34, and 34 years old, respectively. Written informed consent was obtained from all patients. The study was approved by Danish Scientific Ethical Committee (Approval number: KF299017 and J7KF/01/170/99) and the Danish Data Protection Agency. From the donated random selected tissue pieces 539 primordial follicles and 261 primary follicles were collected using the Laser Capture Microdissection (LCM) technique using Veritas™ Microdissection Instrument Model 704 (Arcturus XT™, Molecular Devices, Applied Biosystems, Life Technologies, Foster City, CA, U.S.A). The follicles and oocytes were isolated based on their morphological appearance.
LCM, library preparation, sequencing, bioinformatics management, and enrichment analysis was performed essentially as described previously (Ernst et al., 2017, 2018). Briefly, thee human cortical fragments (2 × 2 × 1 mm) were thawed and fixed by immersion into 4% paraformaldehyde (PFA) at 4°C for 4 h followed by dehydration and embedment in paraffin, and the embedding and sectioning was performed as previously described (Markholt et al., 2012). For the LCM isolation, whole follicles and oocytes were captured based on morphological appearance. Oocytes surrounded by 3–5 flattened pre-granulosa cells were defined as primordial follicles, whereas primary follicles were identified as an oocyte surrounded by one layer of cuboidal granulosa cells. During the laser capture, an outline surrounding the cells of interest (oocyte only or whole follicles isolates) was marked microscopically and subsequently cut using the ultraviolet laser. Membrane glass slides (Arcturus® PEN Membrane Glass Slides, Applied Biosystems, Life Technologies, Foster City, CA, U.S.A.), enabled to lift the cells onto a sterile cap (Arcturus® CapSure® HS LCM Caps, Applied Biosystems, Life Technologies, Foster City, CA, U.S.A.) using infrared pulses.
RNA extraction, Library preparation and sequencing, mapping, and statistical analysis as previously described (Ernst et al., 2017, 2018). Briefly, Total RNA was extracted from LCM-isolated cells using Arcturus® Paradise® Plus RNA Extraction and Isolation Kit (#KIT0312I Arcturus Bioscience Inc., Mountain View, CA, U.S.A.), and subjected to linear amplification using the Ovation® RNA-Seq System V2 kit (NuGen Inc., San Carlos, CA, U.S.A.), and RNA-seq libraries were constructed from the output cDNA using Illumina TruSeq DNA Sample and Preparation kit (Illumina, San Diego, CA, USA), performed at AROS Applied Biotechnology, according to the manufacturer's protocol. BAM files were generated using Tophat (2.0.4), and Cufflinks (2.0.2) created a list of expressed transcripts. BWA (0.6.2) mapped all readings to the human reference genome (hg19). Expression of each gene in a given sample was normalized and transformed to a measurement of log2 [counts per million (CPM)]. Afterwards, fragments per kilobase of exon per million fragments mapped (FPKM) values were calculated on the basis of log2 (CPM) (R Core Team, 2012).
Output From Statistical Analysis for Enrichment Analysis
In silico extraction of granulosa cell transcriptomes was performed on global transcriptome data from patient triplicates of oocytes and oocytes with surrounding granulosa cells (follicle) for both the primordial and primary stage (Ernst et al., 2017, 2018) applying strict filters. The FPKM for all detected transcripts was quantified by performing a t-test on patient triplicate samples of same type. The level of consistency was based on p-values, with a low p-value noting a high degree of consistency in FPKM mean across patient triplicates. The cut-off in the level of consistency for all transcripts was set at p < 0.2 across triplicates for being included in all downstream analyses. Afterwards, we identified transcripts uniquely detected in the follicle isolates, and not in corresponding oocytes. All transcripts with a value >1.5 FPKM, was considered uniquely expressed in the follicle isolates and regarded as granulosa cell transcriptome contributions.
Extraction of transcripts encoding the androgen receptor and IGF-related molecules was performed from the lists generated (Ernst et al., 2018) and shows SSCEG and DEG in granulosa cells from primordial (Tables 2, 3) and primary follicles (Tables 2, 5), respectively.
The canonical AR and IGF1 Signaling Pathways were built using IPA® software (http://www.ingenuity.com).
Immunofluorescence Microscopy
Ovarian cortical tissue was sectioned in 5 μm slides and mounted on glass slides. Dehydration and antigen retrieval was performed as described elsewhere (Stubbs et al., 2005) followed by serum block (30 min), then primary antibody; anti-IGF2 rabbit polyclonal antibody (ab9574, Abcam, Cambridge, U.K.), (5 μg/ml) overnight at 4°C. This antibody was previously used and validated (Huang et al., 2010) and several other applications (http://www.abcam.com/igf2-antibody-ab9574-references.html). The sections were subsequently incubated in a 1:250 dilution of appropriate secondary antibody (donkey-anti-rabbit for IGF2) conjugated with Alexa Fluor 488 Dye (Life Technologies, Carlsbad, CA, U.S.A.). Sections were incubated in 1/3,500 Hoechst (Life Technologies, Carlsbad, CA, U.S.A.) followed by mounting with Dako Fluorescent Mounting Medium (Agilent Technologies, Santa Clara, CA, U.S.A) and analyzed using a LSM510 laser-scanning confocal microscope using a 63x C-Apochromat water immersion objective NA 1.2 (Carl Zeiss, Göttingen, Germany). Zen 2011 software (Carl Zeiss, Göttingen, Germany) was used for analysis and image capturing. The quantification of IGF2 immunofluorescence was done by as ImageJ (Jensen, 2013).
Author Contributions
LS, EHE, and KL-H conceived the study. LS, EHE, and KL-H analyzed NGS and IPA® data. MA performed ICH and analyzed data. EE provided ovarian tissue from patients. LS, EHE, and KL-H wrote the manuscript. All authors approved the final manuscript.
Funding
EHE was supported by a Ph.D. scholarship from Health Faculty, Aarhus University. This work was further supported by The Independent Research Fund (DFF), Denmark (DFF−6120-00027 to KL-H), which supported LS and by grants from the Novo Nordisk Foundation (NNF16OC0022480 to KL-H (which supported MA), Fonden til Lægevidenskabens Fremme (to KL-H), Kong Christian Den Tiendes Fond (to KL-H), and Augustinus Fonden (to KL-H).
Conflict of Interest Statement
The authors declare that the research was conducted in the absence of any commercial or financial relationships that could be construed as a potential conflict of interest.
Acknowledgments
We wish to thank the clinical, paramedical and laboratory team of the Fertility Clinic, Aarhus University Hospital and Lykke-Hartmann laboratory (AU) for help and scientific discussions. We thank Elisabeth Bruun for her help on immunofluorescence quantification.
References
Adashi, E. Y., Resnick, C. E., D'Ercole, A. J., Svoboda, M. E., and Van Wyk, J. J. (1985). Insulin-like growth factors as intraovarian regulators of granulosa cell growth and function. Endocr. Rev. 6, 400–420. doi: 10.1210/edrv-6-3-400
Adashi, E. Y., Resnick, C. E., Hernandez, E. R., Hurwitz, A., Roberts, C. T., Leroith, D., et al. (1991). Insulin-like growth factor I as an intraovarian regulator: basic and clinical implications. Ann. N. Y. Acad. Sci. 626, 161–168. doi: 10.1111/j.1749-6632.1991.tb37910.x
Adhikari, D., Gorre, N., Risal, S., Zhao, Z., Zhang, H., Shen, Y., et al. (2012). The safe use of a PTEN inhibitor for the activation of dormant mouse primordial follicles and generation of fertilizable eggs. PLoS ONE 7:e39034. doi: 10.1371/journal.pone.0039034
Allard, J. B., and Duan, C. (2018). IGF-binding proteins: why do they exist and why are there so many? Front. Endocrinol. 9:117. doi: 10.3389/fendo.2018.00117
Armstrong, D. G., Hogg, C. O., Campbell, B. K., and Webb, R. (1996). Insulin-like growth factor (IGF)-binding protein production by primary cultures of ovine granulosa and theca cells. Effects of IGF-I, gonadotropin, and follicle size. Biol. Reprod. 55, 1163–1171.
Baumgarten, S. C., Convissar, S. M., Fierro, M. A., Winston, N. J., Scoccia, B., Stocco, C., et al. (2014). IGF1R signaling is necessary for FSH-induced activation of AKT and differentiation of human Cumulus granulosa cells. J. Clin. Endocrinol. Metab. 99, 2995–3004. doi: 10.1210/jc.2014-1139
Becerra-Fernández, A., Perez-Lopez, G., Roman, M. M., Martin-Lazaro, J. F., Lucio Perez, M. J., Asenjo Araque, N., et al. (2014). Prevalence of hyperandrogenism and polycystic ovary syndrome in female to male transsexuals. Endocrinol. Nutr. 61, 351–358. doi: 10.1016/j.endonu.2014.01.010
Cataldo, N. A., Woodruff, T. K., and Giudice, L. C. (1993). Regulation of insulin-like growth factor binding protein production by human luteinizing granulosa cells cultured in defined medium. J. Clin. Endocrinol. Metab. 76, 207–215.
Chadha, S., Pache, T. D., Huikeshoven, J. M., Brinkmann, A. O., and van der Kwast, T. H (1994) Androgen receptor expression in human ovarian uterine tissue of long-term androgen-treated transsexual women. Hum. Pathol. 25, 1198–1204. doi: 10.1016/0046-8177(94)90037-X
Dey, B. R., Furlanetto, R. W., and Nissley, S. P. (1998). Cloning of human p55 gamma, a regulatory subunit of phosphatidylinositol 3-kinase, by a yeast two-hybrid library screen with the insulin-like growth factor-I receptor. Gene 209, 175–183. doi: 10.1016/S0378-1119(98)00045-6
Duan, C., and Xu, Q. (2005). Roles of insulin-like growth factor (IGF) binding proteins in regulating IGF actions. Gen. Comp. Endocrinol. 142, 44–52. doi: 10.1016/j.ygcen.2004.12.022
el-Roeiy, A., Chen, X., Roberts, V. J., Shimasakai, S., Ling, N., LeRoith, D., et al. (1994). Expression of the genes encoding the insulin-like growth factors (IGF-I and II), the IGF and insulin receptors, and IGF-binding proteins-1-6 and the localization of their gene products in normal and polycystic ovary syndrome ovaries. J. Clin. Endocrinol. Metab. 78, 1488–1496.
Eppig, J. J. (2001). Oocyte control of ovarian follicular development and function in mammals. Reproduction 122, 829–838. doi: 10.1530/rep.0.1220829
Ernst, E. H., Franks, S., Hardy, K., Villesen, P., and Lykke-Hartmann, K. (2018). Granulosa cells from human primordial and primary follicles show differential global gene expression profiles. Hum. Reprod. 33, 666–679. doi: 10.1093/humrep/dey011
Ernst, E. H., Grondahl, M. L., Grund, S., Hardy, K., Heuck, A., Sunde, L., et al. (2017). Dormancy and activation of human oocytes from primordial and primary follicles: molecular clues to oocyte regulation. Hum. Reprod. 32, 1684–1700. doi: 10.1093/humrep/dex238
Firth, S. M., and Baxter, R. C. (2002). Cellular actions of the insulin-like growth factor binding proteins. Endocr. Rev. 23, 824–854. doi: 10.1210/er.2001-0033
Franks, S. (1995). Polycystic ovary syndrome. N. Engl. J. Med. 333, 853–861. doi: 10.1056/NEJM199509283331307
Franks, S., and Hardy, K. (2010). Aberrant follicle development and anovulation in polycystic ovary syndrome. Ann. Endocrinol. 71, 228–230. doi: 10.1016/j.ando.2010.02.007
Gelmann, E. P. (2002). Molecular biology of the androgen receptor. J. Clin. Oncol. 20, 3001–3015. doi: 10.1200/JCO.2002.10.018
Gervasio, C. G., Bernuci, M. P. Silva-de-Sa, M. F., and Rosa E-Silva, A. C. (2014). The role of androgen hormones in early follicular development. ISRN Obstet Gynecol. 2014:818010. doi: 10.1155/2014/818010
Hague, W. M., Adams, J., Rodda, C., Brook, C. G., de Bruyn, R., Grant, D. B., et al. (1990). The prevalence of polycystic ovaries in patients with congenital adrenal hyperplasia and their close relatives. Clin. Endocrinol. 33, 501–510. doi: 10.1111/j.1365-2265.1990.tb03887.x
Heinlein, C. A., and Chang, C. (2002). The roles of androgen receptors and androgen-binding proteins in nongenomic androgen actions. Mol. Endocrinol. 16, 2181–2187. doi: 10.1210/me.2002-0070
Hu, Q., Zhou, Y., Ying, K., and Ruan, W. (2017). IGFBP, a novel target of lung cancer? Clin. Chim. Acta 466, 172–177. doi: 10.1016/j.cca.2017.01.017
Huang, G. S., Brouwer-Visser, J., Ramirez, M. J., Kim, C. H., Hebert, T. M., Lin, J., et al. (2010). Insulin-like growth factor 2 expression modulates Taxol resistance and is a candidate biomarker for reduced disease-free survival in ovarian cancer. Clin. Cancer Res. 16, 2999–3010. doi: 10.1158/1078-0432.CCR-09-3233
Jensen, E. C. (2013). Quantitative analysis of histological staining and fluorescence using Image. J. Anat. Rec. 296, 378–381. doi: 10.1002/ar.22641
Kamanga-Sollo, E., White, M. E., Hathaway, M. R., Chung, K. Y., Johnson, B. J., and Dayton, W. R. (2008). Roles of IGF-I and the estrogen, androgen and IGF-I receptors in estradiol-17beta- and trenbolone acetate-stimulated proliferation of cultured bovine satellite cells. Domest Anim. Endocrinol. 35, 88–97. doi: 10.1016/j.domaniend.2008.02.003
Knapczyk-Stwora, K., Grzesiak, M., Duda, M., Koziorowski, M., and Slomczynska, M. (2013). Effect of flutamide on folliculogenesis in the fetal porcine ovary–regulation by Kit ligand/c-Kit and IGF1/IGF1R systems. Anim. Reprod Sci. 142, 160–167. doi: 10.1016/j.anireprosci.2013.09.014
Kousteni, S., Bellido, T., Plotkin, L. I., O'Brien, C. A., Bodenner, L., Han, D. L., et al. (2001). Nongenotropic, sex-nonspecific signaling through the estrogen or androgen receptors: dissociation from transcriptional activity. Cell 104, 719–730. doi: 10.1016/S0092-8674(02)08100-X
Kuijjer, M. L., Peterse, E. F., van den Akker, B. E., Briaire-de Bruijn, I. H., Serra, M., Meza-Zepeda, L. A., et al. (2013). IR/IGF1R signaling as potential target for treatment of high-grade osteosarcoma. BMC Cancer 13:245. doi: 10.1186/1471-2407-13-245
Kwon, H., Choi, D. H., Bae, J. H., Kim, J. H., and Kim, Y. S. (2010). mRNA expression pattern of insulin-like growth factor components of granulosa cells and cumulus cells in women with and without polycystic ovary syndrome according to oocyte maturity. Fertil. Steril. 94, 2417–2420. doi: 10.1016/j.fertnstert.2010.03.053
Laviola, L., Natalicchio, A., and Giorgino, F. (2007). The IGF-I signaling pathway. Curr. Pharm. Des. 13, 663–669. doi: 10.2174/138161207780249146
Lebbe, M., and Woodruff, T. K. (2013). Involvement of androgens in ovarian health and disease. Mol. Hum. Reprod. 19, 828–837. doi: 10.1093/molehr/gat065
Lodhia, K. A., Tienchaiananda, P., and Haluska, P. (2015). Understanding the key to targeting the igf axis in cancer: a biomarker assessment. Front. Oncol. 5:142. doi: 10.3389/fonc.2015.00142
Maciel, G. A., Baracat, E. C., Benda, J. A., Markham, S. M., Hensinger, K., Chang, R. J., et al. (2004). Stockpiling of transitional and classic primary follicles in ovaries of women with polycystic ovary syndrome. J. Clin. Endocrinol. Metab. 89, 5321–5327. doi: 10.1210/jc.2004-0643
Magamage, M. P. S., Zengyo, M., Moniruzzaman, M., and Miyano, T. (2011). Testosterone induces activation of porcine primordial follicles in vitro. Reprod. Med. Biol. 10, 21–30. doi: 10.1007/s12522-010-0068-z
Makker, A., Goel, M. M., and Mahdi, A. A. (2014). PI3K/PTEN/Akt and TSC/mTOR signaling pathways, ovarian dysfunction, and infertility: an update. J. Mol. Endocrinol. 53, R103–R118. doi: 10.1530/JME-14-0220
Markholt, S., Grondahl, M. L., Ernst, E. H., Andersen, C. Y., Ernst, E., and Lykke-Hartmann, K. (2012). Global gene analysis of oocytes from early stages in human folliculogenesis shows high expression of novel genes in reproduction. Mol. Hum. Reprod. 18, 96–110. doi: 10.1093/molehr/gar083
Mazerbourg, S., and Monget, P. (2018). Insulin-like growth factor binding proteins and igfbp proteases: a dynamic system regulating the ovarian folliculogenesis. Front Endocrinol. 9:134. doi: 10.3389/fendo.2018.00134
Mazerbourg, S., Bondy, C. A., Zhou, J., and Monget, P. (2003). The insulin-like growth factor system: a key determinant role in the growth and selection of ovarian follicles? A comparative species study. Reprod. Domest. Anim. 38, 247–258. doi: 10.1046/j.1439-0531.2003.00440.x
Mellström, B., and Naranjo, J. R. (2001). Mechanisms of Ca(2+)-dependent transcription. Curr. Opin. Neurobiol. 11, 312–319. doi: 10.1016/S0959-4388(00)00213-0
Mondschein, J. S., Canning, S. F., Miller, D. Q., and Hammond, J. M. (1989). Insulin-like growth factors (IGFs) as autocrine/paracrine regulators of granulosa cell differentiation and growth: studies with a neutralizing monoclonal antibody to IGF-I. Biol. Reprod. 41, 79–85. doi: 10.1095/biolreprod41.1.79
Niwa, J., Yamada, S., Ishigaki, S., Sone, J., Takahashi, M., Katsuno, M., et al. (2007). Disulfide bond mediates aggregation, toxicity, and ubiquitylation of familial amyotrophic lateral sclerosis-linked mutant SOD1. J. Biol. Chem. 282, 28087–28095. doi: 10.1074/jbc.M704465200
Novella-Maestre, E., Herraiz, S., Rodriguez-Iglesias, B., Diaz-Garcia, C., and Pellicer, A. (2015). Short-term pten inhibition improves in vitro activation of primordial follicles, preserves follicular viability, and restores amh levels in cryopreserved ovarian tissue from cancer patients. PLoS ONE 10:e0127786. doi: 10.1371/journal.pone.0127786
Padmanabhan, V., and Veiga-Lopez, A. (2013). Sheep models of polycystic ovary syndrome phenotype. Mol. Cell Endocrinol. 373, 8–20. doi: 10.1016/j.mce.2012.10.005
R Core Team (2012). R: A Language and Environment for Statistical Computing. Vienna. Available online at: http://www.R-project.org/
Rice, S., Ojha, K., Whitehead, S., and Mason, H. (2007). Stage-specific expression of androgen receptor, follicle-stimulating hormone receptor, and anti-Mullerian hormone type II receptor in single, isolated, human preantral follicles: relevance to polycystic ovaries. J. Clin. Endocrinol. Metab. 92, 1034–1040. doi: 10.1210/jc.2006-1697
Romanuik, T. L., Wang, G., Holt, R. A., Jones, S. J., Marra, M. A., and Sadar, M. D. (2009). Identification of novel androgen-responsive genes by sequencing of LongSAGE libraries. BMC Genomics. 10:476. doi: 10.1186/1471-2164-10-476
Saunders, P. T., Millar, M. R., Williams, K., Macpherson, S., Harkiss, D., Anderson, R. A., et al. (2000). Differential expression of estrogen receptor-alpha and -beta and androgen receptor in the ovaries of marmosets and humans. Biol. Reprod. 63, 1098–1105. doi: 10.1095/biolreprod63.4.1098
Sen, A., and Hammes, S. R. (2010). Granulosa cell-specific androgen receptors are critical regulators of ovarian development and function. Mol. Endocrinol. 24, 1393–1403. doi: 10.1210/me.2010-0006
Spinder, T., Spijkstra, J. J., van den Tweel, J. G., Burger, C. W., van Kessel, H., Hompes, P. G., et al. (1989). The effects of long term testosterone administration on pulsatile luteinizing hormone secretion and on ovarian histology in eugonadal female to male transsexual subjects. J. Clin. Endocrinol. Metab. 69, 151–157. doi: 10.1210/jcem-69-1-151
Spitschak, M., and Hoeflich, A. (2018). Potential functions of IGFBP-2 for ovarian folliculogenesis and steroidogenesis. Front Endocrinol. 9:119. doi: 10.3389/fendo.2018.00119
Stubbs, S. A., Hardy, K., Da Silva-Buttkus, P., Stark, J., Webber, L. J., Flanagan, A. M., et al. (2005). Anti-mullerian hormone protein expression is reduced during the initial stages of follicle development in human polycystic ovaries. J. Clin. Endocrinol. Metab. 90, 5536–5543. doi: 10.1210/jc.2005-0907
Stubbs, S. A., Webber, L. J., Stark, J., Rice, S., Margara, R., Lavery, S., et al. (2013). Role of Insulin-like growth factors in initiation of follicle growth in normal and polycystic human ovaries. J. Clin. Endocrinol. Metab. 98, 3298–3305. doi: 10.1210/jc.2013-1378
Suzuki, T., Sasano, H., Kimura, N., Tamura, M., Fukaya, T., Yajima, A., et al. (1994). Immunohistochemical distribution of progesterone, androgen and oestrogen receptors in the human ovary during the menstrual cycle: relationship to expression of steroidogenic enzymes. Hum. Reprod. 9, 1589–1595. doi: 10.1093/oxfordjournals.humrep.a138757
Takayama, K., Fukaya, T., Sasano, H., Funayama, Y., Suzuki, T., Takaya, R., et al. (1996). Immunohistochemical study of steroidogenesis and cell proliferation in polycystic ovarian syndrome. Hum. Reprod. 11, 1387–1392. doi: 10.1093/oxfordjournals.humrep.a019405
Tian, S., Lin, X. H., Xiong, Y. M., Liu, M. E., Yu, T. T., Lv, M., et al. (2017). Prevalence of prediabetes risk in offspring born to mothers with hyperandrogenism. EBioMedicine 16, 275–283. doi: 10.1016/j.ebiom.2017.01.011
Vendola, K., Zhou, J., Wang, J., and Bondy, C. A. (1999a). Androgens promote insulin-like growth factor-I and insulin-like growth factor-I receptor gene expression in the primate ovary. Hum. Reprod. 14, 2328–2332.
Vendola, K., Zhou, J., Wang, J., Famuyiwa, O. A., Bievre, M., and Bondy, C. A. (1999b). Androgens promote oocyte insulin-like growth factor I expression and initiation of follicle development in the primate ovary. Biol. Reprod. 61, 353–357. doi: 10.1095/biolreprod61.2.353
Wandji, S. A., Srsen, V., Voss, A. K., Eppig, J. J., and Fortune, J. E. (1996). Initiation in vitro of growth of bovine primordial follicles. Biol. Reprod. 55, 942–948. doi: 10.1095/biolreprod55.5.942
Webber, L. J., Stubbs, S., Stark, J., Trew, G. H., Margara, R., Hardy, K., et al. (2003). Formation and early development of follicles in the polycystic ovary. Lancet 362, 1017–1021. doi: 10.1016/S0140-6736(03)14410-8
Weil, S. J., Vendola, K., Zhou, J., Adesanya, O. O., Wang, J., Okafor, J., et al. (1998). Androgen receptor gene expression in the primate ovary: cellular localization, regulation, and functional correlations. J. Clin. Endocrinol. Metab. 83, 2479–2485. doi: 10.1210/jcem.83.7.4917
Weil, S., Vendola, K., Zhou, J., and Bondy, C. A. (1999). Androgen and follicle-stimulating hormone interactions in primate ovarian follicle development. J. Clin. Endocrinol. Metab. 84, 2951–2956. doi: 10.1210/jcem.84.8.5929
Willis, D. S., Mason, H. D., Watson, H., and Franks, S. (1998). Developmentally regulated responses of human granulosa cells to insulin-like growth factors (IGFs): IGF-I and IGF-II action mediated via the type-I IGF receptor. J. Clin. Endocrinol. Metab. 83, 1256–1259.
Yang, J. L., Zhang, C. P., Li, L., Huang, L., Ji, S. Y., Lu, C. L., et al. (2010). Testosterone induces redistribution of forkhead box-3a and down-regulation of growth and differentiation factor 9 messenger ribonucleic acid expression at early stage of mouse folliculogenesis. Endocrinology 151, 774–782. doi: 10.1210/en.2009-0751
Keywords: human granulosa cells, transcriptome, follicle development, AR, IGF
Citation: Steffensen LL, Ernst EH, Amoushahi M, Ernst E and Lykke-Hartmann K (2018) Transcripts Encoding the Androgen Receptor and IGF-Related Molecules Are Differently Expressed in Human Granulosa Cells From Primordial and Primary Follicles. Front. Cell Dev. Biol. 6:85. doi: 10.3389/fcell.2018.00085
Received: 03 April 2018; Accepted: 18 July 2018;
Published: 10 August 2018.
Edited by:
Eiman Aleem, College of Medicine Phoenix, University of Arizona, United StatesReviewed by:
Rajprasad Loganathan, School of Medicine, Johns Hopkins University, United StatesAhmed Waraky, University of Gothenburg, Sweden
Copyright © 2018 Steffensen, Ernst, Amoushahi, Ernst and Lykke-Hartmann. This is an open-access article distributed under the terms of the Creative Commons Attribution License (CC BY). The use, distribution or reproduction in other forums is permitted, provided the original author(s) and the copyright owner(s) are credited and that the original publication in this journal is cited, in accordance with accepted academic practice. No use, distribution or reproduction is permitted which does not comply with these terms.
*Correspondence: Karin Lykke-Hartmann, kly@biomed.au.dk
†Present Address: Emil H. Ernst, Department of Obstetrics and Gynaecology, Herning Hospital, Herning, Denmark