- 1Smart Shelter Research, Alphen aan den Rijn, Netherlands
- 2Graduate School of Environmental Studies, Nagoya University, Nagoya, Japan
A literature review was carried out with the aim to determine the current state-of-the-art with regards to non-engineered stone masonry in seismic areas. Specific search criteria and definitions were determined for school buildings with loadbearing walls of rubble stone masonry in cement mortar, nominally tied with horizontal reinforcements. A total of 47 relevant field manuals between 1972 and 2017 were analyzed for eligibility and checked for compatibility, initially with 109 national building codes. An overview was created of similarities, contradictions, gaps and differences between the publications. As the majority of the practical manuals target the Himalayan context, the most relevant codes for the comparison were the Indian and Nepali seismic and building codes. Only 9 manuals describe design and construction processes for schools, even though this conflicts with the codes which specifically prohibit the use of stone masonry for buildings with importance factor 1.5 or higher. It was noticed that 7 out of 9 manuals are (co-)written by the same author, and that the available knowledge, which is largely based on empirical evidence, can be traced back to just a few main sources. However, no consistency nor consensus was found on almost all key topics, such as main dimensions, openings and reinforcing elements. Also, the same illustrations and tables are copied over and over again, including apparent conflicts between the details. The fact that this has never been rectified, as well as the fact that the Indian and Nepali seismic codes have not been properly updated since 1994, are indications that the knowledge has not evolved much since the 1980's. It is concluded that the available information contains many contradictions and has become ambiguous. This raises questions about the correctness, reliability and actual value of the knowledge. The paper suggest that the existing knowledge must be fully assessed, validated, optimized and complemented by means of the current state-of-the-art for calculating, testing and modeling. To address this, the authors have started an initiative named SMARTnet, and make an appeal to experts and academics worldwide, to exchange their knowledge and to support the project with their time and expertise.
Introduction
Between 2007 and 2012 the Dutch non-for-profit organization Smart Shelter Foundation built several earthquake resistant schools in rubble stone masonry in Nepal. The designs were made by Martijn Schildkamp, architect and first author of this paper, and the design rules were obtained from the numerous technical guidelines and practical manuals that can be found online. These general rules of thumb are commonly referred to as “best practice” or “non-engineered construction principles.” While studying the available knowledge, it was the personal experience of Schildkamp that the information was often unclear, contradictive and incomplete. Therefore, he reached out to several members of Earthquake Engineering Research Institute (EERI), to ask for guidance during the different design and construction phases. For instance, questions were asked about plan dimensions, horizontal bands and vertical reinforcements. These were reviewed and discussed by a group of EERI members until a consensus was reached and a collective personalized recommendation was formulated, which was followed and executed by Smart Shelter Foundation.
Although all 15 schools have withstood the 2015 Gorkha earthquakes without any significant damage (mostly hairline cracks, this is further explained in the section about the sill band), during the design and construction of the projects many technical questions and practical issues came to light. This paper undertakes a literature review to determine the state-of-the-art with regards to rubble stone masonry construction in seismically-prone developing countries. It further aims to summarize the generalities, similarities, contradictions and discrepancies, as well as any need for further validation, optimization and complementation of the existing knowledge. To achieve this, all possible design requirements, construction details and practical implications are described and compared, resulting in a complete overview of all the necessary steps toward completion of a school construction project.
The review also aims to determine whether the publications follow the latest building codes and if they sufficiently address the current needs, such as for the reconstruction effort in post-earthquake Nepal. As of today (October 2018) official numbers have still not been released by the Nepali government, but it is estimated that nearly 1,000,000 houses and 57,000 classrooms were destroyed and damaged throughout the country (The Post Disaster Recovery Framework, 2016). It is further estimated that 81% of all building damage took place in the rural areas, where 95% of all collapsed structures consisted of low-strength masonry; the majority being stone with mud mortar (National Planning Commission, 2015). Due to limited finance and access to resources it is expected that the vast majority of these will be rebuilt in stone masonry again. Therefore, availability of reliable and up-to-date information is of the utmost importance.
This research paper is the first time a literature review of seismic codes, technical guides and construction manuals has been compiled in this subject matter. The focus of the review is to determine the applicability and reliability of existing publications, and to understand the need for revision of the existing knowledge, development of reliable manuals and upgrading of national seismic codes. The authors acknowledge the fact that this review is the starting point of a more complex process. It is not a validation of the technical content, as this would involve in-depth scientific research through multi-disciplinary collaborations, for which suggestions are given in the conclusion.
Definitions and Search Criteria
This section describes what was actually built by Smart Shelter Foundation in Nepal. It follows the rules of thumb as advised by the experts of EERI and as outlined in Schildkamp (2015a), and details the type of masonry, the loadbearing wall typology and the inclusion of different horizontal and vertical reinforcements of the school buildings. Based on this description, the search criteria are defined for the literature reviews of national seismic codes and practical construction manuals. Some further parameters are included about the different types of publications and their content. Although Nepal has officially adopted the Metric System (Government of Nepal, 1968), most villages still use the Imperial System and therefore the drawings of Figure 1 are expressed in feet and inches. All units in this paper however follow the SI metric system such as meter (m) and millimeter (mm).
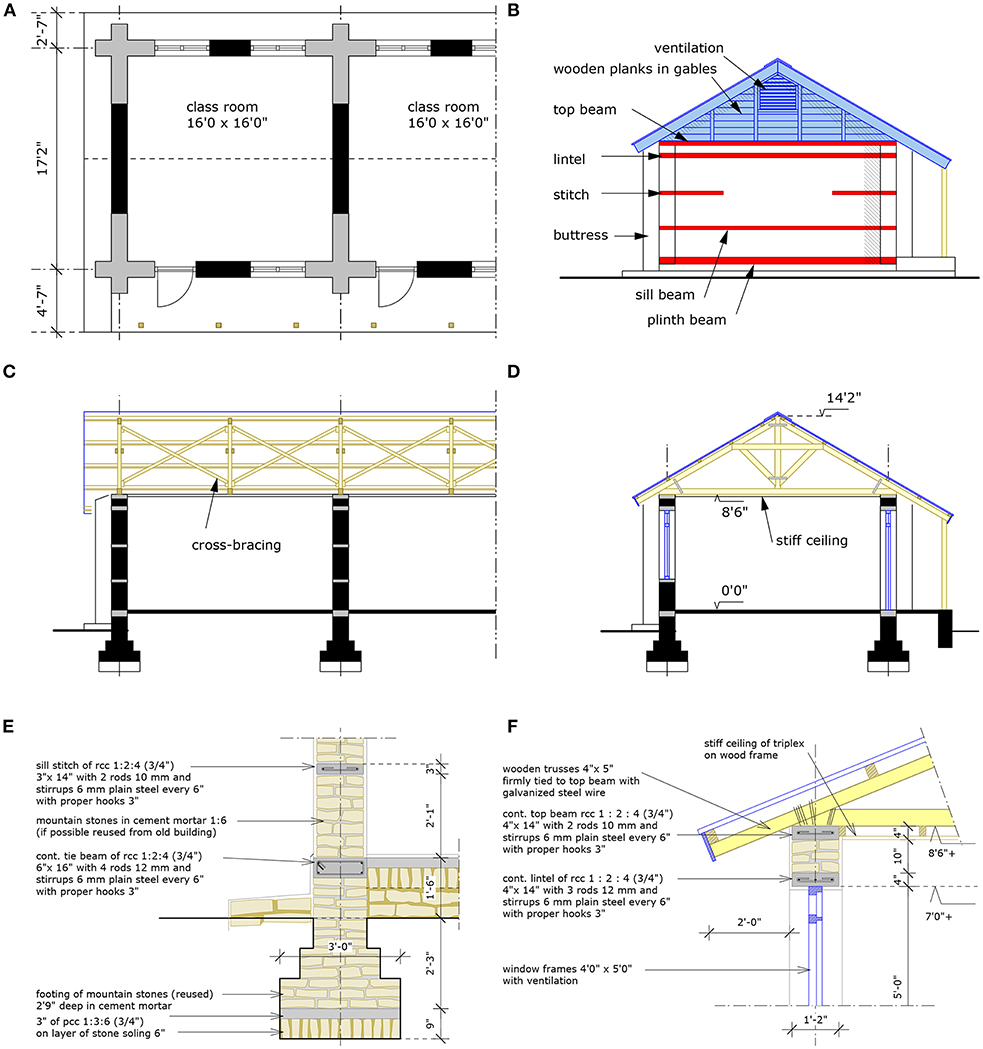
Figure 1. (A) School plan in rubble stone masonry and (B) Side elevation with horizontal reinforcements and buttresses. (C,D) Cross-sections over building. (E) Detail of foundation, floor and wall in rubble stone masonry, and (F) Detail of window, wall, and roof connection (all by courtesy of Smart Shelter Foundation).
School Designs of Smart Shelter Foundation
The classrooms have a maximum interior floor plan of 4.8 × 4.8 m, Figure 1A. The dimensions of the building volume do not exceed the maximum ratio of width vs. depth of 1:3. This translates to maximum 3 classrooms in a row, or else a separation gap is introduced of 75 mm between the volumes.
A stepped strip foundation in rubble stone masonry with cement-sand mortar is placed on a plain concrete screed, on top of a layer of rough boulders. The top of the foundation including tie beam is raised 450 mm above ground level (Figure 1E).
The walls consist of 350 mm thick random rubble stone masonry in cement-sand mortar, with buttresses at all wall ends. The walls have a maximum height of 2.6 m, from the top of the foundation beam to the top of the wall. The mountain stones are not dressed, but regularly sized stones are chosen and placed in courses as consistent as possible. The masonry includes many bond stones that are placed over the entire thickness of the wall, to decrease the risk of delamination of the stone wythes.
The total combined width of openings does not exceed more than 50% of the length of a wall panel, with a minimum distance between inside of the corner and opening, as well as a minimum width for piers between openings, of 600 mm. The doors open to the outside for safe exit during an emergency.
As the schools are located in a high seismic zone, the walls are tied together with horizontal bands made of reinforced concrete at five different levels in height, Figure 1B. These are a continuous plinth beam on the foundation, and a sill band under the windows which is semi-continuous as it is interrupted by the doors. In-between stitches in the corners and T-sections break the height between sill and the most important lintel beam, that runs over all door and window openings. Same as the lintel, the top beam is also fully continuous. Different thicknesses of the beams, as well as different numbers and diameters for the steel reinforcements were used.
Other than stone masonry buttresses, no vertical reinforcements are incorporated in the critical wall connections, such as at the corners, t-sections and around openings. In 2007, the EERI advisory team reasoned that the limited amount of steel will not provide the desired amount of ductility. Also, the steel will disrupt the masonry bonding in these critical connections, which possibly makes these weaker rather than stronger, and therefore may create more problems than benefits. However, this remains to be a highly debated subject among the experts (and is basically the reason for this paper and starting point of all upcoming in-depth research). The need for vertical reinforcements will be further discussed in the review of the practical manuals.
Instead of heavy masonry gables that have the risk of toppling during an earthquake, wooden trusses are placed on all interior and on the end walls, and then closed with wooden planks with openings for cross-ventilation. Further trusses are placed at intermediate points which are inter-connected with cross-bracing elements and purlins, and a stiff ceiling is placed underneath. This way the roof structure acts as one, thus enhancing the box action of the total building. Around 2007, big bolts were not available in the local markets, so strands of 4 mm galvanized steel wire were cast into the top beam, to firmly tighten the wooden trusses.
To guarantee a high construction quality, much emphasis was put on the training and supervision of the local laborers during the construction process, following the practical principles as described in Schildkamp (2015b). Much emphasis was put on the use of correct materials, preparation of proper mixes for mortars and concretes, and detailing of steel reinforcements. After completion of the building, all earthquake resistant measures were painted on the outside of the building, with explanation in Nepali text, so that the building becomes a full-size billboard for earthquake resistant construction.
Types of Stone Masonry and Mortars
Stone as a material can be categorized into numerous typologies, but in terms of stone masonry it can essentially be brought back to two major categories: Rubble stone and Ashlar. Units for rubble stone masonry may consist of field stones, river boulders (Figure 2A), or mountain rocks, either randomly stacked (Figure 2B) or brought to courses (Figure 2C). When rocks are cut into rectangular units with straight adjoining sides it is called Ashlar, also known as cut, squared or dressed stone (Figure 2D). To cut such neat units by hand involves lots of intensive labor, which is highly dependent on the hardness of the stone and the required level of shaping and finishing. This makes Ashlar much more expensive than rubble stone and it is therefore less often used in the rural areas.
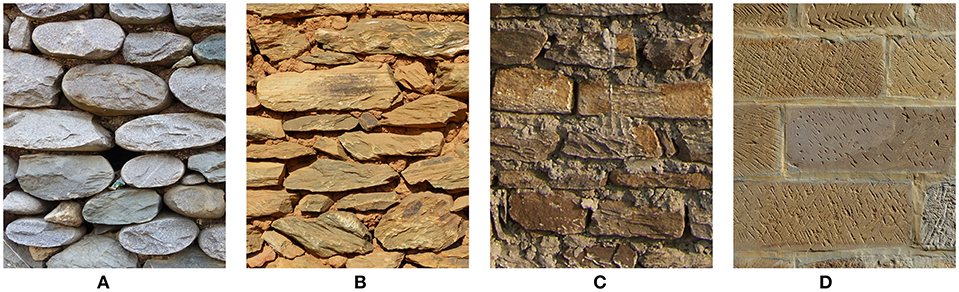
Figure 2. (A) Round river boulders with mud mortar. (B) Random rubble stone masonry with mud mortar. (C) Random rubble stone masonry brought to courses with cement mortar. (D) Ashlar stone masonry with lime-sand mortar (all by courtesy of Smart Shelter Foundation).
The shape of the stone is important for the structural stability of the wall. Generally said, the rounder the boulder, or the more irregular the shape of the rock, the more difficult it is to build a consistent and stable wall. A distinction is made between uncoursed or coursed stone masonry, and the strength of a wall is further influenced by the way the stone units are laid, such as the detailing of corners and junctions, the bonding patterns, overlapping and interlocking of the units, and the thickness and continuity of the joints.
Of equal importance is the type of masonry mortar that is used. Mud is the main choice in the rural and remote areas in most developing countries, followed by cement mortar if the people can afford it, or lime-sand mortar if lime is available, although this is not very common in the Himalayan regions. Mortar-less masonry, as used in parts of Pakistan and India, behaves much different in an earthquake compared to mortared walls (Carabbio et al., 2018) and therefore dry-stacked stone masonry falls outside the scope of this review. No publications are known by the authors about the use of stabilized mud mortar in seismic areas.
By combining stone unit and mortar type, Arya (2003) grades stone masonry in six classes relative to its seismic safety, thus placing the schools of Smart Shelter Foundation in the second safety level, Table 1.
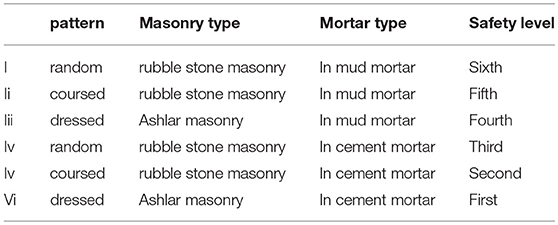
Table 1. Grading of stone masonry types according to Arya (2003).
Types of Load-bearing Masonry Systems
A distinction can be made between three important different typologies of load-bearing masonry systems, which are reinforced masonry (RM), confined masonry (CM) and unreinforced masonry (URM), as follows:
Reinforced masonry has regular horizontal and/or vertical reinforcements throughout the wall which are embedded in such way, that they act together with the masonry units in resisting lateral forces in both in-plane and out-of-plane directions. RM must be designed and calculated by engineers and is therefore categorized as an engineered construction technique.
Confined masonry walls act as shear panels which serve as the lateral load-bearing system. These walls are built first, usually with a toothed pattern at the wall ends, and then tie beams and tie columns of reinforced concrete are cast around the panels, serving as confining members. Ashlar stone units certainly qualify for confined masonry, but likely due to the high cost of these, no references were found of CM with Ashlar stones. Regarding confined rubble stone masonry only one experimental study was found, which shows benefits of using confining elements for the improvement of in-plane strength and ductility of the stone masonry walls (Ahmadizadeh and Shakib, 2016).
Unreinforced masonry has, as the name implies, no reinforcements whatsoever incorporated in the walls. Almost all seismic codes worldwide prohibit the use of URM in earthquake zones, unless “additional requirements for unreinforced masonry are in place” (Eurocode 8 1998-1, 2004) such as concrete beams or steel ties. However, this makes the term “unreinforced” somewhat ambiguous, for these buildings can no longer be classified as purely URM, whereas it is not RM either as the reinforcements merely tie the walls together. Figure 3 shows such an example of a school building in a highly seismic zone in Nepal, where the rubble stone walls are strengthened by using cement mortar and the addition of buttresses and horizontal reinforced concrete beams.
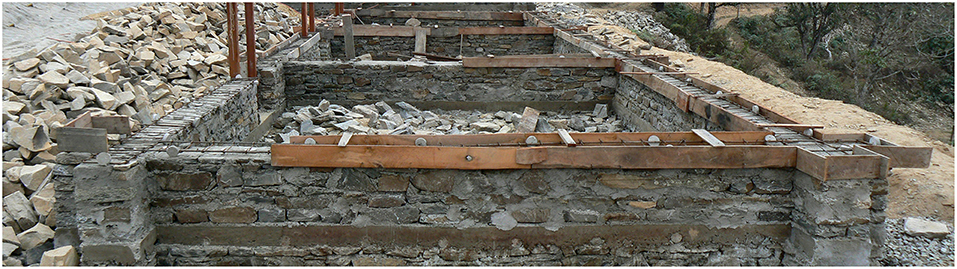
Figure 3. School building in Nepal with rubble stone masonry in cement mortar, brought to courses, and with nominal reinforcements (by courtesy of Smart Shelter Foundation).
The recently developed Multi-Hazard Building Taxonomy GED4ALL (Silva et al., 2018) “enables the user to describe a building by assigning characteristics relevant to its structural response under multi-hazard actions.” One important attribute is the material of the lateral load-resting system, which for masonry is divided in unreinforced (MUR), confined (MCF) and reinforced (MR). Another important attribute is the ductility of the system, divided in non-, low, moderate and high ductility. As this would classify the example in Figure 3 as “unreinforced masonry with low ductility,” it raises the question whether a fourth category should be introduced to further avoid confusion; such as “nominally reinforced, strengthened or semi-reinforced masonry.” An example of a code that addresses this is the Iranian Seismic Code (Standard 2800, 2015), by including a separate chapter called “Provisions for Masonry Buildings with Ties.”
Engineered vs. Non-engineered
During the 12th World Conference on Earthquake Engineering in New Zealand, A.S. Arya presented the often quoted definition for non-engineered buildings as “those which are spontaneously and informally constructed in various countries in the traditional manner, without any or little intervention by qualified architects and engineers in their design” (Arya, 2000).
The first time an appeal was made for the development of separate seismic codes for “unengineered buildings” is by Arya (1977) during the 6th World Conference on Earthquake Engineering in New Delhi in 1977. Due to differences between developed, developing and underdeveloped economies, as well as between rural and urban contexts, he concluded that two types of code specifications were required; one for engineered buildings and one for non-engineered traditional constructions. A workgroup was formed that included Arya and Boen, who may be regarded as the pioneers of researching the seismic behavior of non-engineered techniques. The outcome was the first official guideline fully dedicated to non-engineered construction named “Basic Concepts, part 2: Non-Engineered Construction” (Arya et al., 1980), which was further developed into the well-known “Guidelines for Earthquake Resistant Non-Engineered Construction,” firstly published in 1986 (Arya et al., 1986), reprinted in 2004 (IAEE, 2004), and slightly revised in 2014 (Arya et al., 2014).
Reinforced masonry needs to be designed and calculated by engineers and is therefore categorized as an engineered construction technique. Unreinforced masonry on the other hand, such as stone masonry which is still commonly used in the Himalayan regions, is often referred to as a non-engineered construction type. Whether non-engineers should be involved in earthquake resistant construction of unreinforced and/or nominally reinforced masonry structures is a question on its own, but fact is that engineers are seldom available in the rural areas in developing countries. For that reason, many practical manuals are directly targeting the non-engineered user groups. All building, seismic and masonry codes are per definition engineered publications, as these are meant for qualified engineers and architects, and not for the general public. Publications that introduce detailed explanations and equations about design spectra, dynamic response and base shear forces, such as in Arya (1987b), Tomazevic (1999), and Indian Railways (2017), are also not written for readers without engineering background. On the other hand, design guidelines, technical manuals and on-site construction booklets, from now on referred to as “practical manuals,” may target both user groups and are divided in “E” for engineered and “n-E” for non-engineered in Table 3a.
Building Categories
Stricter design rules may apply for a school building in a high seismic zone on soft soil vs. a house on rock soil in a region with low seismic risk. The Building Category is an important classification, as it defines these restrictions and limitations as well as the level of necessary reinforcements for different types of buildings in different types of contexts. How this building category is determined is also a good indication of the engineering level of the publication.
In some publications, for instance BMTPC (1999), the building category is related to a basic seismic coefficient, which is a combination of seismic zonation, ground conditions and building importance. The zoning data is derived from national seismic zonation maps that represent expected seismic hazard levels based on frequency and intensity of expected earthquakes in different areas. It may require interpolation of the seismic zoning factor (Z), which represents the average peak ground acceleration. Ground conditions can greatly influence the seismic behavior of a building, and the strength and stiffness of soil relates to certain values of geotechnical engineering properties, such as the soil-foundation factor (ß) and the allowable bearing capacity (Na). To obtain and interpret all this specific seismic data requires qualified engineering background. In that regard, a recommendation in Arya et al. (2014) that “soil investigations should be carried out to establish the appropriate allowable bearing capacity” will be outside the scope of its intended target group of non-engineers.
Some publications have introduced a simplified classification system, such as the latest Indian seismic design code IS 4326:2013 (2013), which bases the building category solely on the determination of the seismic zone and the importance of the building, Table 2A. Schools are public buildings with a higher occupancy level compared to houses and are therefore rated with a higher Importance Factor (I), and the zoning can be read from the seismic map of India (IS 1893 (part 1): 2002, 2002). A manual developed for Afghanistan (which is not a code) has merged the two highest zones but has itemized both the seismic zones and the building categories with capital letters, which is highly confusing (Arya, 2003), Table 2B. The Nepali codes have further added a soil classification with just two options (firm and soft). However, for reasons not clearly specified this results in two different sets of building categories in NBC 109:1994 (2007) “Masonry: Unreinforced” and NBC 202:1994 (2007) “Mandatory Rules of Thumb for Loadbearing Masonry,” Tables 2C,D. In NBC202 different terminology is introduced, data is missing, and the important buildings are partially removed, where at the same time the soil class in Category II is not in line with NBC109.
Another possibility is that the Building Category is not mentioned at all, and the publication is presented as a “one-size-fits-all” solution. If no distinction is made between higher and lower seismic levels and importance of building, this general approach may result in excessively reinforced houses in low seismic zones, or worse, in insufficiently reinforced important buildings in an area with high seismic hazard. Or said differently, if the Building Category is not specified, it is not possible to determine suitable design rules that address the different seismic hazards. Regardless of the techniques described, the authors deem such one-size-fits-all publications unsuitable for detailed design and construction purposes and have therefore rejected these from the in-depth review.
Types of Publications
The majority of the practical manuals address the topic of non-engineered construction in a general way and include chapters about different types of masonry, concrete frames, wood construction and earthen structures such as Daldy (1972); ERRA (2007) and Arya et al. (2014). This means the reader constantly has to go back-and-forth between chapters about foundations, general masonry, reinforcements and roofing, while filtering out the relevant lines for stone masonry. The building codes often refer to information that is printed in other codes, outside the actual publication. For example, Indian Standard IS 13828:1993 (2008) refers to IS:1904-1986 (1989) for the foundation, to IS 1893 (part 1): 2002 (2002) for zoning and building categories, then to IS 4326:1993 (2005) “Code of Practice” which refers to IS:1905-1987 (1995) “Unreinforced Masonry,” which in turn refers to IS 1597(part1):1992 (1996) “Code of Practice for Rubble Stone Masonry.”
This back-and-forth paging is not only time-consuming, it also increases the risk that information is misinterpreted or overlooked. In (Arya et al., 1980) the maximum allowed free span for stone walls was found in a footnote under a figure in the general masonry chapter. And in IS 13828:1993 (1993) the diameter for vertical steel reinforcements was found in a footnote under Table 4, which refers to another footnote under Table 3 of that code. Besides, an important question that needs to be raised is whether certain dimensions and specifications for brick masonry can be freely interchanged with applications for stone walls.
To underline the risk of misinterpreting or overlooking of information, the actual content that covers the topic of stone masonry was checked within the 47 practical manuals. The manuals amount to a total of 4,417 pages front-to-back, which includes forewords, acknowledgments, tables of content, lists of figures, glossaries, abbreviations, appendices, reference lists etc. (18%). The remaining actual text can then be separated from background information that is irrelevant for stone, as well as chapters about different techniques like wood, earth and retrofitting (48%). The remaining chapters for masonry are then divided in relevant background information such as zoning, soil conditions and building shape (8%), masonry in general (15%), and finally into pages that are specifically dedicated to stone masonry, either in cement mortar (8%) or mud mortar (2%).
Overall, the relevant content about stone masonry within the 47 manuals is about 10%, meaning that the reader needs to go through 90% of additional text, in order to filter out the relevant information that is needed. A “Stand-Alone” (SA) stone masonry publication, or a publication that has a clearly separated chapter solely dedicated to stone masonry, will prevent any possible confusion. However, not one Stand-Alone building code exists for stone masonry, and only 11 out of 47 practical manuals are marked as “SA” in Table 3b.
Eligibility of the National Seismic Codes
Based on the definitions and search criteria as described, an initial review was carried out on a total of 109 national building, seismic and masonry codes from 48 countries. These include countries that either had a rich heritage in stone masonry in the past, or where variations of the technique are still in use, such as in Algeria, Morocco, Peru, Croatia, Greece, Italy, Portugal, Iran, Turkey, Armenia, Tajikistan and Uzbekistan, to name a few. The review focused on the following parameters: i) Stone masonry publication; ii) For design and construction of school buildings; iii) Built in rubble stone masonry with cement mortar; iv) With nominally reinforcements added to the loadbearing system; v) According to clearly defined Building Categories. Generally, building codes are meant for engineers, and no Stand-Alone code for stone masonry was found. It also became apparent that the detailed review could be limited to just the Himalayan region, for three reasons:
The first reason is that hardly any country in the world currently permits the use of rubble stone masonry in high seismic zones. This is the case for all South American, European and almost all Middle Eastern countries. The use of rectangular stone units (Ashlar) may be permitted for reinforced and confined masonry in some countries, but for rubble stone the rules are in between “very restricted” to “not allowed at all.” For instance, the leading European seismic norm Eurocode 8 1998-1 (2004) refers to Eurocode 6 1996-1-1 (2005) “Design of Masonry Structures” for specifications of the masonry units. Here it is described that only dimensioned stone units are acceptable as specified in EN 771-6:2011 + A1:2015 (2015) “Natural Stone Masonry Units,” meaning that the Eurocodes prohibit the use of newly built squared rubble and random rubble stone masonry in seismic areas for all of Europe. Only Italy makes an exception by largely incorporating the regulations of their 1987 masonry code (Decreto Ministriale, 1987) into their Italian seismic code NTC 2018 (2018). Italy allows natural stone (materiale lapideo) such as volcanic tuff stone, limestone and granite for masonry; but only in their lowest seismic zone 4 and use of cement mortar is mandatory in any case.
The second reason is the risk of wrong interpretation of the codes when these are written in the local languages. In many Central Asian and Middle Eastern countries masonry is regarded as a structure with stone-like units, either from natural stone, fired bricks, concrete blocks, or combinations of these, which may cause confusion during the translation of the code. Misunderstanding of the local context can occur when bylaws or local regulations are not mentioned in the publication. The Iranian Seismic Code (Standard 2800, 2015) is one of very few codes that does permit buildings in rubble stone masonry, even in their highest seismic zone 1. However, all schools in Iran are designed and constructed by the Organization of Renovation, Development, and Equipping of Schools under the Ministry of Education, who are in a position to deviate from the codes and may apply different regulations for the urban or the rural settings.
Thirdly, the majority of the practical design and construction manuals that were found (32 out of 47), are written for the Himalayan region. For these three reasons the seismic code review, further on, limits itself to the codes of Afghanistan, Bhutan, China, Pakistan, India and Nepal, which are also among those countries with the highest seismic hazard in the world.
Eligibility of Practical Manuals
Based on the definitions and search criteria as described, an eligibility check was carried out on a total of 47 relevant practical construction manuals that are published between 1972 and 2017, focusing on the following parameters: i) Stone masonry publication; ii) For design and construction of school buildings; iii) Built in rubble stone masonry with cement mortar; iv) With nominally reinforcements added to the loadbearing system; v) According to clearly defined Building Categories; vi) Specifically targeted for Non-Engineers; vii) Preferably a Stand-Alone publication.
As an additional parameter the stone content was analyzed to determine to what extent all the necessary design and construction requirements are addressed, as an indication of the “technical completeness” of the publications. Following the example of Papanikolaou and Taucer (2004), who conducted a literature review on the topic of non-engineered houses in Latin-America, a point system was developed for fair comparison of 10 main topics, by dividing 78 points over 73 items (marked as xitems/xxpts). Certain items, such as main dimensions, openings and reinforcements, were given more weight in this completeness analysis, which roughly amounts to 70% for main dimensions vs. 30% for construction quality related issues. The 10 main topics are: (i) overall building dimensions, 6 items/10 pts; (ii) foundation, 8 items/7 pts.; (iii) wall dimensions, 4 items/9 pts.;( iv) masonry and mortar, 9 items/8 pts.; (v) buttresses, 3 items/5 pts.; (vi) openings in walls, 8 items/9 pts.; (vii) horizontal reinforcements, 14 items/9 pts.; (viii) material specifications, 9 items/6 pts.; (ix) vertical steel reinforcements, 7 items/7 pts.; and (x) roof construction, 5 items/8 pts. The overall scores are included in Table 3e.
When combining all the above parameters, it is concluded that out of 47 publications only one manual (Desai et al., 2012) qualifies for the exact given parameters, and only one more manual has a Stand-Alone chapter for stone masonry (Arya, 2005). Both publications cover houses as well as schools, but the difference between these categories is clearly defined. Overall only three manuals are specifically drafted for school buildings (Arya and Chandra, 1982; Arya, 1987a; Bothara et al., 2002), but in these the overall theme is brick and block masonry, with just a few additions for stone. The eligibility check shows a clear division of three groups, as presented in Table 3.
Group 1 includes 22 rejected manuals, of which 19 are directly excluded because the Building Category is not specified, Table 3d, of which 7 are post-earthquake reconstruction manuals for India and Nepal. Two manuals (GSDMA, 2001; DMMD, 2007) do describe the Building Category, but apply the one-size-fits-all approach to all techniques, which is highly confusing (marked with “?”). Manuals that score lower than 50% on technical completeness, Table 3e, are deemed unsuitable for practical use and were also rejected from the review. Among these rejected manuals are two Stand-Alone stone publications (Murty, 2002b; Bothara and Brzev, 2011) and one Stand-Alone reconstruction manual for post-earthquake Nepal (Pandey et al., 2017). Most unfortunate is the exclusion of Bothara et al. (2002), which is specifically drafted for design of school buildings in developing countries. The Building Categories are well defined and it scores highest with 88% completeness. But unfortunately these guidelines are not for rubble stone with cement, as the manual only covers field stone with mud, bricks with mud and bricks with cement. The characteristics of group 2 and group 3 are explained in detail in the chapters ahead.
It is very important to note that the technical completeness is certainly no indication of the value, nor the validity of the stated information. It merely gives insight in how often and detailed the various elements are addressed in the literature, if at all. More insight about the value of the information provided about each of the sub-items is addressed in detail in the technical review for groups 2 and 3, further on.
Review of National Seismic Codes
The eligibility check and initial review of the codes revealed that the majority of the practical design and construction manuals are written for the Himalayan region. Outside this region, only few countries allow stone in low seismic areas, and currently only the code of Iran allows rubble stone masonry in their highest seismic zone, but no practical manual was found for the Iranian context. It was therefore decided to limit the in-depth seismic code review to the codes of Afghanistan, Bhutan, China, Pakistan, India and Nepal.
Seismic Codes of Afghanistan, Bhutan, China, and Pakistan
In Afghanistan stone masonry is still widely practiced, but the country does not have its own seismic or masonry codes and follows either the Russian code (SNiP II.7.81*, 1981) or refers to the International Building Code. IBC 2015 (2014) refers to ACI 530/530.1 (2011) “Specification for Masonry Structures”, which describes stone masonry only for empirical design and only for use in the lowest Seismic Design Category A, which does not need any seismic provisions. This means that for any seismic level that requires seismic strengthening, stone masonry is not allowed according to the IBC.
Bhutan, another country with a rich past and present culture of stone masonry, does not have a seismic code of its own and refers to the Indian seismic codes. Stone masonry is also not included in the 2002 Building Rules (BBR-2002, 2003) or the Rural Construction Rules (RCR-2013, 2013) which for masonry in general both refer to the Traditional Architectural Guidelines (Royal Government of Bhutan, 2014). These guidelines however focus solely on aesthetic features such as building shape, roof form, window ornamentation and such, without any consideration for structural stability of the building.
The Chinese seismic code GB 50011-2010 (2010) does allow stone masonry in “fortification intensity levels 6, 7 and 8”, as described in a one-page section for stone houses. Where the 2001 version (GB 50011-2001, 2002) only permits confined masonry with dressed stones, the 2010 version is more generous and allows confined masonry with cut stone, as well as with rubble stone. Unreinforced or nominally reinforced rubble stone masonry is not allowed in the national standards that apply to all of China, and (mainly due to the language barrier) only one practical guideline was found that describes confined masonry with stone for rebuilding after the 2008 Sichuan Earthquake (Construction Standard 132, 2008).
Pakistan never had any provisions for stone masonry, nor for low-strength masonry in general. As early as in the Quetta Building Code of 1937 (QBC-1937, 1937), developed after the very heavy and damaging Quetta earthquake in 1935, it is clearly stated that “dry masonry without mortar is strictly forbidden and stone boulders may on no account be used”. This statement is repeated once more in the Quetta Building Rules of 1976 (G.P.(Q)23-3,100-10-77, 1976), yet stone is still widely used, especially in the Northern parts of the country. In 2015 it was estimated that roughly 5% of the total building stock of Pakistan continues to be built with stone (Lodi, 2015), which is around 1.25 million units, mostly located in the Himalayan region. After the 2005 Kashmir earthquake, which left nearly 3.000.000 people homeless, it was observed that a traditional construction type called Bhatar, made of dry-stacked loadbearing stone walls with horizontal timber lacing, resisted the devastating earthquake very well (Carabbio et al., 2018). But structurally this system behaves different than loadbearing masonry with mortar.
Seismic Codes of India
The Indian Standard IS 1893 (part 1): 2002 (2002) “Criteria for Earthquake Resistant Design of Structures” is the main seismic code that deals with the assessment of seismic loads and which defines design factors and the seismic zones. Mohapatra and Mohanty (2010) describe that the first Indian zonation map divided the country into 7 zones in 1962 (VI-0), which was brought back to 5 zones (V-I) after the Konya earthquake in 1967, and then further merged to the current 4 zones (V-II) in 2002 (Table 2), meaning that today all of India is subjected to seismic hazard levels. With regards to stone masonry, IS.1893 states that “in highly seismic areas construction of a type which entails heavy debris and consequent loss of life and property, such as masonry, particularly mud masonry and rubble masonry, should preferably be avoided.”
For guidance on earthquake resistant construction of buildings, IS.1893 refers to Standard IS 4326:1993 (2005). The first print of this “Code of Practice for Earthquake Resistant Design and Construction of Buildings” (IS:4326-1967, 1968) was developed in 1967 and allowed random rubble stone masonry, but its successor of 1976 does not recommend rubble stone “unless it cannot be avoided” (IS:4326-1976, 1977). For that case certain dimensions and strengthening additions are given, but the most vital information is missing, such as wall thicknesses, free span of walls, and the need for buttresses. These need to be calculated by following IS:1905-1969 (1975) for “Unreinforced Masonry” and IS:1597(part 1)-1967 (1973) “Code of Practice for Rubble Stone Masonry” for general stone properties. Since the first edition of 1967, IS.4326 forbids the use of mud mortar for rubble stone masonry in any case.
In 1993, IS.4326 is revised drastically by introducing Building Categories related to the basic seismic coefficient (αh), which is a combination of seismic zonation (V-I), soil-foundation factor (ß) and importance of building (I) as per IS.1893. The highest Building Category E requires maximum provisions for strengthening whereas category A requires the least. Regarding stone masonry, IS 4326:1993 (2005) only covers the use of rectangular stone units (Ashlar) and from here onward, rubble stone is addressed in the newly published seismic code IS 13828:1993 “Improving Earthquake Resistance of Low Strength Masonry Buildings”. With this introduction India is “perhaps the first country to have developed codes on low-strength non-engineered masonry constructions” (Jain, 2000). Here low-strength stone masonry is described as “random rubble; uncoursed, undressed or semi-dressed stone masonry in weak mortars; such as cement-sand, lime-sand and clay mud.” There is a bit of contradiction between the supposed non-engineered level of this code, as the interpretation and application of design criteria still demands rather advanced engineering skills. The code is similarly structured as its predecessors IS:4326-1967 (1968) and IS:4326-1976 (1977), but it describes all elements in more detail, with corrections and with added information that was previously missing, such as maximum dimensions and thicknesses. IS.13828 clearly defines that low-strength masonry is not permitted in building category E and should be avoided in category D. The code also explicitly states that these constructions should not be permitted for important buildings with importance factor ≥1.5. This concludes that school buildings in low-strength masonry (two main search criteria of this literature review) are not allowed to be built anywhere in India.
Seismic Codes of Nepal
The first Nepalese set of National Building Codes was drafted in 1993-1994 after the 1988 Udayapur earthquake in the east of Nepal. They are all based on the Indian codes and the Nepali codes of interest for stone masonry are NBC 105:1994 (2007) “Seismic Design of Buildings,” NBC 109:1994 (2007) “Masonry: Unreinforced,” NBC 202:1994 (2007) “Mandatory Rules of Thumb for Loadbearing Masonry” and NBC 203:2015 (2015) “Guidelines for Earthquake Resistant Construction: Low Strength Masonry.” NBC 105 is a similar document as IS.1893, describing design loads, the seismic zoning of Nepal (factor Z based on contours), soil conditions (rock, medium, soft, weak) and the importance factor for buildings (1.5 for schools). NBC109 only covers masonry with rectangular units (Ashlar in the case of stone) and the application of rules is meant for qualified engineers.
With regards to rubble stone masonry it all becomes very confusing. NBC202 is meant for “mid-level engineers” and covers buildings that are “not normally engineered” and which are constructed of “fired brick or stone masonry, either in cement or mud mortars.” This should add up to 4 techniques, however NBC202 only specifies 3 classes as follows:
i) “Up to three-storied load-bearing brick (and other rectangular building units) masonry buildings constructed in cement mortars”;
ii) “Up to two-storied load-bearing stone masonry buildings constructed in cement mortar”;
iii) “Up to two-storied load-bearing brick masonry buildings constructed in mud mortar.”
The following is noted. Class i) in NBC202 seems similar to NBC109, but they differ between the engineered vs. non-engineered approach. However, this only becomes apparent after reading NBC 000:1994 (2007) “Requirements for State-of-the-Art Design: An Introduction,” which introduces these different user levels. For class ii) no requirements or properties are described for the stone units (Ashlar or rubble); this is only done for bricks. Rather than in NBC202, class iii) is covered in NBC203, which is specifically meant for low strength masonry that includes “non-erodible walling units such as stones, burnt clay bricks … with mud mortar as a binder.” Lastly, no class iv) for stone masonry in mud mortar is specified in NBC202, but the title of the stone masonry chapter all of a sudden specifies “one-and-a-half-story buildings in mud.” This is further contradicted in NBC203 which allows two-story buildings plus attic for stone with mud.
As noted previously, more discrepancy is found in the determination of Building Categories between NBC109 and NBC202, as shown in Tables 2C,D. NBC202 defines the highest category I as “Important Buildings on Soft Soil in Zone A,” whereas categories II, III and IV are solely meant for Residential-cum-Shop Buildings, and Important Buildings are no longer specified. The code also mentions that buildings in class I must always be designed by engineers, which contradicts with the intended typology of non-engineered buildings and the intended target group of “mid-level engineers who are not trained to undertake independently the structural design of buildings.” Therefore, intentionally or not, the category of school buildings in rubble stone masonry with cement mortar is strictly spoken not covered in the Nepali codes.
Legal Status
An important note must be made about the legal status of the Indian and Nepali codes. The first edition of IS:1893-1962 (1962) was titled: “Recommendations for earthquake resistant design of structures,” and the first edition of IS:4326-1967 (1968) was an adjunct to that. In none of the further codes the legal state of the document is clearly addressed, and after the Bhuj earthquake of 2001 a call for stricter regulations was made. Yet as of today these codes are not mandatory from the state level. The latest National Building Code of India IS SP7:2016 (2016) has incorporated the latest seismic standards, but this code itself indicates that “it is non-statutory in nature and is intended to serve as a model for adoption by Public Works Departments, local bodies and other construction agencies.” Especially in urban areas it has become more common to acquire some kind of certification. For instance, to obtain a housing loan, a bank may require certain proof of code compliance, thus currently giving the Indian seismic codes a status “between desirable and mandatory.”
The Nepali codes all largely follow the Indian codes and have been reprinted a few times over the years with no revisions or amendments. The only difference is an added disclaimer on the cover page stating that “this publication represents a standard of good practice and therefore takes the form of recommendations. Compliance with it does not confer immunity from relevant legal requirements, including bylaws.” In some municipalities, architects and engineers must submit a detailed (structural) design report (DUDBC, 2018), or else no building permit will be issued. Similar to the Indian situation the codes are indirectly mandatory, but if and how this is enforced, especially in the rural areas, is unclear.
In a document from 2009 titled “Recommendation for Update of Nepal National Building Code” (UNDP/ERRRP-project: NEP/07/010, 2009) all the above-mentioned issues and discrepancies are noted, such as the need for code enforcement, as well as “the requirement for technical and literature editing to eliminate errors and misprints.” It recommends the replacement of NBC202 (Mandatory Rules of Thumb) with standard designs of typical buildings that comply with the codes. It also recommends making NBC203 mandatory for all types of low-strength masonry. However, the updated 2015 version of NBC203, so far the first and only updated code since 1994, has fully excluded the most important design criteria for zoning, soil quality and importance of the building. It does not define any Building Categories and has become a one-size-fits-all publication, which does not offer solutions that address the different seismic hazard levels in the country.
Compatibility of Housing Designs with the Seismic Codes
Although the review of houses is not the aim of this paper, the following brief comparison gives an interesting insight in the compatibility and practical application of the seismic codes. After exclusion of drafts and duplicates, group 2 contains 8 manuals that are rejected because the Building Categories are not defined. However, these manuals have two interesting things in common. Firstly, they all are meant for Himalayan countries that still build with stone (Pakistan, Bhutan, Nepal) and secondly, they only address housing solutions (Table 3c).
Table 4 clearly shows that (i) there is hardly any agreement between both codes; (ii) the manuals are not in line with the codes on almost all points, and (iii) there is also lots of disagreement between the manuals themselves. This is basically the case for all main dimensions such as for lengths, heights, thicknesses, openings and reinforcements. For instance, both codes recommend a maximum free span of walls of 5.0 m, whereas the manuals give values ranging from 4.5 to 7.0 m. And while NBC202 defines that houses should be built only one story high, all manuals that refer to this Nepali building code recommend heights between 2 and 3 stories.
Most striking are the differences between the ERRA (2006b) and ERRA (2007) manuals for Pakistan, both published within 1 year by the same organization, but seemingly compiled by two completely different teams. Also noticed are the discrepancies between the DUDBC (2015) building catalog and the JICA (2016a) manual for house owners, which are both based on the exact same building codes and housing plans. Such differences may arise when information for general masonry is copied into stone masonry chapters, which is either incompatible, or perhaps has been altered somewhere along the line. But other than speculation about careless copy-pasting of wrong data, lack of expertise by the publisher, or overcautiousness after a disaster, no clear indication was found why the manuals deviate from the codes they refer to, and why they all publish such highly contradicting information.
Technical Review of the Practical Manuals for School Buildings
Group 3 represents the remaining 13 practical manuals that are eligible for the in-depth comparison and review of school buildings in rubble stone masonry with cement mortar. This is remarkable, as all manuals in this group refer directly or indirectly to the principles of the Indian seismic codes, while these codes explicitly prohibit the use of rubble stone masonry for school buildings in any seismic zone.
The two manuals for Educational Buildings (Arya and Chandra, 1982; Arya, 1987a) are brought back to just one, as they are almost identical. It is noted that the information in these contradicts at almost all points with the other manual Arya published in the same year (Arya, 1987b). Furtherly, the three manuals named “Guidelines for Earthquake Resistant Non-Egineered Construction” (Arya et al., 1986, 2014; IAEE, 2004) are also merged into one review, as they are nearly identical with regards to the stone masonry chapter. It means that this particular information has not changed for over 30 years since 1986. With removal of drafts and duplicates, a total of 9 practical manuals are included in the in-depth technical review.
Noteworthy is that 7 out of these 9 manuals [from now on marked as (xx/xx)] are written or co-written by Arya. He played a major role in drafting the very first manual for non-engineered construction named “Basic Concepts” (Arya et al., 1980), followed by the three “Guidelines for Earthquake Resistant Non-Egineered Construction” manuals. These are the most referred-to manuals of this review, and even the Indian code IS.13828:1993 and the Nepali code NBC202:1994 make a reference to these. Given the high influence of one author on this particular subject, and the fact that all manuals constantly refer to the same main sources over and over, it is remarkable to note that each manual presents different facts and information, as shown in Table 5. This overview starts with a clear lack of consensus on one of the most important parameters, being the maximum allowed Building Categories in which stone masonry is permitted (marked with *).
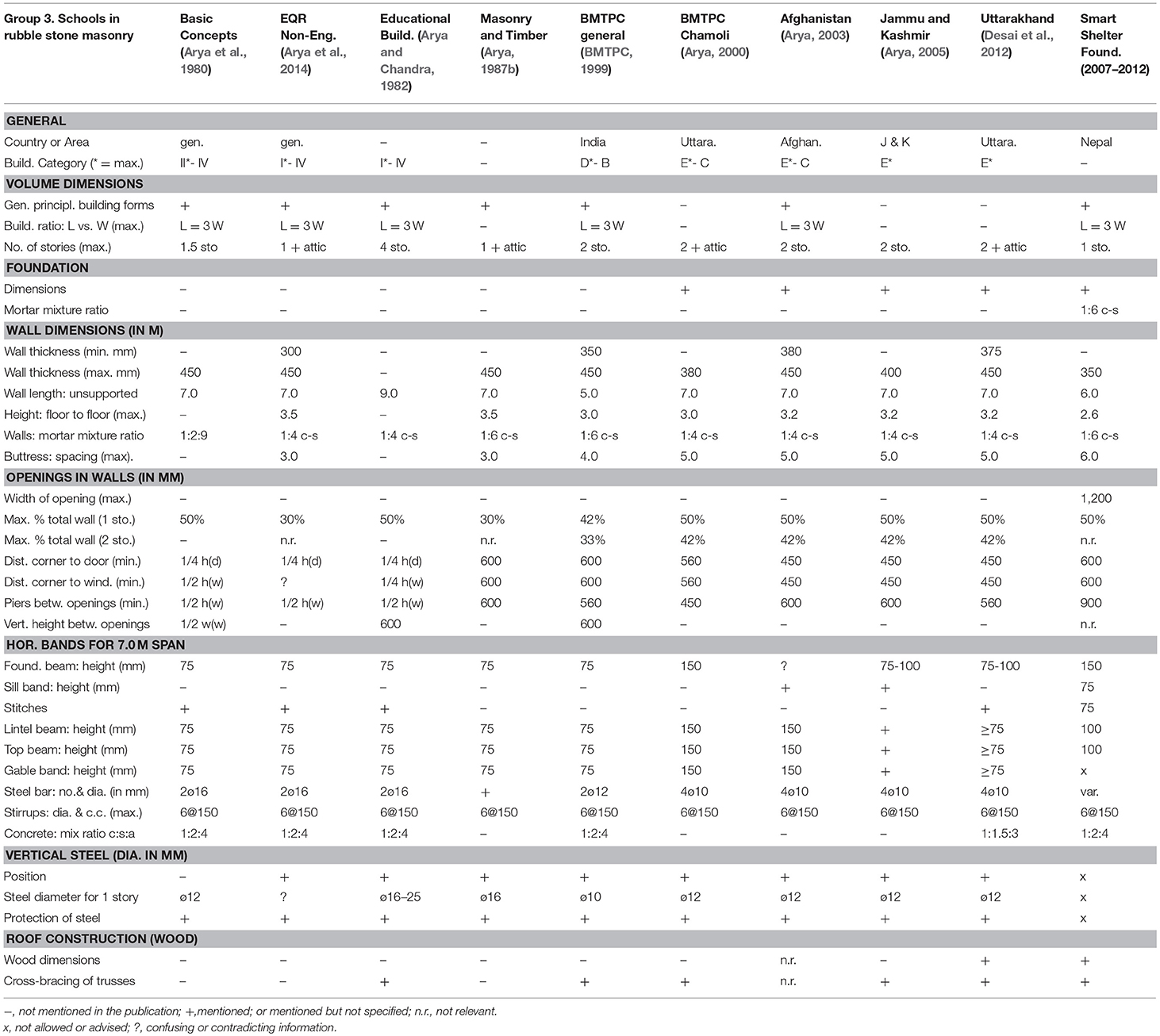
Table 5. In-depth technical review for school designs according to the practical manuals of group 3.
The next paragraphs highlight some further differences and discrepancies, as well as similarities and other notable facts between the publications. As a reference for the comparison of elements, the most generally recommended unsupported wall length of 7.0 m is chosen. Brief remarks are made regarding the actually built schools in Nepal by Smart Shelter Foundation, included in the last column. Also, some remarks are included about a set of 12 post-disaster school designs in rubble stone masonry that are made available online by the National Reconstruction Authority (NRA, 2018), of which 5 are approved by the Ministry of Education in Nepal.
Overall Building Dimensions
The maximum ratio of width vs. length for the overall dimension of the building volume is only mentioned in (25/47) but the majority (20/25) agrees on L = 3 W, although L = 2 W, L = 4 W and even L = 3.5 W were spotted as well. There is no consensus in group 3 on the maximum height of the building, where five different options are noted; 1+attic, 1.5 story, 2 stories, 2+attic, and 4 stories.
Most manuals include some guidance on site considerations (31/47) and on building shapes (33/47). Separation between building volumes by creating a gap is explained in (25/47) but varies from 1.5-15 cm. Detailed building plans however are seldom found. Illustrations are generally limited to one-box-type examples (such as Figure 4A) to explain mechanisms or to point out elements. The JICA (2016a) housing manual and DUDBC (2015) building catalog have very nicely detailed illustrations and isometric views of houses, but nothing similar was found for schools.

Figure 4. Principles of (A) intermediate buttresses (following Desai et al., 2012). (B) buttresses at all ends of walls (following NBC 202:1994, 2007). (C) buttresses only at ends of short walls (following NBC 202:1994, 2007). (D) buttresses at all ends of walls and at intermediate points (following NRA, 2018; all by courtesy of Smart Shelter Foundation).
Foundation
The least covered and most incomplete topic of all, the foundation is not even mentioned in (20/47). Only (4/9) in group 3 have included some general information about width, depth and shape, and/or have specified this for different soil types. But not one makes any distinction for multi-story buildings, mentions application and function of a firm layer in the bottom, has defined the minimum height above ground level, or mentions anything about drainage around the building. Waterproofing or a damp-proof course (DPC) on top of the foundation is mentioned in (5/47), but none specifies exactly how to apply this.
Most surprisingly, no mortar specifications for the foundation are given at all in group 3, and neither in (37/47) overall. Within the remaining (10/47) some recommend mud mortar below ground level, where others specifically prohibit mud.
Wall Dimensions and Specifications
There is no consensus on the wall thickness, with a minimum ranging from 300 to 380 mm and a maximum from 380 to 450 mm. The maximum free span highly varies between 4.5 and 9.0 m, although the consensus is 7.0 m (7/9). However, it is seldom clear what exactly is defined: the distance between the interior sides of two cross-walls, or the center-to-center dimension. The maximum wall heights in group 3 vary between 3.0, 3.2 and 3.5 m, but also here it is seldom clarified from exactly where to where this is measured; free interior height or center-to-center of floors.
Many varieties are specified for the mortar of the rubble stone masonry, such as cement-sand mortars, lime-based mortars and even mud, whereas group 3 is divided between 1:4 or 1:6 cement-sand mixtures. ADPC (2005) notes that “the thickness of mortar plays a vital role in the strength of masonry and should be optimum. Thin mortars cannot bond the units properly, and thick mortar makes the wall weaker.” However, the thickness of the joints is only mentioned in (4/47), ranging from 8 to 25 mm. Only (3/47) manuals specify that freshly mixed mortar must be used within 25 min to 1 h. Plastering of walls is also seldom mentioned (7/47).
Almost all manuals include items related to construction quality, such as how to lay the stones in coursed and level layers of 60 cm lift, or how to place and overlap them in corners and sections. Few manuals warn against use of round boulders, and just some specify stone dimensions, such as 450 × 275 × 150 mm in MWHS (2014). Most recommended is the use of bond stones in a staggering pattern of 1.2 m horizontally and 0.6 m vertically (37/47).
Buttresses
Only (27/47) have included information about buttresses. Both Indian and Nepali codes mention that buttresses are required only when the maximum allowed free wall span is exceeded. But the Indian codes locate these at intermediate points (Figure 4A), whereas NBC 202:1994 (2007) shows buttresses at all wall ends of corners and T-sections (Figure 4B), which is contradicted by another illustration of a “correctly buttressed single-story school-building”, with buttresses only at the short walls (Figure 4C). That particular example also contradicts its own recommendations for dimensions next to openings and minimum width of piers (explained in the next paragraph). Such discrepancies may lead to confusion in school designs (Figure 4D), as noticed in the examples that are published online by (NRA, 2018) after the 2015 earthquake in Nepal. Furtherly, not one manual explains how to place stones into the buttresses in order to create good bonding with the wall, nor describes the effect of placing openings directly next to buttresses, as in Figures 4A,C.
Openings in Walls
This section explains different methods to determine the maximum dimensions of openings in a wall, and the implications when these maximum values are exceeded.
Maximum Dimensions of Openings
Arya et al. (2014) explains that “openings tend to weaken the walls, and the fewer the openings, the less the damage suffered during an earthquake.” Therefore, a maximum percentage is determined for the total length of openings, divided by the total length of the wall panel. For single-story buildings the group 3 manuals (6/9) define different values of 30, 42, and 50%, and for more stories these values decrease. It must be noted that maximum 30% of opening length is very limited. If we follow NBC 202:1994 (2007) with maximum free span of 5.0 m, this means that just one opening of 1.5 m per side of the room is possible. To install two smaller openings of 0.75 m width is not likely, as also the thickness of the wooden frame must be deducted, with the result that insufficient daylight will enter the room. Door openings must certainly be wider than that.
Another approach is to define minimum dimensions for wall lengths, needed for the following 5 situations: from corner to door, corner to window, pier between door and window, pier between two windows, and vertical height between two openings. Some manuals specify fixed values ranging between 230 and 900 mm, whereas others calculate the wall length as a quart or half of the adjoining opening height. In some cases the measurements are taken from the insides between the cross-walls, in others from center-to-center of walls, or even from the outside corner of the building. Clearly the Indian codes (Figure 5A) and Nepali codes (Figure 5B) show a different approach for the wall dimensions around the interior walls. The reader must also pay attention to the different types of notation, such as > or ≥ or ≧ or ≯. Overall, it results in numerous variations without any consensus or consistency whatsoever.
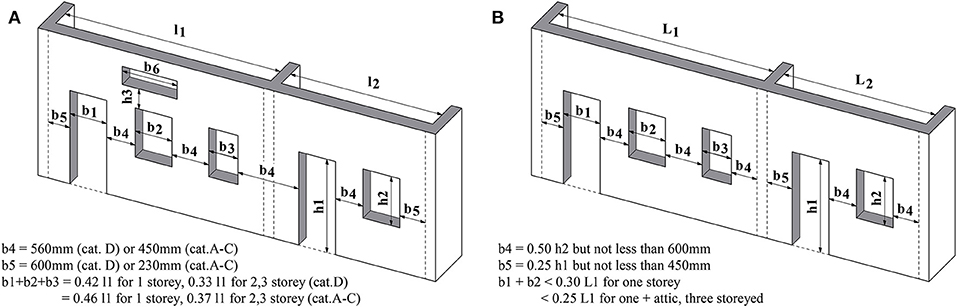
Figure 5. Principles of dimensions of openings according to (A) Indian seismic codes (following IS 13828:1993, 1993) and (B) Nepali seismic codes (following NBC 202:1994, 2007; both by courtesy of Smart Shelter Foundation).
Boxing of Openings
Both Indian and Nepali codes dictate “boxing of openings,” but only when the opening size exceeds the allowed dimensions. For such case, IS 13828:1993 describes that openings “should be strengthened by providing reinforced concrete lining with 2 high strength deformed (HSD) bars of 8 mm dia.,” but without any mention or specification for the stirrups. According to Arya (2000) such frames “will not be as effective in aiding the shear wall action unless properly connected to the walls through shear keys”. In total (19/47) have included a variation of Figure 6A, but this concept of boxing is meant for brick masonry. Not one manual describes in detail how to execute this for rubble stone masonry, such as masonry pattern and interlocking of stones. NBC 202:1994 (2007) puts extra steel rods inside the teeth, but without any reason or further specification.
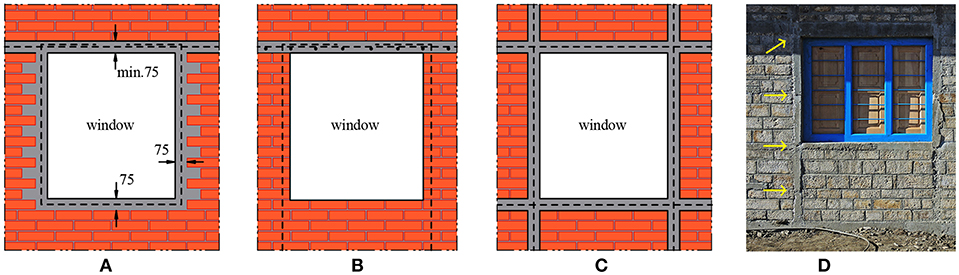
Figure 6. Principles of boxing of openings with (A) concrete frames with shear keys (following IS 13828:1993, 1993). (B) vertical bars (following NBC 202:1994, 2007). (C) continuous vertical posts and horizontal beams (following NRA, 2018). (D) Practical example of vertical posts next to openings. (A–C by courtesy of Smart Shelter Foundation, D by courtesy of Preci).
NBC 202:1994 (2007) also shows an alternative detail, Figure 6B, in case “the vertical opening of the wall is more than 50% of the wall height.” This rule certainly applies to all doors, as they literally divide a wall into two portions, but only (1/47) follows this advice. The figure shows vertical bars that must be installed in the jambs, but without any steel specifications or further detailing. The latest trend is to include concrete posts next to windows, all the way from the top beam to the foundation beam (Figure 6C), as noted in 6 of the 12 school designs published by NRA (2018). However, the need for this is not described in any code or manual. Figure 6D show such example, which is built without sill beam and without the tooth connection. The builders told the authors that it created all kinds of practical issues, such as accumulation of debris falling into the gap, and that proper casting was only possible in lifts of 60 cm. This creates interruptions in the posts, which likely results in insufficient strength of these elements. Furtherly, recent testing on confined masonry panels by Singhal and Rai (2017) show that a continuous sill beam under an opening behaves much better than continuous vertical posts next to openings. This should be verified for nominally reinforced stone masonry as well.
Horizontal Reinforcements
The Nepali code NBC 202:1994 states that “the most important horizontal reinforcing is by means of reinforced concrete bands provided continuously through all load-bearing longitudinal and transverse walls, at plinth, lintel and roof-eave levels, and also at the top of gables.” This section is focusing on beams of reinforced concrete (whereas thinner members are often referred to as bands), although alternatives can be made of wood, steel and bamboo.
The importance of horizontal reinforcements is not disputed by any of the codes or publications, but their positions and dimensions certainly are. Figure 7 shows the evolution of different principles over the years. The majority of publications follow the recommendations of the Indian and Nepali codes, such as Arya (2000); Arya et al. (2014) and include only the lintel and top beam (Figure 7A). Gradually over time, some manuals start to add more reinforcements such as the plinth beam, sill band (Figure 7B) and stitches (Figure 7C). The older manuals often had included small concrete columns at all corners, but over time these have disappeared, or have been replaced by buttresses or vertical steel bars (not included in these illustrations). NBC 203:2015 (2015) for low-strength masonry is the only code that recommends all these extras and shows maximum 5 levels of reinforcement in a wall panel (without gable), while NBC 202:1994 (2007) only shows 2 beams. Therefore, it is surprising to see that many designs after the 2015 Nepali earthquakes incorporate reinforcements at 6 or even 7 levels (as published online by NRA, 2018), sometimes at intervals of <300 mm in height, for which no logic or explanation is found by the authors (Figure 7D). Besides, these designs are approved by the Nepali Government, which goes directly against the building codes as dictated by the same government, that prohibit school buildings in rubble stone to begin with.

Figure 7. Principles and evolution of adding horizontal bands with (A) 2 beams and gable band (following Arya, 1987a). (B) 4 beams and gable band (following ERRA, 2006b). (C) total 5 beams & stitches (following Schildkamp, 2015a). (D) total 7 beams & stitches, plus gable band and full vertical posts next to openings (following examples as published online by NRA, 2018; all by courtesy of Smart Shelter Foundation).
Plinth Beam
Both Indian and Nepali codes clearly mention that the beam resting on top of the foundation only needs to be provided for masonry strip footings in soft soils, or “when the soil is uneven in its properties, as frequently happens in hill tracts” (IS 13828:1993, 1993). All (9/9) agree that a plinth beam is not needed in firm or rock soil, whereas (3/9) further mention that the plinth beam is not critical to begin with. The ADPC (2005) manual of group 2 however, has interpreted the “foundation band” as a continuous reinforcement in the bottom of the foundation.
Lintel Beam and Top Beam
The lintel beam is generally seen as the most important beam of all (9/9), which continuously runs directly over all openings. Together with the top band, also known as floor, eave or roof band, these beams add the most to the box-action of the building, by preventing separation of wall connections and out-of-plane failure of the walls. If the floor height is limited, meaning there is only a thin layer of spandrel masonry on top of the lintel, some manuals recommend combining lintel and top beam into one. Arya et al. (2014) specifically mentions this for walls with <2.5 m floor-to-floor height.
Sill Band and Stitches
There is not much agreement about the need for the sill band under the windows and/or stitches in corners and T-sections. The sill band is only recommended for the highest Building Category and in just (2/9), but both for different reasons; Arya (2003) demands it only for 2 to 3 story buildings and Arya (2005) for buildings with a high Importance factor. The main critique on the sill band is that it is not continuous due to disruption of door openings, although ERRA (2007) states that it is “best to provide continuous sill band also”, which simply is impossible. It is interesting to note that a thorough inspection of all 15 Smart Shelter Foundation school projects (either in stone or block masonry) after the 2015 earthquakes, revealed only some minor hairline cracks in 8 schools, right between the sill beam and the masonry on top of it. This is likely caused by slight rocking of the heavy masonry piers, which may be avoided by inserting dowels between the bands and the masonry. The fact that the damage was insignificantly minor also suggest that the horizontal reinforcements played an important role to avoid shear cracking in the piers and spandrel masonry, but all these assumptions need further in-depth validation.
Stitches are mentioned in (5/9) and many alternatives are given such as concrete bands, wooden ladders and steel dowels or metallic mesh in horizontal joints. Desai et al. (2012) describes stitches only for the highest seismic zones, and Arya and Chandra (1982) recommends stitches at every 40 cm lift. Arya (1987a) explains that dowels can be used as an alternative for the lintel in the lower seismic zones at 60 cm intervals throughout the height of the wall, and in the higher zones stitches can be added at sill level in addition to the lintel beam.
Gable Band
The gable band is mentioned in all (9/9) of group 3, in case a masonry gable is built. Some manuals place stone gables only on the end walls (Figure 7A) and others on the cross walls as well (Figure 7B). However, the more recent manuals such as (Desai et al., 2012) and NRA (2017) recommend a fully trussed roof with light wooden gables, as heavy masonry gables, even with gable band, still have the risk of toppling during an earthquake. The roofs of Smart Shelter Foundation are also built this way (Figure 7C).
Dimensions of Concrete Beams and Steel Reinforcements
All (9/9) agree that the beam dimensions and their required steel reinforcement depends on room span, importance of building and number of stories. But at the same time all manuals follow a general one-size-fits-all approach, similar to IS 13828:1993 which recommends minimum 75 mm thickness of beams with 2ø8 mm steel rods, regardless of floor span or type of beam.
Firstly, the manuals make no difference between the type of masonry, for instance Arya et al. (2014) states that “all the horizontal reinforcing recommended for brick buildings, may be used for random rubble constructions as well”. As the width of brick walls is generally around 100–230 mm vs. 350–450 mm for stone walls, the question is whether these values and dimensions can indeed be freely interchanged. Secondly, all (9/9) define just one thickness and apply it for each beam, band or stitch. However, no consistency or consensus is found for this thickness, and neither for the numbers and diameters of the steel reinforcements. Recommendations range from bands of 75 mm thickness with 2ø10 or 2ø16 mm steel bars, to beams of 150 mm thickness with 4ø10 mm (Table 5), to even a foundation beam of 305 × 430 mm with 4ø12 bars (ABARI, 2016). Only (1/47) (Bothara et al., 2002) suggests placing an extra third horizontal bar over openings in the lintel beam. Dimensions of the stirrups are mentioned in (32/47), generally set at 6 mm steel bars with 150 mm center-to-center interval (21/32), with deviations of 4–10 mm diameter rods with 200–370 mm spacing.
Detailing of Horizontal Reinforcements
Most manuals include details for bending of steel bars in corners and T-sections, for which three different options are found, Figure 8. Overall, no indication was found for which one behaves better or worse during a seismic event. Some of these patterns will be difficult to bend with thick steel diameters of ø16 mm or even ø20 mm (UNDP, 2007). The splicing length is mentioned only in (13/47), ranging between 400 and 750 mm, or specified as lengths of minimum 40d, 50d and 60d. However, (6/13) including (Arya et al., 1980) clearly mention that splicing is not allowed in corners, which goes against Figure 8C. The length of the steel hooks for stirrups is generally set at 60 mm (18/47), but not one manual mentions whether these hooks should be applied in an alternating pattern, as often seen in publications about reinforced concrete.

Figure 8. Principles of different steel reinforcement patters in beams, following the recommendations made in (A) Arya et al. (1980). (B) UNDP (2007). (C) Arya et al. (2014); all by courtesy of Smart Shelter Foundation.
Steel qualities are only specified in (16/47). Arya et al. (2014) writes in a footnote: “Bar diameters are for mild-steel. For high strength deformed bars, equivalent diameter may be used,” but these then must be found from external sources. The steel quality for stirrups is specified only (7/47) times, being mild or plain steel (4/7), high strength deformed steel (1/47), or specifically “no plain steel” (2/47). Not one manual explains the difference between using plain or deformed steel for stirrups. More importantly, the ratio of the concrete mix is not mentioned in almost half the publications (22/47), and the remaining half offers two different options, being 1:1.5:3 or 1:2:4 of cement: sand: aggregates. Some manuals specify the mixes as M15 and M20 or mention that the concrete must have a minimum strength of 15 MPa after 28 days curing, but such specifications may not be understood by the non-engineered target groups. The maximum size of aggregates is defined in only (7/47) and results in 6 different sizes, which are 10, 12, 18, 20, 25, and 30 mm.
The minimum concrete cover on steel bars is specified in (27/47), ranging from 20 to 30 mm, whereas most examples show a distance from center of steel bar to the outside of beam. If we take 30 mm cover and we have bars of 12 mm and stirrups of 6 mm, this would result in a concrete layer of just 18 mm at all stirrups, which seems insufficient. Furtherly, only (6/47) mention the importance of keying, by means of sticking pieces of steel or stone into the wall before casting a beam, in order to improve bonding between masonry and concrete beam or band. And only (5/47) include information about the procedures of mixing, watering and curing to obtain good quality concrete.
Vertical Steel Reinforcements
A heavily debated topic is the need of incorporating vertical reinforcements in the walls. Smart Shelter Foundation left these out for two reasons. Firstly, it is questioned whether a relatively small number of steel rods will provide sufficient ductility in such thick walls. And secondly, a vertical disruption in the critical connections may weaken, rather than strengthen these.
Clearly, the practical manuals are in favor (35/47) of inserting them, whereas (31/35) prefer steel bars, (1/35) wooden or bamboo poles, and (3/35) have not defined the material. Of the remaining (12/47) none explicitly prohibits the vertical reinforcements, but it is unclear whether this is done intentionally, or if the topic is simply overlooked. The most recommended locations of steel bars are in the corners (27/31), T-sections (25/31) and next to openings (25/31), although boxing is often preferred when dimensions of openings are exceeded. However, not one manual has defined the need and maximum spacing of vertical reinforcements in cross-walls, which often have no openings at all. In some manuals steel bars are inserted inside the buttresses (5/31).
There is no consensus on the steel diameter, with diameters ranging from 10, 12 to 16–25 mm, and (20/31) describe different diameters in walls of multi-story buildings. Start and end detailing of the bars is seldom described (9/31) and there is no clear verdict whether bars start in the bottom of the foundation or in the plinth beam, and if they end in the lintel or in the top beam. The bending length for anchoring is either 450 mm, 55d or 60d, and splicing of the steel for multiple stories (3/31) is defined as 600 mm, 50d or 60d. Only (1/31) suggests that the bars must be tied to the horizontal reinforcement of the concrete bands (Desai et al., 2012).
In (25/31) it is described how to protect the steel against corrosion, by casting a concrete core around the vertical bar, following the principle of Figure 9A. This detail is often printed together with Figure 9B that shows the position of a through stone in the T-section, such as in IS 13828:1993 (1993); NBC 202:1994 (2007), IAEE (2004), and Arya et al. (2014). It must be stressed that this solution is simply not possible, as wherever a bond stone is located, there simply cannot be a vertical bar, and vice versa. However, this contradiction has not been rectified for over 30 years in these main publications, since it was first published in Arya et al. (1986). Some manuals however did spot this discrepancy and have published the principle of Figure 9C, where trough stones are placed next to the vertical steel bar. These describe a concrete core of 75–100 mm and through stones of 150 mm thickness, but this means that detailing of through stones all around the steel bar will not fit in a wall of 350 mm thick. Not one manual has fully detailed these masonry patterns for the corners, T-sections, jambs of openings or the buttresses.

Figure 9. Principles of masonry details with (A) vertical steel bar in T-section and (B) through stone in T-section (both following Arya et al., 2014). (C) vertical steel bar and through stone in T-section (following BMTPC, 2000; all by courtesy of Smart Shelter Foundation).
Roof Structure
The roof structure plays an important role in the box-like behavior of a building. But together with the foundation this is the least covered and most incomplete topic, where (18/47) don't mention anything at all, (2/47) only address flat earthen roofs, and (14/47) have included one single line, either that “the roof needs to be as light as possible”, or that “the roof must be properly anchored to the walls”. Bracing in the roof planes is recommended in (8/47), cross-bracing in-between the wooden trusses only in (1/47) (ERRA, 2007) and installing of a stiff ceiling in (4/47). Detailing and specification of wood dimensions is defined in just (4/47).
Conclusion
A literature review was carried out for the specific search criteria of school buildings with loadbearing walls of rubble stone masonry in cement mortar, tied with horizontal reinforcements, in seismic-prone regions. An initial review of 109 national seismic building codes of 48 different countries revealed that Iran is currently the only country that allows such buildings in their highest seismic areas. It must however be noted that the wall system is slightly different as it includes vertical concrete columns, and additional building regulations may locally apply. Only few countries, including Italy, allow it but only in their lowest seismic regions. With regards to stone masonry in the Himalayan region, with India and Nepal being the main focus of this review, the codes of these countries only allow stone masonry for buildings with importance factor 1.0. As school buildings have an importance factor of 1.5, this means these codes explicitly prohibit the use of stone masonry for construction of schools in any seismic zone. In that light it is remarkable that the Nepali Government goes against its own building codes, by approving school designs in rubble stone masonry.
The literature review also examined 47 practical manuals that cover the topic of stone masonry in seismically prone areas. Based on a set of particular search criteria, 22 manuals were rejected, and 12 manuals were found that address solely the design rules for houses; These are all from Pakistan, Bhutan and Nepal, and were briefly compared with the Indian code IS 13828:1993 and the Nepali code NBC 202:1994. Only 9 manuals (of which 7 are co-written by Aray) were eligible for an in-depth review for school buildings, even though this conflicts with the regulations of the current seismic building codes. The conclusion for both reviews of houses (even though not the aim of this paper) and schools is the same: Among these manuals, no consistency nor consensus was found for any of the design or construction related features for almost all key topics, such as main dimensions, openings and reinforcing elements.
It is also noticed that most information comes from just a few main sources, being “Basic Concepts” (Arya et al., 1980), “Educational Buildings” (Arya and Chandra, 1982) and “Guidelines for Earthquake Resistant Non-Engineered Construction” (Arya et al., 1986), but no reasons were found why the information gets altered along the way. The same illustrations and tables are copied over and over again, including apparent conflicts between the details, as seen for vertical steel and through stones. The fact that such contradictions have not been rectified in the latest versions, such as Arya et al. (2014), as well as the fact that key documents such the Indian code IS.13828:1993 and the Nepali code NBC202:1994 have never been properly updated, are indications that the knowledge has not evolved or progressed much since the 1980's. Ironically this brings us to the same conclusion made by Arya at the 1977 World Conference on Earthquake Engineering in New Delhi (Arya, 1977), which ultimately led to the development of the first publication for non-engineered construction in 1980. He states that “A review of the earthquake codes of various countries shows that much of the information is empirically based and not theoretically derived. In that respect the recommendations must be subject to continuous review and change as more data becomes available.”
Looking at the current state of the available information, Arya's statement of 1977 still seems valid today, and the authors of this review find this situation no longer acceptable. Fact is that millions of people will continue to live in stone houses in India, Nepal, Pakistan, Bhutan, Afghanistan, China, and likely in regions in Central Asia, the Middle East and Northern Africa as well. They need clear and reliable information that is up-to-date and complete. Therefore, the existing knowledge must be fully assessed, validated, optimized and complemented by means of the current state-of-the-art for calculating, testing and modeling. The authors acknowledge that this literature review is the first step of a more complex process that involves in-depth scientific research through multi-disciplinary collaborations, possibly by building upon current research topics which includes determination of reliable material properties (Barr et al., 2015; Rai and Dhanapal, 2015), experimental testing (Milosevic et al., 2012; Ahmad et al., 2013), numerical modeling (Parajuli, 2009; Tarque et al., 2014), and ultimately code revision (UNDP/ERRRP-project: NEP/07/010, 2009; Magenes and Penna, 2011), to name a few.
To address all of the above, the authors have started an initiative by the name of SMARTnet, which stands for Seismic Methodologies for Applied Research and Testing of non-engineered Techniques. The aim is to update the knowledge of traditional techniques in general, for which the rubble stone schools of Smart Shelter Foundation in Nepal will serve as a test case. A second aim is to make this knowledge understandable and available for engineers and non-engineers all over the globe, which hopefully creates renewed interest in countries that currently prohibit the technique as well. This paper is the first in a series, which will further include a cost analysis of the earthquake resistant measures in traditional building techniques, determination of realistic material properties of locally built rubble stone specimens, development of reliable test procedures for stone masonry, and methods for calculation and modeling of traditional techniques in general. SMARTnet invites experts, professionals, academics as well as final-year students in these fields to exchange their knowledge and to support the project with their time and expertise.
Author Contributions
MS collected all data, developed all drawings and estimates, prepared the comparisons and wrote the paper. YA checked all data and reviewed, revised and restructured the manuscript.
Funding
This work is supported by the Grant-in-Aid for Scientific Research (KAKENHI) of Japan Society for the Promotion of Science (JSPS) under grant number (B) 17H04592. Their support is greatly appreciated.
Conflict of Interest Statement
The authors declare that the research was conducted in the absence of any commercial or financial relationships that could be construed as a potential conflict of interest.
Acknowledgments
We wish to acknowledge everyone that helped by our search for the national seismic and building codes, and in particular Dr. A. Naderzadeh for his translations and explanations with regards to the Iranian codes and regulations, Dr. D. Rai for tracking down the oldest versions of the Indian codes, and J. Bothara for his explanations with regards to the Nepali codes and bylaws. The authors also wish to acknowledge C. Bodnar-Anderson, librarian of the NISEE-PEER Library for complementing the list of practical manuals. And last but not least, L. McCarton for his invaluable feedback on the draft of this paper.
References
ACI 530/530.1 (2011). Building Code Requirements and Specification for Masonry Structures and Companion Commentaries. Farmington Hills, MI: American Concrete Institute, with The Masonry Society and American Society of Civil Engineers.
Ahmad, N., Ali, Q., Ashraf, M., Alam, B., and Naeem, A. (2013). Seismic vulnerability of the Himalayan Half-dressed rubble stone masonry structures, experimental and analytical studies. Nat. Hazards Earth Syst. Sci. 12, 3441–3454. doi: 10.5194/nhess-12-3441-2012
Ahmadizadeh, M., and Shakib, H. (2016). Experimental study of the in-plane behavior of confined stone masonry walls. J. Struct. Eng. 142:04015145. doi: 10.1061/(ASCE)ST.1943-541X.0001417
Arya, A.S., and Chandra, B. (1982). Digest on Earthquake Protection of Educational Buildings. Paris: UNESCO.
Arya, A. S. (1977). “Codes,” in Proceedings of the 6th World Conference on Earthquake Engineering (New Delhi).
Arya, A. S. (1987a). Protection of Educational Buildings Against Earthquakes. Educational Building Report 13, Bangkok: UNESCO.
Arya, A. S. (1987b). Masonry & Timber Structures: Including Earthquake Resistant Design. Roorkee: Nem Chand & Bros.
Arya, A. S. (2000). “Non-engineering construction in developing countries–An approach toward earthquake risk reduction,” in Proceedings of the 12th World Conference on Earthquake Engineering (Auckland).
Arya, A. S. (2003). Guidelines for Earthquake Resistant Design, Construction and Retrofitting of Buildings in Afghanistan. Kobe: UNCRD Hyogo Office.
Arya, A. S. (2005). Earthquake Resistant Construction of Stone Buildings in Earthquake Affected Areas of Jammu & Kashmir. Delhi: Ministry of Home Affairs, Government of India.
Arya, A. S., Boen, T., Grandori, G., Benedetti, D., Grases, J., and Martemianov, A. (1980). Basic Concepts of Seismic Codes, Vol. 1, Part 2. Tokyo: International Association for Earthquake Engineering (IAEE).
Arya, A. S., Boen, T., and Ishiyama, Y. (2014). Guidelines for Earthquake Resistant Non-Engineered Construction. Paris: UNESCO.
Arya, A. S., Boen, T., Ishiyama, Y., Martemianov, A., Meli, R., and Scawthorn, C. (1986). Guidelines for Earthquake Resistant Non-Engineered Construction, Revised Edn., “Basic Concepts of Seismic Codes,” Vol. 1, Part 2. Tokyo: International Association for Earthquake Engineering (IAEE).
Asian Disaster Preparedness Center (2005). Earthquake Resistant Construction of Buildings, Curriculum for Mason Training, Guidelines for Training Instructors. Kathmandu: National Society for Earthquake Technology – Nepal (NSET).
Barr, S., McCarter, W., and Suryanto, B. (2015). Bond-strength performance of hydraulic line and natural cement mortared sandstone masonry. Construct. Build. Mater. 84, 128–135. doi: 10.1016/j.conbuildmat.2015.03.016
BBR-2002 (2003). Bhutan Building Rules 2002. Thimphu: Department of Urban Development & Housing, Ministry of Communications.
BMTPC (1999). Guidelines Improving Earthquake Resistance of Housing. New Delhi: Building Materials and Technology Promotion Council (BMTPC).
BMTPC (2000). Guidelines for Damage Assessment and Post-Earthquake Action, Part III: Reconstruction and New Construction of Buildings in Chamoli Earthquake Affected Areas of Uttar Pradesh. New Delhi: Housing and Urban Development Corporation (HUDCO).
Bothara, J., and Brzev, S. (2011). A tutorial: Improving the Seismic Performance of Stone Masonry Buildings, 1st Edn. Oakland: Earthquake Engineering Research Institute (EERI).
Bothara, J., Guragain, R., and Dixit, A. (2002). Protection of Educational Buildings against Earthquakes; A Manual for Designers and Builders. Kathmandu: National Society for Earthquake Technology–Nepal (NSET).
Carabbio, R., Pieraccini, L., Silvestri, S., and Schildkamp, M. (2018). How can vernacular construction techniques sustain earthquakes: the case of the Bhatar buildings. Front. Built Environ. 4:18. doi: 10.3389/fbuil.2018.00018
Coburn, A., Hughes, R., Pomonis, A., and Spence, R. (1995). Technical Principals of Building for Safety. London: Intermediate Technology Publications.
Construction Standard 132 (2008). Post-Earthquake Architecture and Technical Guide-Appendix B. Key Points for Reinforcing Traditional Buildings in Minority Areas. Beijing: Ministry of Housing and Urban-Rural Development.
Daldy, A. F. (1972). Small Buildings in Earthquake Areas. Watford: Building Research Establishment; Department of the Environment.
Decreto Ministriale (1987). Norme Tecniche per la Progettazione, Esecuzione e Collaudo degli Edifici in Muratura e per il Loro Consolidamento. Roma: Ministero dei Lavori Pubblici.
Department of Urban Development and Building Construction (2011). Engineers' Training on Earthquake Resistant Design of Buildings. Kathmandu: Ministry of Physical Planning and Works, Government of Nepal.
Department of Urban Development and Building Construction (2015). Design Catalogue for Reconstruction of Earthquake Resistant Houses, Vol. I. Kathmandu: Department of Urban Development and Building Construction (DUDBC).
Department of Urban Development Building Construction (2018). Required Documents for Design Check. Available online at: www.dudbc.gov.np/download/cat/12, last (Accessed June 27, 2018).
Desai, R., and Desai, R. (2008). Manual on Hazard Resistant Construction in India. For Reducing Vulnerability in Buildings Built Without Engineers. Ahmedabad: National Centre for People's-Action in Disaster Preparedness (NCPDP).
Desai, R., Desai, R., Jain, P., Mukerji, R., and Talpada, H. (2011). Technical Guidelines & Information for Stone Construction in Uttarakhand (Draft). Dehradun: Disaster Management and Mitigation Center.
Desai, R., Desai, R., Jain, P., Mukerji, R., and Talpada, H. (2012). Technical Guidelines & Information for Stone Construction in Uttarakhand (Draft). Dehradun: Disaster Mitigation and Management Center.
Disaster Management and Mitigation Department (2007). Guidelines for Reconstruction of Houses Affected by Tsunami, General and Public Buildings (Masonry). Chennai: Government of Tamil Nadu.
EN 771-6:2011 + A1:2015 (2015). Specifications for Masonry Units–Part6: Natural Stone Masonry Units. Brussels: European Committee for Standardization.
Engineer-in-Chief's Branch (2002). Technical Instruction No.5 of 2002: General Concepts & Guidelines on Earthquake Resistant Design and Construction in Masonry & RCC Structures in Military Engineering Services. New Delhi: Army Headquarters.
ERRA (2006a). Guidelines for Earthquake-Resistant Construction of Non-Engineered Rural and Suburban Masonry Houses in Cement Sand Mortar in Earthquake Affected Areas (Draft May 2006). Islamabad: UN-Habitat.
ERRA (2006b). Guidelines for Earthquake-Resistant Construction of Non-Engineered Rural and Sub Urban Houses in Pakistan, Version 1 April 2006. Islamabad: UN-Habitat.
ERRA (2007). Compliance Catalogue, Guidelines for the Construction of Compliant Rural Houses. Islamabad: UN-Habitat.
Eurocode 6 1996-1-1 (2005). Eurocode 6: Design of Masonry Structures-Part 1-1: General Rules for Reinforced and Unreinforced Masonry Structures. Brussels: European Committee for Standardization.
Eurocode 8 1998-1 (2004). Eurocode 8: Design of Structures for Earthquake Resistance-Part 1: General Rules, Seismic Action and Rules for Buildings. Brussels: European Committee for Standardization.
G.P.(Q)23-3,100-10-77 (1976). Quetta Development Authority Building Rules, 1976. Quetta: Quetta Development Authority.
GB 50011-2001 (2002). Code for Seismic Design of Buildings. Beijing: China Architecture & Building Press.
GB 50011-2010 (2010). Code for Seismic Design of Buildings. Beijing: Ministry of Housing and Urban-Rural Construction of the People's Republic of China, jointly with General Administration of Quality Supervision, Inspection and Quarantine of the People's Republic of China.
Government of Maharashtra (1998). Earthquake-Resistant Construction and Seismic Strengthening of None-Engineered Buildings in Rural Areas of Maharashtra. Mumbai: Revenue and Forests Department, Government of Maharashtra.
Government of Nepal (1968). Standard Measurement and Weight Act, 2025 (1968). Kathmandu: Nepal Bureau of Standards & Metrology.
Government of Tamil Nadu (2006). Disaster Resistant Construction Practices. Chennai: United Nations Development Program (UNDP).
Gujarat State Disaster Management Authority (2001). Reconstruction and New construction of Houses in Kachchh Earthquake Affected Areas of Gujarat, 2nd Edn. Gandhinagar: Government of Gujarat.
IAEE (2004). Guidelines for Earthquake Resistant Non-Engineered Construction, Reprint. Kanpur: National Information Centre of Earthquake Engineering (NICEE).
IBC 2015 (2014). 2015 International Building Code. Country Club Hills, IL: International Code Council. Available online: https://codes.iccsafe.org/public/document/toc/542/ (Accessed May 19, 2018).
Indian Railways (2017). Centre for Advanced Maintenance Technology. Handbook on Earthquake Resistant Buildings. For Official Use Only. Gwalior: Camtech.
IS 13828:1993 (1993). Improving Earthquake Resistance of Low Strength Masonry Buildings–Guidelines. New Delhi: Bureau of Indian Standards.
IS 13828:1993 (2008). Improving Earthquake Resistance of Low Strength Masonry Buildings. Guidelines, 3rd Reprint 2008 incl. Amendments nos 1. 2 & 3, Reaffirmed. New Delhi: Bureau of Indian Standards.
IS 1597(part1):1992 (1996). Construction of Stone Masonry–Code of Practice, Part 1 Rubble Stone Masonry, 1st Revision 1996, Reaffirmed 2002. New Delhi: Bureau of Indian Standards.
IS 1893 (part 1): 2002 (2002). Criteria for Earthquake Resistant Design of Structures. Part 1 General Provisions and Buildings, 5th Revision. New Delhi: Bureau of Indian Standards.
IS 4326:1993 (2005). Earthquake Resistant Design and Construction of Buildings-Code of Practice, 2nd Revision, 3.3 Edn., Reaffirmed 2008. New Delhi: Bureau of Indian Standards.
IS 4326:2013 (2013). Earthquake Resistant Design and Construction of Buildings - Code of Practice, 3rd Revision. New Delhi: Bureau of Indian Standards.
IS SP7:2016 (2016). National Building Code of India 2016, Vol. 1. New Delhi: Bureau of Indian Standards.
IS:1597(part 1)-1967 (1973). Construction of Stone Masonry–Code of Practice, Part 1 Rubble Stone Masonry, 1st Reprint 1973. New Delhi: Bureau of Indian Standards.
IS:1893-1962 (1962). Recommendations for Earthquake Resistant Design of Structures. New Delhi: Bureau of Indian Standards.
IS:1904-1986 (1989). Code of Practice for Design and Construction of Foundation in Soils: General Requirements, 3rd Revision, 1st Reprint, Reaffirmed 2006. New Delhi: Bureau of Indian Standards.
IS:1905-1969 (1975). Code of Practice for Structural Safety of Buildings: Masonry Walls, 1st Revision, First Reprint 1975. New Delhi: Bureau of Indian Standards.
IS:1905-1987 (1995). Code of Practice for Structural Use of Unreinforced Masonry, 3rd Revision, 1st Reprint 1995, Reaffirmed 2002. New Delhi: Bureau of Indian Standards.
IS:4326-1967 (1968). Code of Practice for Earthquake Resistant Construction of Buildings. New Delhi: Indian Standards Institution.
IS:4326-1976 (1977). Code of Practice for Earthquake Resistant Construction of Buildings, 1st Revision. New Delhi: Indian Standards Institution.
Jain, S. (2000). “Historical Developments and Current Status of Earthquake Engineering in India,” Proceedings of the 12th World Conference on Earthquake Engineering (Auckland).
JICA (2016a). Technical Handbook for Reconstruction of Earthquake Resistant Houses, for Masons and Craftsmen. Kathmandu: Japan International Cooperation Agency (JICA).
JICA (2016b). Guidebook for Reconstruction of Earthquake Resistant Houses, for House Owners. Kathmandu: Japan International Cooperation Agency (JICA).
Lodi, S. H. (2015). 10 Years after the 2005 Earthquake: Lessons Learned and Opportunities for Risk Reduction in Pakistan–Earthquake and Vulnerability of Pakistan and Risk Scenarios. Powerpoint Presentation.
Magenes, G., and Penna, A. (2011). “Seismic design and assessment of masonry buildings in Europe: recent research and code development issues,” in Proceedings of the 9th Australasian Masonry Conference (Queenstown).
Milosevic, J., Gago, A., Lopes, M., and Bento, R. (2012). “Experimental Tests on Rubble Masonry Specimens–Diagonal Compression, Triplet and Compression Tests,” in Proceedings of the 15th World Conference on Earthquake Engineering (Lisboa).
Ministry of Works and Human Settlement (2013). Earthquake Resistant Construction Training Manual (Stone Masonry). Thimpu: United Nations Development Program-Bhutan (UNDP).
Ministry of Works and Human Settlement (2014). Earthquake Resistant Construction Training Manual (Stone Masonry). Thimpu: United Nations Development Program-Bhutan (UNDP).
Mohapatra, A., and Mohanty, W. (2010). An Overview of Seismic Zonation Studies in India. Mumbai: Indian Geotechnical Conference.
Murty, C.V.R. (2002a). Earthquake Tip 14; Why Are Horizontal Bands Necessary in Masonry Buildings? New Delhi: Building Materials and Technology Promotion Council (BMTPC).
Murty, C.V.R. (2002b). Earthquake Tip 16; How to Make Stone Masonry Buildings Earthquake Resistant? New Delhi: Building Materials and Technology Promotion Council (BMTPC).
National Planning Commission (2015). Post Disaster Needs Assessment (PDNA). Vol. B, Sector Reports. Kathmandu. Government of Nepal.
National Reconstruction Authority (2017). Correction/Exception Manual for Masonry Structure for Houses That Have Been Built Under the Housing Reconstruction Programme. Kathmandu: National Reconstruction Authority(NRA).
National Reconstruction Authority (2018). 12 Rubble Stone School Designs in Nepal After the 2015 Earthquake. Available online at: Accessed July 14, 2018).
NBC 000:1994 (2007). Requirements for State-of-the-Art Design: An Introduction, V0.RV1 Reprint. Kathmandu: Department of Urban Development and Building Construction.
NBC 105:1994 (2007). Seismic Design of Buildings in Nepal, V2.RV5 Reprint. Kathmandu: Department of Urban Development and Building Construction.
NBC 109:1994 (2007). Masonry: Unreinforced (reprint). Kathmandu: Department of Urban Development and Building Construction.
NBC 202:1994 (2007). Mandatory Rules of Thumb for Loadbearing Masonry, V2.RV7, reprint. Kathmandu: Department of Urban Development and Building Construction.
NBC 203:2015 (2015). Guidelines for Earthquake Resistant Construction: Low Strength Masonry. (RV1). Kathmandu: Department of Urban Development and Building Construction.
NTC 2018 (2018). Decreto Ministeriale del 17/1/2018-Norme Tecniche per le Costruzioni. Roma: Ministro delle infrastrutture e dei Trasporti.
Pandey, B., Brzev, S., Culbert, R., and Schoenfeld, G. (2017). Illustrated Guidelines for Construction of Stone Masonry in Seismic Regions of Nepal. Kathmandu: United Nations Development Program (UNDP).
Papanikolaou, A., and Taucer, F. (2004). Review of Non-Engineered Houses in Latin America with Reference to Building Practices and Self-Construction Projects. Ispra: Institute for the Protection and Security of the Citizen European Laboratory for Structural Assessment.
Parajuli, H. (2009). Dynamic Analyses of Low Strenghth Masonry Houses Based on Site Specific Earthquake Ground Motions, Dissertation. Kyoto: Kyoto University Research Information Repository.
Rai, D., and Dhanapal, S. (2015). Mineralogical and mechanical properties of masonry and mortars of the Lucknow Monuments Circa the 18th Century. Int. J. Archit. Herit. 9, 300–309. doi: 10.1080/15583058.2013.780109
RCR-2013 (2013). Rural Construction Rules 2013. Thimphu: Department of Human Settlement, Ministry of Works and Human Settlement.
Royal Government of Bhutan (2014). Bhutanese Architecture Guidelines. Thimphu: Ministry of Works and Settlement.
Schilderman, T. (1990). Earthquake Resistant Housing. Reprinted as Technical Brief. Bourton-on-Dunsmore: Practical Action.
Schildkamp, M. (2015a). Rules of Thumb for Building Safe Non-Engineered Buildings With Random Rubble Stone Masonry. Alphen aan den Rijn: Smart Shelter Research.
Schildkamp, M. (2015b). Choosing the Right Materials and Quality Control for Non-Engineered Earthquake Resistant Construction. Alphen aan den Rijn: Smart Shelter Research.
Shahzada, K. (2007). Field Practicing Manual. Peshawar: Department of Civil Engineering; NWFP University of Engineering & Technology.
Silva, V., Yepes-Estrada, C., Dabbeek, J., Martins, L., and Brzev, S. (2018). GED4ALL-Global Exposure Database for Multi-Hazard Risk Analysis–Multi-Hazard Exposure Taxonomy. GEM Technical Report 2018-01. Pavia: GEM Foundation.
Singhal, V. V., and Rai, D. (2017). Behavior of confined masonry walls with openings under in-plane and out-of-plane loads. Earthquake Spectra. 34, 817–841. doi: 10.1193/061416EQS097M
SNiP II.7.81* (1981). Construction in Seismic Areas. Moscow: State Committee for Construction in the Soviet Union (Gosstroy).
Standard 2800 (2015). Iranian Code of Practice for Earthquake Resistant Design of Buildings, 4th Edn., Tehran: Building and Housing Research Center.
Standards and Quality Control Authority (2010). Guideline on Proper Construction for Non-Engineered Buildings (Stone Masonry). Thimpu: Ministry of Works and Human Settlement-Bhutan.
Tarque, N., Benedetti, A., Camata, G., and Spacone, E. (2014). “Numerical modelling of the in-plane behaviour of rubble stone masonry,” Proceedings of the 9th International Conference on Structural Analysis of Historical Constructions (Mexico City).
The Post Disaster Recovery Framework (2016). Sector Action Plan (SAP) Rural Housing & Community Infrastructure. Kathmandu: National Reconstruction Authority, Government of Nepal.
Tomazevic, M. (1999). Earthquake Resistant Design of Masonry Buildings, Series on Innovation in Structures and Construction, Vol. 1. London: Imperial College Press.
UNDP team for Tsunami Recovery Support (2007). Disaster Resistant Construction Practices, A Reference Manual. Chennai: United Nations Development Program (UNDP).
UNDP/ERRRP-project: NEP/07/010 (2009). Recommendations for Update of Nepal National Building Code. Final Report. Kathmandu: Government of Nepal, Ministry of Physical Planning and Works.
Winter, T. (2016). Manual for Post-Earthquake Building in Nepalese Valleys. Paris: L'Association Française du Génie Parasismique (AFPS).
Keywords: rubble stone masonry, non-engineered building techniques, literature review, seismic building codes, practical construction manuals
Citation: Schildkamp M and Araki Y (2019) School Buildings in Rubble Stone Masonry With Cement Mortar in Seismic Areas: Literature Review of Seismic Codes, Technical Norms and Practical Manuals. Front. Built Environ. 5:13. doi: 10.3389/fbuil.2019.00013
Received: 14 October 2018; Accepted: 28 January 2019;
Published: 28 February 2019.
Edited by:
Panagiotis G. Asteris, School of Pedagogical & Technological Education, GreeceReviewed by:
Rita Bento, Universidade de Lisboa, PortugalClaudia Casapulla, University of Naples Federico II, Italy
Marijana Hadzima-Nyarko, Josip Juraj Strossmayer University of Osijek, Croatia
Copyright © 2019 Schildkamp and Araki. This is an open-access article distributed under the terms of the Creative Commons Attribution License (CC BY). The use, distribution or reproduction in other forums is permitted, provided the original author(s) and the copyright owner(s) are credited and that the original publication in this journal is cited, in accordance with accepted academic practice. No use, distribution or reproduction is permitted which does not comply with these terms.
*Correspondence: Martijn Schildkamp, aW5mb0BzbWFydHNoZWx0ZXJyZXNlYXJjaC5jb20=