- 1Research Center for Integrated Disaster Risk Management (CIGIDEN), Santiago, Chile
- 2Department of Architecture, Universidad Técnica Federico Santa María, Valparaíso, Chile
- 3Marine Energy Research & Innovation Center (MERIC), INRIA, Santiago, Chile
- 4Civil Engineering Department, Universidad Técnica Federico Santa María, Valparaíso, Chile
- 5Scientific and Technological Center, Valparaíso, Chile
- 6Hydraulic and Environmental Engineering Department, College of Engineering, Pontificia Universidad Catolica de Chile, Santiago, Chile
Cities are increasingly becoming hot-spots for nature-originated disasters. While the role of the urban built environment in fostering disaster resilience has been recognized for some time, it has been difficult to translate this potential into practice. This is especially challenging in the case of rapid onset crises such as near-field tsunamis when appropriate urban forms must support the populations' ability to autonomously carry out safe and timely responses. In this respect, much of current research remains focused on large-scale elements of urban configuration (streets, squares, parks, etc.,) through which people move during an emergency. In contrast, the critical micro-scale of evacuees' experiences within the built environment is not commonly examined. This paper addresses this shortfall through a macro- and micro-scale analysis of a near-field tsunami scenario affecting the city of Viña del Mar, Chile, including a mixed-methods approach that combines computer-based models and fieldwork. The results show significant macro-scale tsunami vulnerability throughout major areas of the city, which nonetheless could be mitigated by existing nearby high ground and an urban form that allows short evacuation times. However, micro-scale outcomes show comparatively deficient spatial conditions that during an emergency might lead to dangerous outcomes including bottlenecks, falls and panic. Vertical evacuation, in turn, is confirmed as a suitable option for reducing vulnerability, but further examination of each shelter's characteristics is required.
Introduction
An increasingly urbanized world implies that most responses to rapid onset disasters must be undertaken within the built environment. The environment's characteristics (particularly its urban form) can increase communities' capacities to deal with unfolding crises and aid them in achieving resilience (Allan et al., 2013). While this is a growing area of research, it has been difficult to fully realize the built environment's potential for delivering disaster risk reduction (DRR) (Wamsler, 2006; Murao, 2008; IPCC, 2012; March and León, 2013). For instance, in the case of near-field tsunamis populations usually must carry out critical response activities (e.g., evacuation and sheltering) in little time (e.g., wave arrival times were as short as 12 min. during the 2015 and 2016 Chilean tsunamis) and without official guidance, as governmental and emergency services are typically overwhelmed by cascading failures (Little, 2002) brought about by the previous large-magnitude earthquake. This haste and autonomy requires a city form capable of providing adequate support to evacuees, thus allowing them safe and timely evacuations. Nevertheless, much of current research and practice efforts on urban tsunami evacuation potential are largely focused on macro-scale elements, such as streets, squares, and parks, and the way they spatially connect to each other. In contrast, the critical micro-scale elements that are revealed through evacuees' experiences while they are escaping throughout the built environment are not commonly examined. This paper analyses this gap in the Chilean earthquake- and tsunami-prone city of Viña del Mar, by undertaking a mixed-methods approach that includes computer-based models and fieldwork.
This paper is divided into four parts: First, it provides a theoretical background about DRR in the built environment. Second, the problem of tsunami risk mitigation is examined in the context of the city of Viña del Mar, Chile and its most vulnerable area, the Población Vergara district. Third, the research methods and their outcomes are presented and discussed, including the examination of an improved scenario with vertical shelters; lastly, the paper's main conclusions are introduced.
Disaster Risk Reduction in the Built Environment
The Sendai Framework for Disaster Risk Reduction 2015–2030 points out that between the years 2005 and 2015 nature-originated disasters affected more than 1.5 billion people globally (UNISDR, 2015). Moreover, growing exposure of persons and assets to hazards, especially in the developing world, has outnumbered global vulnerability reduction efforts. This is particularly critical in the case of cities, where more than 50% of the world's population is currently living (United Nations, 2014). Cities have become hot-spots for disasters (Joerin and Shaw, 2010; Wamsler, 2014), in both formal and informal urban areas (Comfort et al., 1999; Bull-Kamanga et al., 2003; Pelling, 2003). This is the outcome of the concentration of population, infrastructure, and assets (Alexander, 1993; Bull-Kamanga et al., 2003), rising social inequalities, poor physical conditions of buildings or infrastructure, lack of institutional capacity (Pelling, 2003; Gencer, 2013), market-driven urbanization, location choices made by dwellers without proper information, and “inefficient application of construction standards and building design,” as the result of “corruption or mismanagement by related officials” (Gencer, 2013, p. 29).
In this rapidly urbanizing world, the majority of the population lives in a built environment that offers “an important means by which humanity can reduce the risk posed by hazards, thereby preventing a disaster” (Haigh and Amaratunga, 2010, p. 15). Disciplines responsible for shaping this environment through spatial design (e.g., land use management, urban planning, architecture) thus become appropriate tools for strengthening disaster risk governance and fostering resilience (UNISDR, 2015). For instance, urban planning can help manage four types of risk factors: hazards, location-specific vulnerability, in addition to both response and recovery mechanisms and structures (Wamsler, 2014). It also has the potential to affect the location and the design of urban development and can contribute to building citizen awareness of the risks of natural hazards (Burby et al., 1999). Moreover, planning “provides a medium for the reduction of uncertainty in dealing with hazards, allowing mitigation of their worst effects, and even the remediation of past mistakes” (March and Henry, 2007, p. 19). In turn, Mazereeuw (2011, 2015) underlines the importance of design for disaster preparedness, given its ability to work with dynamic urban-environmental systems across multiple scales and to incorporate knowledge from many fields. Moreover, she also points out the importance of design as an anticipatory and pre-emptive strategy to building multiple levels of redundancy into the planning and design of cities.
Nevertheless, it has been difficult to translate spatial design's potential for DRR into practice (Wamsler, 2006; Murao, 2008; IPCC, 2012; March and León, 2013). While some emerging examples of integration between these two areas of knowledge do exist, there is no wider set of principles to achieve it (IPCC, 2012). For instance, Alexander (2004, p. 4) argues that during the window of opportunity after a disaster, when the public and politicians demand higher levels of safety “there is considerable scope for hazard reduction through the normal urban and regional planning process, but at the moment it is very significantly underutilized.” In this regard relocating vulnerable populations has been widely thought to be an effective risk-reduction strategy. However, this has proven to be difficult in several post-disaster contexts, because doing so commonly overlooks the importance of existing livelihood security, site dependency and social ecologies, and the overall inertia of the urban realm (Oliver-Smith, 1991; Twigg, 2004; Menoni and Pesaro, 2008).
An urban form capable of allowing prompt and adequate responses by vulnerable populations is especially important in the case of rapid onset disasters affecting densely inhabited locations, such as near-field tsunamis. In cases like this, little time is available for populations to undertake critical activities such as evacuation and sheltering that in turn have a strong influence on the overall impact of the catastrophe. Furthermore, these actions usually must be conducted without official guidance as the result of overwhelmed governmental emergency services. This context of crisis requires urban forms to include characteristics capable of promoting resilience by supporting the populations' ability to autonomously carry out safe and timely responses.
Tsunami Evacuation and Urban Form in Viña Del Mar, Chile: Increasing Disaster Vulnerability by Development Patterns
Near-Field Tsunamis: Unpredictable and Rapid Onset Disasters
Tsunamis (from the Japanese word “harbor wave”) are long period waves generated by the sudden displacement of a large volume of water. Their most common causes are undersea earthquakes, submarine, and subaerial landslides into a volume of water and volcanic eruptions (NTHMP, 2001; Bryant, 2014). For a given location, destructive tsunamis are relatively unpredictable and rare but have the potential for extensive material damage and loss of human life. Therefore, they pose a serious threat to coastal communities around the world, particularly to those located in the Pacific and Indian Ocean basins and the Mediterranean and Caribbean Seas. Since 1975, 39 destructive tsunamis have killed more than 259,000 people and provoked extensive material losses in these areas (IOC-UNESCO, 2013). When classified in accordance to the distance between the source and the affected area, near-field (or local) tsunamis are characterized by very short impact times (usually <30 min), particularly in those cities located close to the tsunamigenic earthquake's epicenter. In this respect, Gusiakov (2009) argues that around 84% of the casualties during the most destructive trans-oceanic tsunamis over the last 250 years occurred within the first hour of propagation time.
Tsunami Risk Mitigation
Contemporary tsunami risk reduction efforts in coastal settlements can be grouped into three main safety layers (Hoss et al., 2011; Tsimopoulou et al., 2012). These include (1) a prevention layer of extensive civil-engineered defenses aimed at maintaining flood waters outside usually dry areas (e.g., breakwaters, sea gates, seawalls); (2) a spatial solutions layer aimed at decreasing losses if floods occur, something commonly developed through land use and built environment strategies and changes (e.g., zoning, relocation, building codes, and design recommendations); and (3) an emergency management layer composed of crisis readiness systems (e.g., warning systems and evacuation preparation) (Preuss, 1988; Bernard, 1995; NTHMP, 2001; Kazusa, 2004; IOC-UNESCO, 2008; Shuto and Fujima, 2009; Murata et al., 2010). As extensive civil-engineered defenses for tsunami mitigation remain uncommon outside Japan (due to their high technical and monetary requirements), in near-field events with short arrival times evacuation remains “the most important and effective method to save human lives” (Shuto, 2005, p. 8).
In case of a near-field tsunami, the vulnerable population has little time to plan and conduct disaster-response activities such as evacuation and sheltering. Moreover, as the related tsunamigenic earthquake typically triggers a cascading failure of emergency systems and urban lifelines (Alesch and Siembieda, 2012), evacuees have to autonomously undertake these actions, which take place within the spatial canvas provided by the built environment composed of buildings, streets and open spaces (including parks and squares). The disciplines that guide and control the morphological development and change of this environment (such as urban planning and urban design) can therefore have an important influence on the overall outcome of an emergency evacuation. Significant efforts have been recently developed to examine the links between appropriate urban forms and tsunami evacuation potential (González-Riancho Calzada et al., 2013; León and March, 2014, 2016; Wood et al., 2014, 2016). Nevertheless, much of these efforts remain focused on large-scale elements of urban configuration, i.e., the system of linked spatial elements (streets, squares, parks, etc.) through which people move (Hillier et al., 1993) during an emergency. The critical micro-scale of evacuees' spatial experiences within the built environment (usually in deteriorated conditions after a tsunamigenic earthquake) is not commonly examined.
Tsunami Risk in Viña del Mar, Chile
Viña del Mar (33°1′S, 71°33′W) is a coastal city of some 310,000 inhabitants, located ~100 km northwest of the Chilean capital Santiago and 7 km northeast of the neighboring port city of Valparaíso. Viña del Mar's site includes a large coastal plain, ~1.5 × 1.5 km wide and with a maximum elevation of roughly 20 m (the Población Vergara district), surrounded by several steep hills and plateaus where most of the city's population resides (see Figure 1).
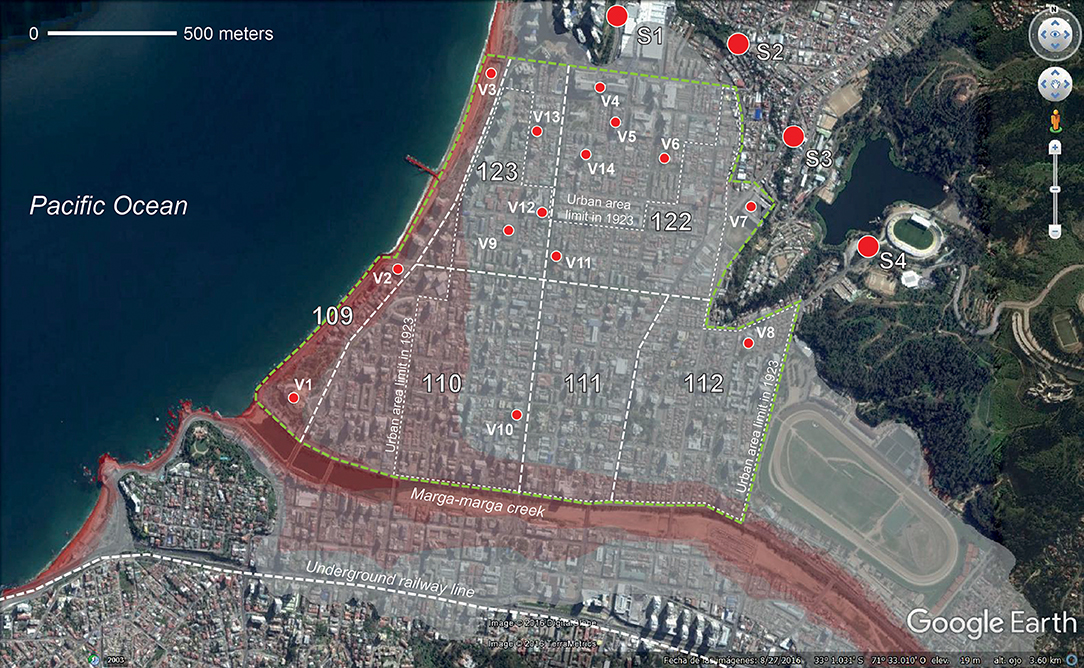
Figure 1. Satellite image of the Población Vergara neighborhood in Viña del Mar, including existing (S1 to S4) and proposed (V1 to V14) evacuation shelters, overlapped with the expected tsunami flood area (in shades of red), according to SHOA's model. SHOA (2012) and Booth (2002).
Viña del Mar is an earthquake- and tsunami-prone territory. It is in the central region of Chile, which was struck by destructive earthquakes in 1575, 1647, 1730, 1822, 1906, and 1985 (Lomnitz, 1970; Comte et al., 1986). In turn, the last four of these events caused tsunamis in the area (with variable magnitudes). The largest recorded destructive tsunami occurred on 8 July 1730, provoked by a Mw 9.1–9.3 mega-earthquake that had its epicenter offshore the city of Valparaíso (Carvajal et al., 2017). This phenomenon wiped out Valparaíso's downtown of <1,000 dwellers. Viña del Mar in that year however was an uninhabited area, so no records exist regarding the impact of the tsunami on its coasts. However, the current flood map of the city developed by SHOA, the Chilean Navy's agency for tsunami research (SHOA, 2012) reveals extensive urban areas in vulnerable situations, comprising diverse land uses, building types and population characteristics, especially in the Población Vergara district (see Figure 1). Moreover, scientists suggest that the ongoing 300-year long gap between mega-earthquakes could be filled in forthcoming decades by a large seismic event, which in turn might trigger a destructive tsunami (Ruiz and Madariaga, 2018).
Increasing Tsunami Vulnerability by Land-Use and Activity Patterns in Viña del Mar
Until the first half of the nineteenth century, Viña del Mar's territory was a large-estates agricultural area with poor accessibility and scarce population. This condition began to change in 1855, when the new Valparaíso-Santiago railway line included Viña del Mar as an intermediate station. In 1874 (when <100 dwellings existed in the area) the former estates began to be subdivided around the railway line (Cáceres Quiero et al., 2002), while in 1892 this area expanded north (across the Marga-marga creek) through the development of the Población Vergara district (Pecchenino Raggi, 1974). The city then began a rapid urbanization process, which during the twentieth century allowed Viña del Mar's population to grow from 10,691 in the year 1895 to 286,931 in 2002 (INE, 2002). This development was driven by the consolidation of Viña del Mar during the first half of the twentieth century as an industrial and tourist hub, and afterwards as a residential and services center for the Great Valparaíso metropolitan area (Booth, 2002; Cáceres and Sabatini, 2003).
Since its occupation in 1892, the Población Vergara district combined industrial and residential land uses; urban development was initially located around 400 m inland (see Figure 1). The town's touristic role and its related waterfront occupation were boosted only after the enactment of the n° 4283 national-level law (in 1927). This act funded the construction of a series of touristic waterfront facilities in Viña del Mar, including the first Chilean casino (V1 on Figure 1), hotels and two public swimming pools (Cáceres Quiero et al., 2002). During subsequent decades, industrial facilities in the Población Vergara district were progressively substituted by residential development and services (mainly commerce). In this respect, census data provided by the Chilean Bureau of Statistics (INE) shows that between the years 1930 and 2002 the district's population grew from 13,118 to 20,302 (i.e., 54.8%). While when this paper was written no subsequent census information was available, the origin-destination study conducted in 2015–16 by the Chilean Ministry of Transport (SECTRA, 2016) estimates a current population of 28,296 people in the area.
This steep increase in the Población Vergara's number of inhabitants is the outcome of a rapid densification process through high-rise apartment buildings (see Figure 2), whose dwellers are attracted by the district's high-quality of life and its central location in the Great Valparaíso metropolitan area. Moreover, a study from 2012 (Soto and Álvarez, 2012) shows that of 13,810 business and professional practice licenses existing in the city of Viña del Mar during that year, 4,403 (31.9%) were located in the Población Vergara district. This however represents only 3.2% of the municipality's area and 7.1% of its population (according to the 2002 Census). The previously discussed origin-destination study also reveals commuting patterns and population distributions that under certain conditions might seriously increase the tsunami vulnerability of the area. During regular weekday morning rush hours (i.e., between 6:45 and 9:45 a.m.) the district's population can grow by as much as 89.9% in comparison to a night-time scenario, as the result of attraction trips generated by large-scale facilities located there (shopping centers, schools, governmental offices, hotels, etc.). Moreover, during the summer season the beaches located in the Población Vergara can receive thousands of visitors coming from other districts of Viña del Mar, the Great Valparaíso area and other Chilean regions.
The district's recent intensive development has been accommodated within an urban fabric originally designed for low-density residential purposes. Nowadays, its narrow streets and few open public spaces struggle to sustain a range of other land uses and the high traffic they involve. These conditions cast doubt on the Población Vergara's suitability for providing an appropriate built environment for rapid and safe tsunami evacuations. The next section provides a careful examination of this situation, at both the macro- and micro-scale of analysis.
Research Methods and Outcomes
This paper is based upon a case study research method of Viña del Mar conducted on two different scales of analysis: (1) the macro-scale of the urban tsunami evacuation system comprised of routes and safe assembly areas, and (2) the micro-scale of the built environment as experienced by evacuees during an emergency.
Macro-Scale Analysis
This scale was examined with the aid of two computer-based models. The first one aimed to analyze the Población Vergara district's urban configuration characteristics that might have an impact on the evacuation process. The second one, an agent-based model, was focused on diagnosing a likely tsunami evacuation scenario in the district and its possible outcomes.
Configuration Analysis
Alongside open areas such as squares and parks, the main element of an urban evacuation spatial system is the street network; an appropriate configuration of this feature can increase the evacuees' chances at successfully evacuating in the case of a tsunami (Fakhrurrazi and Van Nes, 2012). This configuration model examined three characteristics of the Población Vergara district: (1) street network density of (km/km2); (2) connectivity index (n); and (3) average pedestrian route directness (n). Numbers (1) and (2) above contribute to measuring how accessible a street system is and how it enables shorter trips and a wider range of route options between places, which are key features in case of a disaster-related evacuation (Handy et al., 2003; Dill, 2004). In turn, number (3) allows us to examine how short and easy-to-follow the routes to safe destinations are, avoiding large deviations whenever it is possible. This is particularly important in the case of an evacuation, when evacuees try to select the closest destinations in terms of distance and time (Mohareb, 2011). The inputs for this configuration analysis included the Población Vergara's street network and its four-existing tsunami safe assembly areas. These are open public spaces with an elevation of at least 30 m (as identified by ONEMI, the Chilean Emergency Management Agency). These shelter locations avoid crossing the Marga-marga creek during evacuation, as this is an inland penetration path for a tsunami, whose bridges might suffer bottlenecks and structural failure. Another input for the configuration model was a night-time population distribution of 28,296 (according to SECTRA, 2016). These inhabitants were randomly distributed across the district's streets and open spaces, by using the PARI-AGENT software (Arikawa, 2015) that was developed by Japan's Port and Airport Research Institute; this software was also used to build up the agent-based model, as explained in the following sections. The configuration analysis was carried out in the ArcGIS software with the aid of its Network Analyst extension.
A street network density can be defined as the ratio between the total length of streets within a sector and the sector's overall area (Southworth and Owens, 1993). The model's results show that the streets in the Población Vergara district have a street network density of roughly 20.56 (km/km2). This is a relatively high value; for instance, Dill (2004) examined 219 census tracts in the metropolitan area of Portland, Oregon, finding the average street network density to be 13.19 (km/km2) with minimum and maximum values of 5.35 and 26.62 km/km2, respectively. In turn, a study conducted by Southworth and Owens (1993) on Californian suburban growth during the twentieth-century showed that the gridiron street pattern (similar to the Población Vergara's) has an average density of around 16 (km/km2). In Chile, the city of Iquique offers a good comparison to Viña del Mar, with an average value of ~17.4 (km/km2). Past emergencies and research (León and March, 2016) show that Iquique is well-suited for rapid tsunami evacuation, partly as the result of this dense and redundant network of streets.
For a certain urban area, the connectivity index can be defined as the ratio between the street links (i.e., street sections between intersections) and the street nodes (or intersections), according to Handy et al. (2003). They also point out that minimum acceptable connectivity indexes range between 1.2 and 1.4. In the case of the Población Vergara, the configuration model shows that it has an appropriate connectivity index of 1.56 (like Dill's average of 1.54). In this respect, the case of Iquique shows connectivity indexes between 1.31 and 1.74, depending on which part of the city is under examination.
In turn, the pedestrian route directness ratio can be defined as the ratio between a route's actual distance throughout urban space and the geodetic (or straight-line) distance between its origin and destination; for a given urban area, this index should not exceed 1.5 (Randall and Baetz, 2001; Handy et al., 2003; Dill, 2004). In the case of an evacuation analysis, this metric can be applied to examine the degree in which the existing urban grid allows a direct movement between each evacuee's vulnerable location and his/her closest safe assembly area. In the case of the Población Vergara district, the average value for the evacuee's distribution during a night-time evacuation scenario is 1.2. This is a relatively low number, which broadly shows that the district's urban grid makes it possible to reach the evacuation shelters without taking large detours or several changes in the evacuation direction. However, it is necessary to pay attention to some areas located in the district's north-eastern part; close to shelter S2 (see Figure 1). Here, evacuees are located at a safe distance from the ocean (roughly between 700 and 900 m) and close to high ground (i.e., above 30 m). Nevertheless, accessibility to this shelter area requires following intricate and narrow streets, which might imply wayfinding issues for evacuees, especially foreign ones.
Agent-Based Model
Agent-based computer models are powerful bottom-up modeling techniques that allow the examination of complex real-life systems and the dynamic interactions between their individual elements. This can be achieved by (1) disaggregating these elements into representative units: the agents; (2) encoding these agents' behavior in a set of simple rules; and (3) observing how these rules affect the interaction between themselves and with their environment (Klüpfel and Schreckenberg, 2003; Chen and Zhan, 2008; Wilensky and Rand, 2015). In recent years, agent-based models have been extensively used to calculate tsunami-vulnerable communities evacuation potential and the required times to evacuate their at-risk populations (Taubenböck et al., 2009; Imamura et al., 2012; Di Mauro et al., 2013; Mas et al., 2015).
The Población Vergara district's agent-based model was developed in the aforementioned PARI-AGENT software, which couples evacuation and tsunami-flooding parameters (Arikawa, 2015). In turn, these flood parameters were previously modeled with the aid of STOC-ML software (Tomita et al., 2006). As a worst-case scenario a tsunami was used that was similar to the 1730 event, which was triggered by a Mw 9.1–9.3 earthquake (Carvajal et al., 2017). In this case, the tsunami arrives at Viña del Mar's coasts in ~15 min, and the ocean's maximal water height above average sea level reaches 5 m. To develop a more realistic flooding scenario for the city (given the high built-density of the area) the existing urban blocks were included in the model as part of the topography, with a uniform height of 15 m. and setbacks of 2.5 m. on each side of the roads (see Figure 3 left). The results of the model after 2,700 s (45 min, i.e., the time required to achieve the tsunami's maximum penetration inland) show a run-up elevation (i.e., the difference between the ground elevation of the point reached by the tsunami, and the sea level) of around 13 m. with a maximum flow depth (i.e., the height above ground reached by the water) of 4 m. located in the south-western part of the study area, close to the casino building (V1 in Figure 1).
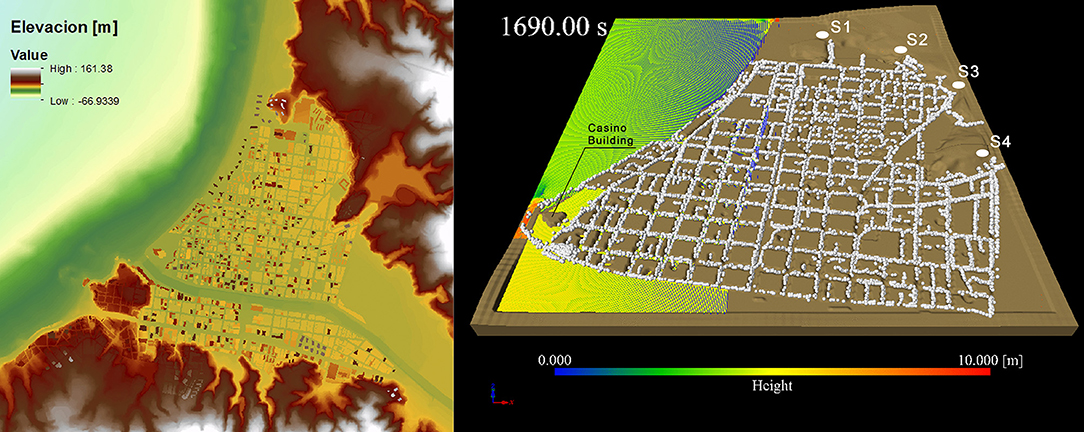
Figure 3. (Left) Digital Surface Model for Población Vergara and (right) A snapshot from the agent-based model, including tsunami flood height.
The PARI-AGENT software uses these flooding parameters as input for the evacuation model, alongside the following four features (see Figure 3 right). The first one is an agent-definition file that sets up the overall quantity of agents (28,296 for the night-time scenario and 53,743 for the rush-hour case, according to SECTRA, 2016) and their individual characteristics. These are: (1) a randomly assigned location within the streets and open spaces of the analysis area; (2) a walking speed for every agent, randomly assigned based on Viña del Mar's population age distribution (Mas et al., 2013) (according to the 2002 Census), i.e., children under 11 years old (16.86% of the city's population, walking speed 1.07 m/s), teenagers from 12 to 17 (9.99%, 1.34 m/s), adults between 18 and 59 (58.04%, 1.34 m/s) and elders over 60 years old (15.11%, 0.94 m/s); (3) a random-walk parameter that introduces an aleatory fluctuation of 10° on every agent's evacuation direction during the computation in order to reflect the incertitude that each evacuee will follow the route to the shelter, which may be provoked by lack of knowledge or guidance, congestion or damage along the road; and (4) a crowd potential parameter which makes the agent follow the direction in which there are other evacuees. In turn, the second input feature for the model is a file that describes the evacuation zone and its accessible (i.e., streets and open spaces) and non-accessible (i.e., buildings) areas. The third input includes the number and location of the shelters (four in this case, according to ONEMI; see S1, S2, S3, and S4 in Figure 1). Lastly, the fourth input includes the number, location and pointing direction of the evacuation signposts in the study area (90, as surveyed on the field), which can change the agents' moving direction for any agent located close enough to them (i.e., at least 50 m).
The original PARI-AGENT code was modified to include two new parameters to enhance the simulation's similarity to a real-world scenario. The first one was the slope effect on the agents' speed, i.e., the steeper the gradient, the slower the movement (for both uphill and downhill movement), according to Tobler's exponential hiking function (Tobler, 1993). The second new parameter for the model was a delayed or slow starting time for each evacuee, reflecting the fact that a slow evacuation (fully triggered only by the imminent threat of an arriving tsunami) can be the predominant behavior during an emergency (Mas et al., 2013). To randomly assign a starting time to each agent, a Rayleigh cumulative distribution was considered, with a mean departure time of 10 min after the beginning of the tsunamigenic earthquake, which reflects the common time gap between the earthquake occurrence and the tsunami warning as released by ONEMI.
To reduce computing times and the consumption of computer memory, the Población Vergara district was divided for analysis into six evacuation zones (numbered 109, 110, 111, 112, 122, and 123, see Figure 1), according to the division established by the previously mentioned origin-destination study for Viña del Mar. Each of these zones was examined separately with the model, following the approach proposed by Imamura et al. (2012). The exceptions to this approach were zones 109 and 110, which were examined together as they constitute a unique and clearly defined urban area.
During each run, the model begins by reading the input parameters- the agents' characteristics, starting times, evacuation area, and shelter locations. Then, it runs the computation of the optimal route for each agent, given its initial position and closest shelter; for this, the code uses the A* algorithm (Yao et al., 2010). After that, the model begins to calculate every agent's position at each time step (1 s). For that, the code reads the water height (from the inundation file) and computes the velocity of each agent according to various parameters (speed, slope, random walk, crowd and signpost influence). Once the new position is assessed, the model updates the agent's status: (1) moving (i.e., alive), (2) dead (i.e., reached by the water), and (3) escaped (i.e., alive in the shelter). When the model's running time overpasses the pre-established final time (2,700 s or 45 min, i.e., the required time to reach the maximum tsunami penetration inland) the computation stops. As three of the model's parameters were aleatory (the initial spatial distribution of agents, the walking speeds, and the starting times), 20 simulation rounds were carried out to obtain average values of the number of escaped, moving and dead evacuees after 45 min. This number of rounds was sufficient to achieve for every zone and scenario a 95% confidence interval with a margin of error <1% in these quantities.
The model's average results (summarized in Table 1 and Figure 4) show that in every zone examined a large percentage of evacuees (between 82.3 and 100% during a daytime scenario, and 82.1 and 100% during a night-time one) can reach a shelter during the first 45 min of tsunami propagation, thus saving their lives. This overall positive result is due to Población Vergara's nearby high ground and its orthogonal, dense and redundant grid of streets that provide short and straight routes between vulnerable and safe areas thus enhancing wayfinding. Nevertheless, the findings also show that zones 109+110 (located on the south-western part of the district) might find significant difficulties to successful evacuation in case of a near-field tsunami. Their vulnerable location combines a long distance to the closest shelter (~2.1 km) with a limited ground elevation (between 4 and 6 m) and the proximity of the Marga-marga creek. These conditions imply that after 45 min of tsunami propagation, about 807 people could lose their lives during a daytime emergency, and 729 during a night-time one. Moreover, while ONEMI's preparedness efforts aim to evacuate Chilean coastal areas in <15 min during a near-field tsunami, the model shows that this milestone might be very difficult to achieve in evacuation zones 109+110 (2.2% of daytime and 2.1% of night-time safe evacuees, respectively, in 15 min), 111 (31 and 31%), and 123 (31.6 and 54.5%). See Figure 4.
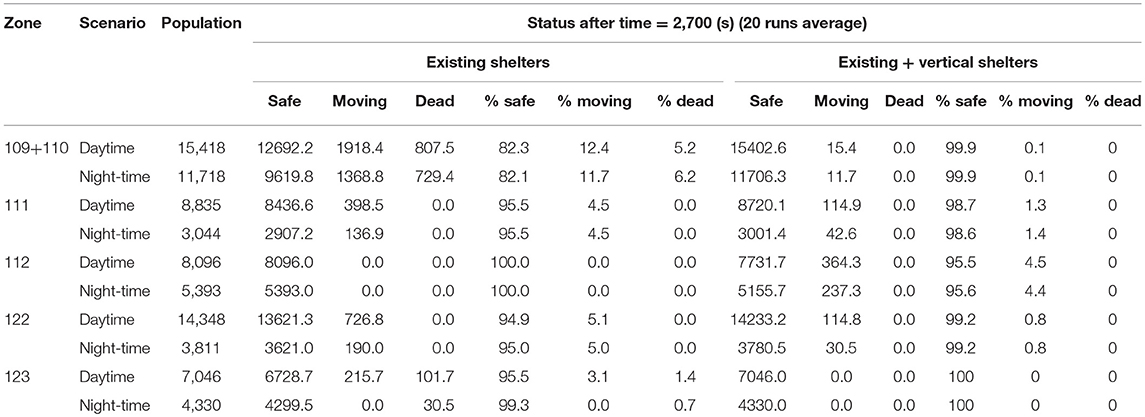
Table 1. Average percentage of safe, moving and dead evacuees after 2,700 s of tsunami propagation, for every evacuation zone and scenario, not-including and including vertical shelters.
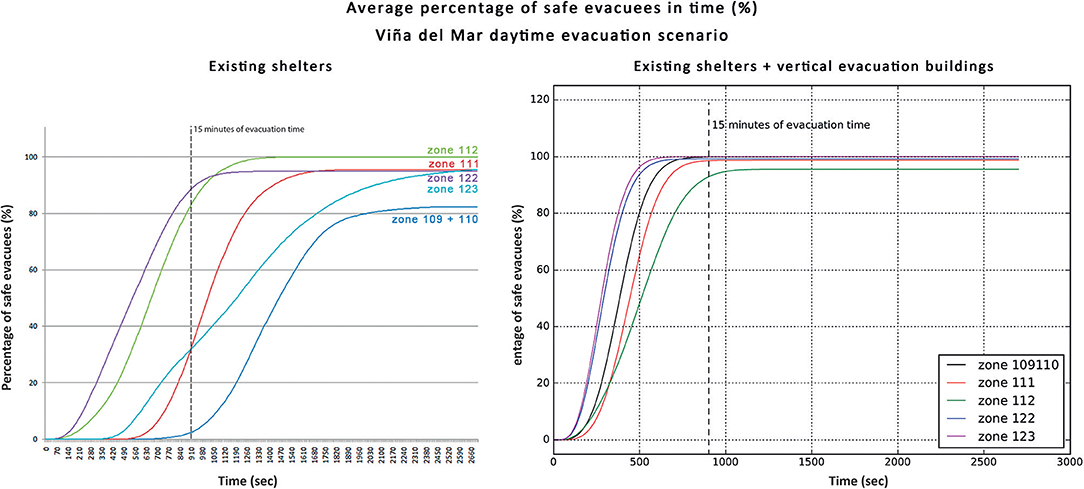
Figure 4. Average percentage of safe evacuees vs. time for every evacuation zone in Población Vergara, during daytime scenario, with only existing shelters (Left) and also including vertical evacuation buildings (Right).
Micro-Scale Analysis
It was argued above that much of contemporary efforts on assessing the supportive role of urban forms (and their physical features) during tsunami evacuations have been focused on large-scale urban configuration. However, despite the underlined need for careful examination of the pivotal micro-scale of the evacuees' spatial experience along routes and in shelter areas (Taubenböck et al., 2009; León and March, 2016), few studies have undertaken such a task (see for instance Reyes and Miura, 2015). Moreover, examples of integration between both scales of analysis cannot be currently found in the literature. This paper aims to help bridge this gap by enhancing the macro-scale analysis with a methodology for micro-scale studies previously tested by the authors in the tsunami-prone city of Iquique, Chile (Álvarez et al., 2018).
During 2016, a fieldwork protocol was applied in the Población Vergara district to survey the likely spatial experience of pedestrian evacuees as they successively interact with changing urban features. Hence, each evacuation route's micro-scale conditions were examined by using sequential trajectories (Cullen, 1961; Clay, 1994) to identify the most relevant risk factors within the built urban environment, capable of hindering the population's evacuation and sheltering actions during a tsunami emergency. The criteria to identify these micro-vulnerabilities were established around two factors. First, according to relevant bibliography on the safety- and evacuation-related characteristics of built indoor and open spaces during earthquakes, tsunamis and other types of hazards (Ciborowski, 1982; Preuss et al., 2001; Ercolano, 2008; Scheer et al., 2012). Secondly, they were based on examiners' reports about the large-scale tsunami evacuation drills carried out by ONEMI in 2013 in the coastal regions of Tarapacá and Biobío, Chile, that underline several built environment's characteristics that hindered or endangered the evacuation of the population (Walker, 2013a,b).
Approximately 29.2 km of evacuation routes were surveyed, measured and then mapped in ArcGIS. According to their causes, the micro-vulnerabilities identified along these routes were classified into three categories: (1) misuse (e.g., car parking on sidewalks); (2) poor maintenance (e.g., cracked roads); and (3) design problems (e.g., narrow sidewalks, uneven steps, and misallocated urban furniture). As these features can have different impedance values on walking speeds (from complete blockage to only a minor reduction), the works of Fujiyama and Tyler (2004), Soule and Goldman (1972) and Schmidtlein and Wood (2015) were used as references to propose the following weighting scheme for each of them in order to obtain an overall “obstructed area” for each route (see Table 2).
Table 3 shows the outcomes of this analysis for every evacuation route in the district, including four different cases of study: (1) percentage of overall escape area occupied by micro-vulnerabilities, with uniform weighting; (2) percentage of pedestrian-only escape area occupied by micro-vulnerabilities, with uniform weighting; (3) percentage of overall escape area occupied by micro-vulnerabilities, weighted by impedance factors; and (4) percentage of pedestrian-only escape area occupied by micro-vulnerabilities, weighted by impedance factors.
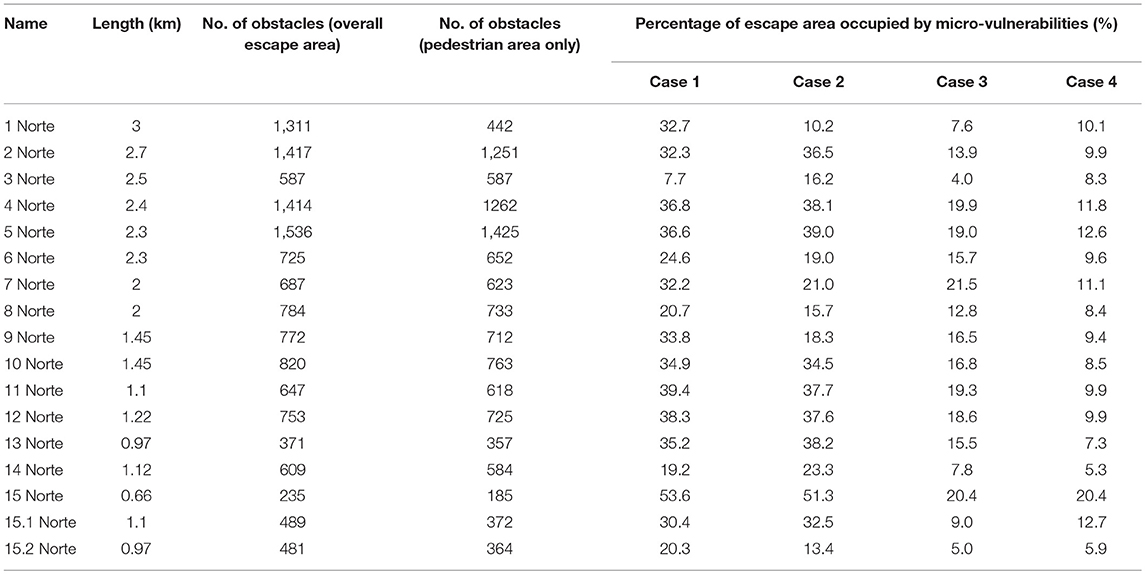
Table 3. Summary of micro-vulnerabilities existing along evacuation routes, Población Vergara district.
Figure 5 shows a satellite image of the study area, including a three-color classification scheme for the surveyed evacuation routes, according to Case 4 results. It can be considered that this case resembles a likely evacuation scenario, as the only guaranteed escape area is the one provided by pedestrian spaces (sidewalks, squares, parks, etc.). Even though pedestrian-only evacuations are strongly recommended by Chilean emergency managers, recent experiences in Viña del Mar showed a significant use of cars during evacuation (Hernández et al., 2017). In case of a real tsunami emergency, this behavior might lead to serious traffic jams and unavailability of pedestrian space along roads.
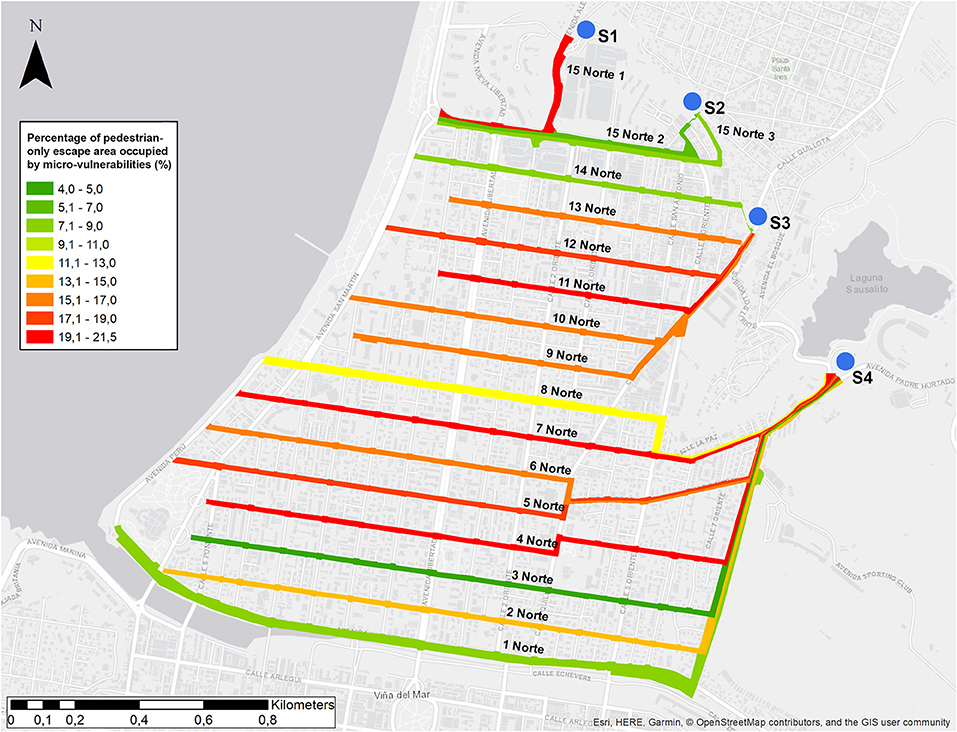
Figure 5. Classification scheme for Población Vergara's evacuation routes, according to existing micro-vulnerabilities.
The results above show a significant number of evacuee-scale features with a potential for hindering pedestrian evacuations in the Población Vergara district. On average, some type of micro-vulnerability covers 28.4% of the pedestrian areas in evacuation routes. While this percentage reduces to 10.1% when these are weighted areas according to their speed-diminishing capacities, some routes such as 5 Norte, 15 Norte, and 15 Norte (section Introduction) exhibit comparatively high values (12.6, 20.4, and 12.7%, respectively). 5 Norte provides one of the most direct paths of escape for zones 109+110 and 111, which have the potentially highest death rates according to the macro-scale model. 15 Norte and 15 Norte (section Introduction), in turn, are located next to the city's main commercial hub (zone 122, which doubles its population during rush hour). As it was discussed above, this area also exhibits comparatively high pedestrian route directness ratio values, which might lead to wayfinding issues among evacuees.
Vertical Evacuation as a Possible Path to Improvement
The previous macro-scale analysis shows significant levels of tsunami vulnerability for populations located in zones 109+110, 111, and 123, who might not be able to access safe high ground before being reached by an incoming tsunami. A possible path to improve these conditions is the use of existing high-rise buildings as vertical evacuation points, which were proven successful during the Great East Japan Earthquake and Tsunami in 2011 (Fraser et al., 2012). While this option is not explicitly sanctioned by the Chilean emergency management framework and has been deemed unfeasible by some Chilean emergency planners (Araneda, 2014), it is currently suggested by ONEMI as an alternative in case of imminent danger (ONEMI, 2014).
During fieldwork 14 buildings were identified in the vulnerable area (see V1–V14 in Figure 1) capable of serving as vertical evacuation points, according to the following requirements (Rojahn, 2004; Yagi and Hasemi, 2010; Fraser et al., 2012; ONEMI, 2014): (1) a reinforced concrete structure for guaranteeing seismic resistance; (2) a height of 30 m or at least 8 levels; (3) a guaranteed open access for people (at least during business hours); and (4) an adequate capacity (considering a required space for each evacuee of 0.8 m2). To develop an enhanced macro-scale analysis, another 20 simulation rounds of the agent-based model were undertaken, now including these 14 buildings as destination shelters (alongside the four previously mentioned ones). Table 1 and Figure 4 display the average outcomes of these simulations. These results clearly demonstrate the positive impact of including vertical evacuation shelters in the Población Vergara district. First, the likely human casualties during a near-field emergency are reduced to 0. Second, every examined zone shows a steep improvement of the evacuation rate, which implies that during the first 15 min more than 90% of evacuees can reach a safe destination, during both daytime and night-time scenarios. Third, the three most exposed zones (109+110, 111, and 123) increase the total percentage of safe evacuees during the model run (daytime and night-time), including full evacuation of zones 109+110 and 123.
Despite this overall improvement, it is also important to consider a series of micro-scale factors capable of determining the overall success of the evacuation process at each identified building. These might include (but no be restricted to) (Tubbs and Meacham, 2007; Yagi and Hasemi, 2010; Fraser et al., 2012): (1) an adequate number of entrances with appropriate characteristics (quantity, visibility from the streets, width) to direct access to the building's inner evacuation routes; (2) a design of the building's circulation system (i.e., internal/external stairs and corridors) capable of fulfilling both daily and emergency operating conditions (e.g., including enhanced widths, absence of obstacles, battery-backed lighting, etc.); and (3) provision of adequate evacuation signage for wayfinding including universal access standards. Lastly, it also important to consider social and behavioral factors that might compromise a successful vertical evacuation, for instance: unwillingness to enter a high-rise building following a strong earthquake, unhelpful attitudes by the original occupants, and panic or anti-social behaviors while inside the buildings.
While further micro-scale research is required to carefully evaluate if the previously identified vertical shelters in the Población Vergara district fulfill all the requirements presented above, a rapid on-site assessment casts doubts of this possibility. Chilean planning, design and building codes (MINVU, 2014) include an entire chapter (n° 2) of standards for emergency-response and evacuation. However, these are aimed at guaranteeing the safe egress of the buildings' occupants to the street, not the other way around. In case of a tsunami evacuation, hundreds of people coming from surrounding areas might enter each of these buildings looking for shelter, thereby doubling or tripling its usual number of inhabitants, and crowding their stairways, corridors and few available gathering spaces (for instance, roofs in Chilean high-rise buildings are usually not accessible due to security concerns). Solutions to these problems might range from retrofitting existing buildings to including vertical evacuation standards in the design of new ones. In both cases, physical measures should be accompanied by appropriate education, management and incentives schemes, such as Yagi and Hasemi (2010), Fraser et al. (2012), and González (2013): (1) trained personnel to guide the evacuations; (2) subsides or tax deductions for private buildings designed as shelters; (3) periodic inspections for these buildings; (4) trained caretakers to unlock the buildings' entrances around the clock; and (5) actions for community engagement (including buildings' owners) to foster awareness and preparedness.
Conclusions
This paper discussed the role of urban form as an essential tool for responding to rapid onset tsunamis, particularly by supporting safe and effective evacuations of the population. The paper examined the earthquake- and tsunami-prone city of Viña del Mar, Chile, on two different scales: the macro-scale of the urban configuration (including street pattern arrangements and location of safe areas) and the micro-scale of the built environment conditions experienced by the evacuees during a likely evacuation. To achieve this, a mixed-methods approach (including two computer-based models and fieldwork, on two different scales of analysis) was undertaken in the city's most vulnerable area to tsunamis: the Población Vergara district.
The results show that at the macro-scale the Población Vergara district's urban form is well-suited for rapid evacuations, as the result of its nearby high ground and its orthogonal, redundant grid of streets that provide short and straight routes between the vulnerable and safe areas (also enhancing wayfinding). The agent-based model shows that during the initial 45 min of tsunami propagation (the required time to achieve the maximum inland penetration of water) between 80 and 100% of the evacuees in the area can reach a safe destination (during both daytime and night-time scenarios). While a death toll of about 807 people (daytime) and 729 people (night-time) is expected in evacuation zones 109+110, alongside about 101 and 30 deaths (during daytime and night-time scenarios, respectively) in zone 123, these casualties are in those zones with the higher exposure to the tsunami threat. Moreover, the inclusion of 14 new vertical evacuation shelters could reduce this death toll to 0 and diminish from 35 to 20 min the required time to achieve more than 80% of safe evacuees in every evacuation zone.
It must be pointed out that this model does not consider the potential impact of real-time official information provided to the population (e.g., through mobile phone applications) on evacuation behaviors. In this respect, improved versions of the model could examine this impact, by comparing the performance of agents with and without access with this real-time data (for instance, examining reactions to warnings about blocked streets by debris or crowding).
Despite the overall positive scenario shown by the agent-based model, at the micro-scale of the evacuees' experience the analysis shows comparatively deficient spatial conditions, capable of hindering evacuation in case of an emergency. Obstacles (or micro-vulnerabilities) cover as much as 28.4% of the available evacuation area along the main escape routes, which might lead to dangerous outcomes and behaviors during an emergency, including bottlenecks, falls and panic. When weighting factors are applied to the micro-vulnerabilities according to their speed-diminishing capacity, it highlights the fact that some of the particularly at-risk routes are the same that allow escaping the Población Vergara's most vulnerable zones, i.e., 109+110. Moreover, fieldwork reconnaissance of (potential) vertical shelters' micro-scale characteristics showed that in general terms these are not designed or built to allow a safe ingress and sheltering of people.
It must be underlined that both the macro- and the micro-scale analyses undertaken in this paper examine pre-earthquake conditions; it is likely that these conditions will be significantly affected by the tsunamigenic earthquake, which might have a magnitude of 9.0 or even larger. Evacuation routes could be blocked or hindered by debris, and vertical shelters be damaged or inoperative. In this respect, further probabilistic analyses should be conducted to identify those elements in the evacuation network with larger possibilities of structural collapse, and to examine how these failures might impact on evacuation times (see for instance Castro et al., 2018). This, with the purpose of supporting risk reduction policies aimed at strengthening those critical points through retrofitting programs.
León and March (2016) underline four types of antecedent conditions that contribute to aggravate or mitigate urban vulnerability to tsunamis. In the case of Viña del Mar (and particularly the Población Vergara district), the phenomenon's long recurrence (last destructive tsunami occurred in 1730) has combined with siting decisions and inertia (i.e., both governmental and private actors fostering the high-density occupation of an exposed area) to build up a high vulnerability to tsunamis. At the same time, the district's morphological characteristics mitigate this condition by providing a sound (at least at the macro-scale) evacuation system to safe high ground. Nevertheless, recent chaotic experiences (e.g., the evacuations of 2010, 2015, and 2017, following large earthquakes and official warnings) cast doubt on the actual responses of the population and emergency systems during a real-life event.
Future work in this area could include, on the one hand, the development of strengthened and more accurate computer-based models to include the micro-scale of evacuees and the physical interactions among them, with their built environment and with the tsunami flood. In this respect, flood-evacuation coupled models (like the one used in this paper) currently have their spatial resolution limited by the exponentially elevated amount of computer processing required. On the other hand, future work could also include analyses of further case studies, leading to the identification of larger potentials for evacuation improvement, at both the micro- and macro-urban scales, according to pre-emptive design standards (Mazereeuw, 2011). These guidelines might encompass features such as street patterns, safe assembly areas, egress routes, population distributions, vehicular traffic, streetscape morphology, and signage.
Lastly, the findings of this paper pose significant implications for emergency planners and governmental stakeholders. As retrofitting changes in the built environment might takes years or decades to be accomplished, it is necessary to ensure that the vulnerable populations have the necessary training and knowledge to provide rapid and autonomous action responses to near-field tsunamis, within unchanged urban physical conditions. Moreover, this “ready-to-act” status should be sustained over time by thorough and widespread education and information-dissemination policies. In turn, these must be informed by science-based findings as those presented in this paper.
Author Contributions
The study was conceived by JL and CM, and the methodology was designed with the contribution of all authors. CF carried out the field survey with the supervision of JL and PC. The video records post-processing stage was developed by CF. CM carried out the data analysis. The first version of the manuscript was prepared by JL with reviews by PC and RC. All authors contributed to editing the final version of the article.
Conflict of Interest Statement
The authors declare that the research was conducted in the absence of any commercial or financial relationships that could be construed as a potential conflict of interest.
Acknowledgments
This research was supported by the Research Center for Integrated Disaster Risk Management (CONICYT/FONDAP/15110017) and the National Fund for Science and Technology, FONDECYT, Research Grant No. 11170024. We are also grateful for the aid provided by Dr. Taro Arikawa from PARI and Chuo University, Japan.
Figures 1, 4 and Table 1 are reprinted from León et al. (2018), with permission from Elsevier. Originally presented in the 7th International Conference on Building Resilience: Using scientific knowledge to inform policy and practice in disaster risk reduction, Bangkok, November 27–29, 2017.
Supplementary Material
The Supplementary Material for this article can be found online at: https://www.frontiersin.org/articles/10.3389/fbuil.2018.00089/full#supplementary-material
References
Alesch, D. J., and Siembieda, W. (2012). The role of the built environment in the recovery of cities and communities from extreme events. Int. J. Mass Emerg. Disasters 30, 197–211.
Alexander, D. (2004). “Planning for post-disaster reconstruction,” in I-Rec 2004 International Conference Improving Post-Disaster Reconstruction in Developing Countries (Coventry, UK), p. 1–12.
Allan, P., Bryant, M., Wirsching, C., Garcia, D., and Rodriguez, M. T. (2013). The influence of urban morphology on the resilience of cities following an earthquake. J. Urban Des. 18, 242–262. doi: 10.1080/13574809.2013.772881
Álvarez, G., Quiroz, M., León, J., and Cienfuegos, R. (2018). Identification and classification of urban micro-vulnerabilities in tsunami evacuation routes for the city of Iquique, Chile. Nat. Hazards Earth Syst. Sci. 18, 2027–2039. doi: 10.5194/nhess-18-2027-2018
Araneda, Y. (2014). Autoridades y expertos enfatizan en evacuación vertical en edificios. La Estrella Iquique 3.
Arikawa, T. (2015). “Development of high precision tsunami simulation based on a hierarchical intelligent simulation,” in Proceedings of the 34th JSST Annual International Conference on Simulation Technology (Toyama).
Bernard, E. N. (1995). Tsunami Hazard Mitigation: A Report to the Senate Appropriations Committee. Seattle, WA: National Oceanic and Atmospheric Administration.
Booth, R. (2002). El Estado Ausente: la paradójica configuración balnearia del Gran Valparaíso (1850–1925). EURE 28, 107–123. doi: 10.4067/S0250-71612002008300007
Bull-Kamanga, L., Diagne, K., Lavell, A., Leon, E., Lerise, F., MacGregor, H., et al. (2003). From everyday hazards to disasters: the accumulation of risk in urban areas. Environ. Urban. 15, 193–204. doi: 10.1177/095624780301500109
Burby, R., Beatley, T., Berke, P., Deyle, R., French, S., Godschalk, D., et al. (1999). Unleashing the power of planning to create disaster-resistant communities. J. Am. Plan. Assoc. 65, 247–258. doi: 10.1080/01944369908976055
Cáceres Quiero, G., Sabatini, F., and Booth, R. (2002). “La suburbanización de Valparaíso y el origen de Viña del Mar: entre la villa balnearias y el suburbio de ferrocarril (1870–1910),” in Las Puertas al Mar: Consumo, Ocio y Política en Mar del Plata, Montevideo y Viña del Mar, ed E. Pastoriza (Buenos Aires: Editorial Biblos), 33–46.
Cáceres, G., and Sabatini, F. (2003). Para entender la urbanización del litoral:: el balneario en la conformación del Gran Valparaíso (siglos XIX y XX). ARQ 50–52. doi: 10.4067/S0717-69962003005500013
Carvajal, M., Cisternas, M., and Catalán, P. A. (2017). Source of the 1730 Chilean earthquake from historical records: implications for the future tsunami hazard on the coast of Metropolitan Chile. J. Geophys. Res. Solid Earth 122, 3648–3660. doi: 10.1002/2017JB014063
Castro, S., Poulos, A., Herrera, J. C., and de la Llera, J. C. (2018). Modeling the impact of earthquake induced debris on tsunami evacuation times of coastal cities. Earthq. Spectra. [Epub ahead of print]. doi: 10.1193/101917EQS218M
Chen, X., and Zhan, F. B. (2008). Agent-based modelling and simulation of urban evacuation: relative effectiveness of simultaneous and staged evacuation strategies. J. Oper. Res. Soc. 59, 25–33. doi: 10.1057/palgrave.jors.2602321
Ciborowski, A. (1982). Physical development planning and urban design in earthquake-prone areas. Eng. Struct. 4, 153–160. doi: 10.1016/0141-0296(82)90003-7
Clay, G. (1994). Real Places: An Unconventional Guide to America's Generic Landscape. Chicago, IL: University of Chicago Press.
Comfort, L., Wisner, B., Cutter, S., Pulwarty, R., Hewitt, K., Oliver-Smith, A., et al. (1999). Reframing disaster policy: the global evolution of vulnerable communities. Environ. Hazards 1, 39–44. doi: 10.3763/ehaz.1999.0105
Comte, D., Eisenberg, A., Lorca, E., Pardo, M., Ponce, L., Saragoni, R., et al. (1986). The 1985 central chile earthquake: a repeat of previous great earthquakes in the region? Science 233, 449–453. doi: 10.1126/science.233.4762.449
Di Mauro, M., Megawati, K., Cedillos, V., and Tucker, B. (2013). Tsunami risk reduction for densely populated Southeast Asian cities: analysis of vehicular and pedestrian evacuation for the city of Padang, Indonesia, and assessment of interventions. Nat. Hazards 68, 373–404. doi: 10.1007/s11069-013-0632-z
Dill, J. (2004). “Measuring network connectivity for bicycling and walking,” in 83rd Annual Meeting of the Transportation Research Board (Washington, DC).
Ercolano, J. M. (2008). Pedestrian disaster preparedness and emergency management of mass evacuations on foot: state-of-the-art and best practices. J. Appl. Secur. Res. 3, 389–405. doi: 10.1080/19361610801981068
Fakhrurrazi, F., and Van Nes, A. (2012). “Space and Panic. The application of Space Syntax to understand the relationship between mortality rates and spatial configuration in Banda Aceh during the tsunami 2004,” in Eighth International Space Syntax Symposium (Santiago, CA).
Fraser, S., Leonard, G. S., Murakami, H., and Matsuo, I. (2012). Tsunami vertical evacuation buildings—Lessons for international preparedness following the 2011 Great East Japan Tsunami. J. Disaster Res. 7, 446–457. doi: 10.20965/jdr.2012.p0446
Fujiyama, T., and Tyler, N. (2004). An Explicit Study on Walking Speeds of Pedestrians on Stairs. Available online at: http://discovery.ucl.ac.uk/1243/1/2004_21.pdf (Accessed March 12, 2018).
Gencer, E. A. (2013). Natural Disasters, Urban Vulnerability, and Risk Management: A Theoretical Overview. Nueva York, NY; Heidelberg: Springer.
González, M. (2013). “Evacuación Vertical” en Chile: Una Alternativa Posible Para Evitar Víctimas Fatales en Caso de Tsunami. Available online at: http://ciperchile.cl/2013/07/01/“evacuacion-vertical”-en-chile-una-alternativa-posible-para-evitar-victimas-fatales-en-caso-de-tsunami/ (Accessed March 12, 2018).
González-Riancho Calzada, P., Aguirre Ayerbe, I., Aniel-Quiroga Zorrilla, Í., Abad Herrero, S., González Rodríguez, E. M., Larreynaga, J., et al. (2013). Tsunami evacuation modelling as a tool for risk reduction: application to the coastal area of El Salvador. Nat. Hazards Earth Syst. Sci. 13, 3249–3270. doi: 10.5194/nhess-13-3249-2013
Gusiakov, V. K. (2009). “Tsunami history: recorded,” in Tsunamis, eds E. N. Bernard and A. R. Robinson (Cambridge: Harvard University Press), 23–54.
Haigh, R., and Amaratunga, D. (2010). An integrative review of the built environment discipline's role in the development of society's resilience to disasters. Int. J. Disaster Resil. Built Environ. 1, 11–24. doi: 10.1108/17595901011026454
Handy, S., Paterson, R. G., and Butler, K. (2003). Planning for Street Connectivity: Getting from Here to There. Chicago, IL: American Planning Association.
Hernández, L., Johnson, C., Norvell, C., and Rubinos, Á. (2017). Tsunami Evacuation Study|Viña del Mar Zone 4. Oakland, CA: Earthquake Engineering Research Institute.
Hillier, B., Penn, A., Hanson, J., Grajewski, T., and Xu, J. (1993). Natural movement: or, configuration and attraction in urban pedestrian movement. Environ. Plan. B Plan. Des. 20, 29–66. doi: 10.1068/b200029
Hoss, F., Jonkman, S. N., and Maaskant, B. (2011). “A comprehenisve assessment of multilayered safety in flood risk management—The Dordrecht case study,” in 5th International Conference on Flood Management (Tokyo: IAHS Publisher), 57–65.
Imamura, F., Muhari, A., Mas, E., Pradono, M. H., Post, J., and Sugimoto, M. (2012). Tsunami disaster mitigation by integrating comprehensive countermeasures in Padang City, Indonesia. J. Disaster Res. 7, 48–64. doi: 10.20965/jdr.2012.p0048
IPCC (2012). Managing the Risks of Extreme Events and Disasters to Advance Climate Change Adaptation. Cambridge: Cambridge University Press.
Joerin, J., and Shaw, R. (2010). “Climate change adaptation and urban risk management,” in Climate Change Adaptation and Disaster Risk Reduction: Issues and Challenges, eds R. Shaw, J. Pulhin, and J. Pereira (Bingley: Community, Environment, and Disaster Risk Management), 195–215.
Kazusa, S. (2004). Tsunami and Storm Surge Hazard Map Manual. Tokyo: Cabinet Office Disaster Management.
Klüpfel, H., and Schreckenberg, M. (2003). A Cellular Automaton Model for Crowd Movement and Egress Simulation. Ph.D. thesis, University of Duisburg-Essen.
León, J., and March, A. (2014). Urban morphology as a tool for supporting tsunami rapid resilience: a case study of Talcahuano, Chile. Habitat Int. 43, 250–262. doi: 10.1016/j.habitatint.2014.04.006
León, J., and March, A. (2016). An urban form response to disaster vulnerability: improving tsunami evacuation in Iquique, Chile. Environ. Plan. B Plan. Des. 43, 826–847. doi: 10.1177/0265813515597229
León, J., Mokrani, C., Catalán, P., Cienfuegos, R., and Femenías, C. (2018). Examining the role of urban form in supporting rapid and safe tsunami evacuations: a multi-scalar analysis in Viña del Mar, Chile. Proc. Eng. 212, 629–636. doi: 10.1016/j.proeng.2018.01.081
Little, R. G. (2002). Controlling cascading failure: understanding the vulnerabilities of interconnected infrastructures. J. Urban Technol. 9, 109–123. doi: 10.1080/106307302317379855
Lomnitz, C. (1970). Major earthquakes and tsunamis in Chile during the period 1535 to 1955. Int. J. Earth Sci. 59, 938–960. doi: 10.1007/BF02042278
March, A., and Henry, S. (2007). A better future from imagining the worst: land use planning & training responses to natural disaster. Aust. J. Emerg. Manag. 22, 17–22.
March, A., and León, J. (2013). “Urban planning for disaster risk reduction: establishing 2nd wave criteria,” in State of Australian Cities, eds K. Ruming, B. Randolph, and N. Gurran (Sydney, NSW: State of Australian Cities Research Network).
Mas, E., Adriano, B., and Koshimura, S. (2013). An integrated simulation of tsunami hazard and human evacuation in La Punta, Peru. J. Disaster Res. 8, 285–295. doi: 10.20965/jdr.2013.p0285
Mas, E., Koshimura, S., Imamura, F., Suppasri, A., Muhari, A., and Adriano, B. (2015). Recent advances in agent-based tsunami evacuation simulations: case studies in Indonesia, Thailand, Japan and Peru. Pure Appl. Geophys. 172, 3409–3424. doi: 10.1007/s00024-015-1105-y
Mazereeuw, M. (2011). Preemptive landscape—A prototype for coastal urbanization along the pacific ring of fire. Topos Eur. Landsc. Mag. 76:82.
Mazereeuw, M. (2015). In conversation with Miho Mazereeuw. J. Landsc. Archit. 10, 36–37. doi: 10.1080/18626033.2015.1011440
Menoni, S., and Pesaro, G. (2008). Is relocation a good answer to prevent risk?: criteria to help decision makers choose candidates for relocation in areas exposed to high hydrogeological hazards. Disaster Prev. Manag. 17, 33–53. doi: 10.1108/09653560810855865
Mohareb, N. I. (2011). Emergency evacuation model: accessibility as a starting point. Proc. Inst. Civ. Eng. Urban Des. Plan. 164, 215–224. doi: 10.1680/udap.2011.164.4.215
Murao, O. (2008). “Case study of Architecture and Urban design on the disaster life cycle in Japan,” in 14th World Conference on Earthquake Engineering Proceedings (Beijing).
Murata, S., Imamura, F., Katoh, K., Kawata, Y., Takahashi, S., and Takayama, T. (2010). Tsunami. To Survive from Tsunami. Singapore: World Scientific Publishing Co., Pte. Ltd.
NTHMP (2001). Designing for Tsunamis. Seven Principles for Planning and Designing for Tsunami Hazards. Miami, FL: NOAA; NTHMP.
Oliver-Smith, A. (1991). Successes and failures in post-disaster resettlement. Disasters 15, 12–23. doi: 10.1111/j.1467-7717.1991.tb00423.x
Pelling, M. (2003). The Vulnerability of Cities: Natural Disasters and Social Resilience. Sterling: Earthscan Publications. Available online at: http://www.loc.gov/catdir/toc/fy037/2003000697.html
Preuss, J. (1988). Planning for Risk: Comprehensive Planning for Tsunami Hazard Areas. Urban Regional Research, National Science Foundation.
Preuss, J., Raad, P., and Bidoae, R. (2001). “Mitigation strategies based on local tsunami effects,” in Tsunami Research at the End of a Critical Decade, ed G. T. Hebenstreit (Dordrecht: Kluwer Academic Publishers), 47–64.
Randall, T. A., and Baetz, B. W. (2001). Evaluating pedestrian connectivity for suburban sustainability. J. Urban Plan. Dev. 127, 1–15. doi: 10.1061/(ASCE)0733-9488(2001)127:1(1)
Reyes, M., and Miura, F. (2015). “A proposal for qualitative and quantitative analysis methods for vertical evacuation from a tsunami in coastal cities” in Coastal Management: Changing Coast, Changing Climate, Changing Minds, ed A. Baptiste (Amsterdam: ICE Publishing), 97–108. doi: 10.1680/cm.61149.097
Rojahn, C. (2004). Vertical Evacuation from Tsunamis: A Guide for Community Officials. FEMA Redwood City, CA: FEMA.
Ruiz, S., and Madariaga, R. (2018). Historical and recent large megathrust earthquakes in Chile. Tectonophysics 733, 37–56. doi: 10.1016/j.tecto.2018.01.015
Scheer, S., Varela, V., and Eftychidis, G. (2012). A generic framework for tsunami evacuation planning. Phys. Chem. Earth 49, 79–91. doi: 10.1016/j.pce.2011.12.001
Schmidtlein, M. C., and Wood, N. J. (2015). Sensitivity of tsunami evacuation modeling to direction and land cover assumptions. Appl. Geogr. 56, 154–163. doi: 10.1016/j.apgeog.2014.11.014
SECTRA (2016). Encuesta de Origen-Destino de Viajes Gran Valparaíso. Santiago: Ministerio de Obras Públicas, Transportes y Telecomunicaciones.
SHOA (2012). Proyecto CITSU. Available online at: http://www.shoa.cl/index.htm
Shuto, N. (2005). “Tsunamis: their coastal effects and defense works,” in Scientific Forum on the Tsunami, its Impact and Recovery, ed T. Tingsanchali (Bangkok, Thailand: Asian Institute of Technology), 1–12.
Shuto, N., and Fujima, K. (2009). A short history of tsunami research and countermeasures in Japan. Proc. Japan Acad. Ser. B 85, 267–275. doi: 10.2183/pjab.85.267
Soto, M., and Álvarez, L. (2012). Análisis de tendencias en movilidad en el Gran Valparaíso. El caso de la movilidad laboral. Rev. Geogr. Norte Gd. 52, 19–36. doi: 10.4067/S0718-34022012000200002
Soule, R. G., and Goldman, R. F. (1972). Terrain coefficients for energy cost prediction. J. Appl. Physiol. 32, 706–708. doi: 10.1152/jappl.1972.32.5.706
Southworth, M., and Owens, P. M. (1993). The evolving metropolis: studies of community, neighborhood, and street form at the urban edge. J. Am. Plan. Assoc. 59, 271–287. doi: 10.1080/01944369308975880
Taubenböck, H., Goseberg, N., Setiadi, N., Lämmel, G., Moder, F., Oczipka, M., et al. (2009). “Last-Mile” preparation for a potential disaster - Interdisciplinary approach towards tsunami early warning and an evacuation information system for the coastal city of Padang, Indonesia. Nat. Hazards Earth Syst. Sci. 9, 1509–1528. doi: 10.5194/nhess-9-1509-2009
Tobler, W. (1993). Three Presentations on Geographical Analysis and Modeling: Non-isotropic Geographic Modeling; Speculations on the Geometry of Geography; and Global Spatial Analysis. Santa Barbara, CA: National Center for Geographic Information and Analysis.
Tomita, T., Honda, K., and Kakinuma, T. (2006). Application of Storm Surge and Tsunami Simulator in Ocean and Coastal Areas (STOC) to Tsunami Analysis. Available online at: https://www.pwri.go.jp/eng/ujnr/joint/38/paper/38-41tomita.pdf (Accessed March 9, 2018).
Tsimopoulou, V., Jonkman, S. N., Kolen, B., Maaskant, B., Mori, N., and Yasuda, T. (2012). A multi-layered safety perspective on the tsunami disaster in Tohoku, Japan. in FLOODrisk 2012: The 2nd European Conference on FLOODrisk Management (Rotterdam, NL).
Tubbs, J. S., and Meacham, B. J. (2007). Egress Design Solutions. A Guide to Evacuation and Crowd Management Planning. Hoboken, NJ: John Wiley & Sons.
Twigg, J. (2004). Disaster Risk Reduction: Mitigation and Preparedness in Development and Emergency Planning. London: Humanitarian Practice Network.
United Nations (2014). World Urbanization Prospects: The 2014 Revision, Highlights. New York, NY: Department of Economic and Social Affairs, Population Division.
Walker, J.-M. (2013a). Informe Técnico de Evaluación. Simulacro Macrozona de Terremoto y Tsunami, Evacuación del Borde Costero, Regiones del Biobío, La Araucanía, Los Lagos y Aysén.
Walker, J.-M. (2013b). Informe Técnico de Evaluación. Simulacro Macrozona de Terremoto y Tsunami, Evacuación del Borde Costero. Regiones de Arica y Parinacota, Tarapacá, Antofagasta y Atacama.
Wamsler, C. (2006). Mainstreaming risk reduction in urban planning and housing: a challenge for international aid organisations. Disasters 30, 151–177. doi: 10.1111/j.0361-3666.2006.00313.x
Wilensky, U., and Rand, W. (2015). An Introduction to Agent-Based Modeling: Modeling Natural, Social, and Engineered Complex Systems with NetLogo. Cambridge, MA: MIT Press.
Wood, N., Jones, J., Schelling, J., and Schmidtlein, M. (2014). Tsunami vertical-evacuation planning in the US Pacific Northwest as a geospatial, multi-criteria decision problem. Int. J. Disaster Risk Reduct. 9, 68–83. doi: 10.1016/j.ijdrr.2014.04.009
Wood, N., Jones, J., Schmidtlein, M., Schelling, J., and Frazier, T. (2016). Pedestrian flow-path modeling to support tsunami evacuation and disaster relief planning in the U.S. Pacific Northwest. Int. J. Disaster Risk Reduct. 18, 41–55. doi: 10.1016/J.IJDRR.2016.05.010
Yagi, S., and Hasemi, Y. (2010). Requirements and verification methodology for the design performance of Tsunami-Hinan buildings (temporary tsunami refuge building). J. Disaster Res. 5, 591–600. doi: 10.20965/jdr.2010.p0591
Keywords: tsunami, urban form, evacuation, disasters, Chile
Citation: León J, Mokrani C, Catalán P, Cienfuegos R and Femenías C (2019) The Role of Built Environment's Physical Urban Form in Supporting Rapid Tsunami Evacuations: Using Computer-Based Models and Real-World Data as Examination Tools. Front. Built Environ. 4:89. doi: 10.3389/fbuil.2018.00089
Received: 24 July 2018; Accepted: 20 December 2018;
Published: 21 January 2019.
Edited by:
Izuru Takewaki, Kyoto University, JapanReviewed by:
Tiago Miguel Ferreira, University of Minho, PortugalPanshi Wang, University of Maryland, College Park, United States
Copyright © 2019 León, Mokrani, Catalán, Cienfuegos and Femenías. This is an open-access article distributed under the terms of the Creative Commons Attribution License (CC BY). The use, distribution or reproduction in other forums is permitted, provided the original author(s) and the copyright owner(s) are credited and that the original publication in this journal is cited, in accordance with accepted academic practice. No use, distribution or reproduction is permitted which does not comply with these terms.
*Correspondence: Jorge León, am9yZ2UubGVvbkB1c20uY2w=