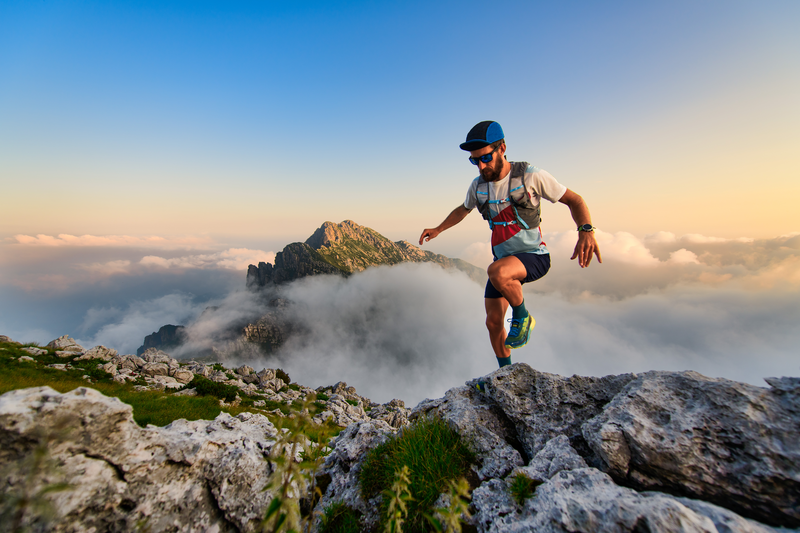
94% of researchers rate our articles as excellent or good
Learn more about the work of our research integrity team to safeguard the quality of each article we publish.
Find out more
REVIEW article
Front. Blockchain , 21 February 2020
Sec. Blockchain for Good
Volume 3 - 2020 | https://doi.org/10.3389/fbloc.2020.00006
This article is part of the Research Topic Blockchain for Food and Agriculture View all 4 articles
This study aims to investigate the application potential of blockchain technology in the agri-food industry, by following a case study approach focused on the early adopter companies. As the topic is emerging, there are not enough academic papers available to warrant a systematic literature review. To confirm this hypothesis and to inform to better understand guide this research, we first conducted a pre-literature review. Based on its outcomes, we formulated three research questions, and developed a protocol to identify case studies, and subsequently analyzed them to answer our questions. We identified and studied six case studies, in which blockchain has been used to address issues of trust and transparency, and to facilitate information sharing among agri-food chain stakeholders. While blockchain adoption is still in its infancy, governance issues are important, as broader partnerships are required for successful, sustainable applications.
Nowadays, agri-food supply chains are structured, global and interconnected. Compliance data and food documentation on safety, sustainability, provenance, and other attributes are typically stored on paper or private databases, and can only be inspected by trusted third-party authorities (Trienekens et al., 2012). This situation makes access to data to be costly, extended, subject to fraud, corruption or error, causing threats of foodborne disease and/or financial losses (Beulens et al., 2005; Aung and Chang, 2014). The industry in collaboration with government, independent watchdogs, and consumer associations intervened to enable better transparency of information and build trust among stakeholders in agri-food supply chains (Beulens et al., 2005; Boucher et al., 2017; Brivio and Malinowska, 2017; Calatayud, 2017; Coleman, 2017; Kersten et al., 2017).
Despite the continuing trend of digitalization of the economy, agri-food is still one of the less digitalized industries (Manyika et al., 2015). Blockchain technology could potentially affect this situation in many ways, clustered in four directions: first, the food sector could benefit from decentralized, self-executing, digital smart contracts to automate transaction processing and certification among supply chain actors (Wright and De Filippi, 2015). Smart contracts may also contribute toward automating the role of regulatory bodies and information exchange interactions in the agri-food sector, however there are growing concerns about the quality of the reported data, and the validity and consistency of smart contracts (Ge et al., 2017). Second, blockchain may facilitate hardware and software integration, leading potentially to better system integration and performance (Kim, 2017; Kim and Laskowski, 2018). Third, blockchain offers an immutable record of chain transaction, accessible across (and sometimes beyond) chain peers (Louise Lemieux, 2015). Thus, it has been seen as an instrument for creating more trust among chain actors in the sector (Buterin, 2015b; Zhao, 2017), thanks to the easier auditability of records. Fourth, blockchain technology could enhance the traceability and visibility of goods within the supply chain, by tracking goods from the farm to fork. For instance, as IBM claims (Lubowe and McDermott, 2016) “long before the wheat arrives on the factory floor,” retailer managers could access the data and plan the inventory or the production. Many companies in the agri-food sector, such as Walmart, Alibaba, and JD.com have demonstrated their interest in blockchain technology applications, mainly for track-and-trace products (Parker, 2016; Zhao, 2017). Auchan Retail and Carrefour Italia reported that have implemented food tracking systems with blockchain (Auchan Retail, 2018; Carrefour Italia, 2018).
This paper aims to systematically investigate the application potential of blockchain technology to solve issues in the agri-food industry by looki’ng into the early adopter companies. Section “Background” briefly introduces the blockchain technology and demonstrates its functionality. In Section “Research Method,” we introduce the methodology we developed to conduct this research, that involves a pre-literature review and investigating a set of case studies. In Section “Blockchain Applications Potentially Relevant for Agri-Food,” we present the pre-literature review findings, and identify potential issues in the agri-food industry that can be addressed using blockchain technology. Section “Blockchain Approaches in the Agri-Food Domain” presents the companies we used as case-studies, and their approaches to implement blockchain technology in the agri-food industry. Section “Discussion,” provides a discussion about the state of practice and the current open issues related to blockchain use in the agri-food sector. Section “Conclusion” concludes the paper.
Blockchain technology is a type of distributed ledger, that has been used for deploying cryptocurrencies as Bitcoin. The blockchain constructs chronological chains data in an irreversible and immutable manner (Mainelli and Smith, 2015; Swanson, 2015; Swan, 2016; Walport, 2016). Data are organized in blocks, and to add a new block to the chain, nodes of the blockchain need to reach consensus. A consensus is a system that assures that all the users involved in the chain agree upon a specific state of the system as its true state. All the blocks that are confirmed and validated via the consensus mechanism are linked together from the first to the last validated block, hence the name blockchain (Christidis and Devetsikiotis, 2016; Croman et al., 2016; Eyal et al., 2016; IBM, 2016; Cachin and Vukolić, 2017; Crouch, 2017).
Blockchain systems rely on several technologies and the various deployments use different combinations of them. Most commonly blockchain applications are associated with smart contracts, peer-to-peer networks, distributed ledgers, and consensus mechanisms (IBM, 2016; Jim Brill et al., 2016; Palfreyman, 2016b). Smart contracts where originally created by Szabo (1997), and can be considered as a set of rules agreed between parties that are automatically enforced when some conditions are met (Brown, 2015; Perugini and Dal Checco, 2015; Idelberger et al., 2016; Swan, 2016). A peer-to-peer network is a connected network of users that exchange information by being both a client and server (Yang and Yang, 2014). Blockchain is a decentralized or distributed network that uses individual nodes to store and distribute the information directly to each connected peer (Lansiti and Lakhani, 2017). Distributed ledger or shared ledger is an immutable, redundant, shared database that records and duplicates data that are potentially spread across different countries, users, institution, or sites (Mainelli and Smith, 2015; Swanson, 2015; Walport, 2016). Consensus is a governance process that uses a decentralized quorum structure in which nodes/members develop and agree to a decision. In the case of blockchains what is at stake is the true history of information. Blockchains enable information systems to be distributed, transparent, immutable, and democratic. These four pillars of blockchain technology allowed it to rise in the past years and it was introduced in a broad spectrum of industries, from finance to supply chain (Lansiti and Lakhani, 2017). Some examples of blockchain applications beyond cryptocurrencies include deployments in the banking sector for inter-banking payments (Guo and Liang, 2016; Tschorsch and Scheuermann, 2016), and in the real-estate domain for property ownership (Huang and Carlsson, 2016).
There are several definitions in the literature about blockchain technology, which have been reviewed by Seebacher and Schüritz (2017). In the same work, Seebacher and Schüritz (2017) identified that blockchain technology bears a variety of characteristics that we summarized and interpreted in Table 1, below. Blockchain characteristics revolve around two main ones: evoking trust and its decentralized nature.
This study aims to investigate the application of blockchain technology in the agri-food industry, by following a case study approach focused on the early adopter companies. As the topic is emerging, very few case studies have been reported in peer-review literature, to warrant a systematic literature review. To confirm this hypothesis and to inform to better understand guide this research, we first conducted a pre-literature review. Based on its outcomes, we formulated three research questions, developed a protocol to identify case studies, and subsequently analyzed them to answer our questions.
First, a pre-literature review was conducted to identify the problems in the agri-food sector by looking into studies available in digital libraries, but also though other sources such as journals, gray literature, white papers, conference papers, and company research. This initial research was performed by following the guidelines of Rowley and Slack (2004) for conducting a literature review. We used the keywords blockchain agri-food, block chain, blockchain technology, track and trace, transparency, smart contract, provenance, distributed ledger, the consensus in the blockchain, trust issue in agri-food, supply chain risk, agri-food collaboration, agri-food trust. A further selection of papers was conducted using the recommendations of Rowley and Slack (2004), so that the articles should (a) be up-to-date, (b) be written by an authoritative author, (c) hold extensive source is referencing, (d) be relevant to the research subject. From the pre-literature review papers, we extracted the elements of blockchain used in the agri-food industry and classified them using the characteristics of Table 1. The findings of the pre-literature study are detailed in Section “Blockchain Applications Potentially Relevant for Agri-Food.”
Afterward, the following three research questions were formulated:
RQ1. What are the potential issues in the agri-food industry that could be addressed using blockchain technology?
RQ2. What are the possible blockchain approaches for solving the identified problems in the agri-food domain?
RQ3. What are the currently open issues in the state-of-the-practice of blockchain use?
A selection of the case studies was conducted using a multiple cases studies comparison approaches and a snowball sampling (Yin, 1994; De Vaus and de Vaus, 2001; Shakir, 2002). The snowball sampling1 was initiated from the companies listed on the portals of two major blockchain software providers and media advocacy, namely Ethereum and the Linux Foundation and Medium Corporation (Ethereum Foundation, 2018; Linux Foundation, 2018a,b,c; Medium, 2018). To analyze the case studies, we used an adaptation of Eisenhardt method by Maimbo and Pervan (2005) to create a protocol of analysis. The protocol outlines the procedures and rules to analyze the six case studies (Figure 1).
Using the protocol rules, we first looked at how companies claim to apply blockchain technology. Each company blockchain applications were studied via their white paper and analyzed in detail. Afterward, it was compared with the others, via three steps. First, we looked for common patterns between the case studies by using the keywords found in the pre-literature review. Secondly, an analysis of the case study was conducted to understand the pattern found. Thirdly, a cross-case studies analysis was conducted look for a standard pattern that could be missed in the first two analysis. These three steps helped to understand the differences and similarities of blockchain use to tackle the problems of the agri-food industry. Lastly, a summary of the findings was written explaining blockchain use for tackling problems in the agri-food industry.
In summary, this research framework aims to investigate the knowledge gap of the current application of blockchain technology in the agri-food industry following a mixed methods approach:
(a) A pre-literature review to find the potential issues in the agri-food industry that can be addressed using blockchain technology (see section “Blockchain Applications Potentially Relevant for Agri-Food”).
(b) Multiple comparison case studies to analyze the first wave of blockchain applications in the agri-food domain, reported in Section “Blockchain Approaches in the Agri-Food Domain,” and identify the challenges that have been addressed.
(c) A combination of case analysis and pre-literature review to describe the current open issues of blockchain in the state-of-the-practice, summarized in Section “Discussion.”
This chapter discusses the potential issues in the agri-food industry that can be addressed using blockchain technology by reporting on the pre-literature review findings, reported in Figure 2. The initial query yielded 307 papers. We further selected the primary studies that satisfy the criteria of Rowley and Slack (2004), as shown in Figure 2. Among them, only seven were related to blockchain applications potentially relevant for the agri-food industry. These seven papers are discussed below.
The study of Hackius and Petersen (2017) summarizes the key properties of blockchain technology (decentralization, verifiability, and immutability) and analyses four use cases about easing paperwork processing, identifying counterfeit products, facilitating origin tracking, and operating in the Internet of Things, in order to understand the possible benefits and implications of blockchain technology adoption. They also report an online survey targeting experts from the logistics and supply chain management, in order to investigate expert knowledge of the blockchain technology; the likelihood of adoption based on the four use cases; and their opinion on who is going to benefit from a blockchain implementation. They conclude that experts see a beneficial implementation and likelihood of adoption of blockchain, especially to ease paperwork processing and operate the Internet of Things, but they are reluctant to invest resources and time into an application. Moreover, they identified signals of a radical industry transformation as business process and models change. Hackius and Petersen approached blockchain technology by focusing on trust, low friction, automation, and shared and public aspects.
English and Nezhadian (2017) studied the architectural components of blockchain to design an effective supply chain management system. They identify the common problem and propose a solution, finding five principles to increase and optimize the efficiency of the supply chain management system. Their five principles mirror the blockchain characteristics of Table 1, and they are: pseudo-anonymity, redundancy (data replication), trust (distributed consensus), peer verification (proof of work), and Shared and Public (provenance of data). In their conclusion, they underline that a blockchain for supply chains should be a private one, previewing blockchain implementations can be able to solve the current problems of track-and-trace in supply chains with real-time information.
Kim and Laskowski (2018) point out that in the current track-and-trace systems do not provide transparency on the provenance of goods due to the international-spanning supply chain. They analyze the combination of the Internet of Things and blockchain technology through the contribution of the TOVE ontology and Ethereum smart contract for a better track-and-trace system. They underline the fundamental role of ontologies in creating blockchain applications for supply chains; as ontologies can lead to better data standards and business practices. Their system focuses on the following blockchain characteristics: automation (smart contract), redundancy (inter-organizational recordkeeping), low friction (lightweight financial systems), immutability (provenance tracking), shared and public (shared database of ontologies).
Tian (2016) analyses the combination of RFID (Radio Frequency Identification) and blockchain technologies for product traceability in agri-food supply chains. The system developed relies on RFID technology to gather data and blockchain technology to share and publish authentic information regarding the goods in the supply chain. This system also covers food quality and safety standard, to facilitate the control and the tracking of goods. Using Tian (2016) words: this traceability system could realize the information identification, inquiry, tracking, monitoring and tracing for the whole supply chain, and it could also be a secure, transparent and traceable platform for all the members in the agri-food supply chain. The solution reported by Tian approaches uses blockchain technology for its trust, cryptography, immutability, and decentralization characteristics.
Kumar and Iyengar (2017) studied an application scenario in the rice industry in India. In their study, they suggest a system implementation using blockchain to enable full traceability to combat food fraud. Their system aims to provide a complete history of across all five phases of the rice supply chain (production, procuring, processing, distributing, and retailing) and automate it using smart contracts. Data immutability and redundancy is expected to lower the system error and facilitate traceability of rice provenance, thus building trust among the actors of the supply chain. Authors claim, even if their system was not implemented, that blockchain technology will ensure the product safeness, increase the overall efficiency, and create better traceability. Using the characteristics of Seebacher and Schüritz (2017), their study looks into automation, trust, low friction, immutability, and redundancy of blockchain.
Lucena et al. (2018) built and analyzed a blockchain system application to track-and-trace grain quality for the Brazilian grain exporters’ business network. They developed a public permissioned blockchain using Hyperledeger Fabric. The supply chain actor transactions are automated via smart contracts, allowing the uniform application of common rules for shipping agreements. Authors conclude that the potential implementation of a blockchain system will help the grain supply chain reduce the information sharing costs among the actors, however legal obstacles may rise due to the newness of the technology. The shared and public, peer verification, immutability, redundancy, and cryptography are characteristics of the public permissioned blockchain with Hyperledger Fabric that have been used. The case study also focuses on trust, automation and low friction characteristics of the blockchain.
It should be noted that the above works do not always look specifically in the agri-food industry needs. Rather they focus on certain aspects that could be potentially enhanced in the food supply chain or the agri-food industry. This is due to the innate characteristics of the agri-food industry, where there are numerous actors, and their interactions are complicated and extensive. The blockchain technology aim to solve such problems by easing the paperwork process, helping to identify counterfeit products, facilitate the tracking of the provenance, automating process, enhancing the transparency and the transmission of information (English and Nezhadian, 2017; Hackius and Petersen, 2017; Haswell and Storgaard, 2017; Kim and Laskowski, 2018).
Table 2 summarizes the paper classification against the blockchain characteristics of Table 1. There are two blockchain characteristics present in all papers: trust and immutability. Immutability is related to data storage of a blockchain system. Instead, trust regards with the governance aspect of a blockchain. These characteristics are followed by shared and public, low friction, automation, and redundancy. None of the papers identified employed blockchain to make advantage of pseudonymity and versatility characteristics.
This chapter discusses the case studies identified via snowball sampling in the two main databases. Six companies were selected, as they implemented a blockchain system in real-world case scenarios in the agri-food sector, i.e., they have (self-)reportedly passed the “proof-of-concept” stage. These six companies exhibit high diversity in their application areas and the use of blockchain technology.
Provenance system aims to enhance the transparency of information among the supply chain peers, by guaranteeing that certification and standards are met by all the actors of the supply chain. The system is composed of six modular programs: registering, standards, production, manufacturing, tagging, and user-facing. These six modular programs are independently compiled but registered on the same blockchain, creating a co-existence environment within the same system. Blockchain is used to record the transactions by storing the data in a public and shared ledger allowing the chain to be auditable. Moreover, it allows the activation of smart contracts that facilitate the operation of the user within the chain also for monetary or information exchange (Provenance, 2015, 2016).
Provenance, in collaboration with the NGO Humanity United and International Pole and Line Foundation (IPNLF), conducted a 6 months project in Indonesia, to track-and-trace yellowtail tuna. The project aimed to use a mobile application together with blockchain technology and smart tagging to track-and-trace the origin and the authenticity of the social sustainability certification. The goal was to create a solid proof of compliance via a solution applicable throughout the supply chain. This would allow to prevent certification double-spending, and test the technology also from the consumer point of view. The whole system implemented data interoperability to track-and-trace items and certification in a secure, continuous, accessible system (Provenance, 2016). For tracking smart tags across the supply chain a point of sale (POS) was introduced. To register the fish, the fishermen needed to send an SMS, with this SMS a new asset is created in the chain with a permanent and unique ID. The unique ID is physically attached to the fish caught using a QR Code, RFID tag, or other technologies. Afterward, the digital asset moves to the supplier along with the catch, and a digital transaction is registered on the blockchain. The identity of the fishermen is saved as well. Tracking and tracing can be verified by exploring the blockchain with public software libraries.
In this case, blockchain was used for sharing information across all stakeholders of the chain, including fishermen, factories, certifier, and consumers. Blockchain was used both for the identification of both physical goods and validating certification. System integration with existing large-scale enterprise resource planning (ERP) was one of the challenges, as most of them do not follow the product throughout the whole supply chain. The unique ID in the form of an address on the blockchain was registered at the beginning of the chain, and propagated across the chain, allowing for matching data across data silos. Having data stored on the blockchain, also enabled it to operate as backend layer on the top of the existing ERP systems, and was used as an audit tool. The backend functionality of the blockchain allowed data to be shared and collected from the first mile with an end-to-end record system. Also using smart sticker and smart packing, the end consumer can use their smartphones to track the provenance of their tuna.
This case study considers the application of Ambrosus in the olive oil industry in France. The stakeholders of this supply chain are the olive farmer, the first processor at the olive mill, the packaging at the factory, the supplier, and the retailer. The first step of the process is when the farmer transports the olives to the olive mill, and this is also when counterfeiting may occur. Adulteration can be more difficult to detect, and there is no unique method to spot all the types of contamination. A traceability management system, called GestOlive, aims to address such problems by collecting information, entered manually by the producer. The system does not follow the product after it lefts the mill.
The alpha version of Ambrosus uses a ‘hardware-in-place’ approach to analyze methods of phenolic compounds,α-tocopherol and oleic acid already exist (Ambrosus, 2017b). Also, RFID tags are used to uniquely identify the product items. The unique identifier are associated with process and transportation information, stored in a blockchain. Consumers can access information stored in the blockchain via QR codes.
Ambrosus architecture is based on Amber, a token that follows food products alongside supply chains, recording and handling sensor data through the combination of an Ethereum smart contract and blockchain solutions. The system enables quality assurance across the supply chain, by allowing a complete and rapid digital transformation of the chosen quality parameter into digital content on the Ethereum blockchain. The system is integrated with a detection system sensor that generates the certificates. The sensor is implemented via smart packaging or assembled with an RFID tag, or bio-tracers inside the packaging (Ambrosus, 2017a). These certificates are then stored on the blockchain (Ambrosus, 2017a, c; Versetti and Meyer, 2017).
The company OriginTrail introduced the concept of traceability in the supply chain using blockchain for storing supply chain data. This system runs on a token economy, the trace token system. It tokenizes data exchange and the supply chain processing functionalities. The project uses Ethereum to ensure the proof of concept and the initial set-up. The company system works around two principles:
(a) Seamless and automatic data connection and interoperability between IT systems of different stakeholders in multi-organization supply chains, with consensus mechanisms for ensuring the integrity of data;
(b) a decentralized public solution for performance, cost and scalability issues by providing a tailored decentralized system for supply chain data based on the blockchain (Rakic et al., 2017).
The regulatory environment of the dairy industry already pushes the company to have a state of the art of the traceability system. The dairy sector mainly focuses on the origin of the milk, and the IT and ERP system is configured for tracing static data. OriginTrail transformed the current IT system to provide the origin of the products and its ingredient to the final user. They have separated the production and data keeping processes, in order to create a complete tracking system. Moreover, they used the packing to gather data, via the EAN code on the packaging. Also, to gather data, they look for the correct proxies to obtain the same type of information that is shared all over the supply chain. They look into the IT/ERP systems for the truck routes, they manually insert data regarding milk farmers, and the rest of information was accessed by the marketing department. Afterward, OriginTrail started to optimize the system for dynamic data. The dynamic data were about the routes and farm included in the dairy production in a specific time frame. The data were input in an XML form. Static data, like name, description, photo, nutritional values of products etc., were also included in the OrginTrail system.
Data are collected via a web interface data. For every incoming sourcing file a hash was created and stored on the Ethereum. Data are still stored centrally, as for this use case a decentralized solution for data storage was inefficient and non-cost effective.
TE-FOOD aims to enhance food traceability in emerging markets. The TE-FOOD system has three layers: a blockchain layer, an off-chain data layer, and a client application layer. The blockchain layer is divided into three subsystems: a market area for exchange called TFOOD market, a management system for the unique ID, and traceability and food quality ledger. The off-chain data layer is composed by the alerts and reporting by actors in the supply chain. The client application layer supports both TE-FOOD and third-party apps. The system includes both hardware and software components. For the hardware, it provides identification tools such as plastic security seals (1D/2D barcodes), label stickers (2D barcodes), RFID tags, printed paper bags (2D barcodes), and TE-FOOD scale labels (2D barcodes). For the software part, TE-FOOD provides a web solution for all the actors in the supply chain.
Regarding the blockchain technology, TE-FOOD provides immutable and public shared data system for the supply chain actors via a token system. The utility of the token is multiple. First, it is used as payment for transactions. Secondly, it is used as a value system for exchanging information between companies. Thirdly, the consumer will be rewarded to use the app to read QR, and she can spend tokens to order food analysis. Also, token holders can rate suppliers in the blockchain, to create a reputation/scoring system. All the data gathered via the token system will be stored in a public ledger (TE-FOOD, 2017).
The first TE-FOOD implementation was done in Ho Chi Minh City to track-and-trace pork meat. The implementation was carried out via a mobile app taking into account all the actors of the supply chain (farms, agents, slaughterhouses, wholesalers, food producers, markets and retailers, veterinaries, and authority). The animal was identified by a unique ID and QR-code that was stored on the blockchain via the mobile app. Another unique ID and QR-Code was given to the truck transporting the pig after been bought by the agents, and checked by the veterinary. At the slaughterhouse, the QR-code and ID are checked via the app for the authenticity of its provenance. After the meat have been slaughtered, a unique ID and QR-code is given by the veterinary who assessed the quality of the pig. The truck that transports processed pork meat also has a unique ID and QR code. The wholesaler may scan the ID via QR-code to access the meat provencance and verify this quality. At the market, retailers attach a new QR-code with a color style barcode to the packaging. Finally, the consumer can scan it to know the provenance and the name, address and all the others relevant information of all the actors of the supply chain that have had any interaction with her meat. Thanks to the unique IDs the authority could any moment check the provenance and quality of the pig or the processed meat (TE-Food and LAUREL, 2017).
The system was rolled out in the beginning of 2017, and more than 6000 companies have been trained to use it in South Vietnam. From September 2017 the system has been expended to tracking eggs and chicken, and TE-FOOD reported that 250,000 chicken and 2 million eggs are tracked every day (TE-Food and LAUREL, 2017).
FoodCoin system is an Ethereum-based blockchain system designed to create a global market of food and agricultural products on the platform 1000 EcoFarm. This market is open to all actors of the agri-food supply chain from the producers to the consumers. The system works with a token system, called FoodCoin, to buy and sell goods on the 1000 EcoFarm platform. The system employs blockchain to ensure the validity of the reported transactions has seven technical elements (Foodcoind, 2017): a database implemented as a distributed ledger; its own cryptocurrency, called FoodCoin; a multi-functional crypto-wallet, called the Wallok; its own payment system, called DiPay; a remote user verification; a system for smart contracts, and a product authentication system, called the Product Origin ID.
A producer or farmer can sell their products, and the corresponding transactions are implemented via smart contracts, which are checked at every stage of the supply chain by its actors (farmer, logistic company, customer broker, consumer, bank, and insurance company). A bank can be used as a third party to verify the transactions and convert the coin into fiat currency.
The 1000 Ecofarm is a business to business and B2C online food market for natural food that accepts payments with cryptocurrency, the Food coin. FoodCoin is the main source of exchange between the users of the platform. The number of coins is mathematically limited, and its exchange with fiat currencies is a deflationary model due to its limited market cap.
Wine Blockchain is a project of EzLab that uses blockchain technology to enhance the traceability and the authenticity of wine. The project aims to fight adulteration and forgery of Italian wines via a QR code on the bottle. The QR code allows the final user to verify the authenticity of the products and their certifications, using an app. The data collected at the first mile comes from hardware and software, and they are stored both on a blockchain and the AgriOpenData platform to certify product and automate the supply chain. All the data stored on the blockchain and the AgriOpenData platform are published using a tool that allows producers to format and control access level to their data. The final consumer can access this information via the QR code. Finally, all data flow into a blockchain to ensure immutability, certified by a single AgriOpenData node.
Wine Blockchain wants to build transparency and trust among the producer and the final consumer by showing the provenance of the wine thanks to the scanning of a QR code via a mobile app. Two wineries have implemented this special QR code for their wine bottles. The QR code on the bottle allows the final consumer to learn about the production process of the wine. Every process is registered on the blockchain by a sequential ID. At the first mile a unique ID is given, such as 23456a, then during the process and production the unique ID is extended, for example, during the production the code become 23456a-1, and after it has been bottled, it is 23456a-1/b. The information stored about the product concern its geographical context, the cultivation process, the winemaking process, and distribution and sale (Chase, 2017; EZ Lab, 2017, 2018a,b; Smart AgriFood, 2018).
We summarized the case studies across three dimensions. The first is concerned with the use of blockchain frameworks, the second with the utilization of tokens, and the third with the implementation realization. Table 3 summarizes our findings. Most case studies use Ethereum to run their blockchain implementation; only Provenance used Hyperledger for their Ethereum Virtual Machine. Moreover, it was not clear which blockchain framework TE-FOOD used. Ambrosus, TE-FOOD and FoodCoin employed tokens for their case studies. The difference between them is that TE-FOOD uses the token both as utility and asset. All the companies, except FoodCoin, offer a software implementation. Provenance, Ambrosus, TE-FOOD, and EzLab combine their software with some kind of hardware implementation to enhance the capability of the software implementation to collect data, typically using RFID or QR codes.
We also placed the case studies against the blockchain characteristics of Table 1, and report our classification in Table 4. Three blockchain characteristics were relevant in all case studies, namely Cryptography, Immutability and Redundancy. These three parameters are followed by Shared and Public and Decentralization. No information was available regarding the Peer Verification via the consensus algorithm and psedonymity (Table 4).
By comparing our findings in Sections “Blockchain Applications Potentially Relevant for Agri-Food” and “Blockchain Approaches in the Agri-Food Domain,” we identified commonalities and discrepancies on the blockchain characteristics, illustrated in Figure 3. Note that we had six papers included in the pre-literature review, and investigated six case studies. Immutability, trust and redundancy are the most used characteristics both in academic reports and industrial case studies. All case studies associated blockchain with immutability, cryptography and redundancy. Shared and public and trust are characteristics that all also shared between academic and case study reports. No case study or academic report seemed to employ blockchain for pseudonyms.
Trust in blockchain technology assures the parties involved in a transaction that the agreement condition and their outcomes cannot be changed. If the condition changed or compromised, the transaction would not take place. From a socio-technical system perspective, trust may help to solve issues related to the lack of trust and reputation or unwillingness to share information among trade partners in every industry (Buterin, 2015b; Louise Lemieux, 2015). The trust is also built thanks to other characteristics of the blockchain, such as Shared and Public, Peer Verification, Cryptography, Immutability and Redundancy. Immutability, Cryptography and Redundancy assure that every transaction is immutable. They also assure that the true state of the blockchain and the app or web interface connected are protected by failures, adversarial conditions, or malicious attacks (Mattila, 2016; Baliga, 2017; Cachin and Vukolić, 2017; Dannen, 2017). The Peer Verification guarantees that every change to the chain should be approved via a consensus algorithm (Swanson, 2015; Baliga, 2017; Cachin and Vukolić, 2017). The Shared and Public parameter allows all the writers/user involved in the blockchain to audit it (Szabo, 1997; Buterin, 2015a; Jim Brill et al., 2016; Ølnes, 2016).
Task automation is also significant, especially as it allows to reduce the transaction costs of the among the actors of the agri-food industry. Automation in the blockchain may be implemented via smart contracts, which are an automatic and self-enforcing contract activated on previously agreed condition. This type of automation minimizes the need for trusted intermediaries, such as banks or notary service (Szabo, 1997; Christidis and Devetsikiotis, 2016; Walport, 2016). The automation via smart contracts can help the agri-food industry to facilitate the bank to bank transfer (Swanson, 2015), supply chain tracking (Ruiz-Garcia et al., 2010; Parker, 2016; Provenance, 2016; Lucena et al., 2018), and to reduce paper trail (Palfreyman, 2016a; Zhao, 2017). This benefit of the blockchain is also confirmed by the industry survey of Weldon et al. (2017), where automation of process is the second perceived benefit, with 43%. We also noticed in our case studies that the use of some hardware device or tangible identifier in the form of QR code was often used for improving automation.
Two of blockchain characteristics, namely Trust and Immutability, can be directly related with two open issues of the agri-food supply chain, respectively trust and transparency/visibility of information. Task automation can be considered that contributes to both building trust and improving the visibility of information.
Trust in agri-food supply chains has a historical foundation. Mistrust between the peers, fear of sharing valuable information to competitors is hindering information sharing. Even if information is shared, the truthfulness of the information is sometimes questioned by the receiver (Matopoulos et al., 2007; Lubowe and McDermott, 2016; Calatayud, 2017; Chopra and Meindl, 2018). Susanty et al. (2017) underline that trust is the foundation to create loyalty among peers of the supply chain. Trust may have also significant impact on the performance and success of the supply chain (Kwon and Suh, 2004). Laureano Paiva et al. (2014) argue that a high trust can be beneficial for the sector performance, and this is especially true for the agri-food sector (Tregurtha and Vink, 1999; Hualda et al., 2005; Batt, 2006; Schulze et al., 2006). As mentioned by Prajogo and Olhager (2012); Lubowe and McDermott (2016) and others, the supply chain is a trustless environment where asymmetry of information and partial disclosure of them are the habits. A blockchain system will help the supply chain to be more open and transparent (Tian, 2016).
Transparency or visibility of information in the agri-food supply chain is hard to achieve as many actors retain the information to achieve competitive advantage. Full transparency of information could help to better forecast and improve the performance of the agri-food industry (Yu et al., 2001; Zhou and Benton, 2007). Full disclosure and sharing of information may also help the stakeholders to understand the agri-food supply chain needs and mitigate some of its risks (Doorey, 2011; Mol, 2015). Enhanced visibility of information is the goal of the every supply chain system to achieve stability and minimize risks (Fawcett et al., 2007). Moreover, various studies have shown the advantage of sharing information and information connectivity within the supply chain (Yu et al., 2001; Buhr, 2003; Sanders, 2011).
The objective of this research was to investigate the early application of blockchain technology in the agri-food industry, by following a case study approach focused on the early-adopter companies. From the pre-literature review were found two characteristics that are historical and recurrent in the agri-food industry, trust and transparency. The two major blockchain characteristics identified in the pre-literature study and the case study in the agri-food industry have been identifying as Trust and Automation. These two characteristics mirror the two issues identified in the agri-food supply chain. Both the literature and the case study research identified the importance of trust and transparency, as they may enhance and facilitate the collaboration and lower the risk among the supply chain stakeholders.
In general, the implementation of a technological system should be useful in the way to communicate information to all the stakeholder in the agri-food supply chain. Blockchain technology due to its technical and governance characteristics seems suitable system to this end, as shown by the case studies analyzed. Implementing a system that can enhance both trust and transparency could be highly beneficial and, if the entire supply chain is covered, could lead to more benefits at a chain level.
Developing and implementing such system is not easy for a small food company, as discussed in the case of OriginTrail and EzLab. In both the case of small dairy farmers and winemakers, the existing implemented paper-based system seems to be already enough and there is no need to introduce a sophisticated system with advanced sensors and blockchain. Moreover, small farmers lack the resources to support the implementation of such systems. In the contrary, initiatives led by governments, bigger corporations, or international organizations, as in the cases of tuna tracking (commissioned by IPNLF) or pork meat traceability (supported by the Ho Chi Minh City) seem to be more sustainable. Thus, bigger partnerships that involve more players across the chain seem to be required for blockchain developments.
At the same time, blockchain technology is often considered not to be mature, as it still lacks consistent technical terminology, proven scalability, interoperability, secure privacy and data governance. Thus, solving issues of trust and transparency should not be only considered technical challenges, but also to take into account the broader socio-technical context, including legislation and rules, food security and safety, the economic and social aspect, and the possible technological threats. Also, performance should extend and enhance the already solved problem by existing/current systems.
Interesting directions for future research include studies about possible integration between the existing system in the agri-food system, multiple blockchain application and their integration, acceptance by the stakeholders, standardization of terminology and vocabulary, usage over time, performance, and governance of blockchain technology in the agri-food industry.
This study was able to elicit new knowledge to accelerate the digital modernization of the agri-food industry, due to the information gathered by the case studies and literature. The study framework gathered possible parameters to evaluate the status of blockchain technology application in the agri-food industry. The parameters chosen are general enabling the study to replace other components to better suit the application of the framework in a different context.
Nevertheless, there certain limitations when analyzing the literature and the case study. As mentioned in the pre-literature review, there are very few academic studies that focus on blockchain implementation in the agri-food industry. Most sources are non-academic, some of which are in a continuous update or partially complete. The same holds also for the case studies that we analyzed based on the information collected from white papers and corporate websites. This limits the applicability of the research, as it depends on the focus of the study considers. There might be many other relevant studies on the topic but where not included as still not published or considered not relevant. However, the study has pointed out the issue of the agri-food industry as reflected by the case studies and literature that address it. Also, our findings depend on publicly available information sources, that are not always up-to-date, complete or reflect that actual status of development. For example, the open code repositories of some of the case studies were rather thin, but this cannot be considered an indication of the project quality.
Another limitation is related to the applications focus. This work is limited to what we identified as a first wave of applications, which certainly is not representative of the potential applications. We anticipate that blockchain technology may have a very broad impact on agri-food domain, as for example with applications in agri-food trade and transaction and agri-food insurance.
GM, IA, and BT contributed to conception and design of the study, contributed to manuscript revision, read and approved the submitted version. GM performed the pre-literature review and analyzed the case studies and wrote the first draft of the manuscript. IA and BT wrote sections of the manuscript.
The authors declare that the research was conducted in the absence of any commercial or financial relationships that could be construed as a potential conflict of interest.
IA was partially supported by the Wageningen University & Research Strategic Investment Theme programme Resilience.
Ambrosus (2017a). Food Sensors and Tracers. Available at: https://ambrosus.com/wp-content/uploads/2017/07/4-2_Food_Sensors_and_TracersNEW.pdf (accessed March 3, 2018).
Ambrosus (2017b). Olive Oil Assuring the Quality and Authenticity of the Liquid Gold of the Mediterranean. Available at: https://ambrosus.com/wp-content/uploads/2017/08/Ambrosus-Olive-A4-v4.pdf (accessed March 3, 2018).
Ambrosus (2017c). Technical Data Integrity and Transmission. Available at: https://ambrosus.com/wp-content/uploads/2017/07/4-3.-Technical-Data-Integrity-and-TransmissionNEWupd.pdf (accessed March 3, 2018).
Auchan Retail (2018). Food Traceability: After Successful Tests in Vietnam, Auchan Retail is Launching Blockchain Technology Internationally [Press release]. Available at: https://www.auchan-retail.com/wp-content/uploads/2019/02/28112018_tracabilite_alimentaire_auchan_retail.pdf (accessed March 3, 2018).
Aung, M. M., and Chang, Y. S. (2014). Traceability in a food supply chain: safety and quality perspectives. Food Control 39, 172–184. doi: 10.1016/j.foodcont.2013.11.007
Baliga, A. (2017). Understanding Blockchain Consensus Models, White Paper. Available at: https://www.persistent.com/wp-content/uploads/2017/04/WP-Understanding-Blockchain-Consensus-Models.pdf (accessed March 3, 2018).
Batt, P. J. (2006). Exploring long-term relationships in vegetable supply chains. Postharvest Rev. 2, 1–11. doi: 10.2212/spr.2006.3.9
Beulens, A. J. M., Broens, D. F., Folstar, P., and Hofstede, G. J. (2005). Food safety and transparency in food chains and networks - relationships and challenges. Food Control 16, 481–486. doi: 10.1016/j.foodcont.2003.10.010
Boucher, P., Nascimento, S., and Kritikos, M. (2017). How Blockchain Technology Could Change Our Lives, Science and Technology Options Assessment. Brussels: European Parliamentary Search Service.
Brivio, E., and Malinowska, K. (2017). Towards a Fairer Food Supply Chain European Commission Asks for Input [Press release]. Available at: http://europa.eu/rapid/press-release_IP-17-2521_en.pdf (accessed March 3, 2018).
Brown, R. G. (2015). A Simple Model for Smart Contracts. Available at: https://gendal.me/2015/02/10/a-simple-model-for-smart-contracts/ (accessed March 3, 2018).
Buhr, B. L. (2003). Traceability and information technology in the meat supply chain: implications for firm organization and market structure. J. Food Distrib. Res. 34, 13–26. doi: 10.22004/ag.econ.27057
Buterin, V. (2015a). On Public and Private Blockchains. Available at: https://blog.ethereum.org/2015/08/07/on-public-and-private-blockchains/ (accessed March 3, 2018).
Buterin, V. (2015b). Visions - Part 2: The Problem of Trust. Available at: https://blog.ethereum.org/2015/04/27/visions-part-2-the-problem-of-trust/ (accessed March 3, 2018).
Cachin, C., and Vukolić, M. (2017). Blockchains consensus protocols in the wild. arXiv [Preprint]. arXiv:1707.01873.
Calatayud, A. (2017). The Connected Supply Chain: Enhancing Risk Management in a Changing World. Available at: https://publications.iadb.org/publications/english/document/The-Connected-Supply-Chain-Enhancing-Risk-Management-in-a-Changing-World.pdf (accessed March 3, 2018).
Carrefour Italia (2018). Behind the Scenes of the First European Food Blockchain (in Italian). Available at: https://actforfood.carrefour.it/perche-agire/blockchain (accessed March 3, 2018).
Chase, K. (2017). Ey and ezlab Partner to Create wine Blockchain. Available at: https://dcebrief.com/ey-and-ezlab-partner-to-create-wine-blockchain (accessed April 21, 2017).
Chopra, S., and Meindl, P. (2018). Supply Chain Management: Strategy, Planning & Operation. London: Pearson.
Christidis, K., and Devetsikiotis, M. (2016). Blockchains and smart contracts for the internet of things. IEEE Access 4, 2292–2303. doi: 10.1109/Access.2016.2566339
Coleman, L. (2017). European Commission to Establish eu Blockchain Observatory. Available at: https://www.cryptocoinsnews.com/european-commission-to-establish-eu-blockchain-observatory/ (accessed March 3, 2018).
Croman, K., Decker, C., Eyal, I., Gencer, A. E., Juels, A., Kosba, A., et al. (2016). On scaling decentralized blockchains. Lect. Notes Comput. Sci. 9604, 106–125. doi: 10.1007/978-3-662-53357-4_8
Crouch, M. (2017). A Brief Introduction to Blockchain for Normal People. In: Medium [Internet]. Medium. Available at: https://medium.com/@marccrouch/a-brief-introduction-to-blockchain-for-normal-people-84a4717e473c (accessed February 13, 2020).
Dannen, C. (2017). Introducing Ethereum and Solidity: Foundations of Cryptocurrency and Blockchain Programming for Beginners. Berkeley, CA: Apress. doi: 10.1007/978-1-4842-2535-6
Doorey, D. J. (2011). The transparent supply chain: from resistance to implementation at nike and levi-strauss. J. Bus. Ethics 103, 587–603. doi: 10.1007/s10551-011-0882-1
English, S. M., and Nezhadian, E. (2017). Application of bitcoin data-structures & design principles to supply chain management. arXiv [Preprint]. arXiv: 1703.04206.
Ethereum Foundation (2018). Ethereum Homestead Documentation. Available at: https://ethereum-homestead.readthedocs.io/en/latest/ (accessed March 3, 2018).
Eyal, I., Gencer, A. E., Sirer, E. G., and Van Renesse, R. (2016). Bitcoin-ng: a scalable blockchain protocol. Paper Presented at the NSDI, Santa Clara, CA.
EZ Lab (2017). Arriva Wine Blockchain per la Territorialità, Autenticità e Qualità del Prodotto. Available at: https://www.ezlab.it/news/arriva-wine-blockchain-per-la-territorialita-autenticita-e-qualita-del-prodotto/ (accessed March 3, 2018).
EZ Lab (2018a). Nero d’avola la Mura bio si Certifica con Blockchain. Available at: https://www.ezlab.it/news/nero-davola-la-mura-bio-si-certifica-con-blockchain/ (accessed March 3, 2018).
EZ Lab (2018b). Placido Volpone: La Prima Azienda Vitivinicola Certificata Blockchain. Available at: https://www.ezlab.it/news/placido-volpone-la-prima-azienda-vitivinicola-certificata-blockchain/ (accessed March 3, 2018).
Fawcett, S. E., Osterhaus, P., Magnan, G. M., Brau, J. C., and McCarter, M. W. (2007). Information sharing and supply chain performance: The role of connectivity and willingness. Supply Chain Manag. 12, 358–368. doi: 10.1108/13598540710776935
Foodcoind (2017). Foodcoin Ecosystem. Available at: https://www.foodcoin.io/files/foodcoin-whitepaper-tmp-2017-09-10.pdf (accessed March 3, 2018).
Ge, L., Brewster, C., Spek, J., Smeenk, A., and Top, J. (2017). Blockchain for Agriculture and Food; Findings from the Pilot Study. Wageningen: Wageningen Economic Research.
Guo, Y., and Liang, C. (2016). Blockchain application and outlook in the banking industry. Financ. Innov. 2:24. doi: 10.1186/s40854-016-0034-9
Hackius, N., and Petersen, M. (2017). Blockchain in logistics and supply chain: Trick or treat?” Paper Presented at the Hamburg International Conference of Logistic, Hamburg.
Haswell, H., and Storgaard, M. C. (2017). Maersk and ibm Unveil First Industry-Wide Cross-Border Supply Chain Solution on Blockchain [Press release]. Available at: http://www-03.ibm.com/press/us/en/pressrelease/51712.wss (accessed March 3, 2018).
Hualda, L., Migalbin, L., Montiflor, M., Manalili, N., McGregor, M., Murray-Prior, R., et al. (2005). Exploring the antecedents and consequences of trust between vegetable farmers and their preferred trading partners in southern mindanao. Paper Presented at the Ist International Symposium on Improving the Performance of Supply Chains, Hanoi.
Huang, S., and Carlsson, J. (2016). Blockchain Technology in the Swedish Fund Market: A Study on the Trust Relationships between Actors in a Blockchain-Based Fund Market. Stockholm: KTH, School of Computer Science and Communication (CSC).
Idelberger, F., Governatori, G., Riveret, R., and Sartor, G. (2016). Evaluation of logic-based smart contracts for blockchain systems. Paper Presented at the International Symposium on Rules and Rule Markup Languages for the Semantic Web, Berlin, Springer.
Jim Brill, D., Cuomo, J., Gopinath, R., Korsten, P., McDermott, B., McLean, J., et al. (2016). Fast Forward Rethinking Enterprises, Ecosystem and Economies with Blockchains. Available at: https://www.ibm.com/downloads/cas/QP4AE4GN (accessed March 3, 2018).
Kersten, W., Seiter, M., von See, B., Hackius, N., and Maurer, T. (2017). Trends and Strategies in Logistics and Supply Chain Management – Digital Transformation Opportunities. Hamburg: Dvv media group gmbh.
Kim, H. J. (2017). Information technology and firm performance: the role of supply chain integration. Oper. Manage. Res. 10, 1–9. doi: 10.1007/s12063-016-0122-z
Kim, H. M., and Laskowski, M. (2018). Toward an ontology-driven blockchain design for supply chain provenance. Intell. Syst. Account. Finance Manag. 25, 18–27. doi: 10.1002/isaf.1424
Kumar, M. V., and Iyengar, N. C. S. N. (2017). A framework for blockchain technology in rice supply chain management. Adv. Sci. Technol. Lett. 146, 125–130. doi: 10.14257/astl.2017.146.22
Kwon, I. W. G., and Suh, T. (2004). Factors affecting the level of trust and commitment in supply chain relationships. J. Supply Chain Manag. 40, 4–14. doi: 10.1111/j.1745-493x.2004.tb00165.x
Lansiti, M., and Lakhani, K. R. (2017). The truth about blockchain. Harv. Bus. Rev. 95, 119–127. doi: 10.3390/s19153267
Laureano Paiva, E., Teixeira, R., Marques Vieira, L., and Beheregaray Finger, A. (2014). Supply chain planning and trust: two sides of the same coin. Ind. Manage. Data Syst. 114, 405–420. doi: 10.1108/imds-07-2013-0324
Linux Foundation (2018a). Hyperledger Fabric Wiki. Available at: https://hyperledger-fabric.readthedocs.io/en/latest/ (accessed March 3, 2018).
Linux Foundation (2018b). Hyperledger Sawtooth Wiki. Available at: https://sawtooth.hyperledger.org/docs/core/releases/latest/contents.html# (accessed March 3, 2018).
Linux Foundation (2018c). Hyperledger Wiki. Available at: https://wiki.hyperledger.org/ (accessed March 3, 2018).
Louise Lemieux, V. (2015). Trusting records: is Blockchain technology the answer? Rec. Manage. J. 26, 110–139.
Lubowe, D., and McDermott, B. (2016). Trust in Trade - Toward Stronger Supply Chains. Available at: https://www.ibm.com/downloads/cas/JX9AM9MN (accessed March 3, 2018).
Lucena, P., Binotto, A. P., Momo, F. D. S., and Kim, H. (2018). A case study for grain quality assurance tracking based on a Blockchain business network. Paper Presented at the Symposium on Foundations and Applications of Blockchain (FAB 18), Los Angeles, CA.
Maimbo, H., and Pervan, G. (2005). “Designing a case study protocol for application in is research,” in Proceedings of the 9th Pacific Asia Conference on Information System, ed. P. Y. K. Chau (Hong Kong: University of Hong Kong).
Mainelli, M., and Smith, M. (2015). Sharing ledgers for sharing economies: an exploration of mutual distributed ledgers (aka blockchain technology). J. Financ. Perspect. 3, 38–69.
Manyika, J., Ramaswamy, S., Khanna, S., Sarrazin, H., Pinkus, G., Sethupathy, G., et al. (2015). Digital America: A Tale of the Haves and Have-More. New York, NY: McKinsey &Company.
Matopoulos, A., Vlachopoulou, M., Manthou, V., and Manos, B. (2007). A conceptual framework for supply chain collaboration: empirical evidence from the agri-food industry. Supply Chain Manag. 12, 177–186. doi: 10.1108/13598540710742491
Mattila, J. (2016). The Blockchain Phenomenon – The Disruptive Potential of Distributed Consensus Architectures. Report No. 38. Helsinki: The Research Institute of the Finnish Economy. Available at: https://ideas.repec.org/p/rif/wpaper/38.html
Medium (2018). Medium. Available at: https://medium.com/ (accessed March 3, 2018).
Mol, A. P. J. (2015). Transparency and value chain sustainability. J. Cleaner Production 107, 154–161. doi: 10.1016/j.jclepro.2013.11.012
Ølnes, S. (2016). “Beyond bitcoin enabling smart government using Blockchain technology,” in Electronic Government, ed. H. J. Scholl (Cham: Springer), 253–264. doi: 10.1007/978-3-319-44421-5_20
Palfreyman, J. (2016b). Blockchain, Where is My Stuff? Available at: https://www.ibm.com/blogs/insights-on-business/government/blockchain-wheres-my-stuff/ (accessed August 24, 2017).
Parker, L. (2016). Walmart, IBM and Tsinghua University to use a Blockchain for Food Supply Chain Tracking in China. Available at: http://bravenewcoin. com/news/walmart-ibm-and-tsinghua-university-to-use-a-blockchain-for-food-supply-chain-tracking-in-china (accessed April 23, 2017).
Perugini, M. L., and Dal Checco, P. (2015). Smart Contracts: A Preliminary Evaluation. Available at: https://ssrn.com/abstract=2729548 (accessed Decenber 8, 2015).
Prajogo, D., and Olhager, J. (2012). Supply chain integration and performance: The effects of long-term relationships, information technology and sharing, and logistics integration. Int. J. Prod. Econ. 135, 514–522. doi: 10.1016/j.ijpe.2011.09.001
Provenance (2015). Blockchain: the Solution for Transparency in Product Supply Chains (White Paper). Available at: https://www.provenance.org/whitepaper (accessed March 3, 2018).
Provenance (2016). From Shore to Plate: Tracking Tuna on the Blockchain (Technical Report). Available at: https://www.provenance.org/tracking-tuna-on-the-blockchain#overview (accessed March 3, 2018).
Rakic, B., Levak, T., Drev, Z., Savic, S., and Veljkovic, A. (2017). Origintrail White Paper - First Purpose Built Protocol for Supply Chains Based on Blockchain. Available at: https://origintrail.io/storage/documents/OriginTrail-White-Paper.pdf (accessed March 3, 2018).
Rowley, J., and Slack, F. (2004). Conducting a literature review. Manage. Res. News 27, 31–39. doi: 10.1108/01409170410784185
Ruiz-Garcia, L., Steinberger, G., and Rothmund, M. (2010). A model and prototype implementation for tracking and tracing agricultural batch products along the food chain. Food Control 21, 112–121. doi: 10.1016/j.foodcont.2008.12.003
Sanders, N. R. (2011). The impact of buyer firm information connectivity enablers on supplier firm performance: a relational view. Int. J. Logist. Manag. 22, 179–201. doi: 10.1108/09574091111156541
Schulze, B., Wocken, C., and Spiller, A. (2006). Relationship quality in agri-food chains: supplier management in the German pork and dairy sector. J. Chain Netw. Sci. 6, 55–68. doi: 10.3920/JCNS2006.x065
Seebacher, S., and Schüritz, R. (2017). “Blockchain technology as an enabler of service systems: a structured literature review,” in Proceedings of the International Conference on Exploring Services Science (Berlin: Springer), 12–23. doi: 10.1007/978-3-319-56925-3_2
Shakir, M. (2002). The selection of case studies: strategies and their applications to is implementation case studies. Res. Lett. Inf. Math. Sci. 3, 191–198.
Smart AgriFood (2018). Blockchain: Traceability, Transparency and Certification - the Agricultural Safety from Farmers to Consumers. Available at: http://www.smartagrifood.it/index-en.html (accessed March 3, 2018).
Susanty, A., Bakhtiar, A., Jie, F., and Muthi, M. (2017). The empirical model of trust, loyalty, and business performance of the dairy milk supply chain a comparative study. Br. Food J. 119, 2765–2787. doi: 10.1108/Bfj-10-2016-0462
Swan, M. (2016). “Blockchain temporality: smart contract time specifiability with blocktime,” in Rule Technologies. Research, Tools, and Applications, eds J. J. Alferes, L. Bertossi, G. Governatori, P. Fodor, and D. Roman (Cham: Springer), 184–196. doi: 10.1007/978-3-319-42019-6_12
Swanson, T. (2015). Consensus-as-a-Service: A Brief Report on the Emergence of Permissioned, Distributed Ledger Systems. Available at: http://www.ofnumbers.com/wp-content/uploads/2015/04/Permissioned-distributed-ledgers.pdf (accessed March 3, 2018).
Szabo, N. (1997). Formalizing and securing relationships on public networks. First Monday 2. doi: 10.5210/fm.v2i9.548
TE-Food (2017). Making Business Profit by Solving Social Problems, White Paper. Available at: https://www.te-food.com/te-food-white-paper.pdf (accessed March 3, 2018).
TE-Food and LAUREL (2017). The Largest Farm-to-Table Food Traceability Project in the World. Available at: http://te-food.co.za/use_case.html (accessed March 3, 2018).
Tian, F. (2016). “An agri-food supply chain traceability system for china based on rfid & blockchain technology,” in Proceedings of the 13th International Conference on Service Systems and Service Management (Icsssm) (Kunming: IEEE), 1–6.
Tregurtha, N., and Vink, N. (1999). Trust and supply chain relationships: a South African case study. Agrekon 38, 755–765. doi: 10.1080/03031853.1999.9524886
Trienekens, J. H., Wognum, P. M., Beulens, A. J. M., and van der Vorst, J. G. A. J. (2012). Transparency in complex dynamic food supply chains. Adv. Eng. Inform. 26, 55–65. doi: 10.1016/j.aei.2011.07.007
Tschorsch, F., and Scheuermann, B. (2016). Bitcoin and beyond: a technical survey on decentralized digital currencies. IEEE Commun. Surv. Tutor. 18, 2084–2123. doi: 10.1109/Comst.2016.2535718
Versetti, A., and Meyer, S. (2017). Food Supply Chain 2.0 - Ambrosus Vision Paper. Available at: https://ambrosus.com/wp-content/uploads/2017/07/Ambrosus-Vision-Paper-V.2.3.2-EN.pdf (accessed March 3, 2018).
Walport, M. (2016). Distributed Ledger Technology: Beyond Block Chain. London: Government Office for Science.
Weldon, R., Herridge, M., and Cohen, J. (2017). Retail: Opening the Doors to Blockchain (White Paper). Available at: https://www.cognizant.com/whitepapers/retail-opening-the-doors-to-blockchain-codex2879.pdf (accessed March 3, 2018).
Wright, A., and De Filippi, P. (2015). Decentralized Blockchain Technology and the Rise of Lex Cryptographia. Available at: https://ssrn.com/abstract=2580664 (accessed March 3, 2018).
Yang, M., and Yang, Y. Y. (2014). Applying network coding to peer-to-peer file sharing. IEEE Transact. Comput. 63, 1938–1950. doi: 10.1109/Tc.2013.88
Yu, Z. X., Yan, H., and Cheng, T. C. E. (2001). Benefits of information sharing with supply chain partnerships. Ind. Manage. Data Syst. 101, 114–119. doi: 10.1108/02635570110386625
Zhao, W. (2017). IBM Reveals Blockchain Supply Chain Trial with Singapore Port Operator. Available at: https://www.coindesk.com/ibm-reveals-blockchain-supply-chain-trial-singapore-port-operator/ (accessed August 18, 2017).
Keywords: blockchain, agri-food, case study, transparency, trust
Citation: Motta GA, Tekinerdogan B and Athanasiadis IN (2020) Blockchain Applications in the Agri-Food Domain: The First Wave. Front. Blockchain 3:6. doi: 10.3389/fbloc.2020.00006
Received: 29 April 2019; Accepted: 04 February 2020;
Published: 21 February 2020.
Edited by:
Hang Xiong, Huazhong Agricultural University, ChinaReviewed by:
Puqing Wang, Wuhan Polytechnic University, ChinaCopyright © 2020 Motta, Tekinerdogan and Athanasiadis. This is an open-access article distributed under the terms of the Creative Commons Attribution License (CC BY). The use, distribution or reproduction in other forums is permitted, provided the original author(s) and the copyright owner(s) are credited and that the original publication in this journal is cited, in accordance with accepted academic practice. No use, distribution or reproduction is permitted which does not comply with these terms.
*Correspondence: Ioannis N. Athanasiadis, aW9hbm5pcy5hdGhhbmFzaWFkaXNAd3VyLm5s
Disclaimer: All claims expressed in this article are solely those of the authors and do not necessarily represent those of their affiliated organizations, or those of the publisher, the editors and the reviewers. Any product that may be evaluated in this article or claim that may be made by its manufacturer is not guaranteed or endorsed by the publisher.
Research integrity at Frontiers
Learn more about the work of our research integrity team to safeguard the quality of each article we publish.