- 1Department of Prosthodontics, School and Hospital of Stomatology, Wenzhou Medical University, Wenzhou, China
- 2Department of Oral Implantology and Prosthetic Dentistry, Academic Centre for Dentistry Amsterdam, MOVE Research Institute, University of Amsterdam and Vrije University Amsterdam, Amsterdam, Netherlands
- 3Institute of Stomatology, School and Hospital of Stomatology, Wenzhou Medical University, Wenzhou, China
- 4Department of Oral and Maxillofacial Surgary/Pathology, Amsterdam UMC and Academic Centre for Dentistry Amsterdam, Amsterdam Movement Science, Vrije Universitetit Amsterdam, Amsterdam, Netherlands
- 5Laboratory for Myology, Amsterdam Movement Sciences, Faculty of Behavioral and Movement Sciences, Vrije Universiteit Amsterdam, Amsterdam, Netherlands
- 6Department of Stomatology, Taizhou Hospital, Wenzhou Medical University, Linhai, China
- 7Department of Periodontology, School and Hospital of Stomatology, Wenzhou Medical University, Wenzhou, China
- 8Department of Endodontics, School and Hospital of Stomatology, Wenzhou Medical University, Wenzhou, China
Osteoblasts dysfunction, induced by oxidative stress (OS), is one of major pathological mechanisms for osteoporosis. Curcumin (Cur), a bioactive antioxidant compound, isolated from Curcumin longa L, was regarded as a strong reactive oxygen species (ROS) scavenger. However, it remains unveiled whether Cur can prevent osteoblasts from OS-induced dysfunction. To approach this question, we adopted a well-established OS model to investigate the preventive effect of Cur on osteoblasts dysfunction by measuring intracellular ROS production, cell viability, apoptosis rate and osteoblastogenesis markers. We showed that the pretreatment of Cur could significantly antagonize OS so as to suppress endogenous ROS production, maintain osteoblasts viability and promote osteoblastogenesis. Inhibiting Glycogen synthase kinase (GSK3β) and activating nuclear factor erythroid 2 related factor 2 (Nrf2) could significantly antagonize the destructive effects of OS, which indicated the critical role of GSK3β-Nrf2 signaling. Furthermore, Cur also abolished the suppressive effects of OS on GSK3β-Nrf2 signaling pathway. Our findings demonstrated that Cur could protect osteoblasts against OS-induced dysfunction via GSK3β-Nrf2 signaling and provide a promising way for osteoporosis treatment.
Introduction
Osteoporosis, a systemic degenerative disease during aging, is associated with increased fragility and fracture risk of bone. It is characterized by decrease of bone mass and deterioration of bone architectural structure due to the imbalance between bone formation and resorption (Fonseca et al., 2014). Roughly nine million osteoporotic or fragility (low-trauma) fractures occur worldwide per year (Johnell and Kanis, 2006). In developed countries, around one in three women and one in five men aged 50 years or older will have a fragility fracture during their remaining lifetime, thereby significantly affecting their life quality and expectancy (Shepstone et al., 2018). However, hitherto, the mechanisms for osteoporosis are not completely unveiled and there is still a lack of efficacious treatment strategies helping bone tissue regeneration under this circumstance.
A great line of evidences reveal that oxidative stress (OS) is a crucial pathogenic factor for osteoporosis (Manolagas, 2010; Molnar et al., 2016). Our previous meta-analysis study demonstrates that the decrease of bone mineral density (BMD) in osteoporotic postmenopausal women is closely related to the status of OS (Zhou et al., 2016). In animal models, significantly decreased endogenous antioxidant defense and increased oxidative damages are detected in osteoporotic bone tissue induced by ovariectomy (Mohd Effendy et al., 2015; Serizawa et al., 2016). Furthermore, approaches to attenuate OS have been found to effectively delay or inhibit the progress of osteoporosis (Li et al., 2017; Zhu et al., 2018). All these findings indicate the paramount importance of OS in the pathogenic process of osteoporosis.
Osteoblasts play a crucial role in bone maintenance and regeneration. Its cellular interactions with osteoclasts are essential to regulate bone mass homeostasis as well as bone quality (Nevius et al., 2015; Maria et al., 2018). During osteoblastogenesis, mesenchymal stem cells are first osteogenically committed and become preosteoblasts. Thereafter preosteoblasts undergo the process of osteoblastogenesis during which the alkaline phosphatase (ALP) activity (a marker for an early osteoblastogenic differentiation) and osteocalcin (OCN) expression (a marker for a late osteoblastogenic differentiation) in cells will be significantly enhanced, finally leading to cell matrix mineralization. During this process, the expression of osteoblastogenic genes, such as Runt-related transcription factor 2 (Runx2), Collagen Iα (Col I), will also be significantly enhanced. It has been established that the reduction of osteoblasts viability and function during aging leads to the imbalance of bone formation and bone resorption, which is significantly associated with the onset and progression of osteoporosis (Farr et al., 2017; Xu et al., 2017). Persistent or prolonged OS can not only inhibit proliferation (Li et al., 2009) and differentiation (Zhong et al., 2009) of osteoblasts but also induce their apoptosis (Almeida et al., 2010; Wu et al., 2011; Mao et al., 2018), which results in bone loss during osteoporosis. Therefore, bioactive agents to attenuate OS damages to osteoblasts may be promising in treating osteoporosis (Hendrickx et al., 2015; Mao et al., 2018).
One of such bioactive agents is Curcumin (Cur), the major active ingredient of turmeric plant (Curcumin longa L). Cur has been long recognized as an anti-inflammatory and anti-bacterial agent (Yodkeeree et al., 2009; Feng et al., 2019) to treat various chronic inflammatory diseases (Goel et al., 2008). Moreover, emerging evidences reveal that Cur can protect liver and kidney from drugs- or toxins-induced acute or chronic injury by scavenging reactive oxygen species (ROS) and improving anti-oxidative ability (Sahin et al., 2012; Tokac et al., 2013; Wu et al., 2017). Further report demonstrates that Cur improves bone microarchitecture and enhances mineral density in amyloid precursor protein (APP)/presenilin (PS1) transgenic mice (Yang et al., 2011), a transgenic mouse representing the pathological changes of senile dementia and osteoporosis. On the one hand, such an effect of Cur is attributed to its capacity of suppressing osteoclastogenesis by inhibiting receptor activator of nuclear factor-κB ligand (RANKL) signaling, an essential signaling for the differentiation of bone-resorbing osteoclasts (Folwarczna et al., 2010; Kim et al., 2011; Hussan et al., 2012; Cho et al., 2013). On the other hand, in our previous study, we also showed that Cur attenuated OS-induced apoptosis of human osteoblastic cell (Saos-2) by preserving the mitochondrial functions and upregulating phosphorylated protein kinase B (Akt)-Glycogen synthase kinase 3β (GSK3β) signaling (Chao et al., 2017). However, hitherto, it remains unknown whether Cur can antagonize oxidative damages so as to maintain the osteogenic function of osteoblasts under the OS attack.
Glycogen synthase kinase 3β is a serine/threonine kinase that is involved in cell apoptosis, inflammatory reactions as well as OS related diseases (Qi et al., 2015). GSK3β can significantly down-regulate the expression of nuclear factor (erythroid-derived 2)-like 2 (Nrf2), a key transcription factor accounting for cell antioxidant defense (Zhuang et al., 2019). Nrf2 has been suggested to be a novel target to slow down the progression of bone degenerative disorders (Jiang et al., 2018; Olagnier et al., 2018). However, whether GSK3β-Nrf2 signaling pathway is involved in regulating OS-induced osteoblasts dysfunction remains unknown.
In this study, we adopted a well-established cell oxidative injury model aiming (1) to investigate the effects of Cur on OS-induced osteoblasts dysfunction and (2) to verify whether GSK3β-Nrf2 signaling pathway mediated the protective effect of Cur.
Materials and Methods
Experiment Design
We treated a mouse preosteoblast cell line (MC3T3-E1 cells) with hydrogen peroxide (H2O2) to establish an oxidative injury model. Cur or N-acetyl-L-cysteine (NAC) was preincubated as antioxidant. The intracellular ROS production, cell viability, apoptosis rate and osteoblastogenesis markers were measured and compared among different groups. After detecting the level of phosphorylated-GSK3β and Nrf2, the specific chemical GSK3β inhibitor 2-Methyl-4-(phenylmethyl)-1,2,4-thiadiazolidine-3,5-dione (TDZD-8) and Nrf2 activator tertiary butylhydroquinone (tBHQ) were added to the model to further confirm the role of this signaling pathway in OS suppressing, cell viability protecting and osteogenesis promoting.
Cell Culture
MC3T3-E1 cells (obtained from American Type Culture Collection, ATCC) were cultured in α-minimum essential medium (α-MEM) supplymented with 10% fetal bovine serum (FBS) and 100 U/mL penicillin, and 100 U/mL streptomycin. This basic medium was replaced every 3 days. For osteogenetic differentiation induction, when, β-glycerophosphate (5 mM, Sigma, United States) and ascorbic acid (100 mg/mL, Sigma, United States) were added to basic culture medium after cells at 80% confluence, and differentiation medium was changed every 3 days.
Cell Treatment
The different treating condition of the compounds were as follows: H2O2 (0.1–1 mM, Sigma, United States) for 6 h, Cur (0.25 μM, Sigma, United States) for 24 h, TDZD-8 (5 μM, Sigma, United States) for 1 h, tBHQ (5 μM, Sigma, United States) for 1 h, NAC, the widely used antioxidant for positive control (2.5 mM, Sigma, United States) for 1 h, according to previous studies (Dai et al., 2017; Cuadrado et al., 2018; Huang et al., 2019) and our preliminary data (data not shown). Cells were treated with or without H2O2 and the indicated compounds for various conditions in basic medium or osteogenesis differentiation medium. The final concentration of dimethyl sulfoxide (DMSO, Sigma, United States) was diluted to less than 0.5% in all experiments.
Cell Viability Assay
Apoptosis of MC3T3-E1 cells were detected by 3(4,5-dimethylthiazol-2-yl)-2,5-diphenyltetrazolium (MTT, Sigma, United States) bromide method. MC3T3-E1 cells (1 × 104 cells/well) were plated on 96-well plates and treated as indicated above. Cell viability at 24, 48, and 96 h was measured as previously described (Dai et al., 2017).
Measurement of Apoptosis by Flow Cytometry and Deoxynucleotidyl Transferase dUTP Nick End Labeling Assays
Apoptosis of MC3T3-E1 cells were detected by Annexin V-fluorescein isothiocyanate (FITC, 5 μl, Thermo Fisher Scientific, United States); propidium iodide (PI; 10 μl, Thermo Fisher Scientific, United States) was used to determine cell necrosis. After treatment, cells were harvested and resuspended influorochromes at 37°C, then incubated in the dark for 15 min. Cytofluorometric analysis was using a FACScan (Becton Dickinson, NY, USA).
Apoptosis was also evaluated by the terminal transferase dUTP nick end labeling (TUNEL) staining (Roche, Switzerland), following the manufacturer’s instructions. Cells were incubated on different coverslips. After treatment, the cells were fixed in 4% paraformaldehyde (PFA), gently washed with PBS and mixed with 0.2% Triton X-100. Samples were incubated with newly prepared TUNEL assay solution 1h, then counterstained with 4′, 6-diamidino-2-phenylindole (DAPI, Sigma, United States) 5 min in the dark. Cells were observed by fluorescence microscope (Leica TCS SPE, Germany), and the nucleus fluoresced brightly green were considered as TUNEL positive cell. Percentages of TUNEL positive cells were calculated by counting 300 cells in random fields.
ROS Assay
2′,7′-Dichlorofluorescin Diacetate (DCFH-DA, Thermo Fisher Scientific, United States) were used to assess ROS generation. MC3T3-E1 cells (1 × 104 cells/well) were seeded in chamber slides. Cells were treated with or without H2O2 (0.75 mM, 6 h), pre-incubating with Cur for 24 h or the positive control NAC for 1 h. After treatments, MC3T3-E1 cells were incubated with 10 μM DCFH-DA for 30 min at 37°C and fixed in 4% PFA for 30 min at room temperature. After being washed with PBS, the fixed cells were stained with 20 μg/mL DAPI in the dark for 5 min at room temperature. Then the fluorescence of cells was detected by a fluorescence microscope and quantified by NIH Image J software (public domain).
ALP Activity Assay and ALP Staining
MC3T3-E1 cells (3 × 104 cells/well) were seeded on 48-well plates and stimulated with osteogenesis differentiation medium for 7 days. After treatment, ALP activity of the cell lysate was assayed by an ALP assay kit (Beyotime, China). In brief, 50 μL of sample was incubated for 10 min in a 96-well plate with 50 μL newly prepared work solution in 37°C. After stop of the reaction with 100 μL stop solution, the absorbance was measured at 405 nm with a micro-plate reader. Protein concentration was determined using BCA protein assay (Thermo Fisher Scientific, United States).
Alkaline phosphatase staining was performed by a standard protocol. Briefly, samples were fixed with 4% PFA for 30 min at 4°C and stained by a BCIP/NBT ALP color development kit (Beyotime, China) according to the manufacture’s instruction. After staining, cells were washed with deionized water three times.
Mineralization Assay
MC3T3-E1 cells (3 × 104 cells/well) were seeded on 48-well plates and were stimulated with osteogenic differentiation medium for 14 days. After osteogenic differentiation, the cells were gently washed twice with PBS and fixed with 4% PFA for 30 min at 4°C. Then, cells were stained with 0.1% Alizarin red (Sigma, United States) for 1 h at room temperature. Excess dye was then washed away with deionized water and each well was photographed and quantified by ImageJ.
Quantitative Real-Time Polymerase Chain Reaction (rt-PCR)
Total RNA from MC3T3-E1 cells was extracted using Trizol reagent (Invitrogen, United States). cDNA synthesis was performed with 1 mg RNA using PrimeScript RT reagent Kit with gDNA Eraser (Takara, Japan) and quantified by measuring the absorbance at 260 and 280 nm. Samples were analyzed in triplicate. cDNA was amplified using following gene-specific primers listed in Table 1. PCR was then carried out for 30 cycles consisting 1min each for 94°C (denaturation), 60°C (annealing), and 72°C (elongation), and final extension was done at 72°C for 10 min using TB Green Premix Ex Taq (Takara, Japan) according to the manufacture’s instruction.
Protein Extraction and Western Blot Analysis
After the indicated treatment, MC3T3-E1 cells were harvested and proteins from them were extracted using radioimmunoprecipitation assay buffer (RIPA) buffer (Sigma, United States). Equal amounts of protein were separated by SDS-PAGE and transferred into a polyvinylidene difluoride membrane. Concentration of primary antibodies are as follows: anti-phospho-GSK3β (1:4000, Cell Signaling, United States), anti-GSK3β (1:4000, Cell Signaling, United States), anti-Nrf2 (1:1000, Santa, United States), and anti-β-actin (1:8000, Sigma, United States). The following secondary antibody were horseradish peroxidase conjugated anti-mouse IgG antibody (1:4000, Invitrogen, United States) or anti-rabbit IgG antibody (1:4000, Invitrogen, United States), followed by the incubation of enhanced chemiluminescence (ECL) substrate. The immunoreactive bands intensities were quantified using ImageJ software and normalized with β-actin levels.
Statistical Analysis
Data are described as mean ± SD (the standard deviation of the mean). All statistical analysis was performed with Statview software (SAS Institute, Version 5.0.1). Differences between groups were assessed by one-way analysis of variance (ANOVA) with Fisher’s post hoc test. Significant difference was accepted at P < 0.05.
Results
Cur Attenuated H2O2-Induced Apoptosis and ROS Generation in MC3T3-E1 Cells
In order to investigate the antioxidant effect of Cur, MC3T3-E1 cells were exposed to H2O2 at different concentration with different enduring time according to our previous study (Dai et al., 2017). Compared with the vehicle group, H2O2 significantly decreased osteoblasts viability in the dose and time dependent way (Figure 1A). Flow cytometric analysis also showed a dose-dependent increasing of apoptosis. Early apoptosis was detected after the administration of 0.5 mM H2O2, and higher concentration of H2O2 induced late apoptosis with a slight increase of necrosis (Figures 1B,C). Treated with H2O2 at a concentration of 0.75 mM with 6 h was the half inhibitory concentration (IC) of MC3T3-E1 cells and can cause cell apoptosis to a certain degree. Therefore, this condition was chosen in the following experiments. Our results showed that Cur ranging from 0.01 to 1.0 μM was not cytotoxic to MC3T3-E1 cells. It reversed cell viability reduced by H2O2 and played its best role under the condition of 0.25 μM, pretreating 24 h (Figure 1D). Furthermore, the result of TUNEL staining (Figures 1E,G) indicated the decreased percentage of apoptotic cells by using Cur. In Figures F,H, we showed that the ROS level increased by H2O2 were attenuated by Cur. The effect of Cur was the similar to classical antioxidant NAC, which indicated that Cur had positive effect against OS.
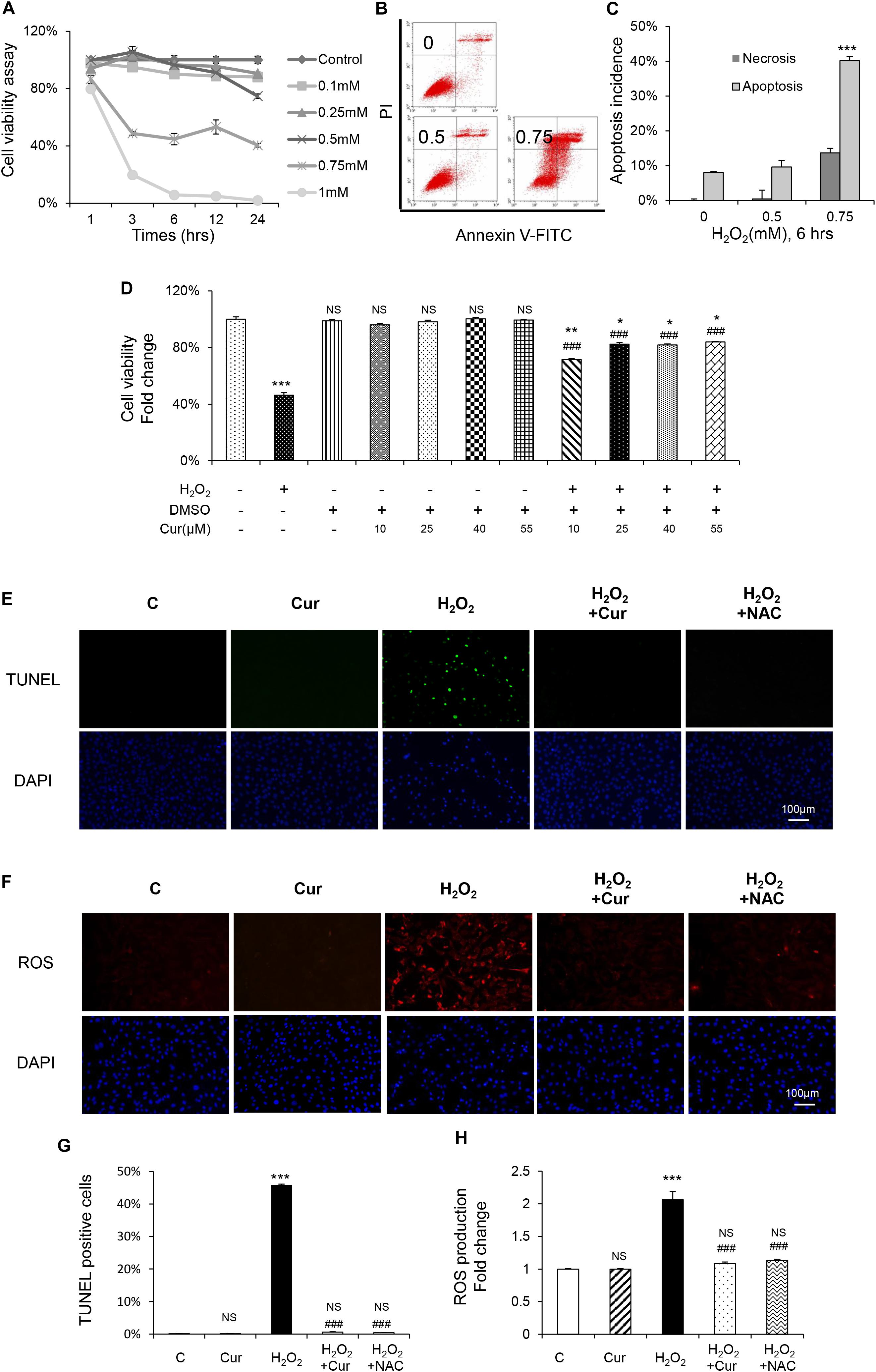
Figure 1. Cur attenuated H2O2-induced apoptosis and ROS generation in MC3T3-E1 cells. (A) MC3T3-E1 cells were treated with or without H2O2 in the basic medium. Cell viability was determined by MTT reduction in MC3T3-E1 cells in the presence of different concentration of H2O2 for 1, 3, 6, 12, 24 h. (B,C) The flow cytometric analysis of staining from control group, 0.5 and 0.75 mM H2O2 for 6 h. (D) Cur was added 24 h before H2O2. Cell viability was determined by MTT reduction in MC3T3-E1 cells in the presence of 0.10, 0.25, 0.40, and 0.55 μM Cur for 24 h with (+) or without (–) H2O2. (E,G) The cells were immunostained for TUNEL (green). DAPI staining was used to mark the position of the nuclei. Scale bars = 100 μm. (F,H) The cells were harvested and stained with DCFH-DA (red). Scale bars = 100 μm. Data are presented as the mean ± SD from at least three independent experiments. *p < 0.05, **p < 0.01, ***p < 0.001, versus C group; NS, non-significantly different from C group. #p < 0.05, ##p < 0.01, ###p < 0.001, versus the H2O2 group; ns, non-significantly different from H2O2 group.
Cur Rescue H2O2-Induced Osteoblasts Dysfunction
MC3T3-E1 cells were supplemented with differentiation medium to initiate osteogenic induction. ALP, as the by-product of osteoblasts activity, was decreased by H2O2 and recovered by Cur during the differentiation process showed by the results of both ALP staining and activity (Figures 2A,C). The cell capability of differentiation and mineralization examined by Alizarin red staning (ARS) reduced by H2O2 was also attenuated by Cur (Figures 2B,D). Further, mRNA test showed the decreased expression of typical osteogenic marker genes (ALP, OCN, COL I, and Runx2) in OS injured model, were recovered after Cur administration (Figure 2E). Notably, no significant difference was obtained between the Cur group and NAC group.
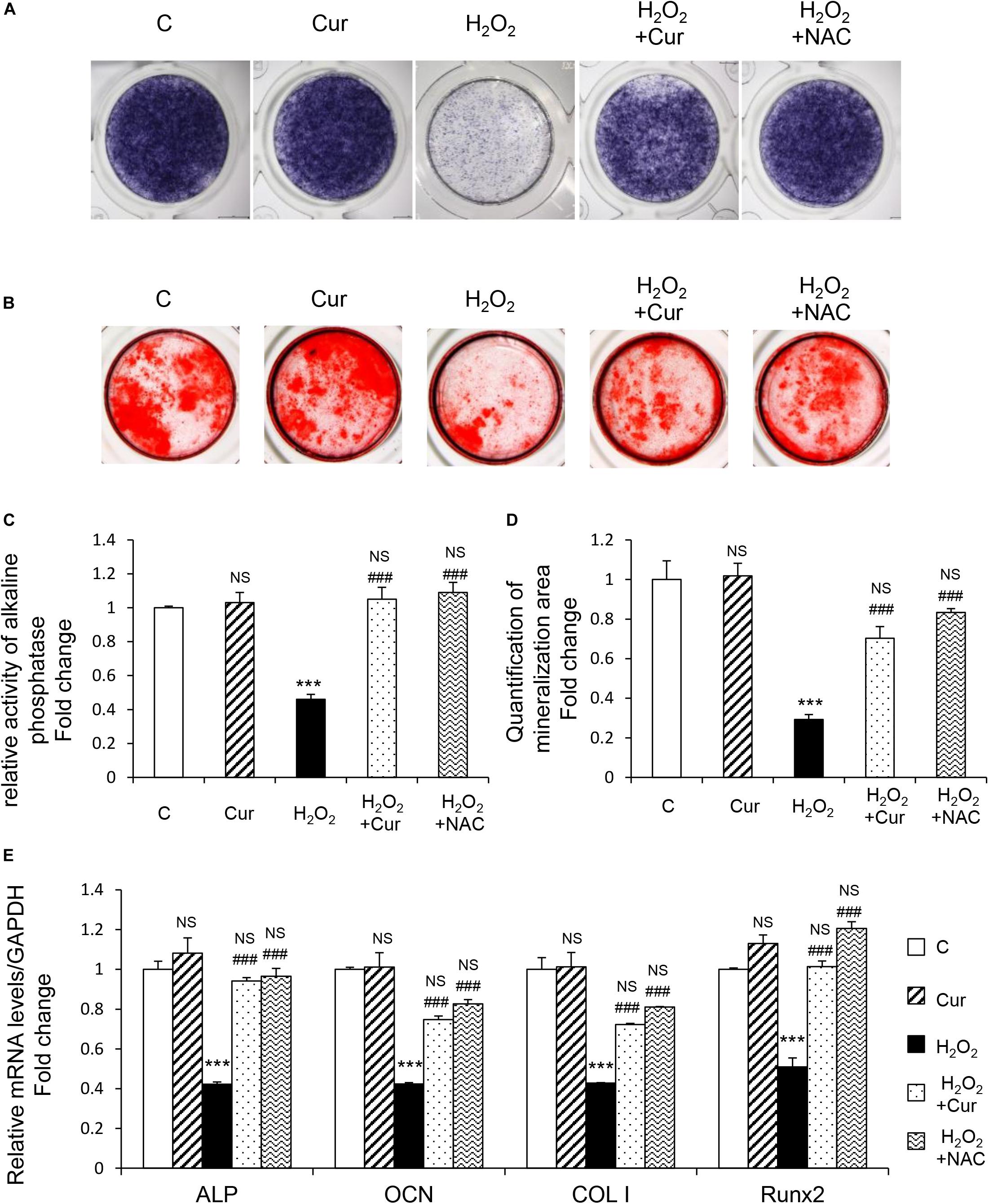
Figure 2. Cur rescue H2O2 induced osteoblast dysfunction. The MC3T3-E1 cells were treated in the osteogenesis differentiation medium with or without H2O2 and Cur. (A) After the cells cultured for a week and they were subjected to ALP staining in the indicated treatment groups. (B) The alizarin red staining of MC3T3-E1 cells showed the mineralizing matrix after cultured for 2 weeks. (C) ALP activity tested in MC3T3-E1 cells as indicated groups. (D) Mineralization area was evaluated by tests. (E) When cultured for 3 days the expression of osteogenic marker genes were analyzed. Data are presented as the mean ± SD from at least three independent experiments. *p < 0.05, **p < 0.01, ***p < 0.001, versus C group; NS, non-significantly different from C group. #p < 0.05, ##p < 0.01, ###p < 0.001, versus the H2O2 group; ns, non-significantly different from H2O2 group.
Cur Rescued the Expression of p-GSK3β and Nrf2 Decreased by H2O2
To further study the mechanism underlying protective effect of Cur, GSK3β-Nrf2 signaling pathway was detected.GSK3β activity was evaluated by the level of phosphorylated GSK3β at inhibitory serine 9 residues (Romorini et al., 2016). It was shown that H2O2 reduced phosphorylation of GSK3β without changing the total GSK3β levels (Figures 3A,B). Nrf2 is the highly sensitive transcription factor of OS and its expression was downregulated induced by H2O2 (Figures 3A,C). Cur increased the levels of p-GSK3β (Figures 3D,F) and Nrf2 (Figures 3E,G) in H2O2 treatment group, so as the NAC treatment group. Appling TDZD-8, the specific GSK3β inhibitor, reversed the reduction of p-GSK3β and Nrf2 (Figures 3H,I), while using tBHQ, the activator of Nrf2, only increased the level of Nrf2 but not p-GSK3β (Figures 3J,K). These results indicated that GSK3β was the upstream regulator of Nrf2. Remarkably, the H2O2-induced inhibition of GSK3β-Nrf2 signaling pathway was reversed by Cur.
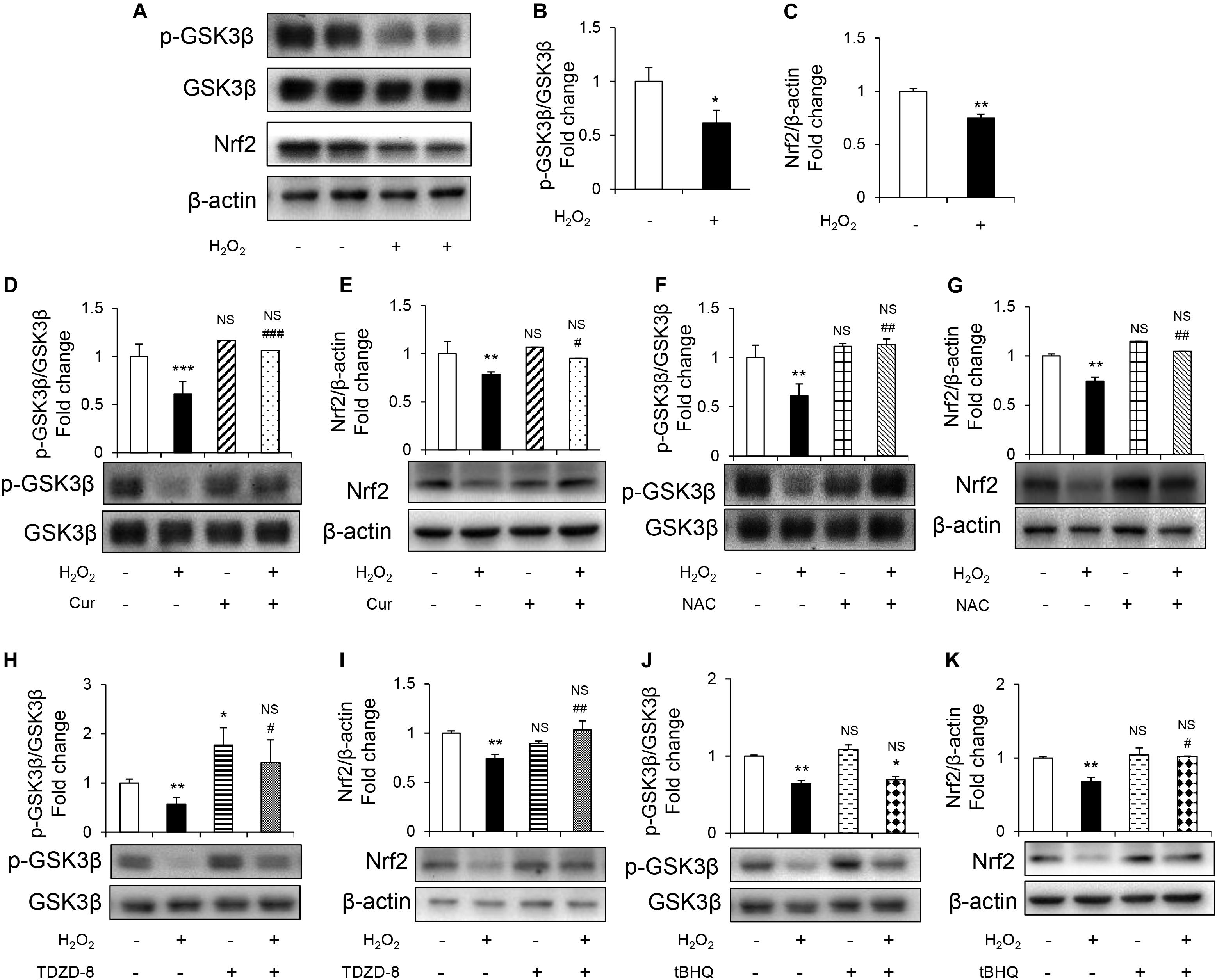
Figure 3. Cur attenuated H2O2-induced osteoblast apoptosis through GSK3β-Nrf2 signaling pathway. MC3T3-E1 cells were treated in the basic medium with or without H2O2 for 6 h. (A) Representative immunoreactive bands for phosphorylated GSK3β, GSK3β, and Nrf2 in MC3T3-E1 cells. (B,C) Quantification of immunoreactive bands for phosphorylated GSK3β relative to GSK3β and Nrf2 relative to β-actin. (D,E) Cur was added 24 h before H2O2. Densitometry of immunoreactive bands for p-GSK3β and GSK3β, Nrf2 and β-actin in MC3T3-E1 cells with (+) or without (–) Cur in the presence of H2O2 (+) or culture medium (–). (F,G) NAC was added 1 h before H2O2. Representative immunoreactive bands and relative levels of p-GSK3β and GSK3β, Nrf2 and β-actin in MC3T3-E1 cells with (+) or without (–) NAC treatment in the presence of H2O2 (+) or culture medium (–). Representative immunoblots are shown at the bottom. (H,I) TDZD-8 was added 1 h before H2O2. Representative immunoreactive bands and relative levels of p-GSK3β and GSK3β, Nrf2, and β-actin in MC3T3-E1 cells with (+) or without (–) TDZD-8 treatment in the presence of H2O2 (+) or culture medium (–). (J,K) tBHQ was added 1 h before H2O2. Representative immunoreactive bands and relative levels of p-GSK3β and GSK3β, Nrf2, and β-actin in MC3T3-E1 cells with (+) or without (–) tBHQ treatment in the presence of H2O2 (+) or culture medium (–). Data are presented as the mean ± SD from at least three independent experiments. *p < 0.05, **p < 0.01, ***p < 0.001, versus C group; NS, non-significantly different from C group. #p < 0.05, ##p < 0.01, ###p < 0.001, versus the H2O2 group; ns, non-significantly different from H2O2 group.
GSK3β-Nrf2 Signaling Pathway Activating Protects MC3T3-E1 Cells From the Oxidative Damage Induced by H2O2
TDZD-8 and tBHQ inhibited apoptosis induced by H2O2, as demonstrated by MTT test and TUNEL staining (Figures 4A–D). And the ROS homeostasis indicating H2O2-induced OS was reversed by TDZD-8 and tBHQ (Figures 4E–H). Furthermore, they also recovered the osteogenic differentiation ability, shown by ALP staining/activity and ARS (Figures 5A–D) as well as the expression of osteogenic genes (Figure 5E). All the results revealed that GSK3β-Nrf2 signaling pathway played a pivotal role in regulating osteoblasts apoptosis and dysfunction induced by OS.
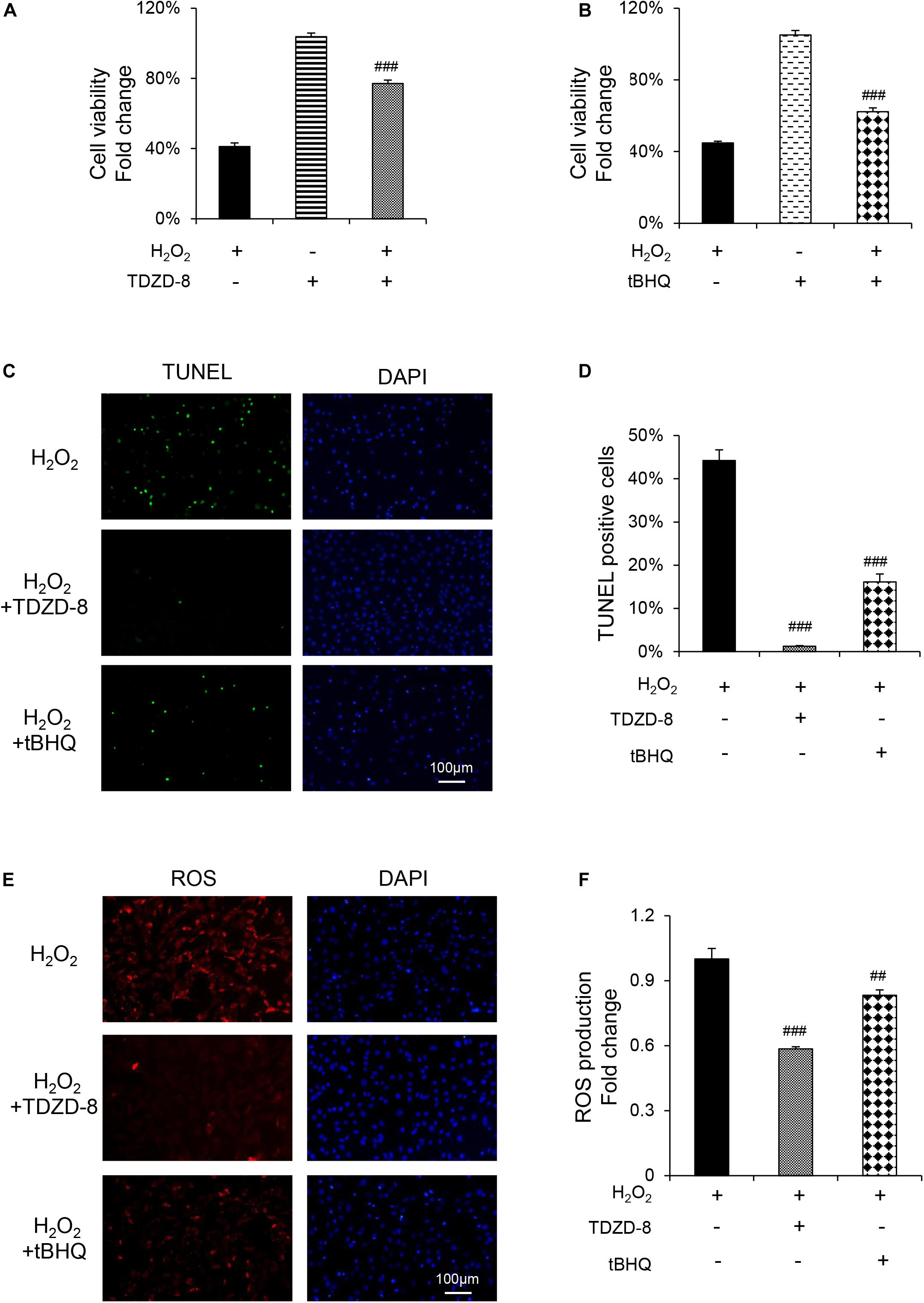
Figure 4. GSK3β-Nrf2 signaling pathway is involved in H2O2-induced apoptosis and ROS generation in MC3T3-E1 cells. MC3T3-E1 cells were treated with or without H2O2 in the basic medium for 6 h. TDZD-8 or tBHQ were added 1 h before H2O2. (A) Cell viability determined by MTT reduction in MC3T3-E1 cells with (+) or without (–) TDZD-8 treatment in the presence or absence of H2O2 (+). (B) Cell viability determined by MTT reduction in MC3T3-E1 cells with (+) or without (–) tBHQ treatment in the presence or absence of H2O2 (+). (C,D) The cells were immunostained for TUNEL (green). DAPI staining was used to mark the position of the nuclei. Scale bars = 100 μm. (E,F) The cells were harvested and stained with DCFH-DA (red). Scale bars = 100 μm. Data are presented as the mean ± SD from at least three independent experiments. *p < 0.05, **p < 0.01, ***p < 0.001, versus C group; NS, non-significantly different from C group. #p < 0.05, ##p < 0.01, ###p < 0.001, versus the H2O2 group; ns, non-significantly different from H2O2 group.
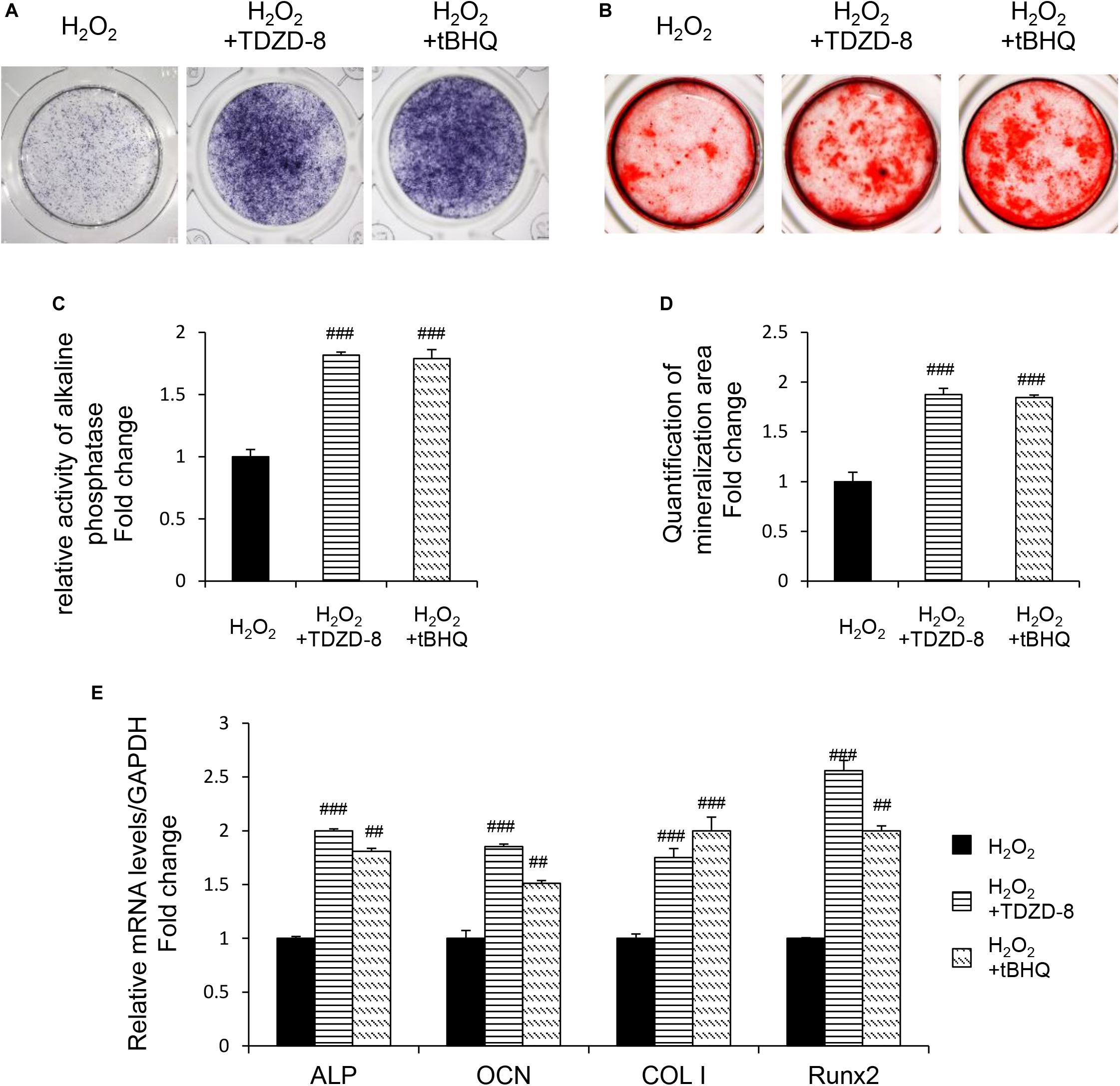
Figure 5. GSK3β-Nrf2 signaling pathway is involved in H2O2-induced osteoblast dysfunction. The MC3T3-E1 cells were treated in the osteogenesis differentiation medium with or without H2O2 for 6 h. TDZD-8 or tBHQ were added 1 h before H2O2. (A) After the cells cultured for 7 days and they were subjected to ALP staining in the indicated treatment groups. (B) The alizarin red staining of MC3T3-E1 cells showed the mineralizing matrix after cultured for 14 days. (C) ALP activity tested in MC3T3-E1 cells as indicated groups. (D) Mineralization capacity was evaluated by tests. (E) When cultured for 3 days the expression of osteogenic marker genes were analyzed. Data are presented as the mean ± SD from at least three independent experiments. *p < 0.05, **p < 0.01, ***p < 0.001, versus C group; NS, non-significantly different from C group. #p < 0.05, ##p < 0.01, ###p < 0.001, versus the H2O2 group; ns, non-significantly different from H2O2 group.
Discussion
Numerous studies have proved that OS-induced osteoblasts dysfunction plays an important role in the pathogenic progression of osteoporosis (Maggio et al., 2003; Baek et al., 2010). On the other hand, Cur, a classical antioxidant herb can efficaciously prevent and treat osteoporosis (Fu et al., 2015). However, the underlying molecular mechanism remains largely unveiled. In the present study, we found that OS significantly decreased osteoblasts viability and their osteoblastogenic differentiation. For the first time, we demonstrate that the suppression of GSK3β-Nrf2 signaling pathway could be a key molecular event accounting for these phenomena. We further showed that Cur effectively attenuated the oxidative damages and restored the differentiation of osteoblasts by retaining GSK3β-Nrf2 signaling.
Oxidative stress is characterized by the excessive generation of ROS, e.g., H2O2, O, and ⋅OH (Hu et al., 2019). H2O2 is the most stable and common form of ROS, and is suitable to be used both as an intra- and an intercellular signal (Denisova et al., 2001). Thus, H2O2 is considered to be a widely accepted initiator of cell oxidative injury model (Xu et al., 2011; Liang et al., 2013; Fu et al., 2015). In this study, we found that H2O2 could result in significantly increased ROS production, apoptosis as well as decreased cell viability in MC3T3-E1 cells. These results were in line with our previous findings that H2O2 exerted a profound cytotoxic effect on a human osteoblast-like cell line (Dai et al., 2017). We further showed that H2O2 also downregulated the osteoblastogenic differentiation, which was reflected by the significant reduction of ALP activity, calcium deposition and osteogenic genes’ expression. These findings were consistent with the previous reports that the suppressed bone regeneration in humans and animals was mainly caused by reduced osteoblast number and hindered osteogenic function (Marie and Kassem, 2011).
Curcumin can trap radicals to exert its antioxidant function, thereby being used to prevent and treat chronic metabolic diseases (Goel et al., 2008), such as osteoporosis (Hussan et al., 2012; Cho et al., 2013). Results of this study showed that the administration of Cur significantly attenuated cell toxicity effects of H2O2. DCFH-DA staining showed that, similar as NAC – a classical exogenous antioxidant, Cur could dramatically alleviate cytosolic ROS level induced by H2O2. In our previous study, we also demonstrate that Cur effectively decreases mitochondrial ROS and restores consequence dysfunction in human osteoblast-like cells (Chao et al., 2017). These findings suggested that Cur was a powerful and cell-organelle-non-specific antioxidant to suppress endogenous ROS production. Furthermore, in a non-OS microenvironment, Cur could not significantly influence cell viability and osteogenic functions. Consequently, the protective effect of Cur on osteoblasts was most likely attributed to its antioxidant property but not to its stimulatory effect on osteoblastogenesis. This character of Cur may partly explain why Cur is mostly used in primary or secondary osteoporosis (Hussan et al., 2012; Chen et al., 2016) other than bone formation under normal conditions. On the other hand, previous studies also show that Cur inhibits the proliferation and differentiation of human or rat osteoblasts (Notoya et al., 2006; Moran et al., 2012). These inconsistencies may be due to the varieties of cell types and different concentrations of Cur used in different studies. For example, Cur might lead to the apoptosis of MG-63 osteosarcoma cells at low concentrations, while it did not affect the viability of human osteoblasts at the same condition (Chang et al., 2014, 2015). Therefore, a further exploration needs to be performed to figure out the exact mechanisms for these phenomena.
Glycogen synthase kinase 3β is a rate-limiting enzyme of glycogen synthesis and acts as an important negative regulator of bone metabolism (Qi et al., 2015). Heterozygous GSK3β deficient mice exhibit increased bone formation (Noh et al., 2009). Pharmacological antagonists of GSK3β prevent skeletal unloading or ovariectomy-induced bone loss in mice (Warden et al., 2010; Zahoor et al., 2014). In the present study, H2O2 could dramatically decrease GSK3β phosphorylation (Ser 9) and lead to its activation. The inactivation of GSK3β by TDZD-8 not only attenuated H2O2-induced apoptosis, but also restored the osteogenic activity of the osteoblasts. Our previous study indicated that GSK3β inhibitor mitigated mitochondrial dysfunction of diabetic mice hippocampal (Huang et al., 2015) and Saos-2 cells (Dai et al., 2017), thereby alleviating oxidative damages. This finding suggested that GSK3β played a key role in the OS-induced osteoblasts injury. Cur significantly restored the level of p-GSK3β that was reduced by H2O2. Furthermore, Cur treatment also decreased ROS and protected osteoblasts from OS-damages. These results suggested that Cur might protect osteoblasts against H2O2-induced disturbance though the redox homeostasis-regulating function of GSK3β pathway.
Nuclear factor erythroid 2 related factor 2 has also been reported to play an important role in the regulation of bone metabolism. Nrf2-deficient mice exhibit a loss in BMD in femora and a reduction in cortical bone area in vertebrae (Ibanez et al., 2014). Our previous study demonstrates that Nrf2 downregulation is closely related to the enhanced alveolar bone loss in diabetic periodontitis in rats (Li et al., 2018). Moreover, the deletion of Nrf2 suppresses antioxidant enzymes and elevates the intracellular ROS level in osteoblasts, which significantly compromises their ability to differentiate and mineralize (Rana et al., 2012). In the present study, our results also found that the expression of Nrf2 significantly decreased in OS injury model of osteoblasts. The activation of Nrf2 by tBHQ, a specific chemical agonist of Nrf2, restored both cell viability and osteogenic activity. Notably, Cur efficaciously restored the expression of Nrf2, which alleviated OS and consequently attenuated H2O2-induced damages of osteoblasts. As a major regulatory transcription factor, Nrf2 responds to various cellular stresses and regulates the transcription of broad range of antioxidant genes. More recently, it is reported that Nrf2 and its downstream genes opposes transcriptional up-regulation of apoptosis-related genes (Lin et al., 2014; Pellegrini et al., 2016). The decreased apoptosis rate in our results suggested that the protective effect of Cur could be, at least partially, attributed to Nrf2 activation. On the other hand, our results showed that Cur increased the level of osteoblastogenic genes including Runx2, a transcription factor regulates other osteoblast-related genes (e.g., ALP, OCN, COL I) (Bialek et al., 2004; Komori, 2010), in osteoblasts under the exposure to H2O2. It is well recognized that Runx2 plays a key role in osteoblastic differentiation and bone formation. Nrf2 is reported to positively regulate the expression of Runx2 (Sim et al., 2016). Therefore, our finding suggested that Nrf2 stabilization and activation mediated the protective effects of Cur on the viability and differentiation of osteoblasts.
Nuclear factor erythroid 2 related factor 2, as a cell signaling sensor, can be negatively regulated by GSK3β (Liu et al., 2017; Cuadrado et al., 2018). In this study, TDZD-8 treatment significantly increased the expression of Nrf2, while tBHQ did not restore the expression of p-GSK3β. This finding suggested that Nrf2 was the downstream molecule of GSK3β. GSK3β-Nrf2 signaling pathway plays a crucial role in regulating the redox homeostasis and the downstream cell behavior (Lv et al., 2017; Yi et al., 2018). In this study, we, for the first time, demonstrated that Cur pretreatment significantly antagonized the destructive effects of H2O2 so as to enhance the level of p-GSK3β as well as Nrf2 expression. The enhancement of GSK3β-Nrf2 signaling was correlated to the reduced ROS level, decreased apoptosis, and retained cell differentiation and mineralization. Consistent with the results in this study, inactivation of GSK3β by TDZD-8 or activation of Nrf2 by tBHQ also protect osteoblasts from H2O2-mediated oxidative damages. Our finding suggested that Cur protected osteoblasts from H2O2-induced OS damages were most likely mediated by its capacity of retaining GSK3β-Nrf2 signaling pathway (Figure 6).
There are still some limitations in this study. We only adopted a mouse preosteoblast cell line. More primary cultured osteoblasts or cell lines are needed to affirm the mechanism underlying the protective effect of Cur on OS induced osteoblasts dysfunction. On the other hand, further in vivo studies should be arranged to verify the preventive effect of Cur on osteoporosis and corroborate the role of GSK3β-Nrf2 singling pathway.
Conclusion
Glycogen synthase kinase 3β-nuclear factor erythroid 2 related factor 2 activation and ROS scavenging could be the key mechanism responsible for Cur’s pro-survival and differentiation-promoting actions in H2O2 induced oxidative damage of MC3T3-E1 cells. Cur may act as a potential bone-protective therapeutic agent for the prevention or treatment of osteoporosis and effectively stimulate bone regeneration under OS pathological condition.
Data Availability Statement
The datasets generated for this study are available on request to the corresponding author.
Author Contributions
XL, SH, GW, and JM conceived and designed the study. XL, YC, YM, PD, XZ, HC, YW, and IB carried out the experiments and collated the data. XL and YC wrote the original draft. XS, SH, GW, and TF reviewed and edited the manuscript. All authors have read and approved the final submitted manuscript.
Funding
This project was funded by Science and Technology Project of Zhejiang Province (No. 2017C33081), National Natural Science Foundation of China (No. 81870777), Natural Science Foundation of Zhejiang Province (No. LY17H140010), Traditional Chinese Medicine Science and Technology Program of Zhejiang Province (No. 2019ZB077), Wenzhou Technology Bureau Project (No. 2018Y0724), Orthopedics Key Laboratory Open Fund of Zhejiang Province (No. ZJGK1807Y), China Scholarship Council (No. 201808330402), and Zhejiang Provincial College Students’ Science and Technology Innovation Project and Fresh Talent Program (Nos. 2019R413059 and 2019R413084). The funders had no role in study design, data collection, and analysis, the decision to publish, or the preparation of the manuscript.
Conflict of Interest
The authors declare that the research was conducted in the absence of any commercial or financial relationships that could be construed as a potential conflict of interest.
References
Almeida, M., Han, L., Ambrogini, E., Bartell, S. M., and Manolagas, S. C. (2010). Oxidative stress stimulates apoptosis and activates NF-kappaB in osteoblastic cells via a PKCbeta/p66shc signaling cascade: counter regulation by estrogens or androgens. Mol. Endocrinol. 24, 2030–2037. doi: 10.1210/me.2010-0189
Baek, K. H., Oh, K. W., Lee, W. Y., Lee, S. S., Kim, M. K., Kwon, H. S., et al. (2010). Association of oxidative stress with postmenopausal osteoporosis and the effects of hydrogen peroxide on osteoclast formation in human bone marrow cell cultures. Calcif. Tissue Int. 87, 226–235. doi: 10.1007/s00223-010-9393-9
Bialek, P., Kern, B., Yang, X., Schrock, M., Sosic, D., Hong, N., et al. (2004). A twist code determines the onset of osteoblast differentiation. Dev. Cell 6, 423–435. doi: 10.1016/s1534-5807(04)00058-9
Chang, R., Sun, L., and Webster, T. J. (2014). Short communication: selective cytotoxicity of curcumin on osteosarcoma cells compared to healthy osteoblasts. Int. J. Nanomed. 9, 461–465. doi: 10.2147/IJN.S55505
Chang, R., Sun, L., and Webster, T. J. (2015). Selective inhibition of MG-63 osteosarcoma cell proliferation induced by curcumin-loaded self-assembled arginine-rich-RGD nanospheres. Int. J. Nanomed. 10, 3351–3365. doi: 10.2147/IJN.S78756
Chao, P. Y., Lin, J. A., Ye, J. C., Hwang, J. M., Ting, W. J., Huang, C. Y., et al. (2017). Attenuation of oxidative stress-induced cell apoptosis in schwann RSC96 cells by Ocimum gratissimum aqueous extract. Int. J. Med. Sci. 14, 764–771. doi: 10.7150/ijms.19535
Chen, Z., Xue, J., Shen, T., Mu, S., and Fu, Q. (2016). Curcumin alleviates glucocorticoid-induced osteoporosis through the regulation of the Wnt signaling pathway. Int. J. Mol. Med. 37, 329–338. doi: 10.3892/ijmm.2015.2432
Cho, D. C., Jung, H. S., Kim, K. T., Jeon, Y., Sung, J. K., and Hwang, J. H. (2013). Therapeutic advantages of treatment of high-dose curcumin in the ovariectomized rat. J. Korean Neurosurg. Soc. 54, 461–466. doi: 10.3340/jkns.2013.54.6.461
Cuadrado, A., Kugler, S., and Lastres-Becker, I. (2018). Pharmacological targeting of GSK-3 and NRF2 provides neuroprotection in a preclinical model of tauopathy. Redox Biol. 14, 522–534. doi: 10.1016/j.redox.2017.10.010
Dai, P., Mao, Y., Sun, X., Li, X., Muhammad, I., Gu, W., et al. (2017). Attenuation of oxidative stress-induced osteoblast apoptosis by curcumin is associated with preservation of mitochondrial functions and increased Akt-GSK3beta signaling. Cell. Physiol. Biochem. 41, 661–677. doi: 10.1159/000457945
Denisova, N. A., Cantuti-Castelvetri, I., Hassan, W. N., Paulson, K. E., and Joseph, J. A. (2001). Role of membrane lipids in regulation of vulnerability to oxidative stress in PC12 cells: implication for aging. Free Radic. Biol. Med. 30, 671–678. doi: 10.1016/s0891-5849(00)00513-x
Farr, J. N., Xu, M., Weivoda, M. M., Monroe, D. G., Fraser, D. G., Onken, J. L., et al. (2017). Targeting cellular senescence prevents age-related bone loss in mice. Nat. Med. 23, 1072–1079. doi: 10.1038/nm.4385
Feng, J., Xu, H., Zhang, L., Wang, H., Liu, S., Liu, Y., et al. (2019). Development of nanocomplexes for curcumin vehiculization using ovalbumin and sodium alginate as building blocks: improved stability, bioaccessibility, and antioxidant activity. J. Agric. Food Chem. 67, 379–390. doi: 10.1021/acs.jafc.8b02567
Folwarczna, J., Zych, M., and Trzeciak, H. I. (2010). Effects of curcumin on the skeletal system in rats. Pharmacol. Rep. 62, 900–909. doi: 10.1016/s1734-1140(10)70350-9
Fonseca, H., Moreira-Goncalves, D., Coriolano, H. J., and Duarte, J. A. (2014). Bone quality: the determinants of bone strength and fragility. Sports Med. 44, 37–53. doi: 10.1007/s40279-013-0100-7
Fu, C., Xu, D., Wang, C. Y., Jin, Y., Liu, Q., Meng, Q., et al. (2015). Alpha-lipoic acid promotes osteoblastic formation in H2O2 -Treated MC3T3-E1 cells and prevents bone loss in ovariectomized rats. J. Cell. Physiol. 230, 2184–2201. doi: 10.1002/jcp.24947
Goel, A., Kunnumakkara, A. B., and Aggarwal, B. B. (2008). Curcumin as “Curecumin”: from kitchen to clinic. Biochem. Pharmacol. 75, 787–809. doi: 10.1016/j.bcp.2007.08.016
Hendrickx, G., Boudin, E., and Van Hul, W. (2015). A look behind the scenes: the risk and pathogenesis of primary osteoporosis. Nat. Rev. Rheumatol. 11, 462–474. doi: 10.1038/nrrheum.2015.48
Hu, K., Li, Y., Rotenberg, S. A., Amatore, C., and Mirkin, M. V. (2019). Electrochemical measurements of reactive oxygen and nitrogen species inside single phagolysosomes of living macrophages. J. Am. Chem. Soc. 141, 4564–4568. doi: 10.1021/jacs.9b01217
Huang, S., Wang, Y., Gan, X., Fang, D., Zhong, C., Wu, L., et al. (2015). Drp1-mediated mitochondrial abnormalities link to synaptic injury in diabetes model. Diabetes 64, 1728–1742. doi: 10.2337/db14-0758
Huang, S., Zheng, B., Jin, X., Yu, Q., Zhang, X., Sun, X., et al. (2019). Blockade of Cyclophilin D attenuates oxidative stress-induced cell death in human dental pulp cells. Oxid. Med. Cell. Longev. 2019:1729013. doi: 10.1155/2019/1729013
Hussan, F., Ibraheem, N. G., Kamarudin, T. A., Shuid, A. N., Soelaiman, I. N., and Othman, F. (2012). Curcumin protects against ovariectomy-induced bone changes in rat model. Evid. Based Complement. Alternat. Med. 2012:174916. doi: 10.1155/2012/174916
Ibanez, L., Ferrandiz, M. L., Brines, R., Guede, D., Cuadrado, A., and Alcaraz, M. J. (2014). Effects of Nrf2 deficiency on bone microarchitecture in an experimental model of osteoporosis. Oxid. Med. Cell. Longev. 2014:726590. doi: 10.1155/2014/726590
Jiang, X., Liu, Y., Liu, X., Wang, W., Wang, Z., Hu, Y., et al. (2018). Over-expression of a cardiac-specific human dopamine D5 receptor mutation in mice causes a dilated cardiomyopathy through ROS over-generation by NADPH oxidase activation and Nrf2 degradation. Redox Biol. 19, 134–146. doi: 10.1016/j.redox.2018.07.008
Johnell, O., and Kanis, J. A. (2006). An estimate of the worldwide prevalence and disability associated with osteoporotic fractures. Osteoporos. Int. 17, 1726–1733. doi: 10.1007/s00198-006-0172-4
Kim, W. K., Ke, K., Sul, O. J., Kim, H. J., Kim, S. H., Lee, M. H., et al. (2011). Curcumin protects against ovariectomy-induced bone loss and decreases osteoclastogenesis. J. Cell. Biochem. 112, 3159–3166. doi: 10.1002/jcb.23242
Komori, T. (2010). Regulation of bone development and extracellular matrix protein genes by RUNX2. Cell Tissue Res. 339, 189–195. doi: 10.1007/s00441-009-0832-8
Li, J., Wang, Q., Yang, R., Zhang, J., Li, X., Zhou, X., et al. (2017). BMI-1 mediates estrogen-deficiency-induced bone loss by inhibiting reactive oxygen species accumulation and T cell activation. J. Bone Miner. Res. 32, 962–973. doi: 10.1002/jbmr.3059
Li, M., Zhao, L., Liu, J., Liu, A. L., Zeng, W. S., Luo, S. Q., et al. (2009). Hydrogen peroxide induces G2 cell cycle arrest and inhibits cell proliferation in osteoblasts. Anat. Rec. 292, 1107–1113. doi: 10.1002/ar.20925
Li, X., Sun, X., Zhang, X., Mao, Y., Ji, Y., Shi, L., et al. (2018). Enhanced oxidative damage and Nrf2 downregulation contribute to the aggravation of periodontitis by diabetes mellitus. Oxid. Med. Cell. Longev. 2018:9421019. doi: 10.1155/2018/9421019
Liang, D., Xiang, L., Yang, M., Zhang, X., Guo, B., Chen, Y., et al. (2013). ZnT7 can protect MC3T3-E1 cells from oxidative stress-induced apoptosis via PI3K/Akt and MAPK/ERK signaling pathways. Cell. Signal. 25, 1126–1135. doi: 10.1016/j.cellsig.2013.02.003
Lin, H., Wei, B., Li, G., Zheng, J., Sun, J., Chu, J., et al. (2014). Sulforaphane reverses glucocorticoid-induced apoptosis in osteoblastic cells through regulation of the Nrf2 pathway. Drug Des. Devel. Ther. 8, 973–982. doi: 10.2147/DDDT.S65410
Liu, B., Zhang, H., Tan, X., Yang, D., Lv, Z., Jiang, H., et al. (2017). GSPE reduces lead-induced oxidative stress by activating the Nrf2 pathway and suppressing miR153 and GSK-3beta in rat kidney. Oncotarget 8, 42226–42237. doi: 10.18632/oncotarget.15033
Lv, H., Liu, Q., Wen, Z., Feng, H., Deng, X., and Ci, X. (2017). Xanthohumol ameliorates lipopolysaccharide (LPS)-induced acute lung injury via induction of AMPK/GSK3beta-Nrf2 signal axis. Redox Biol. 12, 311–324. doi: 10.1016/j.redox.2017.03.001
Maggio, D., Barabani, M., Pierandrei, M., Polidori, M. C., Catani, M., Mecocci, P., et al. (2003). Marked decrease in plasma antioxidants in aged osteoporotic women: results of a cross-sectional study. J. Clin. Endocrinol. Metab. 88, 1523–1527. doi: 10.1210/jc.2002-021496
Manolagas, S. C. (2010). From estrogen-centric to aging and oxidative stress: a revised perspective of the pathogenesis of osteoporosis. Endocr. Rev. 31, 266–300. doi: 10.1210/er.2009-0024
Mao, Y. X., Cai, W. J., Sun, X. Y., Dai, P. P., Li, X. M., Wang, Q., et al. (2018). RAGE-dependent mitochondria pathway: a novel target of silibinin against apoptosis of osteoblastic cells induced by advanced glycation end products. Cell Death Dis. 9:674. doi: 10.1038/s41419-018-0718-3
Maria, S., Samsonraj, R. M., Munmun, F., Glas, J., Silvestros, M., Kotlarczyk, M. P., et al. (2018). Biological effects of melatonin on osteoblast/osteoclast cocultures, bone, and quality of life: implications of a role for MT2 melatonin receptors, MEK1/2, and MEK5 in melatonin-mediated osteoblastogenesis. J. Pineal Res. 64:10.1111/ji.12465. doi: 10.1111/jpi.12465
Marie, P. J., and Kassem, M. (2011). Osteoblasts in osteoporosis: past, emerging, and future anabolic targets. Eur. J. Endocrinol. 165, 1–10. doi: 10.1530/EJE-11-0132
Mohd Effendy, N., Abdullah, S., Yunoh, M. F., and Shuid, A. N. (2015). Time and dose-dependent effects of Labisia pumila on the bone strength of postmenopausal osteoporosis rat model. BMC Complement. Altern. Med. 15:58. doi: 10.1186/s12906-015-0567-x
Molnar, G. A., Kun, S., Selley, E., Kertesz, M., Szelig, L., Csontos, C., et al. (2016). Role of tyrosine isomers in acute and chronic diseases leading to oxidative stress – a review. Curr. Med. Chem. 23, 667–685. doi: 10.2174/0929867323666160119094516
Moran, J. M., Roncero-Martin, R., Rodriguez-Velasco, F. J., Calderon-Garcia, J. F., Rey-Sanchez, P., Vera, V., et al. (2012). Effects of curcumin on the proliferation and mineralization of human osteoblast-like cells: implications of nitric oxide. Int. J. Mol. Sci. 13, 16104–16118. doi: 10.3390/ijms131216104
Nevius, E., Pinho, F., Dhodapkar, M., Jin, H., Nadrah, K., Horowitz, M. C., et al. (2015). Oxysterols and EBI2 promote osteoclast precursor migration to bone surfaces and regulate bone mass homeostasis. J. Exp. Med. 212, 1931–1946. doi: 10.1084/jem.20150088
Noh, T., Gabet, Y., Cogan, J., Shi, Y., Tank, A., Sasaki, T., et al. (2009). Lef1 haploinsufficient mice display a low turnover and low bone mass phenotype in a gender- and age-specific manner. PLoS One 4:e5438. doi: 10.1371/journal.pone.0005438
Notoya, M., Nishimura, H., Woo, J. T., Nagai, K., Ishihara, Y., and Hagiwara, H. (2006). Curcumin inhibits the proliferation and mineralization of cultured osteoblasts. Eur. J. Pharmacol. 534, 55–62. doi: 10.1016/j.ejphar.2006.01.028
Olagnier, D., Brandtoft, A. M., Gunderstofte, C., Villadsen, N. L., Krapp, C., Thielke, A. L., et al. (2018). Nrf2 negatively regulates STING indicating a link between antiviral sensing and metabolic reprogramming. Nat. Commun. 9:3506. doi: 10.1038/s41467-018-05861-7
Pellegrini, G. G., Morales, C. C., Wallace, T. C., Plotkin, L. I., and Bellido, T. (2016). Avenanthramides prevent osteoblast and osteocyte apoptosis and induce osteoclast apoptosis in vitro in an Nrf2-independent manner. Nutrients 8:423. doi: 10.3390/nu8070423
Qi, J., Hu, K. S., and Yang, H. L. (2015). Roles of TNF-alpha, GSK-3beta and RANKL in the occurrence and development of diabetic osteoporosis. Int. J. Clin. Exp. Pathol. 8, 11995–12004.
Rana, T., Schultz, M. A., Freeman, M. L., and Biswas, S. (2012). Loss of Nrf2 accelerates ionizing radiation-induced bone loss by upregulating RANKL. Free Radic. Biol. Med. 53, 2298–2307. doi: 10.1016/j.freeradbiomed.2012.10.536
Romorini, L., Garate, X., Neiman, G., Luzzani, C., Furmento, V. A., Guberman, A. S., et al. (2016). AKT/GSK3beta signaling pathway is critically involved in human pluripotent stem cell survival. Sci. Rep. 6:35660. doi: 10.1038/srep35660
Sahin, K., Orhan, C., Tuzcu, Z., Tuzcu, M., and Sahin, N. (2012). Curcumin ameloriates heat stress via inhibition of oxidative stress and modulation of Nrf2/HO-1 pathway in quail. Food Chem. Toxicol. 50, 4035–4041. doi: 10.1016/j.fct.2012.08.029
Serizawa, K., Yogo, K., Tashiro, Y., Takeda, S., Kawasaki, R., Aizawa, K., et al. (2016). Eldecalcitol prevents endothelial dysfunction in postmenopausal osteoporosis model rats. J. Endocrinol. 228, 75–84. doi: 10.1530/JOE-15-0332
Shepstone, L., Lenaghan, E., Cooper, C., Clarke, S., Fong-Soe-Khioe, R., Fordham, R., et al. (2018). Screening in the community to reduce fractures in older women (SCOOP): a randomised controlled trial. Lancet 391, 741–747. doi: 10.1016/S0140-6736(17)32640-5
Sim, H. J., Kim, J. H., Kook, S. H., Lee, S. Y., and Lee, J. C. (2016). Glucose oxidase facilitates osteogenic differentiation and mineralization of embryonic stem cells through the activation of Nrf2 and ERK signal transduction pathways. Mol. Cell. Biochem. 419, 157–163. doi: 10.1007/s11010-016-2760-8
Tokac, M., Taner, G., Aydin, S., Ozkardes, A. B., Dundar, H. Z., Taslipinar, M. Y., et al. (2013). Protective effects of curcumin against oxidative stress parameters and DNA damage in the livers and kidneys of rats with biliary obstruction. Food Chem. Toxicol. 61, 28–35. doi: 10.1016/j.fct.2013.01.015
Warden, S. J., Hassett, S. M., Bond, J. L., Rydberg, J., Grogg, J. D., Hilles, E. L., et al. (2010). Psychotropic drugs have contrasting skeletal effects that are independent of their effects on physical activity levels. Bone 46, 985–992. doi: 10.1016/j.bone.2009.12.031
Wu, J., Pan, X., Fu, H., Zheng, Y., Dai, Y., Yin, Y., et al. (2017). Effect of curcumin on glycerol-induced acute kidney injury in rats. Sci. Rep. 7:10114. doi: 10.1038/s41598-017-10693-4
Wu, Y., Wang, D., Wang, X., Wang, Y., Ren, F., Chang, D., et al. (2011). Caspase 3 is activated through caspase 8 instead of caspase 9 during H2O2-induced apoptosis in HeLa cells. Cell. Physiol. Biochem. 27, 539–546. doi: 10.1159/000329955
Xu, Z., Greenblatt, M. B., Yan, G., Feng, H., Sun, J., Lotinun, S., et al. (2017). SMURF2 regulates bone homeostasis by disrupting SMAD3 interaction with vitamin D receptor in osteoblasts. Nat. Commun. 8:14570. doi: 10.1038/ncomms14570
Xu, Z. S., Wang, X. Y., Xiao, D. M., Hu, L. F., Lu, M., Wu, Z. Y., et al. (2011). Hydrogen sulfide protects MC3T3-E1 osteoblastic cells against H2O2-induced oxidative damage-implications for the treatment of osteoporosis. Free Radic. Biol. Med. 50, 1314–1323. doi: 10.1016/j.freeradbiomed.2011.02.016
Yang, M. W., Wang, T. H., Yan, P. P., Chu, L. W., Yu, J., Gao, Z. D., et al. (2011). Curcumin improves bone microarchitecture and enhances mineral density in APP/PS1 transgenic mice. Phytomedicine 18, 205–213. doi: 10.1016/j.phymed.2010.05.011
Yi, J., Yuan, Y., Zheng, J., and Hu, N. (2018). Hydrogen sulfide alleviates uranium-induced kidney cell apoptosis mediated by ER stress via 20S proteasome involving in Akt/GSK-3beta/Fyn-Nrf2 signaling. Free Radic. Res. 52, 1020–1029. doi: 10.1080/10715762.2018.1514603
Yodkeeree, S., Chaiwangyen, W., Garbisa, S., and Limtrakul, P. (2009). Curcumin, demethoxycurcumin and bisdemethoxycurcumin differentially inhibit cancer cell invasion through the down-regulation of MMPs and uPA. J. Nutr. Biochem. 20, 87–95. doi: 10.1016/j.jnutbio.2007.12.003
Zahoor, M., Cha, P. H., Min do, S., and Choi, K. Y. (2014). Indirubin-3’-oxime reverses bone loss in ovariectomized and hindlimb-unloaded mice via activation of the Wnt/beta-catenin signaling. J. Bone Miner. Res. 29, 1196–1205. doi: 10.1002/jbmr.2147
Zhong, Z. M., Bai, L., and Chen, J. T. (2009). Advanced oxidation protein products inhibit proliferation and differentiation of rat osteoblast-like cells via NF-kappaB pathway. Cell. Physiol. Biochem. 24, 105–114. doi: 10.1159/000227818
Zhou, Q., Zhu, L., Zhang, D., Li, N., Li, Q., Dai, P., et al. (2016). Oxidative stress-related biomarkers in postmenopausal osteoporosis: a systematic review and meta-analyses. Dis. Markers 2016:7067984. doi: 10.1155/2016/7067984
Zhu, S. Y., Zhuang, J. S., Wu, Q., Liu, Z. Y., Liao, C. R., Luo, S. G., et al. (2018). Advanced oxidation protein products induce pre-osteoblast apoptosis through a nicotinamide adenine dinucleotide phosphate oxidase-dependent, mitogen-activated protein kinases-mediated intrinsic apoptosis pathway. Aging Cell 17:e12764. doi: 10.1111/acel.12764
Keywords: curcumin, oxidative stress, osteoblast, dysfunction, GSK3β, Nrf2
Citation: Li X, Chen Y, Mao Y, Dai P, Sun X, Zhang X, Cheng H, Wang Y, Banda I, Wu G, Ma J, Huang S and Forouzanfar T (2020) Curcumin Protects Osteoblasts From Oxidative Stress-Induced Dysfunction via GSK3β-Nrf2 Signaling Pathway. Front. Bioeng. Biotechnol. 8:625. doi: 10.3389/fbioe.2020.00625
Received: 25 April 2020; Accepted: 21 May 2020;
Published: 16 June 2020.
Edited by:
Yin Xiao, Queensland University of Technology, AustraliaReviewed by:
Kaili Lin, Shanghai Jiao Tong University, ChinaLorenzo Fassina, University of Pavia, Italy
Copyright © 2020 Li, Chen, Mao, Dai, Sun, Zhang, Cheng, Wang, Banda, Wu, Ma, Huang and Forouzanfar. This is an open-access article distributed under the terms of the Creative Commons Attribution License (CC BY). The use, distribution or reproduction in other forums is permitted, provided the original author(s) and the copyright owner(s) are credited and that the original publication in this journal is cited, in accordance with accepted academic practice. No use, distribution or reproduction is permitted which does not comply with these terms.
*Correspondence: Gang Wu, g.wu@acta.nl; Jianfeng Ma, dentistmacn@aliyun.com; Shengbin Huang, huangsb003@wmu.edu.cn
†These authors have contributed equally to this work