- 1Department of Hand Surgery, The Second Hospital of Jilin University, Changchun, China
- 2Department of Burn Surgery, The First Hospital of Jilin University, Changchun, China
- 3Department of Biobank, Division of Clinical Research, The First Hospital of Jilin University, Changchun, China
- 4Key Laboratory of Organ Regeneration and Transplantation, The First Hospital of Jilin University, Changchun, China
The increasing number of patients with chronic wounds caused by diseases, such as diabetes, malignant tumors, infections, and vasculopathy, has caused severe economic and social burdens. The main clinical treatments for chronic wounds include the systemic use of antibiotics, changing dressings frequently, operative debridement, and flap repair. These routine therapeutic strategies are characterized by a long course of treatment, substantial trauma, and high costs, and fail to produce satisfactory results. Biomaterial dressings targeting the different stages of the pathophysiology of chronic wounds have become an active research topic in recent years. In this review, after providing an overview of the epidemiology of chronic wounds, and the pathophysiological characteristics of chronic wounds, we highlight the functional biomaterials that can enhance chronic wound healing through debridement, anti-infection and antioxidant effects, immunoregulation, angiogenesis, and extracellular matrix remodeling. It is hoped that functional biomaterials will resolve the treatment dilemma for chronic wounds and improve patient quality of life.
Introduction
Owing to an increasing number of patients with chronic diseases such as diabetes, there are more than 6.5 million patients with chronic wounds worldwide, and the overall medical cost for treating chronic wounds exceeds 25 billion US dollars per year (Sen et al., 2009). Chronic diseases including diabetes, cardiovascular and cerebrovascular diseases, hypoxia, cancer, and immunosuppression are causes of chronic wounds, as are local vascular disease, infection, and repetitive trauma (Morton and Phillips, 2016). According to the etiology, the categories with the highest incidence of chronic wounds are diabetic foot ulcers (DFUs), venous leg ulcers, and pressure ulcers. DFUs alone occur in more than 450 million adult diabetes patients, and there is a 25% lifetime chance of developing diabetes (Boulton et al., 2005; Cho et al., 2018). The high prevalence, high amputation rate, and high recurrence rate of DFUs cause a serious economic and social burden (Boulton et al., 2005; Cho et al., 2018). However, innovations in clinical treatment methods and wound care have been insufficient for the past century (Harding, 2015). Familiarity with the anatomical structure and function of the skin, as well as the difference between acute wound healing and chronic wound healing, is essential for the treatment of chronic wounds.
The anatomy of normal skin is mainly composed of epidermis, dermis, and subcutaneous tissue. The avascular epidermis layer is closely connected to the dermis layer through the basement membrane, and the barrier function and prevention of fluid loss of the skin are mainly provided by the stratum corneum structure in the epidermal. Dermal matrix components, predominantly collagen (Col) and elastin, are derived from fibroblasts. The main cell types of the subcutaneous tissue are fibroblasts and adipocytes (Mathes et al., 2014).
Epidermal stem cells located in the epidermis, sweat glands, and sebaceous glands can effectively promote skin regeneration under physiological and pathological conditions (Snippert et al., 2010). To ensure the continuity of the skin structure and the integrity of its function, a dynamic and highly controlled recovery process can be initiated after injury. This healing process, which involves different types of cells and intracellular and intercellular signaling pathways, has been extensively studied (Gurtner et al., 2008). The healing process of acute wounds in healthy individuals is rapid and orderly. This process consists of four sequential and partially intertwined stages, hemostasis, inflammation, proliferation, and tissue remodeling, which represent the repair principles of most tissues (Gurtner et al., 2008; Gonzalez et al., 2016). The fibrin network formed during the hemostatic phase not only helps to enhance the barrier function but also promotes cell migration and fibroblast proliferation. Increased local penetration of neutrophils, lymphocytes, and monocytes represents the entry into the inflammatory phase, which generally lasts less than a week. During the proliferation phage, after the keratinocytes migrate to the damaged dermis, granulation tissue replaces the fibrin network formed during the hemostasis period. This new matrix is conducive to the maturation of keratinocytes, which completes the epithelialization process. The tissue remodeling period can start 2 weeks after injury, and the duration may be up to 1 year. The cells activated in the previous stage are reduced, and the conversion of type III Col to type I Col under the action of matrix metalloproteinases (MMPs) can enhance the repair of skin tissue during this stage (Gurtner et al., 2008; Gonzalez et al., 2016). The outcomes of acute wound healing can be classified as regeneration or repair, that is, epidermal-specific tissue replacement or repair with scar tissue, respectively (Reinke and Sorg, 2012).
If the healing process does not proceed smoothly, the wound will evolve into a chronic wound (Nunan et al., 2014). It is generally believed that chronic wounds cannot be repaired in a normal, orderly, and timely manner. In humans, anatomical and functional integrity cannot be restored within 3 months (Nunan et al., 2014). The physical space-occupying effect of the increase in local necrotic tissue, infection caused by various pathogens, poor local vascular conditions, and excessive levels of pro-inflammatory cytokines, proteases, and reactive oxygen species (ROS), etc. can all cause a wound to stagnate at the inflammatory reaction stage, resulting in delayed healing, or failure to heal. This may occur even after some tissue remodeling in partial wounds, which often leads to severe scar hyperplasia or keloids (Nunan et al., 2014; Zhao et al., 2016). There are clinical treatments for these complications, including systemic application of antibiotics, frequent changing of dressings, operative debridement, and flap repair. Other therapeutic techniques include the application of hyperbaric oxygen therapy (HBOT) to improve the wound microenvironment by increasing local oxygen content, the use of negative pressure-assisted wound therapy (RNPT) to improve the local blood supply, and the use of platelet-rich plasma (PRP) that is rich in cytokines and nutritional factors to treat chronic wounds (O'reilly et al., 2011; Topaz, 2012; Powers et al., 2016; Weller et al., 2019). However, the conventional treatment methods have the several disadvantages, including the need for anesthesia in patients undergoing operative debridement, the side effects of systemic application of antibiotics, and the repair of chronic wounds caused by flaps and skin grafts. HBOT, RNPT, and PRP only serve as adjuvant treatments for wounds. In short, all the above treatment methods fail to change the microenvironment of chronic wounds, which is not conducive to repair, and thus they do not represent breakthroughs in the treatment of chronic wounds. In addition, progress in wound care over the past century has been very slow (Harding, 2015).
In view of the limitations of traditional treatments, biomaterials have received extensive attention regarding their potential applications in chronic wounds. Many commercial wound dressings, such as alginate and hydrocolloid-based dressings, shorten the healing time of chronic wounds by maintaining a moist microenvironment that can maintain cell activity and accelerate debridement and vascularization, and have been shown to have therapeutic potential (Field and Kerstein, 1994; Serena et al., 2016; Jones et al., 2018). Protease-modulating matrix products have also shown promise in the treatment of chronic wounds (Jones et al., 2018). In view of the increasing number of basic research studies of the treatment of chronic wounds with biomaterials, it is necessary to systematically summarize the functionalization of biomaterials. In this review, we focus on research progress with respect to biomaterials used for debridement and to achieve anti-infection and antioxidation effects, immunoregulation, angiogenesis, and extracellular matrix (ECM) remodeling in chronic wounds. A schematic of this review is shown in Scheme 1.
Functional Biomaterials for Treatment of Chronic Wounds
The main role of functional biomaterials in the treatment of chronic wounds is to promote skin repair by adjusting the microenvironment of the wound (Kruse et al., 2015). Here, we introduce the characteristics of various functional biomaterials, their mechanisms, and their effects on chronic wound healing. Modern functional biomaterial dressings for chronic wounds are summarized in Table 1.
Debridement
Debridement is one of the key processes in preparing chronic wound beds. Bacteria and toxins often accumulate in necrotic tissue, which is composed mainly of inactivated Col. The long-term presence of necrotic tissue can cause local or even systemic infections. Tight combination of the necrotic tissue and the wound bed also has a physical occupying effect, which seriously hinders wound healing. Clearing the necrotic tissue can reduce the burden of bacteria and abnormal-phenotype cells, reduce local edema, and normalize the microenvironment of the wound surface (Falanga, 2004). Despite the obvious advantages of wound debridement, the impact of multiple operative debridement procedures and the requirement for anesthesia have severely limited its wide application in patients with underlying diseases.
Hydrogels with different gel-forming mechanisms and components are among the most widely used biomaterials (Wang Y. et al., 2019; Zhang et al., 2019c; Lin et al., 2020), and also play an important part in chronic debridement. Hydrogel dressings achieve debridement by rehydration, molting, and removal of inactivated and necrotic tissue. Sterigel®, an amorphous hydrogel derived from corn bran, was used for debridement of wound necrotic tissue and sloughing as early as 1997 (Williams, 1997). Hydrogel dressings are not suitable for chronic wounds with substantial or continuous exudation, and their applications are limited by the water absorption capacity of the hydrogel. As carboxymethyl cellulose adsorption particles have strong water absorption capacity, hydrocolloid occlusive dressings composed of carboxymethyl cellulose and polyurethane are suitable for wounds with more exudate. When the hydrocolloid dressing comes into contact with the wound exudate, the hydrogel matrix forms a hydrogel layer after swelling with water; the moist milieu created by the hydrocolloid promotes debridement and stimulates the formation of granulation tissue (Eaglstein, 2001). One meta-analysis of 12 randomized controlled trials including patients with venous ulcers and pressure ulcers showed that the healing rate of ulcers in the hydrocolloid dressing group was higher than that in the traditional gauze dressing group (Singh et al., 2004). Protease loaded polyacrylate-based dressing can also achieve effective debridement of chronic wounds (Humbert et al., 2014).
The effect of autolytic debridement after injury is often limited and the time is longer, the application of dressings with various debridement functions has also achieved good therapeutic effects in chronic wounds. Such dressings are especially suitable for patients in poor physical condition.
Anti-infection
Chronic wounds have an increased risk of endogenous and exogenous infections. Infections lead to increased local or systemic inflammation, which is often an important cause of chronic wounds not healing or even deepening (Gjdsbl et al., 2006; Agostinho et al., 2011). Chronic wounds are often colonized by more than two bacterial species, the most common of which are Staphylococcus aureus and Pseudomonas aeruginosa (Gjdsbl et al., 2006). Bacteria in chronic wounds do not exist in a suspended state but colonize the ECM in the form of complex bacterial biofilms. This is often the reason for the emergence of drug-resistant bacteria, such as extended-spectrum β-lactamase-positive Escherichia coli (ESBL E. coli) and methicillin-resistant S. aureus (MRSA) (Suryaletha et al., 2018).
The treatment of chronic wounds with bacterial colonization generally involves interfering with the formation of new bacterial biofilms, removing the biofilms that have formed, and minimizing the use of antibiotics. A variety of natural and synthetic polymer-based antibacterial functional dressings, including types of hydrogel, foam, and fiber, have been shown to have anti-infection effects. Hydrogels are polymer solid materials with a three-dimensional (3D) network structure containing water as a dispersion medium. Chitosan (CS)-based dressings have many advantages, including excellent degradability and compatibility; they are also non-toxic and exhibits excellent antibacterial activity (Wang et al., 2012). In short, antibacterial CS dressings can effectively improve the local microenvironment of a wound (Archana et al., 2013); Ding et al. synthesized a series of polyurethane foam dressings with different cationic group contents, which showed antibacterial properties against both Gram-positive cocci and Gram-negative bacilli (Ding Y. et al., 2019); the uniquely high surface area to volume ratio and high porosity of fiber and electrospun nanofiber scaffolds enable them to effectively simulate the microenvironment of the ECM. Thus, such scaffolds are widely used in tissue repair, including in antibacterial treatments for chronic wounds (Ranjbar-Mohammadi et al., 2016; Ding J. et al., 2019; Feng et al., 2019a; Zhang et al., 2019a,b). Arenbergerova et al. prepared a polyurethane electrospun nanofiber dressing loaded with a photosensitizer, tetraphenylporphyrin (TPP). The activation of TPP by visible light produced cytotoxic singlet oxygen, which enhanced the antibacterial function of the dressing. The thin and transparent nature of this dressing maximized the photosensitizer activity (Arenbergerova et al., 2012).
Silver ion products such as silver sulfadiazine are excellent antibacterial agents and have been widely used in the treatment of burn wounds. However, they have shortcomings, including causing increased MMP expression. In recent years, silver nanoparticles (AgNPs) have been widely used in the treatment of chronic wounds owing to their broad-spectrum antimicrobial properties and safety. AgNPs inactivate pathogenic microorganisms by breaking disulfide bonds in proteins, thereby changing the tertiary structure of the proteins (Liau et al., 1997; Lara et al., 2011). Masood et al. fabricated AgNPs incorporated in a CS/poly(ethylene glycol) (PEG) hydrogel. Silver nitrate was reduced by CS and PEG solution to prepare the AgNPs, and then cross-linked with glutaraldehyde to produce the AgNP-loaded CS/PEG hydrogel. The incorporation of AgNPs increased the porosity and swelling capacity of the CS/PEG hydrogel. Further in vivo experiments confirmed that the AgNP-CS/PEG hydrogel effectively promoted the healing of diabetic wounds in rabbits (Masood et al., 2019). As one of the important trace elements, copper ions have attracted attention for their antibacterial function in chronic wounds. Qiao et al. developed protein-stabilized nano-copper sulfate (CuS) nanodots, using the photothermal effect of CuS to enhance their bactericidal properties. As shown in Figure 1, the synergistic action of the photothermal effect of the CuS nanodots and the release of copper ions produced a robust bactericidal effect on ESBL E. coli and MRSA in vitro. Further in vivo experiments used an MRSA-infected circular wound with a diameter of 0.7 cm in diabetic mice, and found that the experimental group basically healed after 12 days (Qiao et al., 2019).
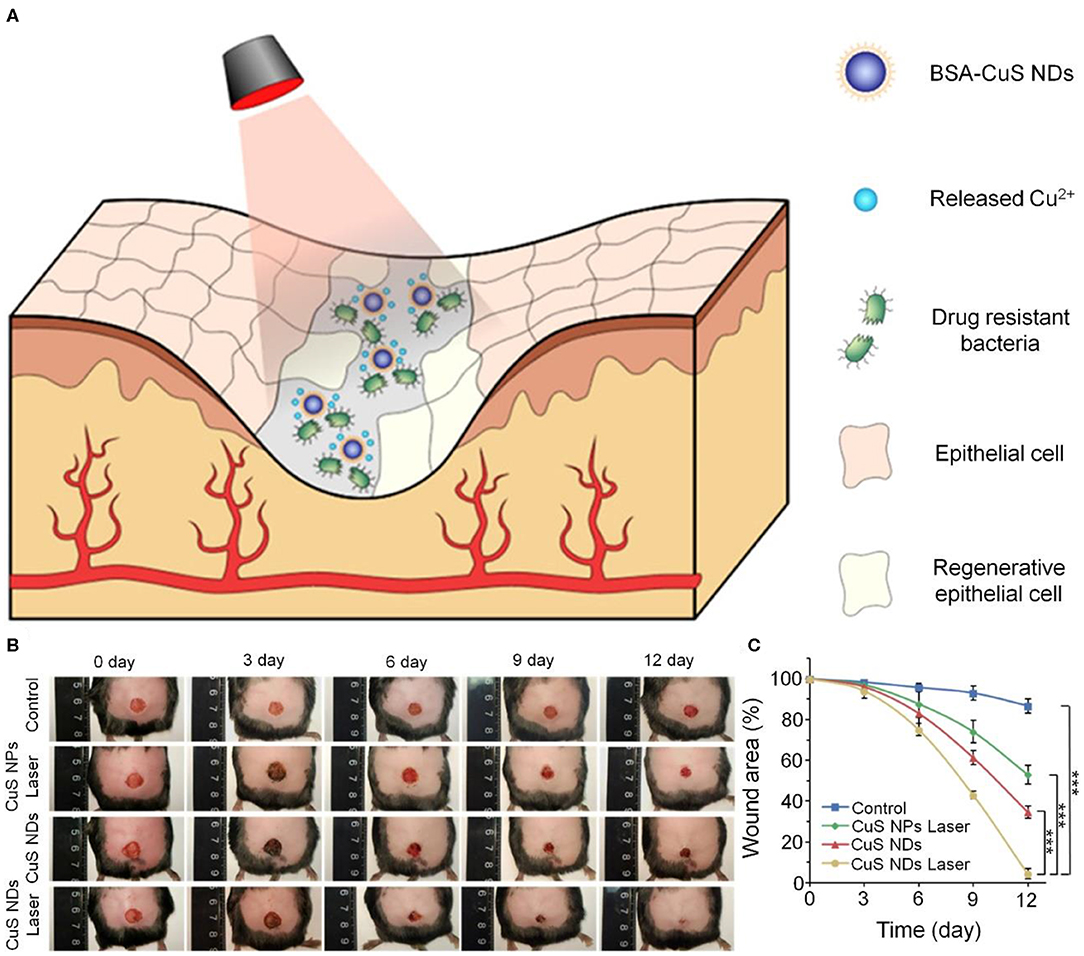
Figure 1. Antibacterial effect of laser-activated CuS nanodots. (A) Schematic diagram of CuS nanodots combined with laser irradiation to enhance antibacterial effect. (B) Wound images of MRSA-infected diabetic wound in mice. (C) Quantitative analysis of wound areas. ***p < 0.001. Reproduced with permission from Qiao et al. (2019).
Optimization of drug delivery systems is also an effective way to improve antibacterial performance. A cyclodextrin-based hydrogel was used as a drug delivery system to improve the efficacy of gallic acid, a polyphenol and broad-spectrum antimicrobial agent. The hydrophobic porosity and hydrophilic surfaces of cyclodextrin-based hydrogels improve their ability to encapsulate multiple molecules, which in this case helped to maintain the activity of gallic acid and enhance its antibacterial ability (Pinho et al., 2014). López-Iglesias et al. used CS/aerogel particles as carriers to enable sustained release of vancomycin, and achieved effective inhibition of MRSA while reducing the amount of antibiotic used and avoiding bacterial resistance (López-Iglesias et al., 2019). He et al. prepared a two-stage wound dressing, using nano silver and Calculus bovis as anti-infection and anti-inflammatory agents, respectively. Drug release experiments showed that the antibacterial phase occurred earlier than the long-duration drug release phase. Thus, the drug-loading system achieved rapid anti-infection, continuous reduction of inflammatory response, and accelerated healing of S. aureus-infected wounds (He et al., 2017).
Antioxidant
The increase of the right amount of oxidant accelerates the healing of wounds in mice, as low concentrations of ROS, including superoxide anions (), hydrogen peroxide (H2O2), and hydroxyl radicals (·OH), serve as signaling messengers to regulate gene expression. Low level of ROS are beneficial for maintaining cellular environmental homeostasis (Sen et al., 2002), whereas excessive ROS causes significant damage to nucleic acids and growth factors, and impairs angiogenesis in chronic wounds, which in turn hinders the healing process.
It has been proven that biomaterials and bioactive agents with antioxidative function, including diol-citrate esters, edaravone, and cerium dioxide, can accelerate chronic wound healing (Luo et al., 2004; Zhu et al., 2016; Speidel et al., 2017; Fan et al., 2019; Wu et al., 2019).
Zhu et al. fabricated a poly(polyethylene glycol citrate-co-N-isopropylacrylamide) (PPCN) hydrogel through continuous polycondensation and free radical polymerization reactions. The antioxidant diol-citrate esters endowed this temperature-sensitive PPCN hydrogel with antioxidant properties. Compared with the PBS group, the antioxidant PPCN hydrogel group more effectively promoted the regeneration of the epidermis and the appendages. Moreover, the PPCN content of the hydrogel could be adjusted to obtain better encapsulation and slow release of angiogenic chemokine stromal cell derived factor-1 (SDF-1), which achieved the desired functions of antioxidation and promotion of neovascularization (Zhu et al., 2016). Kim et al. reported that in the absence of biologically active agents, phytochemically stabilized gold nanoparticles increased the content of superoxide dismutase in acute wounds, with an antioxidant effect. They also showed that increasing angiopoietin 1 (ang1) and ang2 expression promoted angiogenesis in in vivo experiments (Kim et al., 2015). However, the effectiveness of these approaches in chronic wounds needs to be verified.
Edaravone, a commercial drug with the ability to scavenge ·OH, is used to treat cerebral ischemia and has also been used in chronic wounds. The therapeutic effect was dose-dependent, which proved the dual role of ROS in chronic wound healing (Fan et al., 2019). Ceria have also attracted attention as potential treatments for excessive-ROS-related diseases owing to their ability to mimic the activity of multiple antioxidant enzymes. miR-26a is an important microRNA that inhibits neovascularization in DFUs, only on the basis of removing excessive ROS, antagomiR-26a can effectively reverse the neovascularization inhibition caused by miR-26a and promote vascular regeneration. Wu et al. combined the use of antagomiR-26a and ceria nanozymes to treat non-healing wounds in diabetes patients. Ceria nanozymes with a diameter of 3 nm were prepared and covalently modified with 25 kDa polyethyleneimine (PEI25K) to produce PCN. PCN-miR was fabricated by electrostatic adsorption with negatively charged antagomiR-26a. The design of this drug delivery system ensured that antagomiR-26a had direct contact with the ceria nanozymes, preventing ROS from destroying its activity, as shown in Figure 2. In vivo experiments showed that this self-protecting hydrogel effectively promoted the repair of non-healing wounds in diabetes (Wu et al., 2019).
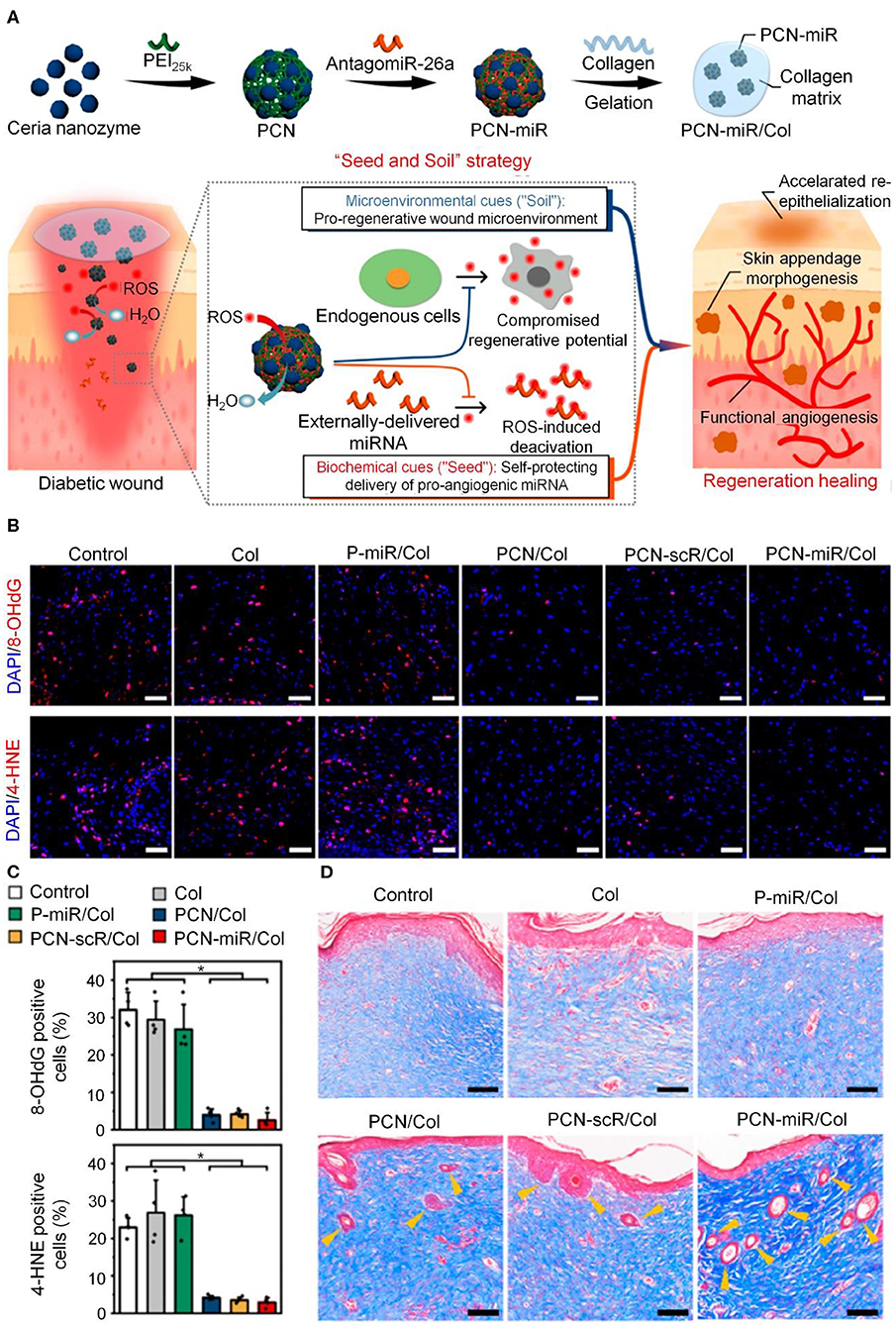
Figure 2. PCN-miR/Col hydrogel exerts antioxidant and neovascularization properties to promote healing of oxidative diabetes wound. (A) Schematic of PCN-miR/Col hydrogel preparation, antioxidation, and vascularization in chronic wounds. (B) In vivo results of ROS by immunofluorescence. (C) Quantitative analysis of results shown in (B). *p < 0.05. (D) Masson staining images from day 28 post-operation. Scale bars, 100 μm. Reproduced with permission from Wu et al. (2019).
Immunoregulation
The immune response is essential to clear pathogens in the early stages of wound healing. Toll-like receptors recognize signals from necrotic tissue and damaged ECM to activate tissue-resident macrophages and recruit other inflammation-related cells, including neutrophils and lymphocytes. Neutrophils produce antibacterial substances and secrete cytokines that promote angiogenesis to promote the recovery of tissues. However, a persistent inflammatory response can lead to the formation of chronic wounds (Percival et al., 2014). Furthermore, it is closely related to the activation type of macrophages in the wound microenvironment. Pro-inflammatory M1 macrophages aggravate tissue damage, whereas anti-inflammatory M2 macrophages are conducive to wound healing (Krzyszczyk et al., 2018).
The polarization of M1 macrophages into M2 macrophages is an effective treatment method that can effectively reduce an excessive inflammatory response (Hesketh et al., 2017; Feng et al., 2019b). Therefore, the development of anti-inflammatory biomaterials based on immunoregulation is a research hotspot. As shown in Figure 3, studies have shown that konjac glucomannan-modified SiO2 nanoparticles (KSiNPs) can induce macrophages to differentiate into M2 phenotypes by inducing the accumulation of nano-clusters of the mannose receptor on the macrophage surface. Without containing any drugs, the material itself has an anti-inflammatory effect and promotes wound healing (Gan et al., 2019).
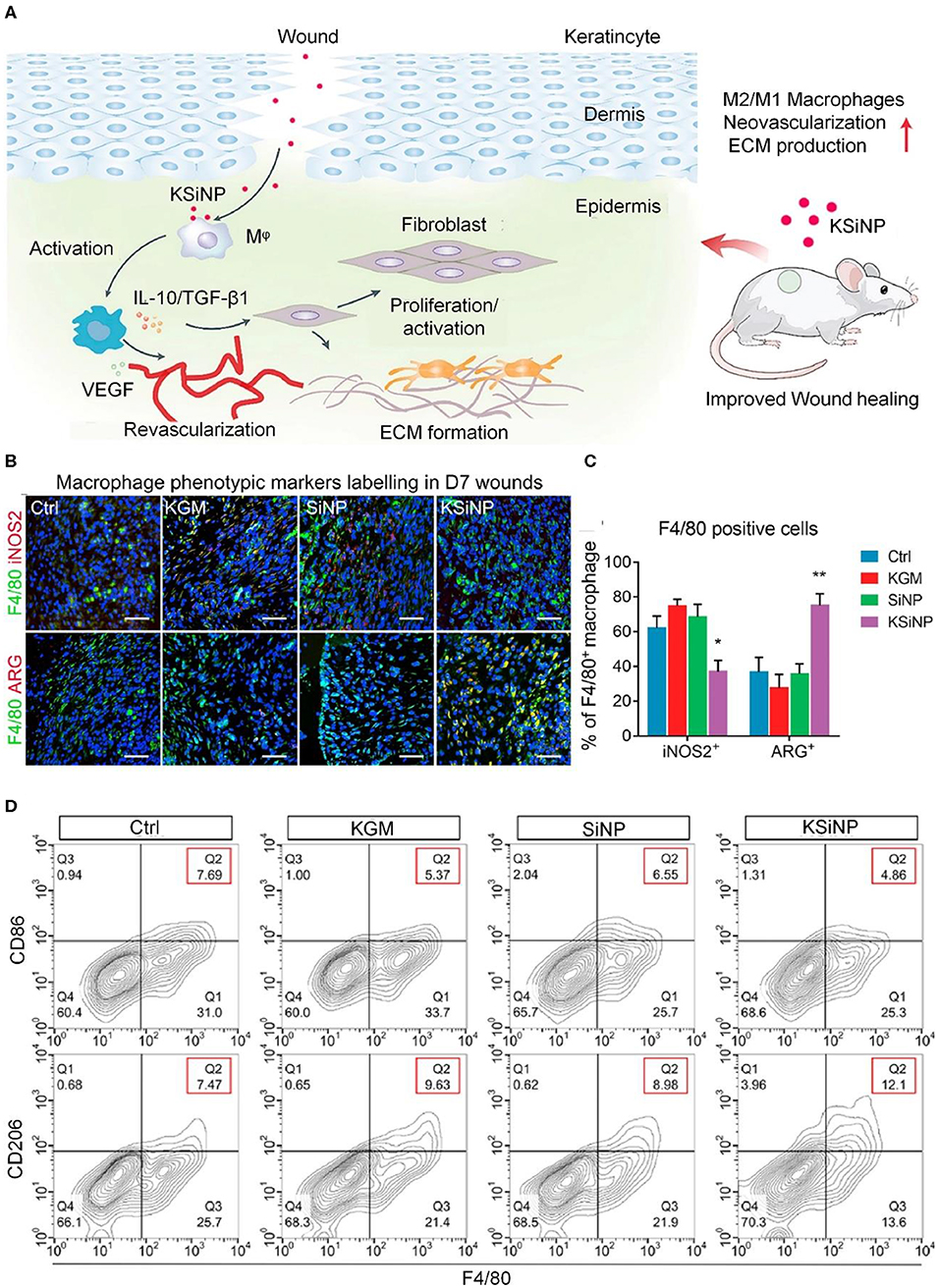
Figure 3. KSiNPs activate macrophages to differentiate into M2 phenotypes and promote wound healing. (A) Schematic of the mechanism by which KSiNPs promote chronic wound healing. (B) Immunofluorescence identification of macrophages in each experimental group 7 days post-operation. Scale bars, 20 μm. (C) Quantitative analysis of the results from (B), showing that the proportion of M2 macrophages in the KSiNP group increased. *P < 0.05, **P < 0.01. (D) Flow cytometry detection of macrophage activation status in different groups of wounds on day 7. Reproduced with permission from Gan et al. (2019).
Proteases and excessive ROS produced by the immune response also hinder wound healing by inactivating growth factors (Sies, 1991; Schafer and Werner, 2008; Westby et al., 2018). Inhibiting the inflammatory response and reducing the cytokines produced during the inflammatory response are of great significance for improving the microenvironment of chronic wounds, and many biomaterial dressings or medicines with anti-inflammatory effects have been used in chronic wounds.
The anti-inflammatory effects of new hydrocellular foam dressings have been verified in acute wound models; they reduced the gene expression levels of inflammation-related factors including IL-1β, IL-6, and IL-10 (Banks et al., 1997; Yamane et al., 2015). Amin et al. compared the anti-inflammatory effect of Chinese medicine bee venom on chronic wounds with that of diclofenac hydrogel. They incorporated bee venom into a poly(vinyl alcohol) (PVA)/CS-based hydrogel using a freezing-thawing method. In vivo experiments showed that this bee venom-loaded PVA/CS hydrogel increased the gene expression levels of hydroxyproline and glutathione and reduced the level of IL-6, and its anti-inflammatory effect was comparable to that of the diclofenac hydrogel (Amin and Abdel-Raheem, 2014).
Angiogenesis
Various conditions, such as hyperglycemia, cause endothelial dysfunction, resulting in insufficient blood supply to a chronic wound and an insufficient supply of nutrients and oxygen around the wound (Sorg et al., 2018). Therefore, reconstruction of the vascular network is essential to accelerate the healing of chronic wounds with local blood supply shortage. Impaired angiogenesis is associated with abnormal migration, proliferation, and regulation of endothelial progenitor cells. Some studies have confirmed that endogenous gas signaling molecules such as nitric oxide and hydrogen sulfide, which promote angiogenesis and granulation tissue formation, are closely related to wound healing (Durante, 2016; Malone-Povolny et al., 2019). In addition, insufficient blood supply will also affect the local skin temperature and thus the wound microenvironment. Acute wounds will increase the temperature of the wound under the mediation of certain factors, thereby causing vasodilation and achieving the purpose of providing more nutrition. Chronic wound blood flow is blocked in vascular disease, which reduces skin temperature and affects wound healing (Wilmore et al., 1977; Fierheller and Sibbald, 2010). At present, many kinds of dressings made using hydrogels, electrospinning, etc. with angiogenic effects have been used alone or in combination with angiogenic bioactive substances for the neovascularization of chronic wounds.
Hyaluronic acid (HA), a non-sulfated glycosaminoglycan, is the main component of ECM. HA promotes angiogenesis through controllable mild inflammation caused by degradation products (Toole and Slomiany, 2008; Gaffney et al., 2010; Hemshekhar et al., 2016). Tokatlian et al. used HA hydrogels with different pore sizes to carry a plasmid containing VEGF plasmids for the treatment of chronic wounds. When applied to a splinted wound in a diabetic mouse model, the hydrogel with pores of 60 μm in diameter had the strongest healing ability, but the combination of VEGF plasmids did not further enhance the regeneration of granulation tissue (Tokatlian et al., 2015). Gelatin, also known as hydrolyzed Col, has also been used as a drug delivery vehicle for chronic wound neovascularization. It has excellent biocompatibility and biodegradability, and low antigenicity (Yeh et al., 2011). Mesenchymal stem cells (MSCs) exert a chemotactic effect through paracrine signaling to promote the regeneration of chronic wounds. In order to verify the therapeutic effect of chemokines that can induce the migration of MSCs on chronic wounds, Yoon et al. developed an IL-8 or macrophage inflammatory protein-3α (MIP-3α)-loaded gelatin-hydroxyphenyl propionic acid (GH) hydrogel. This GH hydrogel achieved the encapsulation of IL-8 and MIP-3α during the in-situ polymerization process and maintained the vitality of chemokines. In vivo experiments using a 1.0 cm diameter round wound in diabetic mice confirmed that IL-8, which is involved in recruiting a variety of cell types, including MSCs and endothelial cells, had a stronger repair-promoting ability than MIP-3α. Further histological and immunohistochemical results indicated that the restoration of regeneration ability was due to the enhancement of neovascularization (Yoon et al., 2016). Col-based hydrogels have the advantages of high water absorption, good biocompatibility, and low antigenicity. Col also enhances granulation tissue and neovascularization by eliminating high concentrations of MMPs (Simpson et al., 2007; Laghezza Masci et al., 2019). Alginate is an anionic polysaccharide that can form hydrogels under very mild conditions and in the absence of organic solvents (Lee and Mooney, 2012). In addition, alginate effectively prevents dehydration and has long been regarded as an excellent biomaterial for use in wound healing dressings. Alginate hydrogel can be used as a drug delivery carrier to solve the problems of low bioavailability and poor stability of large molecules such as proteins and growth factors (Momoh et al., 2015). For instance, calcium alginate hydrogel has served as a drug carrier for protamine with a neovascularization function in the treatment of chronic wounds (Wang T. et al., 2019). A CS based-dressing was also used to deliver small interfering RNAs (siRNAs) with ECM remodeling function. This RNA interference therapy also strongly promoted vascular regeneration of chronic wounds (Castleberry et al., 2016). For more detail, see section “Extracellular Matrix Remodeling” below.
In addition to hydrogels, oriented electrospun fibers dressing can also serve as a delivery system for pro-angiogenic drugs for the treatment of chronic wounds. Dimethyl xalylglycine (DMOG) is a small molecule that promotes angiogenesis. To solve the problem of its rapid inactivation in the body, Ren et al. used nano-mesoporous silicon as an intermediate carrier to prepare a DMOG-loaded poly (L-lactic acid) (PLLA)-oriented electrospun dressing. As shown in Figure 4, this drug-loading system achieved slow release of DMOG and Si ions; the oriented electrospun fibers regulated the arrangement of cells and the signal transmission mechanism of special phenotype expression through physical cues guided by orientation. In vitro tests confirmed that it increased gene expression levels in human umbilical vein endothelial cells, and in vivo experiments showed that it promoted wound healing and neovascularization in diabetic mice (Ren et al., 2018).
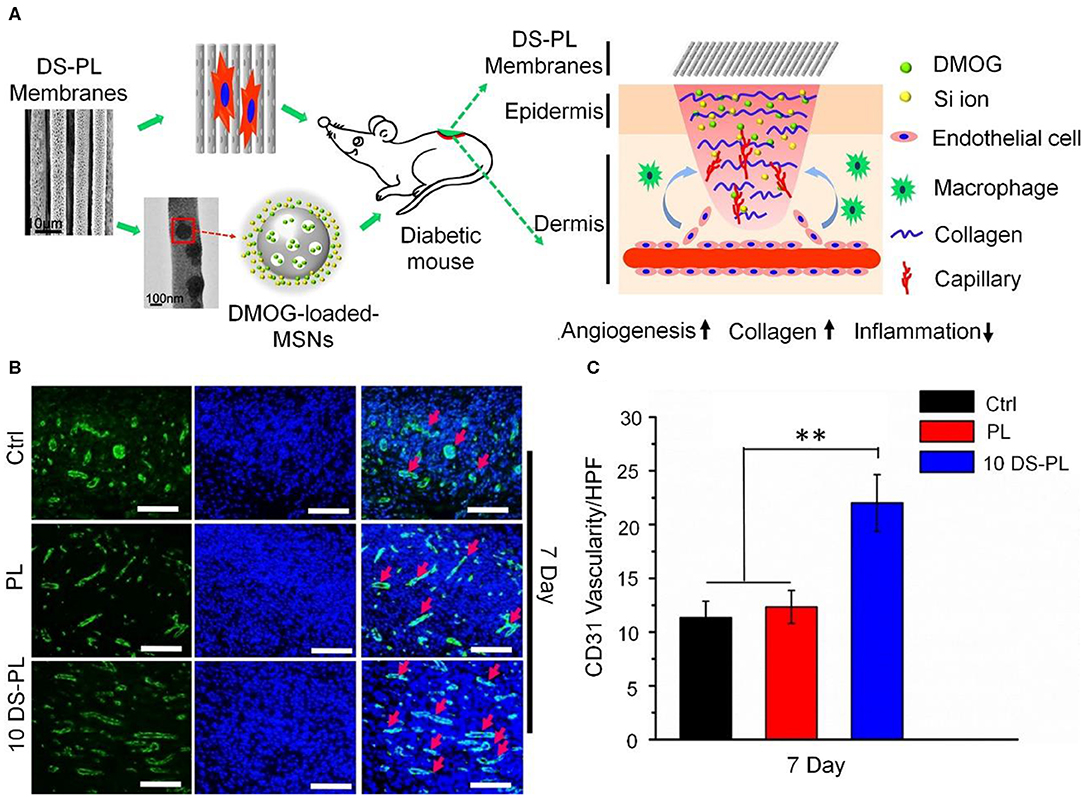
Figure 4. DMOG-loaded PLLA electrospun membrane dressing promotes chronic wound angiogenesis. (A) Schematic of material preparation and angiogenesis-promoting mechanism. (B) Immunofluorescence CD31 staining images of wounds 7 days post-operation. Scale bar, 100 μm. (C) Quantitative analysis of the results shown in (B). **P < 0.01. Reproduced with permission from Li et al. (2018).
Extracellular Matrix Remodeling
The reduction of locally related ECM is an important cause of chronic wound non-healing. Col is the most important component of ECM in skin. Increasing the content and the stability of Col fibers accelerates wound healing. Compared with type III Col, type I Col has better tensile properties. Type III Col is mainly formed in the early stages of wound healing, and the ratio of these two types of Col has a greater impact on the wound healing and the skin quality after healing (Rhett et al., 2008). Mechanistic studies have shown that the reduction in ECM content observed in chronic wounds is related to the overexpression of ECM proteases, such as MMP-9. Decreased ECM results in impaired granulation tissue formation and epithelial formation (Hayden et al., 2011; Castleberry et al., 2016). Therefore, strategies to promote wound healing by optimizing the ECM have gradually attracted attention in recent years.
Caetano et al. confirmed that the polyelectrolyte complex obtained by cross-linking of CS and alginate. The cross-linked polyelectrolyte compound combining the characteristics of CS and alginate reduced the infiltration of neutrophils, promoted the proliferation of fibroblasts, promoted Col formation, and improved the chronic wound microenvironment (Caetano et al., 2015).
An important strategy for ECM remodeling during chronic wound healing is the direct application of Col-based dressings. The components of the dressing can be directly degraded by MMPs, thereby reducing the consumption of Col in the ECM. The 3D structure provided can provide space for vascular regeneration and keratinocyte migration, effectively promoting wound repair (Liden and May, 2013; Bohn et al., 2016).
Local silencing of sequence-specific MMP genes using RNA interference is another important strategy for regulating MMP, which avoids the disadvantages of using MMP inhibitors such as low efficiency and side effects on skeletal muscle (Overall and Kleifeld, 2006). Castleberry et al. used layer-by-layer (LbL) self-assembly technology to fabricate two coatings on commercial nylon bandages to deliver an MMP-9-specific siRNA (siMMP-9). As shown in Figure 5, the first layer of degradable coating material was poly(β-amino ester) 2 (Poly 2)/dextran sulfate (DS); the second coating was CS with siMMP-9. The drug release properties and silencing of MMP-9 were optimized by adjusting the number of layers of coatings. In vivo experiments confirmed that this siMMP-9-loaded (Poly 2/DS)/CS film had a good ability to promote wound healing in diabetic mice and caused a steady increase in Col content. These results demonstrate the therapeutic potential of silencing MMP-9 gene expression in chronic wounds (Castleberry et al., 2016).
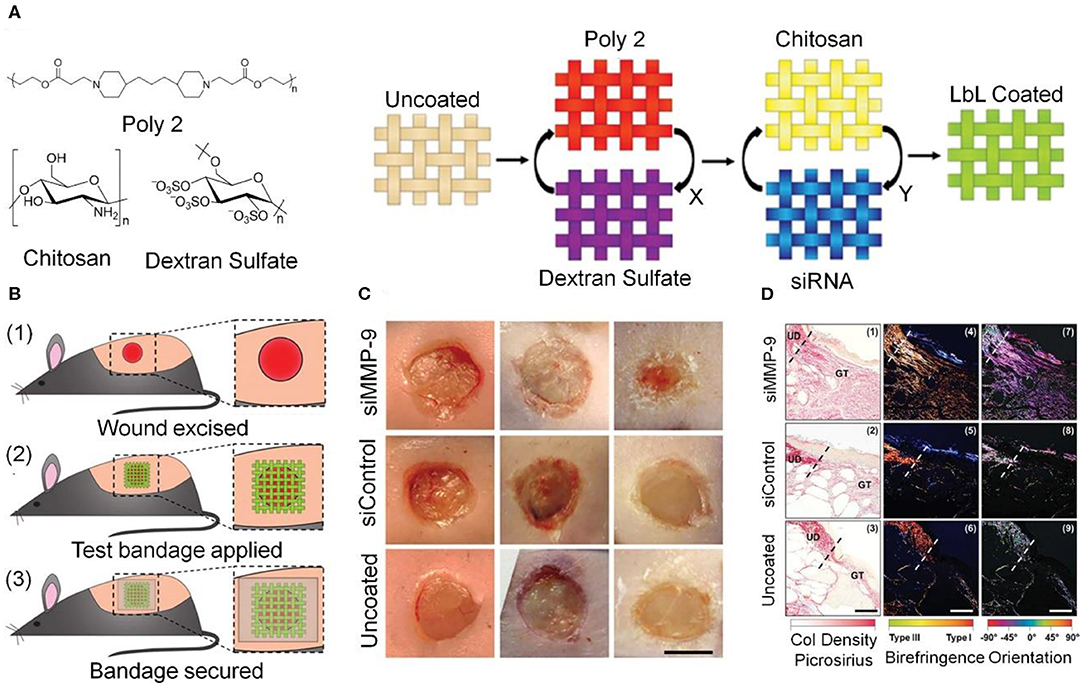
Figure 5. Silencing the MMP-9 gene accelerates the healing of chronic wounds through ECM remodeling. (A) Chemical structures of the materials used in the dressing, and schematic diagram of the double coatings fabricated by LbL self-assembly; X and Y represent the number of layers of Poly 2/DS and CS/siRNA, respectively. (B) Schematic of the in vivo experiments. (C) Post-operative photographs of wounds areas. Scale bar, 5 mm. (D) Sirius Red staining images of Col tissue infiltration and fusion with wound edges. UD, undamaged dermis. GT, granulation tissue. Scale bars, 75 μm. Reproduced with permission from Castleberry et al. (2016).
Others
In addition to the above commonly used functional dressings, other dressings, such as those used for diabetic foot neuropathy and scars, also need attention.
Diabetic neuropathy-related ulcers often occur on the plantar surface, and local ulcers often occur on parts of the foot that are under long-term pressure. The main clinical treatment is to use pressure-reducing shoes (Zimny et al., 2003; Clements et al., 2017; Feldman et al., 2017). Zimny et al. used a commercial felted foam dressing to treat patients with neuropathic DFUs. This felted foam dressing contained a thicker foam layer to relieve pressure on the sole of the foot and a thin sticky layer. In their retrospective study, patients using the felted foam dressing achieved the same treatment results as those using traditional clinical therapies (Zimny et al., 2003).
The formation of chronic wound scars often presents a trend of increasing within a year after the wounds healing. Scar formation often leads to repeated ulceration, infection, and even cancer of the wounds. Some researchers have added epidermal growth factor and silver sulfadiazine to silk protein biomaterials to make dressings. The material encapsulates the drug in three forms: a silk membrane, a layered porous silk membrane, and an electrospun nanofiber. It promotes the proliferation of dermal cells and the synthesis of Col, and reduces the formation of scars, thereby providing a feasible strategy for the treatment of chronic wounds (Gil et al., 2013).
Conclusions and Prospects
In the past two decades, functional biomaterials that can change the wound microenvironment, such as the continuous inflammation, have had a profound impact on the development of dressings for the treatment of chronic wounds. However, many basic studies have been carried out in rodent models, which are characterized by a predominance of M2 macrophages and tend to contracture healing. Thus, the results of these studies need to be further verified using large mammalian models. Current commercial dressings do not meet the needs of patients with chronic wounds, and cost-effective alternative designs containing various types of materials should be developed. Given the clinical problems of patients with chronic wounds, an important research direction is the development of diagnostic functional dressings that clarify the condition of the wound, especially the early symptoms of the subcutaneous tissues with temporary intact skin. As the skin healing process represents the repair process of most tissues, it is widely used in orthopedics and nerve repair technologies, such as 3D printing, and is also suitable for chronic wounds. Therefore, according to the actual characteristics of the patient's wound, such as the position, size, depth, and surrounding tissue of the wound, a more diverse and personalized functional dressing can be produced using computer-aided design. In short, as the application of functionalized biomaterials in chronic wounds is further developed, their therapeutic effects on chronic wounds will be greatly improved, and these materials will replace more clinically invasive operations.
Author Contributions
XZ wrote the manuscript. XZ, WS, QY, and WQ revised the manuscript. YW and RL designed this work of review and revised the manuscript.
Funding
This work was financially supported by the Scientific Research Planning Project of the Education Department of Jilin Province (Grant No. JJKH20201075KJ), the National Natural Science Foundation of China (Grant No. 81901365), and the Jilin Science and Technology Agency funds in China (Grant Nos. 20180101118JC and 20190103076JH).
Conflict of Interest
The authors declare that the research was conducted in the absence of any commercial or financial relationships that could be construed as a potential conflict of interest.
References
Agostinho, A. M., Hartman, A., Lipp, C., Parker, A. E., Stewart, P. S., and James, G. A. (2011). An in vitro model for the growth and analysis of chronic wound MRSA biofilms. J. Appl. Microbiol. 111, 1275–1282. doi: 10.1111/j.1365-2672.2011.05138.x
Amin, M. A., and Abdel-Raheem, I. T. (2014). Accelerated wound healing and anti-inflammatory effects of physically cross linked polyvinyl alcohol-chitosan hydrogel containing honey bee venom in diabetic rats. Arch. Pharm. Res. 37, 1016–1031. doi: 10.1007/s12272-013-0308-y
Archana, D., Singh, B. K., Dutta, J., and Dutta, P. K. (2013). In vivo evaluation of chitosan-PVP-titanium dioxide nanocomposite as wound dressing material. Carbohydr. Polym. 95, 530–539. doi: 10.1016/j.carbpol.2013.03.034
Arenbergerova, M., Arenberger, P., Bednar, M., Kubat, P., and Mosinger, J. (2012). Light-activated nanofibre textiles exert antibacterial effects in the setting of chronic wound healing. Exp. Dermatol. 21, 619–624. doi: 10.1111/j.1600-0625.2012.01536.x
Banks, V., Bale, S., Harding, K., and Harding, E. F. (1997). Evaluation of a new polyurethane foam dressing. J. Wound Care 6, 266–269. doi: 10.12968/jowc.1997.6.6.266
Bohn, G., Liden, B., Schultz, G., Yang, Q., and Gibson, D. J. (2016). Ovine-based collagen matrix dressing: next-generation collagen dressing for wound care. Adv. Wound Care 5, 1016–1031. doi: 10.1089/wound.2015.0660
Boulton, A. J., Vileikyte, L., Ragnarson-Tennvall, G., and Apelqvist, J. (2005). The global burden of diabetic foot disease. Lancet 366, 1719–1724. doi: 10.1016/S0140-6736(05)67698-2
Caetano, G. F., Frade, M. A., Andrade, T. A., Leite, M. N., Bueno, C. Z., Moraes, A. M., et al. (2015). Chitosan-alginate membranes accelerate wound healing. J. Biomed. Mater. Res. B Appl. Biomater. 103, 1013–1022. doi: 10.1002/jbm.b.33277
Castleberry, S. A., Almquist, B. D., Li, W., Reis, T., Chow, J., Mayner, S., et al. (2016). Self-assembled wound dressings silence MMP-9 and improve diabetic wound healing in vivo. Adv. Mater. 28, 1809–1817. doi: 10.1002/adma.201503565
Cho, N. H., Shaw, J. E., Karuranga, S., Huang, Y., Da Rocha Fernandes, J. D., Ohlrogge, A. W., et al. (2018). IDF diabetes atlas: global estimates of diabetes prevalence for 2017 and projections for 2045. Diabetes Res. Clin. Pract. 138, 271–281. doi: 10.1016/j.diabres.2018.02.023
Clements, M. P., Byrne, E., Guerrero, L. F. C., Cattin, A. L., Zakka, L., Ashraf, A., et al. (2017). The wound microenvironment reprograms schwann cells to invasive mesenchymal-like cells to drive peripheral nerve regeneration. Neuron 96, 98–114.e7. doi: 10.1016/j.neuron.2017.09.008
Ding, J., Zhang, J., Li, J., Li, D., Xiao, C., Xiao, H., et al. (2019). Electrospun polymer biomaterials. Prog. Polym. Sci. 90, 1–34. doi: 10.1016/j.progpolymsci.2019.01.002
Ding, Y., Sun, Z., Shi, R., Cui, H., Liu, Y., Mao, H., et al. (2019). Integrated endotoxin adsorption and antibacterial properties of cationic polyurethane foams for wound healing. ACS Appl. Mater. Interfaces 11, 2860–2869. doi: 10.1021/acsami.8b19746
Durante, W. (2016). Hydrogen sulfide therapy in diabetes-accelerated atherosclerosis: a whiff of success. Diabetes 65, 2832–2834. doi: 10.2337/dbi16-0042
Eaglstein, W. H. (2001). Moist wound healing with occlusive dressings: a clinical focus. Dermatol. Surg. 27, 175–181. doi: 10.1097/00042728-200102000-00016
Falanga, V. (2004). The chronic wound: impaired healing and solutions in the context of wound bed preparation. Blood Cells Mol. Dis. 32, 88–94. doi: 10.1016/j.bcmd.2003.09.020
Fan, Y., Wu, W., Lei, Y., Gaucher, C., Pei, S., Zhang, J., et al. (2019). Edaravone-loaded alginate-based nanocomposite hydrogel accelerated chronic wound healing in diabetic mice. Mar. Drugs 17:258. doi: 10.3390/md17050285
Feldman, E. L., Nave, K. A., Jensen, T. S., and Bennett, D. L. H. (2017). New horizons in diabetic neuropathy: mechanisms, bioenergetics, and pain. Neuron 93, 1296–1313. doi: 10.1016/j.neuron.2017.02.005
Feng, X., Li, J., Zhang, X., Liu, T., Ding, J., and Chen, X. (2019a). Electrospun polymer micro/nanofibers as pharmaceutical repositories for healthcare. J. Controlled Release 302, 19–41. doi: 10.1016/j.jconrel.2019.03.020
Feng, X., Xu, W., Li, Z., Song, W., Ding, J., and Chen, X. (2019b). Immunomodulatory nanosystems. Adv. Sci. 6:1900101. doi: 10.1002/advs.201900101
Field, C. K., and Kerstein, M. (1994). Overview of wound healing in a moist environment. Am. J. Surg. 167, S2–S6. doi: 10.1016/0002-9610(94)90002-7
Fierheller, M., and Sibbald, G. (2010). A clinical investigation into the relationship between increased periwound skin temperature and local wound infection in patients with chronic leg ulcers. Adv. Skin Wound Care 23, 369–378. doi: 10.1097/01.ASW.0000383197.28192.98
Gaffney, J., Matou-Nasri, S., Grau-Olivares, M., and Slevin, M. (2010). Therapeutic applications of hyaluronan. Mol. BioSyst. 6, 437–443. doi: 10.1039/B910552M
Gan, J., Liu, C., Li, H., Wang, S., Wang, Z., Kang, Z., et al. (2019). Accelerated wound healing in diabetes by reprogramming the macrophages with particle-induced clustering of the mannose receptors. Biomaterials 219:119340. doi: 10.1016/j.biomaterials.2019.119340
Gil, E. S., Panilaitis, B., Bellas, E., and Kaplan, D. L. (2013). Functionalized silk biomaterials for wound healing. Adv. Healthcare Mater. 2, 206–217. doi: 10.1002/adhm.201200192
Gjdsbl, K., Christensen, J. J., Karlsmark, T., Bo, J., and Krogfelt, K. A. (2006). Multiple bacterial species reside in chronic wounds: a longitudinal study. Int. Wound J. 3, 225–231. doi: 10.1111/j.1742-481X.2006.00159.x
Gonzalez, A. C., Costa, T. F., Andrade, Z. A., and Medrado, A. R. (2016). Wound healing-A literature review. An. Bras. Dermatol. 91, 614–620. doi: 10.1590/abd1806-4841.20164741
Gurtner, G. C., Werner, S., Barrandon, Y., and Longaker, M. T. (2008). Wound repair and regeneration. Nature 453, 314–321. doi: 10.1038/nature07039
Harding, K. (2015). Innovation and wound healing. J. Wound Care 24, 7–13. doi: 10.12968/jowc.2015.24.Sup4b.7
Hayden, D. M., Forsyth, C., and Keshavarzian, A. (2011). The role of matrix metalloproteinases in intestinal epithelial wound healing during normal and inflammatory states. J. Surg. Res. 168, 315–324. doi: 10.1016/j.jss.2010.03.002
He, H., Xia, D. L., Chen, Y. P., Li, X. D., Chen, C., Wang, Y. F., et al. (2017). Evaluation of a two-stage antibacterial hydrogel dressing for healing in an infected diabetic wound. J. Biomed. Mater. Res. B. Appl. Biomater. 105, 1808–1817. doi: 10.1002/jbm.b.33543
Hemshekhar, M., Thushara, R. M., Chandranayaka, S., Sherman, L. S., Kemparaju, K., and Girish, K. S. (2016). Emerging roles of hyaluronic acid bioscaffolds in tissue engineering and regenerative medicine. Int. J. Biol. Macromol. 86, 917–928. doi: 10.1016/j.ijbiomac.2016.02.032
Hesketh, M., Sahin, K. B., West, Z. E., and Murray, R. Z. (2017). Macrophage phenotypes regulate scar formation and chronic wound healing. Int. J. Mol. Sci. 18:1545. doi: 10.3390/ijms18071545
Humbert, P., Faivre, B., Veran, Y., Debure, C., Truchetet, F., Becherel, P. A., et al. (2014). Protease-modulating polyacrylate-based hydrogel stimulates wound bed preparation in venous leg ulcers - a randomized controlled trial. J. Eur. Acad. Dermatol. Venereol. 28, 1742–1750. doi: 10.1111/jdv.12400
Jones, R. E., Foster, D. S., and Longaker, M. T. (2018). Management of chronic wounds-2018. JAMA 320, 1481–1482. doi: 10.1001/jama.2018.12426
Kim, J. E., Lee, J., Jang, M., Kwak, M. H., Go, J., Kho, E. K., et al. (2015). Accelerated healing of cutaneous wounds using phytochemically stabilized gold nanoparticle deposited hydrocolloid membranes. Biomater. Sci. 3, 509–519. doi: 10.1039/C4BM00390J
Kruse, C. R., Nuutila, K., Lee, C. C. Y., Kiwanuka, E., Singh, M., Caterson, E. J., et al. (2015). The external microenvironment of healing skin wounds. Wound Repair Regen. 23, 456–464. doi: 10.1111/wrr.12303
Krzyszczyk, P., Schloss, R., Palmer, A., and Berthiaume, F. (2018). The role of macrophages in acute and chronic wound healing and interventions to promote pro-wound healing phenotypes. Front. Physiol. 9:419. doi: 10.3389/fphys.2018.00419
Laghezza Masci, V., Taddei, A. R., Courant, T., Tezgel, O., Navarro, F., Giorgi, F., et al. (2019). Characterization of collagen/lipid nanoparticle-curcumin cryostructurates for wound healing applications. Macromol. Biosci. 19:e1800446. doi: 10.1002/mabi.201800446
Lara, H. H., Garza-Trevino, E. N., Ixtepan-Turrent, L., and Singh, D. K. (2011). Silver nanoparticles are broad-spectrum bactericidal and virucidal compounds. J. Nanobiotechnol. 9:30. doi: 10.1186/1477-3155-9-30
Lee, K. Y., and Mooney, D. J. (2012). Alginate: properties and biomedical applications. Prog. Polym. Sci. 37, 106–126. doi: 10.1016/j.progpolymsci.2011.06.003
Li, Y. S., Wang, X., Fu, Y. N., Wei, Y., Zhao, L. Y., and Tao, L. (2018). Self-adapting hydrogel to improve the therapeutic effect in wound-healing. ACS Appl. Mater. Interfaces 10, 26046–26055. doi: 10.1021/acsami.8b08874
Liau, S. Y., Read, D. C., Pugh, W. J., Furr, J. R., and Russell, A. D. (1997). Interaction of silver nitrate with readily identifiable groups: relationship to the antibacterial action of silver ions. Lett. Appl. Microbiol. 25, 279–283. doi: 10.1046/j.1472-765X.1997.00219.x
Liden, B. A., and May, B. C. H. (2013). Clinical outcomes following the use of ovine forestomach matrix (endoform dermal template) to treat chronic wounds. Adv. Skin Wound Care 26, 164–167. doi: 10.1097/01.ASW.0000428862.34294.d4
Lin, B., Zhao, L., Wang, H., Zhu, H., Gai, G., Wang, L., et al. (2020). Progress in bioadhesive hydrogels. J. Funct. Polym. 33, 125–140. doi: 10.14133/j.cnki.1008-9357.20190711001
López-Iglesias, C., Barros, J., Ardao, I., Monteiro, F. J., Alvarez-Lorenzo, C., Gómez-Amoza, J. L., et al. (2019). Vancomycin-loaded chitosan aerogel particles for chronic wound applications. Carbohydr. Polym. 204, 223–231. doi: 10.1016/j.carbpol.2018.10.012
Luo, J. D., Wang, Y. Y., Fu, W. L., Wu, J., and Chen, A. F. (2004). Gene therapy of endothelial nitric oxide synthase and manganese superoxide dismutase restores delayed wound healing in type 1 diabetic mice. Circulation 110, 2484–2493. doi: 10.1161/01.CIR.0000137969.87365.05
Malone-Povolny, M. J., Maloney, S. E., and Schoenfisch, M. H. (2019). Nitric oxide therapy for diabetic wound healing. Adv. Healthcare Mater. 8:e1801210. doi: 10.1002/adhm.201801210
Masood, N., Ahmed, R., Tariq, M., Ahmed, Z., Masoud, M. S., Ali, I., et al. (2019). Silver nanoparticle impregnated chitosan-PEG hydrogel enhances wound healing in diabetes induced rabbits. Int. J. Pharm. 559, 23–36. doi: 10.1016/j.ijpharm.2019.01.019
Mathes, S. H., Ruffner, H., and Graf-Hausner, U. (2014). The use of skin models in drug development. Adv. Drug Delivery Rev. 69-70, 81–102. doi: 10.1016/j.addr.2013.12.006
Momoh, F. U., Boateng, J. S., Richardson, S. C., Chowdhry, B. Z., and Mitchell, J. C. (2015). Development and functional characterization of alginate dressing as potential protein delivery system for wound healing. Int. J. Biol. Macromol. 81, 137–150. doi: 10.1016/j.ijbiomac.2015.07.037
Morton, L. M., and Phillips, T. J. (2016). Wound healing and treating wounds: differential diagnosis and evaluation of chronic wounds. J. Am. Acad. Dermatol. 74, 589–605. doi: 10.1016/j.jaad.2015.08.068
Nunan, R., Harding, K. G., and Martin, P. (2014). Clinical challenges of chronic wounds: searching for an optimal animal model to recapitulate their complexity. Dis. Models Mech. 7, 1205–1213. doi: 10.1242/dmm.016782
O'reilly, D., Linden, R., Fedorko, L., Tarride, J. E., Jones, W. G., Bowen, J. M., et al. (2011). A prospective, double-blind, randomized, controlled clinical trial comparing standard wound care with adjunctive hyperbaric oxygen therapy (HBOT) to standard wound care only for the treatment of chronic, non-healing ulcers of the lower limb in patients with diabetes mellitus: a study protocol. Trials 12:69. doi: 10.1186/1745-6215-12-69
Overall, C. M., and Kleifeld, O. (2006). Towards third generation matrix metalloproteinase inhibitors for cancer therapy. Br. J. Cancer 94, 941–946. doi: 10.1038/sj.bjc.6603043
Percival, S. L., Mccarty, S., Hunt, J. A., and Woods, E. J. (2014). The effects of pH on wound healing, biofilms, and antimicrobial efficacy. Wound Repair Regen. 22, 174–186. doi: 10.1111/wrr.12125
Pinho, E., Henriques, M., and Soares, G. (2014). Cyclodextrin/cellulose hydrogel with gallic acid to prevent wound infection. Cellulose 21, 4519–4530. doi: 10.1007/s10570-014-0439-4
Powers, J. G., Higham, C., Broussard, K., and Phillips, T. J. (2016). Wound healing and treating wounds: chronic wound care and management. J. Am. Acad. Dermatol. 74, 607–625. doi: 10.1016/j.jaad.2015.08.070
Qiao, Y., Ping, Y., Zhang, H., Zhou, B., Liu, F., Yu, Y., et al. (2019). Laser-activatable CuS nanodots to treat multidrug-resistant bacteria and release copper ion to accelerate healing of infected chronic nonhealing wounds. ACS Appl. Mater. Interfaces 11, 3809–3822. doi: 10.1021/acsami.8b21766
Ranjbar-Mohammadi, M., Rabbani, S., Bahrami, S. H., Joghataei, M. T., and Moayer, F. (2016). Antibacterial performance and in vivo diabetic wound healing of curcumin loaded gum tragacanth/poly(epsilon-caprolactone) electrospun nanofibers. Mater. Sci. Eng. C 69, 1183–1191. doi: 10.1016/j.msec.2016.08.032
Reinke, J. M., and Sorg, H. (2012). Wound repair and regeneration. Eur. Surg. Res. 49, 35–43. doi: 10.1159/000339613
Ren, X., Han, Y., Wang, J., Jiang, Y., Yi, Z., Xu, H., et al. (2018). An aligned porous electrospun fibrous membrane with controlled drug delivery - an efficient strategy to accelerate diabetic wound healing with improved angiogenesis. Acta Biomater. 70, 140–153. doi: 10.1016/j.actbio.2018.02.010
Rhett, J. M., Ghatnekar, G. S., Palatinus, J. A., O'quinn, M., Yost, M. J., and Gourdie, R. G. (2008). Novel therapies for scar reduction and regenerative healing of skin wounds. Trends Biotechnol. 26, 173–180. doi: 10.1016/j.tibtech.2007.12.007
Schafer, M., and Werner, S. (2008). Oxidative stress in normal and impaired wound repair. Pharmacol. Res. 58, 165–171. doi: 10.1016/j.phrs.2008.06.004
Sen, C. K., Gordillo, G. M., Roy, S., Kirsner, R., Lambert, L., Hunt, T. K., et al. (2009). Human skin wounds: a major and snowballing threat to public health and the economy. Wound Repair Regen. 17, 763–771. doi: 10.1111/j.1524-475X.2009.00543.x
Sen, C. K., Khanna, S., Babior, B. M., Hunt, T. K., Ellison, E. C., and Roy, S. (2002). Oxidant-induced vascular endothelial growth factor expression in human keratinocytes and cutaneous wound healing. J. Biol. Chem. 277, 33284–33290. doi: 10.1074/jbc.M203391200
Serena, T., Connell, H., Mcconnell, S., Patel, K., Doner, B., Sabo, M., et al. (2016). Novel multivalent wound-healing ointment provides bioburden control and moisture management: a retrospective registry data analysis. Adv. Skin Wound Care 29, 461–468. doi: 10.1097/01.ASW.0000490193.96840.9e
Sies, H. (1991). Oxidative stress: from basic research to clinical application. Am. J. Med. 91, 31S−38S. doi: 10.1016/0002-9343(91)90281-2
Simpson, D., Liu, H., Fan, T. H., Nerem, R., and Dudley, S. C. Jr. (2007). A tissue engineering approach to progenitor cell delivery results in significant cell engraftment and improved myocardial remodeling. Stem Cells 25, 2350–2357. doi: 10.1634/stemcells.2007-0132
Singh, A., Halder, S., Chumber, S., Misra, M. C., Sharma, L. K., Srivastava, A., et al. (2004). Meta-analysis of randomized controlled trials on hydrocolloid occlusive dressing versus conventional gauze dressing in the healing of chronic wounds. Asian J. Surg. 27, 326–332. doi: 10.1016/S1015-9584(09)60061-0
Snippert, H. J., Haegebarth, A., Kasper, M., Jaks, V., Van Es, J. H., Barker, N., et al. (2010). Lgr6 marks stem cells in the hair follicle that generate all cell lineages of the skin. Science 327, 1385–1389. doi: 10.1126/science.1184733
Sorg, H., Tilkorn, D. J., Mirastschijski, U., Hauser, J., and Kraemer, R. (2018). Panta rhei: neovascularization, angiogenesis and nutritive perfusion in wound healing. Eur. Surg. Res. 59, 232–241. doi: 10.1159/000492410
Speidel, A. T., Stuckey, D. J., Chow, L. W., Jackson, L. H., Noseda, M., Abreu Paiva, M., et al. (2017). Multimodal hydrogel-based platform to deliver and monitor cardiac progenitor/stem cell engraftment. ACS Cent. Sci. 3, 338–348. doi: 10.1021/acscentsci.7b00039
Suryaletha, K., John, J., Radhakrishnan, M. P., George, S., and Thomas, S. (2018). Metataxonomic approach to decipher the polymicrobial burden in diabetic foot ulcer and its biofilm mode of infection. Int. Wound J. 15, 473–481. doi: 10.1111/iwj.12888
Tokatlian, T., Cam, C., and Segura, T. (2015). Porous hyaluronic acid hydrogels for localized nonviral DNA delivery in a diabetic wound healing model. Adv. Healthcare Mater. 4, 1084–1091. doi: 10.1002/adhm.201400783
Toole, B. P., and Slomiany, M. G. (2008). Hyaluronan: a constitutive regulator of chemoresistance and malignancy in cancer cells. Semin. Cancer Biol. 18, 244–250. doi: 10.1016/j.semcancer.2008.03.009
Topaz, M. (2012). Improved wound management by regulated negative pressure-assisted wound therapy and regulated, oxygen- enriched negative pressure-assisted wound therapy through basic science research and clinical assessment. Indian J. Plast. Surg. 45, 291–301. doi: 10.4103/0970-0358.101301
Wang, B. L., Liu, X. S., Ji, Y., Ren, K. F., and Ji, J. (2012). Fast and long-acting antibacterial properties of chitosan-Ag/polyvinylpyrrolidone nanocomposite films. Carbohydr. Polym. 90, 8–15. doi: 10.1016/j.carbpol.2012.03.080
Wang, T., Zheng, Y., Shi, Y. J., and Zhao, L. (2019). pH-responsive calcium alginate hydrogel laden with protamine nanoparticles and hyaluronan oligosaccharide promotes diabetic wound healing by enhancing angiogenesis and antibacterial activity. Drug Delivery Transl. Res. 9, 227–239. doi: 10.1007/s13346-018-00609-8
Wang, Y., Jiang, Z., Xu, W., Yang, Y., Zhuang, X., Ding, J., et al. (2019). Chiral polypeptide thermogels induce controlled inflammatory response as potential immunoadjuvants. ACS Appl. Mater. Interfaces 11, 8725–8730. doi: 10.1021/acsami.9b01872
Weller, C. D., Gardiner, E. E., Arthur, J. F., Southey, M., and Andrews, R. K. (2019). Autologous platelet-rich plasma for healing chronic venous leg ulcers: clinical efficacy and potential mechanisms. Int. Wound J. 16, 788–792. doi: 10.1111/iwj.13098
Westby, M. J., Dumville, J. C., Stubbs, N., Norman, G., Wong, J. K. F., Cullum, N., et al. (2018). Protease activity as a prognostic factor for wound healing in venous leg ulcers. Cochrane Database Syst. Rev. 9:CD012841. doi: 10.1002/14651858.CD012841.pub2
Williams, C. (1997). The role of sterigel hydrogel wound dressing in wound debridement. Br. J. Nurs. 6, 494–496. doi: 10.12968/bjon.1997.6.9.494
Wilmore, D. W., Aulick, L. H., Mason, A. D., and Pruitt, B. A. Jr. (1977). Influence of the burn wound on local and systemic responses to injury. Ann. Surg. 186, 444–458. doi: 10.1097/00000658-197710000-00006
Wu, H. B., Li, F. Y., Shao, W., Gao, J. Q., and Ling, D. S. (2019). Promoting angiogenesis in oxidative diabetic wound microenvironment using a nanozyme-reinforced self-protecting hydrogel. ACS Cent. Sci. 5, 477–485. doi: 10.1021/acscentsci.8b00850
Yamane, T., Nakagami, G., Yoshino, S., Shimura, M., Kitamura, A., Kobayashi-Hattori, K., et al. (2015). Hydrocellular foam dressings promote wound healing associated with decrease in inflammation in rat periwound skin and granulation tissue, compared with hydrocolloid dressings. Biosci. Biotechnol. Biochem. 79, 185–189. doi: 10.1080/09168451.2014.968088
Yeh, M. K., Liang, Y. M., Cheng, K. M., Dai, N. T., Liu, C. C., and Young, J. J. (2011). A novel cell support membrane for skin tissue engineering: gelatin film cross-linked with 2-chloro-1-methylpyridinium iodide. Polymer 52, 996–1003. doi: 10.1016/j.polymer.2010.10.060
Yoon, D. S., Lee, Y., Ryu, H. A., Jang, Y., Lee, K. M., Choi, Y., et al. (2016). Cell recruiting chemokine-loaded sprayable gelatin hydrogel dressings for diabetic wound healing. Acta Biomater. 38, 59–68. doi: 10.1016/j.actbio.2016.04.030
Zhang, Y., Ding, J., Qi, B., Tao, W., Wang, J., Zhao, C., et al. (2019a). Multifunctional fibers to shape future biomedical devices. Adv. Funct. Mater. 29:1902834. doi: 10.1002/adfm.201902834
Zhang, Y., Liu, X., Zeng, L., Zhang, J., Zuo, J., Zou, J., et al. (2019b). Polymer fiber scaffolds for bone and cartilage tissue engineering. Adv. Funct. Mater. 29:1903279. doi: 10.1002/adfm.201903279
Zhang, Y., Yu, J., Ren, K., Zuo, J., Ding, J., and Chen, X. (2019c). Thermosensitive hydrogels as scaffolds for cartilage tissue engineering. Biomacromolecules 20, 1478–1492. doi: 10.1021/acs.biomac.9b00043
Zhao, R. L., Liang, H. L. N., Clarke, E., Jackson, C., and Xue, M. L. (2016). Inflammation in chronic wounds. Int. J. Mol. Sci. 17:2085. doi: 10.3390/ijms17122085
Zhu, Y. X., Hoshi, R., Chen, S. Y., Yi, J., Duan, C. W., Galiano, R. D., et al. (2016). Sustained release of stromal cell derived factor-1 from an antioxidant thermoresponsive hydrogel enhances dermal wound healing in diabetes. J. Controlled Release 238, 114–122. doi: 10.1016/j.jconrel.2016.07.043
Keywords: chronic wound, functional biomaterials, immunoregulation, antioxidant, angiogenesis
Citation: Zhang X, Shu W, Yu Q, Qu W, Wang Y and Li R (2020) Functional Biomaterials for Treatment of Chronic Wound. Front. Bioeng. Biotechnol. 8:516. doi: 10.3389/fbioe.2020.00516
Received: 08 April 2020; Accepted: 01 May 2020;
Published: 03 June 2020.
Edited by:
Mingqiang Li, Sun Yat-sen University, ChinaReviewed by:
Jinshan Guo, Southern Medical University, ChinaJunchao Wei, Nanchang University, China
Chao Zhao, University of Alabama, United States
Qingqing Xiong, Tianjin Medical University Cancer Institute and Hospital, China
Copyright © 2020 Zhang, Shu, Yu, Qu, Wang and Li. This is an open-access article distributed under the terms of the Creative Commons Attribution License (CC BY). The use, distribution or reproduction in other forums is permitted, provided the original author(s) and the copyright owner(s) are credited and that the original publication in this journal is cited, in accordance with accepted academic practice. No use, distribution or reproduction is permitted which does not comply with these terms.
*Correspondence: Wenrui Qu, quwenrui@jlu.edu.cn; Yinan Wang, wyn112001@jlu.edu.cn; Rui Li, l_rui@jlu.edu.cn