- 1Weed Research Laboratory, Nanjing Agricultural University, Nanjing, China
- 2Key Laboratory for Organic Electronics and Information Displays & Jiangsu Key Laboratory for Biosensors, Institute of Advanced Materials, National Jiangsu Synergetic Innovation Center for Advanced Materials, Nanjing University of Posts and Telecommunications, Nanjing, China
In recent years, tremendous efforts have been devoted into the fields of valuable diagnosis and anticancer treatment, such as real-time imaging, photothermal, and photodynamic therapy, and drug delivery. As promising nanocarriers, gold nanomaterials have attracted widespread attention during the last two decades for cancer diagnosis and therapy due to their prominent properties. With the development of nanoscience and nanotechnology, the fascinating bio-applications of functionalized gold nanomaterials have been gradually developed from in vitro to in vivo. This mini-review emphasizes some recent advances of photothermal imaging (PTI), surface-enhanced Raman scattering (SERS) imaging, and photoacoustic imaging (PAI)-guided based on gold nanomaterials in vivo therapy in near infrared region (>800 nm). We focus on the fundamental strategies, characteristics of bio-imaging modalities involving the advantages of multiples imaging modalities for cancer treatment, and then highlight a few examples of each techniques. Finally, we discuss the perspectives and challenges in gold nanomaterial-based cancer therapy.
Introduction
Because of the high incidence and mortality of cancer, scientists have paid long term attention to the diagnosis and treatment of cancer. Conventional cancer therapies have clear side effects (Liu et al., 2019a), thus these shortcomings have prompted researchers to look for effective diagnostic strategies to struggle against cancer (Chen et al., 2016; Ju et al., 2019). With the development of modern technology and biological medicine, diagnosis, and treatment based gold nanomaterials are emerging to improve the therapeutic effect. Particularly, photothermal therapy (PTT) based on gold nanomaterials is a promising therapeutic modality, which can be combined with the advanced imaging modalities for the multi-functional therapeutic application (Huang et al., 2017; Wu et al., 2019).
Gold nanomaterials have been widely investigated as considerable biocompatible platforms for the biological field due to the advantages of simple synthesis, large surface area, adjustable optical property, and multiple surface modification (Conde et al., 2012; Jackman et al., 2017). Over the past decade, numerous fundamental reviews have comprehensively investigated the synthesis, size, optical properties, and modification of gold nanoparticles (Daniel and Astruc, 2004; Jain et al., 2008; Sardar et al., 2009; Sau et al., 2010; Jones et al., 2011; Cao et al., 2014; Chauhan and Mukherji, 2014; Singh et al., 2014; Rai et al., 2016; Amendola et al., 2017; Pareek et al., 2017), so these characteristics will be only briefly mentioned in this mini-review. Herein, the photothermal imaging (PTI), surface-enhanced Raman scattering (SERS), and photoacoustic imaging (PAI) guided in vivo cancer therapy are focused. What is worth noting is excellent photothermal effect, the localized surface plasmon resonance (LSPR) effect and enhanced electromagnetic field (SERS) of gold nanoparticles in the near infrared (NIR) region. Due to low absorption of water and hemoglobin, the NIR region (700–1,300 nm) is ideal for gold nanomaterials to have a deeper penetration depth in the tumor therapy and imaging (Bao et al., 2016). Gold nanomaterial can convert the absorbed light energy into overheating in the surrounding environment through non-radiative conversion owing to the desirable absorption cross sections and photothermal conversion efficiencies (η) in NIR region (Cao et al., 2019). Therefore, gold nanomaterials are considered as exogenous photothermal transduction agents for PTI and contrast agents for PAI, which can accumulate at tumor tissue via the enhanced permeability and retention (EPR) effect (Henry et al., 2016). Moreover, LSPR effect is a surface plasmon resonance phenomenon of the noble metal nanomaterials, which is heavily dependent on the composition, shape, size, and micro-environmental medium (Guo et al., 2015; Tian et al., 2016, 2018). In addition, SERS is generated from the strong phonon-electron interaction in the nanogaps (Girard et al., 2016). Thus, the Raman signals are enhanced by several orders of magnitude, and gold nanomaterials labeled with reporter molecules can be used as nanotags for in vivo imaging (Ding et al., 2016). In short, these gold nanomaterials having resonance peak within NIR (>800 nm), including gold nanorods, gold nanocages, gold nanoshells, and assemblies, can be used as photothermal agents, imaging agents, contrast agents, and therapeutic agents (Shanmugam et al., 2014). Table 1 presents some examples of in vivo imaging and therapeutic modalities based on different types of gold nanomaterials.
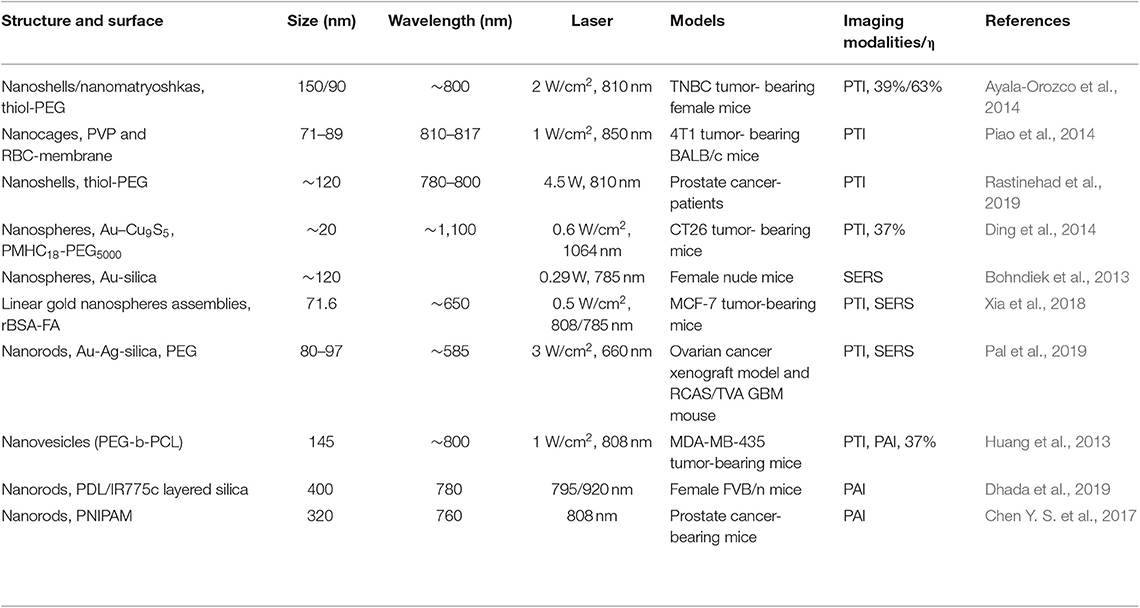
Table 1. Examples of different structural characteristics, optical properties, and in vivo imaging modality.
After intravenous injection, considering the pH, high ionic strength and serum concentration in the complex biological environment of organism, surface functionalization of gold nanomaterials is essential to ensure the adequate repeatability and stability (Chen et al., 2010). Basically, modulation of the surface charge, biocompatible pH values, controllable biodistribution patterns, and better aqueous dispersion of gold nanomaterials could benefit from the surface modification approaches (Huckaby and Lai, 2018; Ruiz-Muelle et al., 2019). Furthermore, the rational surface modification strategies in anticancer application can also reduce the toxicity of nanomaterials, target effectively in the cancer tissue, increase the circulatory half-life, block absorption of serum proteins, avoid unexpected side effects, escape the clearance by the reticuloendothelial system and the liver and spleen macrophages (Otsuka et al., 2003; Kooijmans et al., 2016; Dai et al., 2018; Sztandera et al., 2019). Due to the facile surface chemistry properties, functional groups can combined with gold nanomaterials through weak interactions and the stable covalent anchors (Kou et al., 2009; Moraes Silva et al., 2016; Zou et al., 2016; Wang K. et al., 2017). So far, many modification approaches have been introduced by covering and ligand exchange, so gold nanoparticles can be modified with biopolymer (e.g., polyethylene glycol (PEG), oligonucleotides, antibodies, peptides) (Loh et al., 2016; Chen Y. et al., 2017; Anantha-Iyengar et al., 2019; Delpiano et al., 2019), hydrophobic drug molecules (e.g., paclitaxel, cisplatin, tamoxifen, doxorubicin) (Avitabile et al., 2018; Ma et al., 2018), biofunctional molecules (chitosan, silica, folic acid, polyunsaturated fatty acids, and hyaluronic acid, etc.) (Vigderman and Zubarev, 2013; Sztandera et al., 2019) and other amphiphilic ligands through functional bridges, such as thiol ligands (e.g., thiolate, dithiolate, thioctic acid), amino, and carboxyl moieties (Figure 1) (Daraee et al., 2014; Kong et al., 2017).
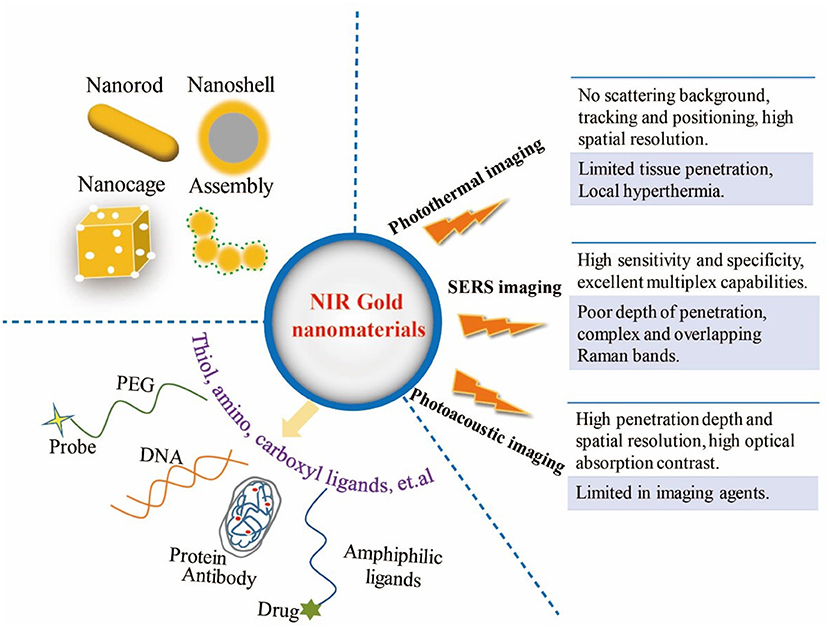
Figure 1. Illustration of multifunctional NIR gold nanomaterials platform for three imaging modalities.
Based on the physical and optical properties of gold nanomaterials, the bioimaging techniques using gold nanomaterials are beneficial to enhance the accuracy of in vivo treatment and effectiveness of real-time monitoring. Recently, the non-invasive imaging-guided cancer therapy based on gold nanomaterials have been applied for providing more valuable information and improving the therapy outcomes. This mini review displays some examples of gold nanomaterials-based imaging modes in NIR region for in vivo diagnosis and therapy, including PTI, SERS, PAI, and their multiple imaging modalities. These characteristics of the three imaging modalities are shown in Figure 1.
Photothermal Imaging (PTI)
For PTT, gold nanomaterials can transduce the absorbed light energy into heat energy for improving the temperature of tumor cells microenvironment. When the temperature exceeds 42°C, malignant cells are destroyed without damaging the adjacent healthy tissues (Qi et al., 2019). As a result, the therapeutic effect is far superior to that of laser irradiation alone (Abadeer and Murphy, 2016). The laser safety standard establish the maximum permissible exposure (MPE) values, which is on the basis of the damage threshold levels of laser light for skin (Jiao et al., 2019). For example, the MPE corresponding to 808 nm laser for skin is 0.33 W cm−2, and the MPE of 1,000–1,350 nm is 1 W cm−2 (Lin et al., 2017). Photothermal effect based on gold nanomaterials also afford a considerable imaging modality, that is, photothermal imaging (PTI), which has significant advantages over fluorescence imaging (Wang et al., 2009). PTI based on gold nanomaterials provides the accurate positioning, effective treatment, non-invasive therapy for various cancers in short time (Kang et al., 2018). Because it does not involve photobleaching or illumination saturation, and it is almost immune to background signals even accompanied with scattering (Boyer et al., 2002; Vines et al., 2019).
Conventional photothermal imaging is capable of detecting single molecules and differentiating gold nanoparticles, which is restricted by diffraction and resolution. A super-resolution photothermal imaging could be performed based on non-linear amplification signal for resolving multiple gold nanoparticles (Nedosekin et al., 2014). On account of the poor blood supply, tumors show reduced heat tolerance and thus can be destroyed in tens of minutes under hyperthermia condition (Qi et al., 2019). As known, intensive laser irradiation may lead to overheating and harm the nearby normal tissue. However, gold nanomaterials serve as the photothermal conversion agents to produce regional heating rapidly for PTT, and this method can effectively reduce irreversible tissue damage caused by laser irradiation (Ren et al., 2013). Owing to maximum transmittance in the blood and tissues in the NIR region, gold nanomaterials have deeper tissue penetration and lower background signal (Liu et al., 2014). With inherent advantages of multi-functionalization and tunable optical properties, gold nanomaterials coated with biomolecules had been developed to increase biological stability and prolong the circulation time in vivo (Choi et al., 2011). For example, red blood cell membranes coated gold nanocages (RBC-Au NCs) were combined with photothermal effects from gold nanocages and biological characteristics from RBCs, which showed good biological stability, observably improved tumor uptake, and longer retention time in blood (Piao et al., 2014). This work achieved 100% survival during 45 days and facilitated the RBC-Au NCs for the in vivo treatment with an improved efficacy using surface engineering.
Compared with other shapes, elongated nanoparticles have better affinity, greater diffusion and transmembrane rates, and they can penetrate the tumors rapidly and accumulate extensively (Lee et al., 2017). These had been demonstrated that the multifunctional nanomaterials had a high accumulation at tumor tissue in mice, which were able to kill cancer cells effectively by producing enough heat and reactive oxygen species. Chitosan/gold nanorod nanocarriers with loading anti-cancer drug cisplatin were prepared, which improved the therapeutic efficacy via synergistic effect of combining PTT and chemotherapy (Chen et al., 2013b). It had been found that the temperature of tumor site could reach 49°C in 10 min with IR irradiation and the tumor growth was almost completely suppressed by cisplatin-loaded nanospheres. As known, lanthanide ions have the ability to convert the NIR long-wavelength into visible wavelengths in photon upconversion process (Wang and Liu, 2009). Customarily, lanthanide doped nanocrystals having novel luminescent properties are considered as upconversion luminescence nanoparticles, which exhibit superior features in biological assays, such as low auto-fluorescence signals, narrow emission bandwidths, large anti-Stokes shifts, high penetration depth, and low toxicity (Huang et al., 2014; Gnach et al., 2015; Liu et al., 2019b). In order to increase the photothermal conversion efficiency and penetration depth in biological tissues, gold nanorods combined with rare-earth ions had been designed for PTT and multimodal imaging for anti-tumor therapy, because upconversion luminescence nanoparticles displayed deeper penetration and lower autofluorescence signals by anti-Stokes emission, and they could also transfer NIR light to gold nanorods via luminescence resonance energy transfer (Wang et al., 2018). With combination of phototherapy, gene therapy, and chemotherapy, the rational treatment strategy has an overwhelming superiority of tumor shrinkage and survival rate, which also could monitor host reactions and comprehensively evaluate tumor microenvironment. Lee et al. introduced silica-coated gold nanorods modified with rabies virus glycoprotein for treating brain tumors (Lee et al., 2017). The surface-modified nanomaterials could enter the brain through central nervous system and bypass the blood–brain barrier. The rod nanostructure increased the chance of interacting with the nicotinic acetylcholine receptor and the response to hyperthermia in NIR laser irradiation.
According to the reports, drug release, and chemotherapy displayed higher cytotoxicity for enhancing the therapeutic efficacy at raised temperatures from PTT (Vines et al., 2019; Wu et al., 2019). Indocyanine green was successfully sealed in chitosan/gold nanorod nanocapsule for PTT and photodynamic combined therapy (Chen et al., 2013a). Conde et al. adapted a triple-combination therapy that relied on drug-gold nanorods and siRNA-gold nanospheres for drug release, distinguish cancer and normal cells, and prevented cancer recurrence in a mouse model of colon cancer (Conde et al., 2016). In spite that drug-loaded nanocarriers with hyperthermia effect have therapeutic advantages for releasing targeting agents for tumor, there are still some drawbacks, such as insufficient or excessive dose by intravenous delivery (Tong et al., 2016; Wilhelm et al., 2016; Nabil et al., 2019). Recently, Lee et al. proposed a transplantable therapeutic interface based on a gold-coated nanoturf structure for on-demand hyperthermia therapy and drug delivery (Lee et al., 2018). The gold-coated polymeric nanoturf structure could not only serve as drug reservoir but also provide an induced heat under NIR irradiation, thus modulating drug releasing rate and controlling the surface temperature precisely for an esophageal cancer model. Moreover, to maximize the synergistic effects by exogenous and endogenous stimuli, two smart gold nanocages containers coated with photothermal and pH responsive polymer were designed for loading doxorubicin (Dox) and erlotinib (Erl), respectively (Feng et al., 2019). In the NIR irradiation and acidic tumor microenvironment stimulation, tumors were killed by synergistic therapy of PTT and time-staggered drug release strategy of Dox and Erl. The combination strategy obviously improved the therapy efficacy by controlling the order and continuance of drug in timing and spacing scale. These results show that it is difficult to completely ablate the tumor without any recurrence only based on PTI, because local photothermal is unable to impede the spread of cancer cells. Therefore, multiple imaging techniques need to be combined for redeeming the shortcomings of PTI.
In addition, gold nanoparticles are eliminated from the body to minimize health hazard at reasonable concentrations, which are supervised as medical devices by the Food and Drug Administration. Tremendous investigations on gold nanomaterials had been studied for biomedical sciences, and the preclinical safety of gold nanoshells had been established in vitro and in vivo (Gad et al., 2012). Recently, Halas et al. reported a study of photothermal ablation for prostate tumors in clinical trials which gold-silica nanoshells (7.5 mL/kg dose volume) were utilized in combination with imaging modality, without significant adverse events in 94% of patients (Rastinehad et al., 2019). This treatment protocol was demonstrated to be safe and feasible procedure for localized prostate cancer with low or intermediate risk, and it would open the door for gold nanomaterials in the clinical anticancer application. However, the bioinert and lack of biodegradation of gold nanomaterials bring uncertainty and toxicity to the human body. These negative feedbacks will directly affect the further clinical application of gold nanomaterials.
SERS Imaging
In general, Raman reporter molecules are attached to the surface of gold nanomaterials, providing unique, and certain representative peaks as the signal source (Lu et al., 2010b). The inelastic scattering of gold nanotags has narrow characteristic bandwidths with several orders of amplification, which provides sufficient structural and quantitative information for biological interactions and analyte (Maiti et al., 2010). SERS imaging is being recognized as a promising optical modality for preclinical and clinical cancer application. Compared with fluorescent imaging, SERS imaging can avoid photobleaching and photoblinking and serve as an eligible alternative with good photostability for in vivo imaging (Pal et al., 2019). Normally, magnetic resonance imaging (MRI) is time-consuming imaging with low sensitivity. While SERS imaging is not only fast speed and super sensitivity, but also has excellent multiplex capabilities and fine specificity for multiplex targeting (Von Maltzahn et al., 2009). In recent decades, SERS-based detection with enhanced electromagnetic field is extremely sensitive for trace analysis in nanoscale regions (Bardhan et al., 2014). More importantly, SERS imaging has weak interference signals in biological tissues, thus it is popular in high-sensitive cell tracking (Wang Z. et al., 2017).
As known, MRI has been usually used for preoperative directions and determining the macroscopic profile of the tumor. However, the typical imaging methods were limited by low sensitivity, and spatial resolution, and it was particularly difficult to describe the actual margins of tumors due to the brain shift in surgery (Orringer et al., 2010). For preoperative evaluation and intraoperative treatment, Kircher et al. showed a triple-modality to delineate the brain tumor boundaries in living mice (Kircher et al., 2012). Ideally, SERS imaging is a type of real-time imaging technique, which plays a main role in navigation for accurately delineating the brain tumor margins and guiding the tumor resection. With the development of SERS imaging for tissue in small animals, an imaging system with large area and high spatial resolution had been exploited (Bohndiek et al., 2013). This unique spectroscopic instrument showed an ultra-high sensitivity for a non-invasive imaging modality. It simplified animal handling, and thus could realize rapid and multiplex detection for the characterization of uptake dynamics in vivo. Gold nanoparticles covered with Raman active molecules are contrast agents with an enhanced scattering intensity. The size of core nanomaterials, the properties of Raman molecules, and the number of the molecules absorbed on the surface determine the order of magnitude of amplification. Harmsen et al. presented an administrative SERS nanoparticle for intraoperative imaging in mouse models of glioblastoma, which was composed of a gold core and a layer of Raman reporter molecules embedded in a silica shell (Harmsen et al., 2015). As a result, the limit of detection (LOD) reached femtomolar. Furthermore, Wang's group synthesized 3D flower-like hierarchical gold nanostructures and gold nanostars for cancer therapy (Song et al., 2016, 2018, 2019). The SERS-active gold nanomaterials could use as SERS tags and nanocarriers for cell imaging and drug delivery, which were suitable candidates for promising SERS-imaging.
For in vivo SERS mapping, gold nanostars conjugated with antibodies and Raman tags were able to detect the immunomodulators and immunomarkers simultaneously (Ou et al., 2018). With the accumulation of gold nanostars by intravenous injection, the real-time longitudinal tracking of the both biomarkers was implemented, and the sensitivity and specificity of the relevant SERS signals displayed different levels in breast cancer tumors. Furthermore, the high-resolution SERS imaging could evaluate the distributions of gold nanostars in tumors which were closely related to vascular density. With wonderful optical properties, one-dimensional gold nanoparticle assemblies (GNAs) with good dispersion, stability, and biocompatibility were developed for in vivo imaging (Xia et al., 2018). The GNAs exhibited numerous ultra-small nanogaps (smaller than 1.0 nm) and flexible caterpillar-like nanostructures, which could change their morphology randomly. Due to the remarkable SERS signal and good photothermal effect, the GNAs were used as an efficient platform for SERS imaging and photothermal imaging. Currently, SERS scanners are dependent on the point-by-point acquisition, with a relatively slow speed, which could not be satisfied for real-time and rapid imaging in oncological application. Encouraged by the versatility of fluorescence imaging and the specificity of SERS detection, rational design of nanoprobes had been carried out for fluorescence and SERS imaging in ovarian cancer xenograft models (Pal et al., 2019). The bimodal nanoprobes based on DNA and gold nanorods with three consecutive layers were used successfully for imaging-guided tumor ablation and PTT. Meanwhile, aptamer-conjugated gold nanocage has also been built as the bifunctional theranostic platforms for SERS imaging and NIR-triggered PTI (Wen et al., 2019). However, the real biological environment of the human body is more intricate than animal models, and most of the modified nanoprobes are likely to be metabolized directly by immune system. Therefore, the results can lead to the reduced active targeting, the limitation of circulation time and insufficient dose of these nanoprobes that will affect the therapeutic and imaging effects.
Photoacoustic Imaging (PAI)
As known, contrast agents or tissue can absorb the non-ionizing laser and be heated, leading to a transient thermoelastic expansion. Subsequently, wideband acoustic waves are produced as photoacoustic waves, which can be captured on the surface of target substance (Ju et al., 2019). Usually, computed tomography (CT) imaging is high toxicity, the non-specific distribution of contrast agents, and short imaging time (Xu et al., 2019). However, PAI relies on the photoacoustic effect with high reliability, and the gold contrast agents can be purposely modified. Additionally, compared with MRI and fluorescent imaging, PAI is non-invasive, quantitative, and speedy, and it also presents high spatial-resolution of ultrasound imaging and contrast of optical imaging (Zackrisson et al., 2014). PAI has been demonstrated as a powerful tool to visualize biological tissues with the advantages of deep penetration and high spatial-resolution (Agarwal et al., 2007).
Gold nanomaterials are one kind of contrast agents for providing improved photoacoustic signals because of chemical inertness and large absorption cross sections in NIR region (Yang et al., 2015). PEG-coated Au nanocage and pegylated hollow gold nanospheres had been described as optical contrast agents for in vivo PAI (Yang et al., 2007; Lu et al., 2010a). Specifically, PEGylation-modified gold nanostructures with different shapes, including nanospheres, nanodisks, nanorods, and cubic nanocages, had already been investigated for bioactivity analyses in EMT6 breast cancer model (Black et al., 2014). Furthermore, gold nanorods had been applied to image in ovarian tumor models with a multimodal imaging (Jokerst et al., 2012a). In this study, the parallel PAI and SERS imaging had complementary capabilities, where the PAI characterized the size, morphology, and stage of the tumor, and SERS imaging guided the surgical resection. The silica-coated gold nanorods as PAI agents exhibited a higher cellular up-take and had no toxic effect on normal cells. They had been prepared to quantitate and image mesenchymal stem cells for living mice in real time, and the results showed the spatial resolution of 340 μm and the temporal resolution of 0.2 s (Jokerst et al., 2012b). To track the stem cell in cardiovascular diseases, silica-gold nanorods with coating IR775c, which was a sensitive dye of reactive oxygen species, had been developed for PAI (Dhada et al., 2019). The nanoprobe had a high spatial and temporal resolution, and they displayed a 5% viability of mesenchymal stem cells after 10 days.
Due to the small size of nanoparticles with the overlap heating volume, the intensity of photoacoustic signal could be increased proportionally to the huge thermal energy which was rapidly generated from the conversion from optical absorption (Chen et al., 2012). Chen et al. synthesized small gold nanoparticle with controlled aggregation in a volume-changing nanocarrier that had photothermal stimuli-responsive behavior (Chen Y. S. et al., 2017). Overall, the PAI shows a dynamic contrast-enhancement, while the background signals from tissue is suppressed. Although the photonic nanoclusters are well-understood and have been applied in a variety of bioimaging, the bottom-up assembly based on nucleic acid scaffolds is still a challenge. As an example, the plasmonic self-assembles had been exploited as photo-responsive probes for multimodal SERS and PAI in vivo (Köker et al., 2018). In this method, the discrete gold nanoparticles were functionalized with two complementary split fragments of green fluorescent protein. Park et al. manufactured a gold nanoclusters based on gold nanoparticles (about 4.5 nm) and albumin for optically visualizing and treating colon cancers via PTT, fluorescence imaging, and PAI (Park et al., 2019). By optimizing the size, shape, and optical absorption of the hybrid albumin nanostructures, the promising platform displayed a strong hyperthermic effect, as well as a balance between LSPR and fluorescence resonance energy transfer effects.
Conclusions and Perspectives
The research on bioimaging has been mainly promoted by the systematic exploitation of nanomaterials and the combination of modern techniques. Different types of functionalized gold nanosensors have been reported with the development of the surface modification. In this review, the multifunctional gold nanomaterials have been showed for imaging-guided in vivo cancer therapy using photothermal, SERS, PAI, and multiples imaging modalities. Various types of gold nanostructured loading platforms have been studied for imaging and treatment of cancers. Nonetheless, there remain some challenges and opportunities for further and higher demand for in vivo and clinical studies. Firstly, the development of gold nanomaterials is crucial for the applications of optical imaging and synergetic therapy. For ideal imaging and therapeutic results, three-dimensional structure, special configuration of assemblies and composite gold nanomaterials are popular for the bio-application. For example, some gold nanomaterials with well-defined architectures and luxuriant hot spots, including composite gold nanomaterials and self-assemblies, may generate stronger electromagnetic-field enhancement for SERS imaging. These novel nanomaterials also can be explored as excellent contrast agents for PAI to overcome the problem of penetration depth. Meanwhile, the multifunctional agents and biological responsive (acceerative or passivated) molecules also necessary to be developed with less toxic and better dispersion for the organism. Therefore, the appropriate multifunctional agents can avoid the possibility that the partial malignant cells are intact after local hyperthermia in the effective PTT. It is also possibility to enhance the NIR responsiveness, improve the accuracy of targeting, and increase the efficiency of the delivery during the cancer treatment. On the other hand, SERS possess single molecule sensitivity in vitro experiment, and the elaborated gold nanomaterials will be promising candidate to reach this level and free from the interference of other species in the biological system. Consequently, efficient composite gold nanoparticles with desirable surface modification agents are pressingly needed to achieve the desired effect in vivo. Similarly, the thermal resistance also can be reduced by modifying interfacial agents to improve the photoacoustic signal and resolution. Almost all of the experimental and theoretical researches have focused on the animal modes, while the clinical study is still lacking. Lastly, it is necessary to integrate diagnosis, multimodal imaging, and enhanced therapies for clinical application. Elaborate detecting strategies and sensitive multiplex techniques can enhance the spatial and temporal resolution, capture actual dynamic processes in real-time, and answer the fundamental biological questions in modern medicine. Finally, most of the research has focused on the biological interactions, intracellular distribution, and the transport behaviors of gold nanomaterials, while the detailed mechanism of different interaction with biomolecules and the process of internalization are still lack of deep understanding.
Author Contributions
LW and SQ designed and revised this maunscript. YT wrote this manuscript.
Funding
This work was financially supported by the National Key Research and Development Program of China (2017YFA0205300), the National Natural Science Foundation of China (61571239), the Program for Changjiang Scholars and Innovative Research Team in University (IRT_15R37), and the Key Research and Development Program of Jiangsu (BE2018732).
Conflict of Interest
The authors declare that the research was conducted in the absence of any commercial or financial relationships that could be construed as a potential conflict of interest.
References
Abadeer, N. S., and Murphy, C. J. (2016). Recent progress in cancer thermal therapy using gold nanoparticles. J. Phys. Chem. C 120, 4691–4716. doi: 10.1021/acs.jpcc.5b11232
Agarwal, A., Huang, S. W., O'Donnell, M., Day, K. C., Day, M., Kotov, N., et al. (2007). Targeted gold nanorod contrast agent for prostate cancer detection by photoacoustic imaging. J. Appl. Phys. 102:064701. doi: 10.1063/1.2777127
Amendola, V., Pilot, R., Frasconi, M., Maragò, O. M., and Iatì, M. A. (2017). Surface plasmon resonance in gold nanoparticles: a review. J. Phys. Condens. Matter. 29:203002. doi: 10.1088/1361-648X/aa60f3
Anantha-Iyengar, G., Shanmugasundaram, K., Nallal, M., Lee, K.-P., Whitcombe, M. J., Lakshmi, D., et al. (2019). Functionalized conjugated polymers for sensing and molecular imprinting applications. Prog. Polym. Sci. 88, 1–129. doi: 10.1016/j.progpolymsci.2018.08.001
Avitabile, E., Bedognetti, D., Ciofani, G., Bianco, A., and Delogu, L. G. (2018). How can nanotechnology help the fight against breast cancer? Nanoscale 10, 11719–11731. doi: 10.1039/C8NR02796J
Ayala-Orozco, C., Urban, C., Knight, M. W., Urban, A. S., Neumann, O., Bishnoi, S. W., et al. (2014). Au nanomatryoshkas as efficient near-infrared photothermal transducers for cancer treatment: benchmarking against nanoshells. ACS Nano 8, 6372–6381. doi: 10.1021/nn501871d
Bao, Z., Liu, X., Liu, Y., Liu, H., and Zhao, K. (2016). Near-infrared light-responsive inorganic nanomaterials for photothermal therapy. Asian J. Pharm. Sci. 11, 349–364. doi: 10.1016/j.ajps.2015.11.123
Bardhan, M., Satpati, B., Ghosh, T., and Senapati, D. (2014). Synergistically controlled nano-templated growth of tunable gold bud-to-blossom nanostructures: a pragmatic growth mechanism. J. Mater. Chem. C 2, 3795–3804. doi: 10.1039/C4TC00160E
Black, K. C., Wang, Y., Luehmann, H. P., Cai, X., Xing, W., Pang, B., et al. (2014). Radioactive au-198-doped nanostructures with different shapes for in vivo analyses of their biodistribution, tumor uptake, and intratumoral distribution. ACS Nano 8, 4385–4394. doi: 10.1021/nn406258m
Bohndiek, S. E., Wagadarikar, A., Zavaleta, C. L., Van de Sompel, D., Garai, E., Jokerst, J. V., et al. (2013). A small animal raman instrument for rapid, wide-area, spectroscopic imaging. Proc. Natl. Acad. Sci. U.S.A. 110, 12408–12413. doi: 10.1073/pnas.1301379110
Boyer, D., Tamarat, P., Maali, A., Lounis, B., and Orrit, M. (2002). Photothermal imaging of nanometer-sized metal particles among scatterers. Science 297, 1160–1163. doi: 10.1126/science.1073765
Cao, J., Sun, T., and Grattan, K. T. V. (2014). Gold nanorod-based localized surface plasmon resonance biosensors: a review. Sens. Actuat. 195, 332–351. doi: 10.1016/j.snb.2014.01.056
Cao, W., He, Y., Zhu, R., He, Y., Hao, Z., Zhao, Q., et al. (2019). Nir light triggered size variable “remote-controlled cluster bomb” for deep penetration and tumor therapy. Chem. Eng. J. 375, 122080. doi: 10.1016/j.cej.2019.122080
Chauhan, S. K., and Mukherji, S. (2014). Gold nanoparticles and nanostructures in optical biosensors. Mater. Technol. 30, B167–B177. doi: 10.1179/1753555714Y.0000000212
Chen, G., Roy, I., Yang, C., and Prasad, P. N. (2016). Nanochemistry and nanomedicine for nanoparticle-based diagnostics and therapy. Chem. Rev. 116, 2826–2885. doi: 10.1021/acs.chemrev.5b00148
Chen, H. J., Ming, T. A., Zhao, L., Wang, F., Sun, L. D., Wang, J. F., et al. (2010). Plasmon-molecule interactions. Nano Today 5, 494–505. doi: 10.1016/j.nantod.2010.08.009
Chen, R., Wang, X., Yao, X., Zheng, X., Wang, J., and Jiang, X. (2013a). Near-ir-triggered photothermal/photodynamic dual-modality therapy system via chitosan hybrid nanospheres. Biomaterials 34, 8314–8322. doi: 10.1016/j.biomaterials.2013.07.034
Chen, R., Zheng, X. C., Qian, H. Q., Wang, X., Wang, J., and Jiang, X. Q. (2013b). Combined near-ir photothermal therapy and chemotherapy using gold-nanorod/chitosan hybrid nanospheres to enhance the antitumor effect. Biomater. Sci. 1, 285–293. doi: 10.1039/C2BM00138A
Chen, Y., Xianyu, Y., and Jiang, X. (2017). Surface modification of gold nanoparticles with small molecules for biochemical analysis. Acc. Chem. Res. 50, 310–319. doi: 10.1021/acs.accounts.6b00506
Chen, Y. S., Frey, W., Aglyamov, S., and Emelianov, S. (2012). Environment-dependent generation of photoacoustic waves from plasmonic nanoparticles. Small 8, 47–52. doi: 10.1002/smll.201101140
Chen, Y. S., Yoon, S. J., Frey, W., Dockery, M., and Emelianov, S. (2017). Dynamic contrast-enhanced photoacoustic imaging using photothermal stimuli-responsive composite nanomodulators. Nat. Commun. 8:15782. doi: 10.1038/ncomms15782
Choi, W. I., Kim, J. Y., Kang, C., Byeon, C. C., Kim, Y. H., and Tee, G. (2011). Tumor regression in vivo by photothermal therapy based on gold-nanorod-loaded, functional nanocarriers. ACS Nano 5, 1995–2003. doi: 10.1021/nn103047r
Conde, J., Doria, G., and Baptista, P. (2012). Noble metal nanoparticles applications in cancer. J. Drug Delivery 2012:751075. doi: 10.1155/2012/751075
Conde, J., Oliva, N., Zhang, Y., and Artzi, N. (2016). Local triple-combination therapy results in tumour regression and prevents recurrence in a colon cancer model. Nat. Mater. 15, 1128–1138. doi: 10.1038/nmat4707
Dai, Q., Wilhelm, S., Ding, D., Syed, A. M., Sindhwani, S., Zhang, Y., et al. (2018). Quantifying the ligand-coated nanoparticle delivery to cancer cells in solid tumors. ACS Nano 12, 8423–8435. doi: 10.1021/acsnano.8b03900
Daniel, M. C., and Astruc, D. (2004). Gold nanoparticles: assembly, supramolecular chemistry, quantum-size-related properties, and applications toward biology, catalysis, and nanotechnology. Chem. Rev. 104, 293–346. doi: 10.1021/cr030698+
Daraee, H., Eatemadi, A., Abbasi, E., Fekri Aval, S., Kouhi, M., and Akbarzadeh, A. (2014). Application of gold nanoparticles in biomedical and drug delivery. Artif. Cells, Nanomed. Biotechnol. 44, 410–422. doi: 10.3109/21691401.2014.955107
Delpiano, G. R., Casula, M. F., Piludu, M., Corpino, R., Ricci, P. C., Vallet-Regí, M., et al. (2019). Assembly of multicomponent nano-bioconjugates composed of mesoporous silica nanoparticles, proteins, and gold nanoparticles. ACS Omega 4, 11044–11052. doi: 10.1021/acsomega.9b01240
Dhada, K. S., Hernandez, D. S., and Suggs, L. J. (2019). In vivo photoacoustic tracking of mesenchymal stem cell viability. ACS Nano 13, 7791–7799. doi: 10.1021/acsnano.9b01802
Ding, S. Y., Yi, J., Li, J. F., Ren, B., Wu, D. Y., Panneerselvam, R., et al. (2016). Nanostructure-based plasmon-enhanced raman spectroscopy for surface analysis of materials. Nat. Rev. Mater. 1:16021. doi: 10.1038/natrevmats.2016.21
Ding, X., Liow, C. H., Zhang, M., Huang, R., Li, C., Shen, H., et al. (2014). Surface plasmon resonance enhanced light absorption and photothermal therapy in the second near-infrared window. J. Am. Chem. Soc. 136, 15684–15693. doi: 10.1021/ja508641z
Feng, Y., Cheng, Y., Chang, Y., Jian, H., Zheng, R., Wu, X., et al. (2019). Time-staggered delivery of erlotinib and doxorubicin by gold nanocages with two smart polymers for reprogrammable release and synergistic with photothermal therapy. Biomaterials 217:119327. doi: 10.1016/j.biomaterials.2019.119327
Gad, S. C., Sharp, K. L., Montgomery, C., Payne, J. D., and Goodrich, G. P. (2012). Evaluation of the toxicity of intravenous delivery of auroshell particles (gold-silica nanoshells). Internet J. Toxicol. 31, 584–594. doi: 10.1177/1091581812465969
Girard, A., Gehan, H., Crut, A., Mermet, A., Saviot, L., and Margueritat, J. (2016). Mechanical coupling in gold nanoparticles supermolecules revealed by plasmon-enhanced ultralow frequency raman spectroscopy. Nano Lett. 16, 3843–3849. doi: 10.1021/acs.nanolett.6b01314
Gnach, A., Lipinski, T., Bednarkiewicz, A., Rybka, J., and Capobianco, J. A. (2015). Upconverting nanoparticles: assessing the toxicity. Chem. Soc. Rev. 44, 1561–1584. doi: 10.1039/C4CS00177J
Guo, L., Jackman, J. A., Yang, H.-H., Chen, P., Cho, N.-J., and Kim, D.-H. (2015). Strategies for enhancing the sensitivity of plasmonic nanosensors. Nano Today 10, 213–239. doi: 10.1016/j.nantod.2015.02.007
Harmsen, S., Huang, R. M., Wall, M. A., Karabeber, H., Samii, J. M., Spaliviero, M., et al. (2015). Surface-enhanced resonance raman scattering nanostars for high-precision cancer imaging. Sci. Transl. Med. 7:11. doi: 10.1126/scitranslmed.3010633
Henry, A. I., Sharma, B., Cardinal, M. F., Kurouski, D., and Van Duyne, R. P. (2016). Surface-enhanced raman spectroscopy biosensing: in vivo diagnostics and multimodal imaging. Anal. Chem. 88, 6638–6647. doi: 10.1021/acs.analchem.6b01597
Huang, P., Lin, J., Li, W., Rong, P., Wang, Z., Wang, S., et al. (2013). Biodegradable gold nanovesicles with an ultrastrong plasmonic coupling effect for photoacoustic imaging and photothermal therapy. Angew. Chem. 52, 13958–13964. doi: 10.1002/anie.201308986
Huang, P., Zheng, W., Zhou, S., Tu, D., Chen, Z., Zhu, H., et al. (2014). Lanthanide-doped liluf(4) upconversion nanoprobes for the detection of disease biomarkers. Angew. Chem. 53, 1252–1257. doi: 10.1002/anie.201309503
Huang, X., Zhang, W., Guan, G., Song, G., Zou, R., and Hu, J. (2017). Design and functionalization of the nir-responsive photothermal semiconductor nanomaterials for cancer theranostics. Acc. Chem. Res. 50, 2529–2538. doi: 10.1021/acs.accounts.7b00294
Huckaby, J. T., and Lai, S. K. (2018). Pegylation for enhancing nanoparticle diffusion in mucus. Adv. Drug Deliv. Rev. 124, 125–139. doi: 10.1016/j.addr.2017.08.010
Jackman, J. A., Rahim Ferhan, A., and Cho, N. J. (2017). Nanoplasmonic sensors for biointerfacial science. Chem. Soc. Rev. 46, 3615–3660. doi: 10.1039/C6CS00494F
Jain, P. K., Huang, X. H., El-Sayed, I. H., and El-Sayed, M. A. (2008). Noble metals on the nanoscale: optical and photothermal properties and some applications in imaging, sensing, biology, and medicine. Acc. Chem. Res. 41, 1578–1586. doi: 10.1021/ar7002804
Jiao, L., Wang, J., Fan, Y., and Yang, Z. (2019). Porcine skin damage thresholds and histological damage characteristics from 1319-nm laser radiation. J. Biomed. Opt. 24, 1–9. doi: 10.1117/1.JBO.24.9.095003
Jokerst, J. V., Cole, A. J., Van de Sompel, D., and Gambhir, S. S. (2012a). Gold nanorods for ovarian cancer detection with photoacoustic imaging and resection guidance via raman imaging in living mice. ACS Nano 6, 10366–10377. doi: 10.1021/nn304347g
Jokerst, J. V., Thangaraj, M., Kempen, P. J., Sinclair, R., and Gambhir, S. S. (2012b). Photoacoustic imaging of mesenchymal stem cells in living mice via silica-coated gold nanorods. ACS Nano 6, 5920–5930. doi: 10.1021/nn302042y
Jones, M. R., Osberg, K. D., Macfarlane, R. J., Langille, M. R., and Mirkin, C. A. (2011). Templated techniques for the synthesis and assembly of plasmonic nanostructures. Chem. Rev. 111, 3736–3827. doi: 10.1021/cr1004452
Ju, Y., Dong, B., Yu, J., and Hou, Y. (2019). Inherent multifunctional inorganic nanomaterials for imaging-guided cancer therapy. Nano Today 26, 108–122. doi: 10.1016/j.nantod.2019.03.006
Kang, H., Hu, S., Cho, M. H., Hong, S. H., Choi, Y., and Choi, H. S. (2018). Theranostic nanosystems for targeted cancer therapy. Nano Today 23, 59–72. doi: 10.1016/j.nantod.2018.11.001
Kircher, M. F., de la Zerda, A., Jokerst, J. V., Zavaleta, C. L., Kempen, P. J., Mittra, E., et al. (2012). A brain tumor molecular imaging strategy using a new triple-modality mri-photoacoustic-raman nanoparticle. Nat. Med. 18, 829–834. doi: 10.1038/nm.2721
Köker, T., Tang, N., Tian, C., Zhang, W., Wang, X., Martel, R., et al. (2018). Cellular imaging by targeted assembly of hot-spot sers and photoacoustic nanoprobes using split-fluorescent protein scaffolds. Nat. Commun. 9:607. doi: 10.1038/s41467-018-03046-w
Kong, F. Y., Zhang, J. W., Li, R. F., Wang, Z. X., Wang, W. J., and Wang, W. (2017). Unique roles of gold nanoparticles in drug delivery, targeting and imaging applications. Molecules 22:1445. doi: 10.3390/molecules22091445
Kooijmans, S. A. A., Fliervoet, L. A. L., van der Meel, R., Fens, M., Heijnen, H. F. G., van Bergen En Henegouwen, P. M. P., et al. (2016). Pegylated and targeted extracellular vesicles display enhanced cell specificity and circulation time. J. Control. Release 224, 77–85. doi: 10.1016/j.jconrel.2016.01.009
Kou, X., Sun, Z., Yang, Z., Chen, H., and Wang, J. (2009). Curvature-directed assembly of gold nanocubes, nanobranches, and nanospheres. Langmuir 25, 1692–1698. doi: 10.1021/la802883p
Lee, C., Hwang, H. S., Lee, S., Kim, B., Kim, J. O., Oh, K. T., et al. (2017). Rabies virus-inspired silica-coated gold nanorods as a photothermal therapeutic platform for treating brain tumors. Adv. Mater. 29:1605563. doi: 10.1002/adma.201605563
Lee, S., Hwang, G., Kim, T. H., Kwon, S. J., Kim, J. U., Koh, K., et al. (2018). On-demand drug release from gold nanoturf for a thermo- and chemotherapeutic esophageal stent. ACS Nano 12, 6756–6766. doi: 10.1021/acsnano.8b01921
Lin, H., Gao, S., Dai, C., Chen, Y., and Shi, J. (2017). A two-dimensional biodegradable niobium carbide (mxene) for photothermal tumor eradication in nir-i and nir-ii biowindows. J. Am. Chem. Soc. 139, 16235–16247. doi: 10.1021/jacs.7b07818
Liu, X., Huang, N., Li, H., Wang, H., Jin, Q., and Ji, J. (2014). Multidentate polyethylene glycol modified gold nanorods for in vivo near-infrared photothermal cancer therapy. ACS Appl. Mater. Interfaces 6, 5657–5668. doi: 10.1021/am5001823
Liu, Y., Bhattarai, P., Dai, Z., and Chen, X. (2019a). Photothermal therapy and photoacoustic imaging via nanotheranostics in fighting cancer. Chem. Soc. Rev. 48, 2053–2108. doi: 10.1039/C8CS00618K
Liu, Y., Meng, X., and Bu, W. (2019b). Upconversion-based photodynamic cancer therapy. Coord. Chem. Rev. 379, 82–98. doi: 10.1016/j.ccr.2017.09.006
Loh, X. J., Lee, T. C., Dou, Q., and Deen, G. R. (2016). Utilising inorganic nanocarriers for gene delivery. Biomater. Sci. 4, 70–86. doi: 10.1039/C5BM00277J
Lu, W., Huang, Q., Ku, G., Wen, X., Zhou, M., Guzatov, D., et al. (2010a). Photoacoustic imaging of living mouse brain vasculature using hollow gold nanospheres. Biomaterials 31, 2617–2626. doi: 10.1016/j.biomaterials.2009.12.007
Lu, W., Singh, A. K., Khan, S. A., Senapati, D., Yu, H., and Ray, P. C. (2010b). Gold nano-popcorn-based targeted diagnosis, nanotherapy treatment, and in situ monitoring of photothermal therapy response of prostate cancer cells using surface-enhanced raman spectroscopy. J. Am. Chem. Soc.132, 18103–18114. doi: 10.1021/ja104924b
Ma, X., Xiong, Y., and Lee, L. T. O. (2018). Application of nanoparticles for targeting g protein-coupled receptors. Int. J. Mol. Sci. 19:E2006. doi: 10.3390/ijms19072006
Maiti, K. K., Dinish, U. S., Fu, C. Y., Lee, J. J., Soh, K. S., Yun, S. W., et al. (2010). Development of biocompatible sers nanotag with increased stability by chemisorption of reporter molecule for in vivo cancer detection. Biosens. Bioelectron. 26, 398–403. doi: 10.1016/j.bios.2010.07.123
Moraes Silva, S., Tavallaie, R., Sandiford, L., Tilley, R. D., and Gooding, J. J. (2016). Gold coated magnetic nanoparticles: from preparation to surface modification for analytical and biomedical applications. Chem. Commun. 52, 7528–7540. doi: 10.1039/C6CC03225G
Nabil, G., Bhise, K., Sau, S., Atef, M., El-Banna, H. A., and Iyer, A. K. (2019). Nano-engineered delivery systems for cancer imaging and therapy: Recent advances, future direction and patent evaluation. Drug Discov. Today 24, 462–491. doi: 10.1016/j.drudis.2018.08.009
Nedosekin, D. A., Galanzha, E. I., Dervishi, E., Biris, A. S., and Zharov, V. P. (2014). Super-resolution nonlinear photothermal microscopy. Small 10, 135–142. doi: 10.1002/smll.201300024
Orringer, D. A., Chen, T., Huang, D. L., Armstead, W. M., Hoff, B. A., Koo, Y. E., et al. (2010). The brain tumor window model: a combined cranial window and implanted glioma model for evaluating intraoperative contrast agents. Neurosurgery 66, 736–743. doi: 10.1227/01.NEU.0000367631.02903.50
Otsuka, H., Nagasaki, Y., and Kataoka, K. (2003). Pegylated nanoparticles for biological and pharmaceutical applications. Adv. Drug. Deliv. Rev. 55, 403–419. doi: 10.1016/S0169-409X(02)00226-0
Ou, Y. C., Webb, J. A., O'Brien, C. M., Pence, I. J., Lin, E. C., Paul, E. P., et al. (2018). Diagnosis of immunomarkers in vivo via multiplexed surface enhanced raman spectroscopy with gold nanostars. Nanoscale 10, 13092–13105. doi: 10.1039/C8NR01478G
Pal, S., Ray, A., Andreou, C., Zhou, Y., Rakshit, T., Wlodarczyk, M., et al. (2019). DNA-enabled rational design of fluorescence-raman bimodal nanoprobes for cancer imaging and therapy. Nat. Commun. 10:1926. doi: 10.1038/s41467-019-09173-2
Pareek, V., Bhargava, A., Gupta, R., Jain, N., and Panwar, J. (2017). Synthesis and applications of noble metal nanoparticles: a review. Adv. Sci. Eng. Med. 9, 527–544. doi: 10.1166/asem.2017.2027
Park, S., Kim, H., Lim, S. C., Lim, K., Lee, E. S., Oh, K. T., et al. (2019). Gold nanocluster-loaded hybrid albumin nanoparticles with fluorescence-based optical visualization and photothermal conversion for tumor detection/ablation. J. Control. Release 304, 7–18. doi: 10.1016/j.jconrel.2019.04.036
Piao, J. G., Wang, L., Gao, F., You, Y. Z., Xiong, Y., and Yang, L. (2014). Erythrocyte membrane is an alternative coating to polyethylene glycol for prolonging the circulation lifetime of gold nanocages for photothermal therapy. ACS Nano 8, 10414–10425. doi: 10.1021/nn503779d
Qi, Z., Shi, J., Zhang, Z., Cao, Y., Li, J., and Cao, S. (2019). Pegylated graphene oxide-capped gold nanorods/silica nanoparticles as multifunctional drug delivery platform with enhanced near-infrared responsiveness. Mater. Sci. Eng. 104:109889. doi: 10.1016/j.msec.2019.109889
Rai, M., Ingle, A. P., Birla, S., Yadav, A., and Santos, C. A. (2016). Strategic role of selected noble metal nanoparticles in medicine. Crit. Rev. Microbiol. 42, 696–719.
Rastinehad, A. R., Anastos, H., Wajswol, E., Winoker, J. S., Sfakianos, J. P., Doppalapudi, S. K., et al. (2019). Gold nanoshell-localized photothermal ablation of prostate tumors in a clinical pilot device study. Proc. Natl. Acad. Sci. U.S.A. 116, 18590–18596. doi: 10.1073/pnas.1906929116
Ren, F., Bhana, S., Norman, D. D., Johnson, J., Xu, L., Baker, D. L., et al. (2013). Gold nanorods carrying paclitaxel for photothermal-chemotherapy of cancer. Bioconjugate Chem. 24, 376–386. doi: 10.1021/bc300442d
Ruiz-Muelle, A. B., Kuttner, C., Alarcón-Fernández, C., López-Romero, J. M., Uhlmann, P., Contreras-Cáceres, R., et al. (2019). Hybrid surfaces active in catalysis based on gold nanoparticles modified with redox-active pendants and polymer brushes. Appl. Surf. Sci. 496:143598. doi: 10.1016/j.apsusc.2019.143598
Sardar, R., Funston, A. M., Mulvaney, P., and Murray, R. W. (2009). Gold nanoparticles: past, present, and future. Langmuir 25, 13840–13851. doi: 10.1021/la9019475
Sau, T. K., Rogach, A. L., Jäckel, F., Klar, T. A., and Feldmann, J. (2010). Properties and applications of colloidal nonspherical noble metal nanoparticles. Adv. Mater. 22, 1805–1825. doi: 10.1002/adma.200902557
Shanmugam, V., Selvakumar, S., and Yeh, C. S. (2014). Near-infrared light-responsive nanomaterials in cancer therapeutics. Chem. Soc. Rev. 43, 6254–6287. doi: 10.1039/C4CS00011K
Singh, G., van Helvoort, A. T. J., Bandyopadhyay, S., Volden, S., Andreassen, J.-P., and Glomm, W. R. (2014). Synthesis of au nanowires with controlled morphological and structural characteristics. Appl. Surf. Sci. 311, 780–788. doi: 10.1016/j.apsusc.2014.05.162
Song, C., Dou, Y., Yuwen, L., Sun, Y., Dong, C., Li, F., et al. (2018). A gold nanoflower-based traceable drug delivery system for intracellular sers imaging-guided targeted chemo-phototherapy. J. Mater. Chem. 6, 3030–3039. doi: 10.1039/C8TB00587G
Song, C., Li, F., Guo, X., Chen, W., Dong, C., Zhang, J., et al. (2019). Gold nanostars for cancer cell-targeted sers-imaging and nir light-triggered plasmonic photothermal therapy (pptt) in the first and second biological windows. J. Mater. Chem. 7, 2001–2008. doi: 10.1039/C9TB00061E
Song, C. Y., Yang, B. Y., Chen, W. Q., Dou, Y. X., Yang, Y. J., Zhou, N., et al. (2016). Gold nanoflowers with tunable sheet-like petals: facile synthesis, sers performances and cell imaging. J. Mater. Chem. 4, 7112–7118. doi: 10.1039/C6TB01046F
Sztandera, K., Gorzkiewicz, M., and Klajnert-Maculewicz, B. (2019). Gold nanoparticles in cancer treatment. Mol. Pharm. 16, 1–23. doi: 10.1021/acs.molpharmaceut.8b00810
Tian, Y., Shuai, Z., Shen, J., Zhang, L., Chen, S., Song, C., et al. (2018). Plasmonic heterodimers with binding site-dependent hot spot for surface-enhanced raman scattering. Small 14:e1800669. doi: 10.1002/smll.201800669
Tian, Y., Zhang, L., Shen, J., Wu, L., He, H., Ma, D. L., et al. (2016). An individual nanocube-based plasmonic biosensor for real-time monitoring the structural switch of the telomeric g-quadruplex. Small 12, 2913–2920. doi: 10.1002/smll.201600041
Tong, X., Wang, Z., Sun, X., Song, J., Jacobson, O., Niu, G., et al. (2016). Size dependent kinetics of gold nanorods in epr mediated tumor delivery. Theranostics 6, 2039–2051. doi: 10.7150/thno.17098
Vigderman, L., and Zubarev, E. R. (2013). Therapeutic platforms based on gold nanoparticles and their covalent conjugates with drug molecules. Adv. Drug. Deliv. Rev. 65, 663–676. doi: 10.1016/j.addr.2012.05.004
Vines, J. B., Yoon, J. H., Ryu, N. E., Lim, D. J., and Park, H. (2019). Gold nanoparticles for photothermal cancer therapy. Front. Chem. 7:167. doi: 10.3389/fchem.2019.00167
Von Maltzahn, G., Centrone, A., Park, J. H., Ramanathan, R., Sailor, M. J., Hatton, T. A., et al. (2009). Sers-coded gold nanorods as a multifunctional platform for densely multiplexed near-infrared imaging and photothermal heating. Adv. Mater. 21, 3175–3180. doi: 10.1002/adma.200803464
Wang, C., Chen, J., Talavage, T., and Irudayaraj, J. (2009). Gold nanorod/fe3o4 nanoparticle “nano-pearl-necklaces” for simultaneous targeting, dual-mode imaging, and photothermal ablation of cancer cells. Angew. Chem. Int. Ed. Engl. 48, 2759–2763. doi: 10.1002/anie.200805282
Wang, C., Xu, C., Xu, L., Sun, C., Yang, D., Xu, J., et al. (2018). A novel core–shell structured upconversion nanorod as a multimodal bioimaging and photothermal ablation agent for cancer theranostics. J. Mater. Chem. B 6, 2597–2607. doi: 10.1039/C7TB02842C
Wang, F., and Liu, X. (2009). Recent advances in the chemistry of lanthanide-doped upconversion nanocrystals. Chem. Soc. Rev. 38, 976–989. doi: 10.1039/b809132n
Wang, K., Shangguan, L., Liu, Y., Jiang, L., Zhang, F., Wei, Y., et al. (2017). In situ detection and imaging of telomerase activity in cancer cell lines via disassembly of plasmonic core-satellites nanostructured probe. Anal. Chem. 89, 7262–7268. doi: 10.1021/acs.analchem.7b01882
Wang, Z., Zong, S., Wu, L., Zhu, D., and Cui, Y. (2017). Sers-activated platforms for immunoassay: Probes, encoding methods, and applications. Chem. Rev. 117, 7910–7963. doi: 10.1021/acs.chemrev.7b00027
Wen, S., Miao, X., Fan, G. C., Xu, T., Jiang, L. P., Wu, P., et al. (2019). Aptamer-conjugated au nanocage/sio2 core-shell bifunctional nanoprobes with high stability and biocompatibility for cellular sers imaging and near-infrared photothermal therapy. ACS Sens. 4, 301–308. doi: 10.1021/acssensors.8b00682
Wilhelm, S., Tavares, A. J., Dai, Q., Ohta, S., Audet, J., Dvorak, H. F., et al. (2016). Analysis of nanoparticle delivery to tumours. Nat. Rev. Mater. 1:16014. doi: 10.1038/natrevmats.2016.14
Wu, Y., Ali, M. R. K., Chen, K. C., Fang, N., and El-Sayed, M. A. (2019). Gold nanoparticles in biological optical imaging. Nano Today 24, 120–140. doi: 10.1016/j.nantod.2018.12.006
Xia, Y., Ma, X., Gao, J., Chen, G., Li, Z., Wu, X., et al. (2018). A flexible caterpillar-like gold nanoparticle assemblies with ultrasmall nanogaps for enhanced dual-modal imaging and photothermal therapy. Small 14:e1800094. doi: 10.1002/smll.201800094
Xu, Y., Wang, X., Cheng, L., Liu, Z., and Zhang, Q. (2019). High-yield synthesis of gold bipyramids for in vivo ct imaging and photothermal cancer therapy with enhanced thermal stability. Chem. Eng. J. 378:122025. doi: 10.1016/j.cej.2019.122025
Yang, X., Skrabalak, S. E., Li, Z. Y., Xia, Y., and Wang, L. V. (2007). Photoacoustic tomography of a rat cerebral cortex in vivo with au nanocages as an optical contrast agent. Nano Lett. 7, 3798–3802. doi: 10.1021/nl072349r
Yang, X., Yang, M., Pang, B., Vara, M., and Xia, Y. (2015). Gold nanomaterials at work in biomedicine. Chem. Rev. 115, 10410–10488. doi: 10.1021/acs.chemrev.5b00193
Zackrisson, S., van de Ven, S. M. W. Y., and Gambhir, S. S. (2014). Light in and sound out: emerging translational strategies for photoacoustic imaging. Cancer Res. 74, 979–1004. doi: 10.1158/0008-5472.CAN-13-2387
Keywords: gold nanomaterials, in vivo, photothermal therapy, surface-enhanced Raman scattering, photoacoustic imaging
Citation: Tian Y, Qiang S and Wang L (2019) Gold Nanomaterials for Imaging-Guided Near-Infrared in vivo Cancer Therapy. Front. Bioeng. Biotechnol. 7:398. doi: 10.3389/fbioe.2019.00398
Received: 18 September 2019; Accepted: 22 November 2019;
Published: 05 December 2019.
Edited by:
Michael Ming-Yuan Wei, Texas Commission on Environmental Quality, United StatesReviewed by:
Raviraj Vankayala, Independent Researcher, Irvine, United StatesJyothi U. Menon, University of Rhode Island, United States
Copyright © 2019 Tian, Qiang and Wang. This is an open-access article distributed under the terms of the Creative Commons Attribution License (CC BY). The use, distribution or reproduction in other forums is permitted, provided the original author(s) and the copyright owner(s) are credited and that the original publication in this journal is cited, in accordance with accepted academic practice. No use, distribution or reproduction is permitted which does not comply with these terms.
*Correspondence: Sheng Qiang, wrl@njau.edu.cn; Lianhui Wang, iamlhwang@njupt.edu.cn