Introduction: The placement of a vascular stent is a common intervention to address the obstruction of blood flow as a result of atherosclerotic disease[1],[2]. Problems associated with metal stents led to the development of bioresorbable stents (BRS)[3]. BRSs allow resistance against late stent thrombosis[4] and restoration of natural vasomotion following stent resorption. Poly(L-lactide) (PLLA) or its copolymer with glycolic acid have been investigated for BRS[6],[6]. However, degradation is very slow, potentially affecting long-term tissue remodeling, while the polymer degradation products are oxidative stress-inducing[7]-[9], which could exacerbate tissue inflammation and intimal hyperplasia[10],[11]. Polymer properties also limit strut designs, complicating BRS manufacture. Finally, none of these stents allow for patient-specific customization, which could improve vessel patency. In this work we investigated a UV-curable, antioxidant polydiolcitrate together with projection microstereolithography (PμSL) to fabricate bioresorbable stents that can be customized for each patient. This flexible additive manufacturing could potentially be used for on-the-spot printing, e.g. in the operating room[12],[13].
Materials and Methods: Biomaterial Ink: Citric acid and 1,12-dodecanediol were melted in a 2:1 ratio, co-polymerized (140°C, 30 min), purified and freeze-dried. This pre-polymer (22g) was dissolved in tetrahydrofuran (180 mL) with imidazole (816 mg) and glycidyl methacrylate (17.04 g), heated (60°C, 6 hrs) and purified to yield methacrylated poly(1,12-dodecamethylene citrate) (mPDC). To formulate B-InkTM, 52% mPDC was mixed with 2.2% Irgacure 819 (photo-initiator), 0.2% Sudan I (UV absorber to control curing depth) and 46% diethyl fumarate (solvent to control viscosity) (Fig. 1A). Degradation: UV-cured films were incubated in PBS, collected at time points, washed with water, freezedried and weighed (Fig. 1B). Antioxidant properties: Cured films were incubated in free radical ABTS solution. The absorption peak at 734 nm can be monitored and color change is a measure of free radical scavenging (Fig. 1C). Cell compatibility: Cured mPDC was gas sterilized, incubated in DMEM media to remove unreacted monomers and seeded with human aortic smooth muscle cells (HASMC). After 3 days, viability was assessed with calcein AM (Fig.1D). Projection microstereolithography (PμSL): Stents were built from the B-InkTM in a 20 µm layer-by-layer fashion directly from a 3D CAD design (Fig. 2B). Each layer was cured by single exposure using a liquid crystal display panel as a dynamic mask for UV light. After the final layer, stents were further polymerized with additional UV exposure (Fig. 2A). Morphology: Morphology of the printed stents was observed via scanning electron microscopy (SEM) (Fig. 2C, D). Mechanical testing: Radial compression tests of stents to 25% of the stents' outer diameter were performed on an Instron 5544 mechanical tester (Fig. 2E).
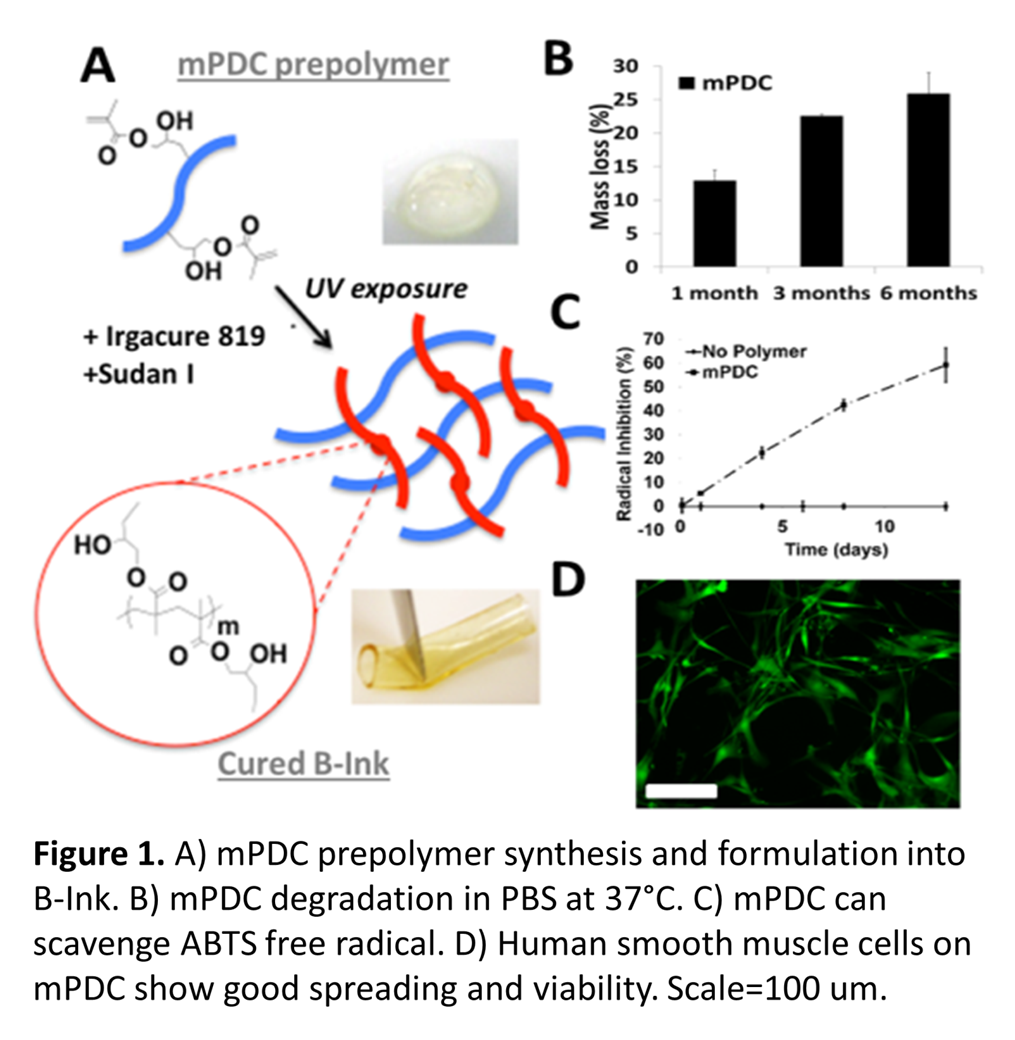
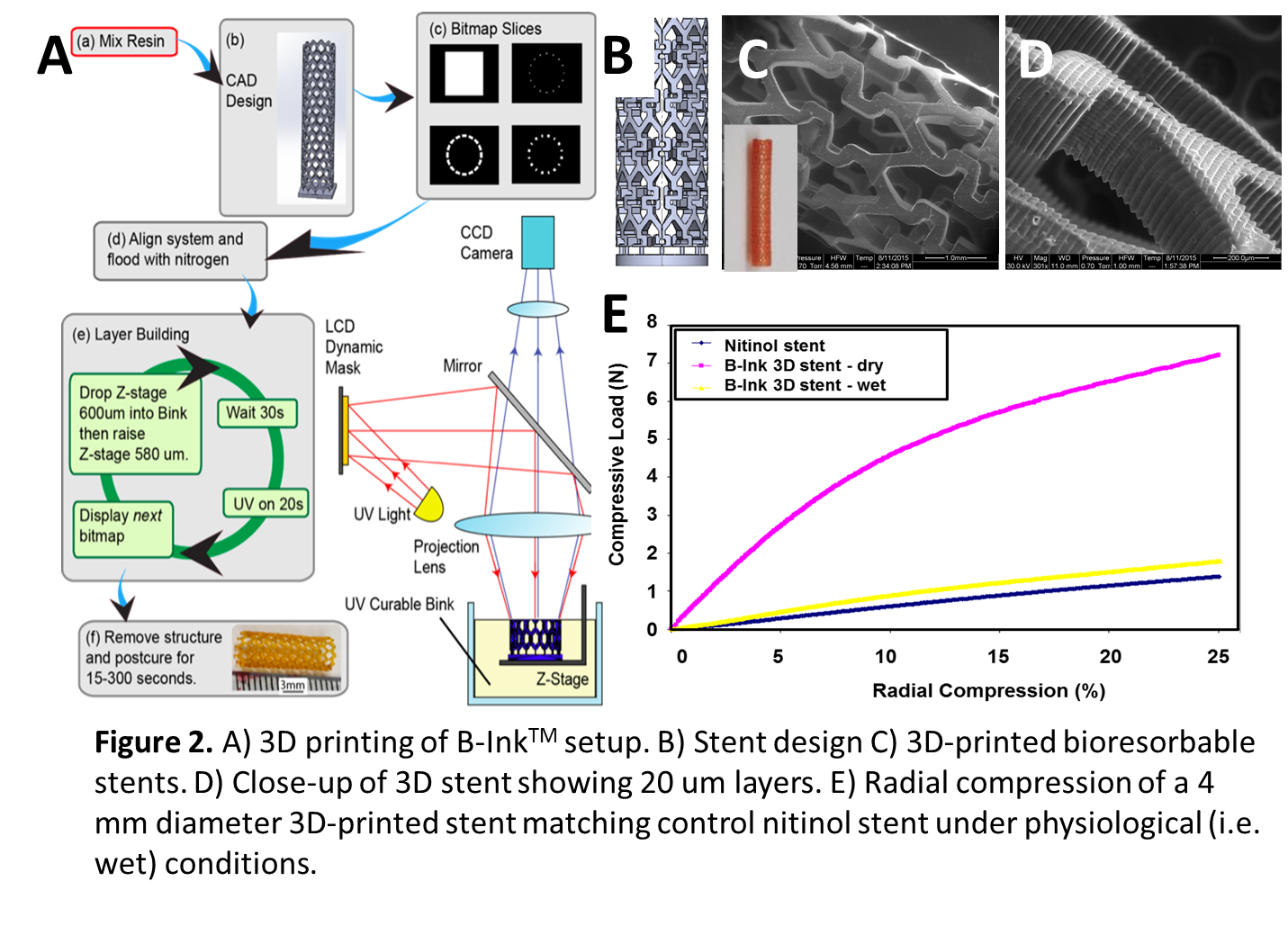
Results and Discussion: Synthesized mPDC could be cured by UV exposure (Fig. 1A). mPDC is degradable, antioxidant and cell compatible (Fig. 1B-D). Formulation into B-InkTM enabled UV-based 3D printing of stents with 20 µm layers using projection microstereolithography (Fig. 2A-D). The mechanical properties of 3D-printed stents with struts of 400 µm were comparable to those of a control nitinol stent (Fig. 2E). These results hold great promise for on-the-spot patient-customized stent manufacture.
References:
[1] Kudagi, V. S.; White, C. J. Am J Cardiovasc Drugs 2013, 13, 199-212. DOl:10.1007/s40256-013-0023-6
[2] Litsky, J.; Chanda, A.; Stilp, E.; Lansky, A.; Mena, C. Medical Devices (Auckland, N.Z.) 2014, 7, 149-156. DOl:10.2147/MDER.S45472
[3] Kassimis, G.; Spiliopoulos, S.; Katsanos, K.; Tsetis, D.; Krokidis, M. E. Expert Rev Cardiovasc Ther 2014, 12, 443-50. DOl:10.1586/14779072.2014.897226
[4] Bosiers, M.; Cagiannos, C.; Deloose, K.; Verbist, J.; Peeters, P. Vascular Health and Risk Management 2008, 4, 553-559.
[5] Onuma, Y.; Serruys, P. W. Circulation 2011, 123, 779-797. DOl:10.1161/circulationaha.110.971606
[6] Iqbal, J.; Onuma, Y.; Ormiston, J.; Abizaid, A.; Waksman, R.; Serruys, P. Eur Heart J 2014, 35, 765-76. DOl:10.1093/eurheartj/eht542
[7] Abbott, D. A.; Suir, E.; Duong, G. H.; de Hulster, E.; Pronk, J. T.; van Maris, A. J. Appl Environ Microbiol 2009, 75, 2320-5. DOl:10.1128/aem.00009-09
[8] Selvam, S.; Kundu, K.; Templeman, K. L.; Murthy, N.; Garcia, A. J. Biomaterials 2011, 32, 7785-92. DOl:10.1016/j.biomaterials.2011.07.020
[9] Zhou, J.; Tsai, Y. T.; Weng, H.; Tang, L. Free Radic Biol Med 2012, 52, 218-26. DOl:10.1016/j.freeradbiomed.2011.10.452
[10] Kawamoto, R.; Yamashita, A.; Nishihira, K.; Furukoji, E.; Hatakeyama, K.; Ishikawa, T.; Imamura, T.; Itabe, H.; Eto, T.; Asada, Y. Pathol Res Pract 2006, 202, 447-56. DOl:10.1016/j.prp.2005.12.011
[11] Juni, R. P.; Duckers, H. J.; Vanhoutte, P. M.; Virmani, R.; Moens, A. L. J Am Coll Cardiol 2013, 61, 1471-81. DOl:10.1016/j.jacc.2012.11.068
[12] Mota, C.; Puppi, D.; Chiellini, F.; Chiellini, E. Journal of Tissue Engineering and Regenerative Medicine 2015, 9, 174-190. DOl:10.1002/term.1635
[13] Bose, S.; Vahabzadeh, S.; Bandyopadhyay, A. Materials Today 2013, 16, 496-504. DOl:http://dx.doi.org/10.1016/j.mattod.2013.11.017