Introduction: For the foreseeable future, bioprosthetic heart valves (BHV) fabricated from xenograft biomaterials will remain the dominant replacement prosthetic valve design. However, BHV durability remains limited to 10-15 years. Failure is usually the result of leaflet structural deterioration mediated by fatigue and/or tissue mineralization. Thus, independent of valve design specifics (e.g. standard stented valve, percutaneous delivery), the development of novel biomaterials with improved durability remains an important clinical goal. This represents a unique cardiovascular engineering challenge resulting from the extreme valvular mechanical demands that occur with blood contact. In the present study a fatigue damage model (FDM) based on our structural constitutive model was developed for heart valve tissues.
Methods: In the present work we utilized a meso-scale structural constitutive modeling approach to formulate a novel approach. We focus on a FDM for the time evolving (i.e. over many thousands of cycles, not beat-to-beat) BHV mechanical properties.One major focus was to delineate differences in bulk mechanical properties due to tissue-level dimensional and structural changes (i.e. due to permanent set like effects resulting from repeated loading) and the intrinsic changes in the constituent fibers (i.e. changes in effective fiber modulus). Conventional fatigue damage approaches are clearly not applicable to BHV tissues due to their mechanical and structural complexity.Thus, we will start with the structural modeling approach laid out in the previous section to formulate a novel approach, utilizing our extensive experience with BHV tissues, to develop a FDM for the time evolving (i.e. over many thousands of cycles, not beat-to-beat) BHV mechanical properties. One major focus was delineation of the differences in bulk mechanical properties due to tissue-level dimensional and structural changes (i.e. due to permanent set like effects resulting from repeated loading) and the intrinsic changes in the constituent fibers (i.e. changes in effective fiber modulus). Following damage theory convention, we will utilize a normalized scalar damage metric variable D(t), which ranges from 0 for new (virgin) material to 1 for completely damage (failed). We will initially assume D(t) follows first-order kinetics, which can be changed to higher order kinetics as needed. Since similar expressions can be developed for each model parameter, this normalized approach will allow the time constants for each variable to be directly compared. The choice of an exponential rate function is supported by available data on porcine stentless GLUT fixed BHV, where first-order kinetics appear to be an appropriate approximation. As a modification from our original formulation, we note that exogenous chemical cross-links present at the collagen molecular level induce a great increase in effective fiber stiffness.
Results: From the pre-implant (virgin) tissue, initial values were obtained from the results of existing data.
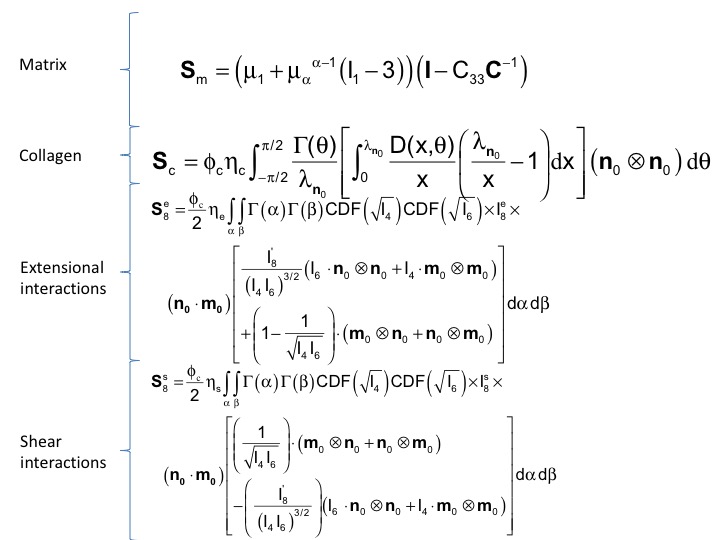
Of particular interest was the observation that it was the fiber-fiber interactions that dominated the functional stress range.
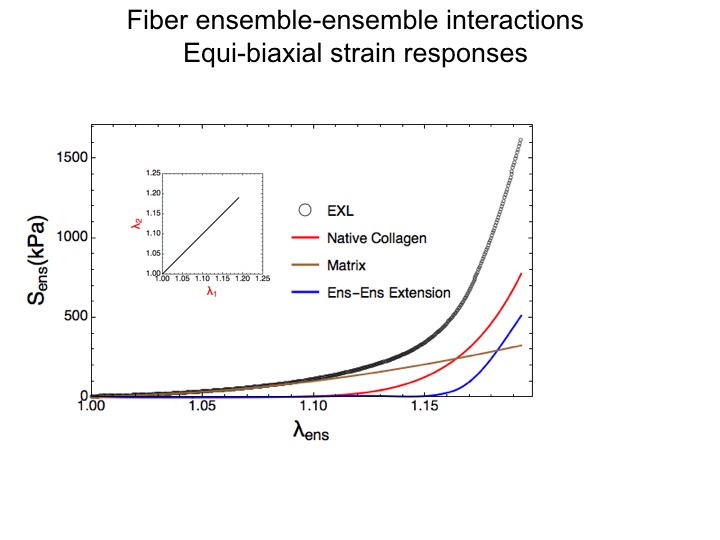
Time course changes obtained for D(t) for each parameter was obtained using extensive existing data from our lab. We were able to quantify, separately, the rates of change in effective fiber stiffness from the changes in fiber splay and collagen fiber recruitment and their net contributions to tissue level behavior and durability. The model was then implemented utilized in ABAQUS to simulate the permanent set effects previously observed by our lab. As a first pass, we were able to simulate permanent set effects at the organ level.
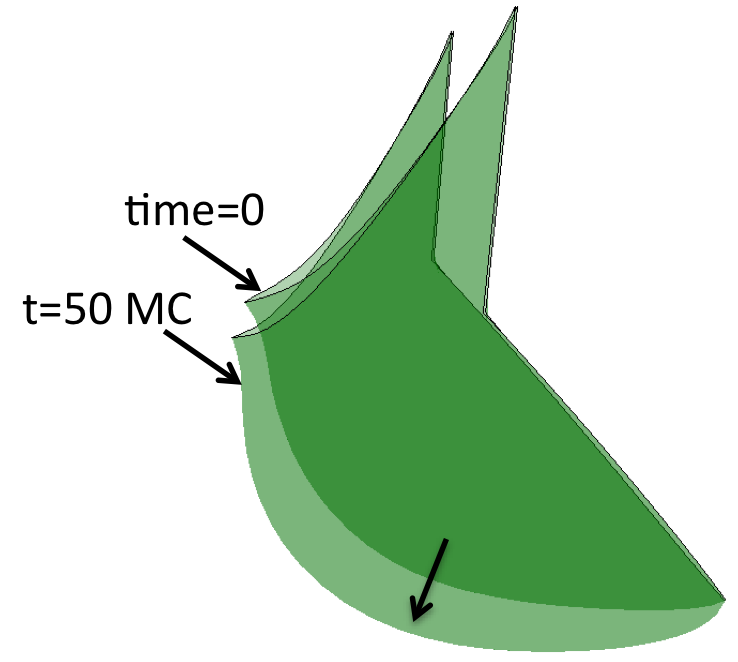
This fact was important in of itself, as changes in prosthetic device level will affect leaflet stress distributions.
Discussion: In the present study a fatigue damage model (FDM) based on our structural constitutive model was developed for heart valve tissues. We are currently extending the model to understand how key ECM components (collagen, elastin, GAGs) individually respond as a biomaterial in-vivo, and to simulate changes in their structure and mechanical function at the tissue level. This model is currently being implemented in a finite element framework for the purposes of BHV life prediction.
NIH R01 HL108330.