Introduction: Mimicking the cellular microenvironment and signaling involved in cell differentiation and tissue healing is paramount for an optimal biofunctionality of biomaterials[1]. In this regard, the biofunctionalization of biomaterials with peptides and proteins from the extracellular matrix (ECM) has long been investigated to improve cell-material interactions[2],[3].
The use of native proteins from the ECM is the most intuitive way to mimic the complexity of cell-matrix interactions. However, their use remains controversial and several disadvantages have been reported[3],[4]. Synthetic peptides encompassing only biologically relevant epitopes, such as the integrin-binding motif RGD, have been proposed as alternative. However, peptides fail to reproduce cell-matrix signaling and to elicit cell-specific responses, resulting in poor clinical outcomes[4].
To overcome these limitations, we have focused on the development of innovative biological coatings with enhanced integrin-specificity and/or multiple biological potential, to design cell-instructive and versatile biomaterials for tissue regeneration. These biomolecular tools include multifunctional peptide-based platforms[5], integrin-selective peptidomimetics[6],[7] and nanopolymeric systems[8] (Fig. 1).
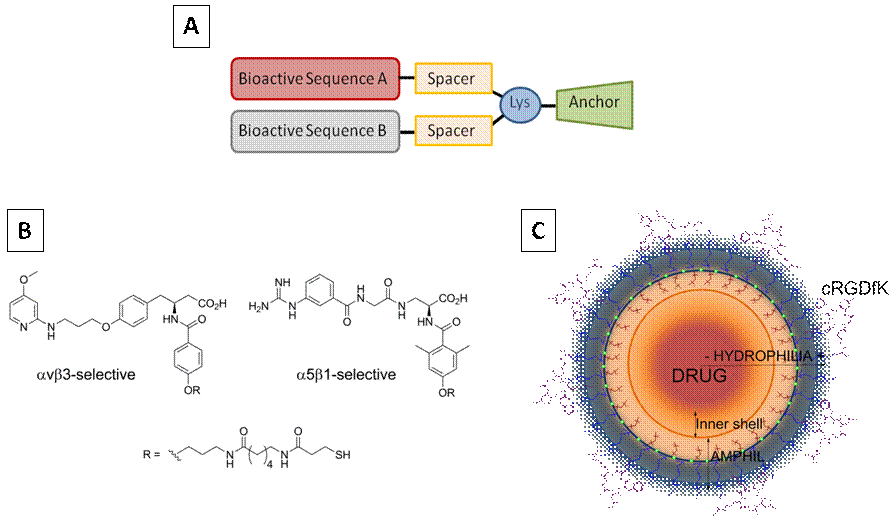
Fig.1. Biomolecular tools for surface coating A) Peptide-based multifunctional platform; B) Integrin-selective peptidomimetics; C) RGD-decorated polymeric nanocapsules.
In this communication, I will focus on the design and synthesis of two novel multifunctional platforms (Fig. 1A) i) A platform containing the RGD and PHSRN motifs, which synergize the binding to integrin α5β1 (PTF 1); ii) and a platform combining an RGD motif with a potent antimicrobial peptide (AMP)[9] (PTF 2). The biofunctionalization of surfaces with these molecules will be characterized at the physicochemical level and their biological performance analyzed in vitro and in vivo.
Materials and Methods: The divalent platforms were synthesized by solid-phase peptide synthesis and characterized by HPLC and MS spectrometry. The molecules were immobilized on titanium disks via silanization. The binding and stability of the platforms was characterized by contact angle and XPS. In vitro studies with Saos-2 cells and mesenchymal stems cells (MSCs) analyzed cell adhesion, proliferation and osteogenic differentiation (mineralization, ALP activity and gene expression). Antibacterial activity was studied with Streptococcus sanguinis by conventional bacterial adhesion assays. In vivo studies were done in rat calvarial defects as animal model of osseointegration. Statistical analysis was done with one-way ANOVA analysis and non-parametric methods.
Results and Discussion: The platforms were successfully immobilized on the surfaces. The binding was proven stable to mechanical challenges. PTF 1 improved osteoblast adhesion, spreading and proliferation, as well as osteogenic differentiation of MSCs in comparison to control samples. Noteworthy, it displayed improved cell responses compared to samples coated with an equimolar mixture of the two motifs. These data was confirmed in vivo in a calvarial rat model. Moreover, the simultaneous presentation of a cell adhesive motif with an AMP in PTF 2 enhanced osteoblast adhesion and strongly reduced bacterial attachment on the surfaces.
Conclusions: The data presented here demonstrates that installing multifunctionality on biomaterials in a chemically controlled manner represents a powerful approach to develop new biomaterials with unprecedented biological potency.
The authors thank the Spanish Government for financial support through Project No. MAT2012–30706, cofunded by the European Union through European Regional Development Funds, and the Government of Catalonia (SGR2009 1039). C.M.-M. thanks the support of the Secretary for Universities and Research of the Ministry of Economy and Knowledge of the Government of Catalonia (2011-BP-B-00042) and the People Programme (Marie Curie Actions) of the European Union’s Seventh Framework Programme (FP7-PEOPLE-2012-CIG, REA Grant Agreement No. 321985).
References:
[1] N. J. Walters, E. Gentleman. Acta Biomater. 2015, 11, 3-16.
[2] A. E. Rodda, L. Meagher, D. R. Nisbet, J. S. Forsythe. Prog. Polym. Sci. 2014, 39, 1312-1347.
[3] C. Mas-Moruno, M. Espanol, E. B. Montufar, G. Mestres, C. Aparicio, F. J. Gil, M. P. Ginebra. In: A. Taubert, J. F. Mano, J. C. Rodríguez-Cabello (editors). Biomaterials Surface Science. Wiley-VCH, Weinheim, Germany. 2013, 337-374.
[4] D. F. Williams. Biomaterials 2011, 32, 4195-4197.
[5] C. Mas-Moruno, R. Fraioli, F. Albericio, J. M. Manero, F. J. Gil. ACS Appl. Mater. Interfaces 2014, 6, 6525-6536.
[6] F. Rechenmacher, S. Neubauer, J. Polleux, C. Mas-Moruno, M. De Simone, E. A. Cavalcanti-Adam, J. P. Spatz, R. Fässler, H. Kessler. Angew. Chem. Int. Ed. 2013, 52, 1572-1575.
[7] R. Fraioli, F. Rechenmacher, S. Neubauer, J. M. Manero, J. Gil, H. Kessler, C. Mas-Moruno. Colloids Surf. B Biointerfaces. 2015, 128, 191-200.
[8] P. Rocas, M. Hoyos-Nogués, J. Rocas, J. M. Manero, J. Gil, F. Albericio, C. Mas-Moruno. Adv. Healthc. Mater. 2015, 4, 1956-60.
[9] M. Godoy-Gallardo, C. Mas-Moruno, M. C. Fernández-Calderón, C. Pérez-Giraldo, J. M. Manero, F. Albericio, F. J. Gil, D. Rodríguez. Acta Biomater. 2014, 10, 3522-3534.