Introduction: Diabetic wounds cause significant morbidity, prolonged hospitalization, and enormous healthcare expense. Timely healing and closure is critical to reducing the cost and morbidity associated with chronic diabetic lower extremity wounds. Debridement of the wound area and grafting with autologous split thickness skin grafts (STSG) is still the gold standard for the treatment of chronic wounds[1]. However, the success rate of STSG for healing chronic wounds is low in the range of 33-73%[2]. Besides, meshed skin grafts usually require large areas of donor skin tissues for wound coverage, which causes the potential risks of donor site morbidity and poor wound healing unique to the diabetic state. Meshing of autografts was developed to allow for coverage of larger areas but to date most clinically useful expansion ratios are 2:1, 3:1, and 4:1. Expansion ratios of 6:1 and 9:1 are possible but they are difficult to handle and require a lengthy period of time to “fill-in” the interstices. Our recent studies demonstrated radially aligned nanofiber scaffolds can direct and promote cell migration from surrounding tissues to the center of wound[3]. We also demonstrated nanofiber scaffolds with square-arrayed microwells and nanostructural cues were capable of guiding and enhancing cell migration in multiple directions to the surrounding skin tissue void area[4]. This study is to combine tissue engineering strategies and currently viable clinical approaches to develop nanofiber skin grafts for diabetic wound healing.
Materials and Methods: Nanofibers were produced utilizing a standard electrospinning setup, described previously[5]. Poly(ε-caprolactone) (PCL) (Mw=80 kDa, Sigma-Aldrich, St. Louis, MO) was dissolved in a solvent mixture consisting of dichloromethane (DCM) and N, N-dimethylformamide (DMF) (Fisher Chemical, Waltham, MA) with a ratio of 4:1 (v/v) (at a concentration 10% (w/v)).
Results and Discussion: Our study has demonstrated the seeding of NIH3T3 fibroblasts to the microwells and further examined the migration and repopulation of NIH3T3 fibroblasts.4 We demonstrated that cells can cover the whole area more quickly and distribute more evenly on our uniquely designed nanofiber scaffolds with square arrayed microwells and structural cues compared to the random and uniaxially-aligned fiber samples. We further demonstrated that covering by radially aligned nanofiber scaffolds can promote the migration of 3T3 fibroblasts seeded to microwells. We also demonstrated that human dermal fibroblasts exhibited the similar behavior as 3T3 fibroblasts when cultured on the nanofiber scaffolds with arrayed microwells and nanostructural cues. Our recent data demonstrated the human skin tissue culture on fibronectin-coated nanofiber scaffolds with square arrayed microwells and nanotopographic cues (Fig. 1). Our preliminary results showed that skin cells can migrate to the surrounding area from microskin tissue islands and respond to the nanotopographic cues rendered by aligned nanofibers, indicating that the major part of the cells migrated out from microskin tissues seeded to the microwells of nanofiber scaffolds were keratinocytes. In addition, we demonstrated the feasibility of implantation of 'sandwich'-type skin graft to an excisional skin wound in a porcine model.
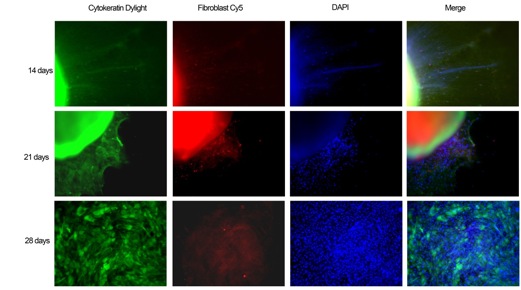
Fig. 1. Fragments of human skin tissues were seeded on PCL microwell scaffolds for 14, 21 and 28 days. The fibers were first treated with air plasma then coated with poly-lysine and fibronectin. Fluorescence microscopy images showed cells migrated out from skin tissues and proliferated on the nanofiber scaffolds. Keratinocytes were labeled with anti-cytokertin Dlight in green, dermal fibroblasts were labeled with Cy5 in red, and nuclei were stained with DAPI in blue.
Conclusion: In summary, these novel nanofiber scaffolds could serve as substrates providing nanotopographic cues to direct and enhance cell migration and sustained release of antimicrobial agents and growth factors for prevention of infection and thus promotion of wound healing.
NIH NIGMS grant 2P20GM103480-08 and UNMC Regenerative Medicine Startup Fund.
References:
[1] R. Simman, L. Phavixay, J Am Col Certif Wound Spec., 2011, 3, 55.
[2] T. Hogsberg, T. Bjarnsholt, J. S. Thomsen, K. Kirketerp-Moller, PloS ONE, 2011, 6, e20492.
[3] J. Xie, M. R. MacEwan, W. Z. Ray, W. Liu, D. Y. Siewe, Y. Xia, ACS Nano, 2010, 4, 5027.
[4] B. Ma, J. Xie, J. Jiang, J. Wu, Biomaterials, 2014, 35, 630.
[5] J. Jiang, J. Xie, B. Ma, D. E. Bartlett, A. Xu, C. H. Wang, Acta Biomater., 2013, 10, 1324.