Developing strontium-substituted bioglass and polycaprolactone composite scaffolds for bone repair via hybrid electrospinning in the direct writing mode
-
1
Queensland University of Technology, The Institute of Health and Biomedical Innovation, Australia
-
2
Imperial College London, Department of Materials, United Kingdom
-
3
Queensland University of Technology, The Medical Engineering Research Facility, Australia
Introduction: Electrospinning is a versatile fibre-based technique for scaffold production with smaller fibre diameter, and thus larger surface area to volume ratio compared to melt extrusion. Recent developments in direct writing melt-electrospinning makes use of the stable and predictable fibre deposition to fabricate scaffolds in the layer-by-layer manner[1],[2].
Synthetic materials including polymers and bioactive glass (BG) are most common for bone scaffold fabrication[3],[4]. Among the synthetic polymers, polycaprolactone (PCL), which is a FDA-approved aliphatic polyester, has been become a promising candidate for bone scaffold material due to its favourable rheological and viscoelastic properties[5]. Bioactive glass (BG) is a synthetic material known to bind seamlessly to bone and promote osteogenesis. Strontium-substituted BG derived from the original 45S5 BG by substituting 0-100% of the calcium component of the 45S5 formulation for strontium[6], and have shown superior osteogenic capacity to 45S5 BG[7]. We hypothesize that melt-electrospun PCL/SrBG composite scaffolds will provide a promising bone graft substitute, which will be osteoinductive, osteoconductive, capable of osteointegration and bioresorbable in a controlled rate. The scaffolds can be manufactured with a tailored structure according to specific defect site, and can be replaced by the body’s own newly regenerated bone over time.
Materials and Methods: The SrBG particles were ground down to < 6 μm prior to composite preparation with a micronizing mill to reduce the risk of needle blockage. The 50 wt% PCL/SrBG composites were prepared by incorporating fine SrBG particles into the PCL bulk using solvent precipitation technique. PCL/SrBG composite scaffolds were fabricated using a novel solvent assisted hybrid melt-electrospinning technique in the direct write mode. Light microscopy and scanning electron microscopy (SEM) were used to characterize surface topography and fibre diameter. Mechanical stiffness of PCL/SrBG fibres were assessed with atomic force microscopy. Cell attachment and morphology were assessed with confocal laser scanning microscopy and SEM . Cell proliferation and osteoblastic differentiation were assessed with PicoGreen and alkaline phosphatase assays.
Results and Discussion: Our hybrid melt-electrospinning system overcame the difficulties of PCL/SrBG composite melt-electrospinning associated with viscoelastic properties of the composite material. These composite scaffolds were produced in a layer-by-layer manner with 90° cross-hatched deposition with the fibre spacing of 1 mm. Examination by scanning electron microscopy showed enhanced micro structure on the surface of PCL/SrBG fibres, while the surface of PCL only fibres were smooth. The submicron structures generated during the hybrid melt-electrospinning process largely increased the surface area of composite scaffolds and created roughness ideal for cell attachment. The in vitro results showed good cell adherence onto both the PCL/SrBG and PCL only scaffolds. However, PCL/SrBG composite scaffolds showed significantly higher osteoblast differentiation compared to PCL only scaffolds.
Conclusion: We are the first to produce the composite PCL/SrBG (50 wt%) scaffolds via a novel hybrid melt-electrospinning technique. These composite scaffolds are promising bone graft substitutes, and may also lead to patient-specific and off-the-shelf solution for clinical treatment of critical-sized bone defects.
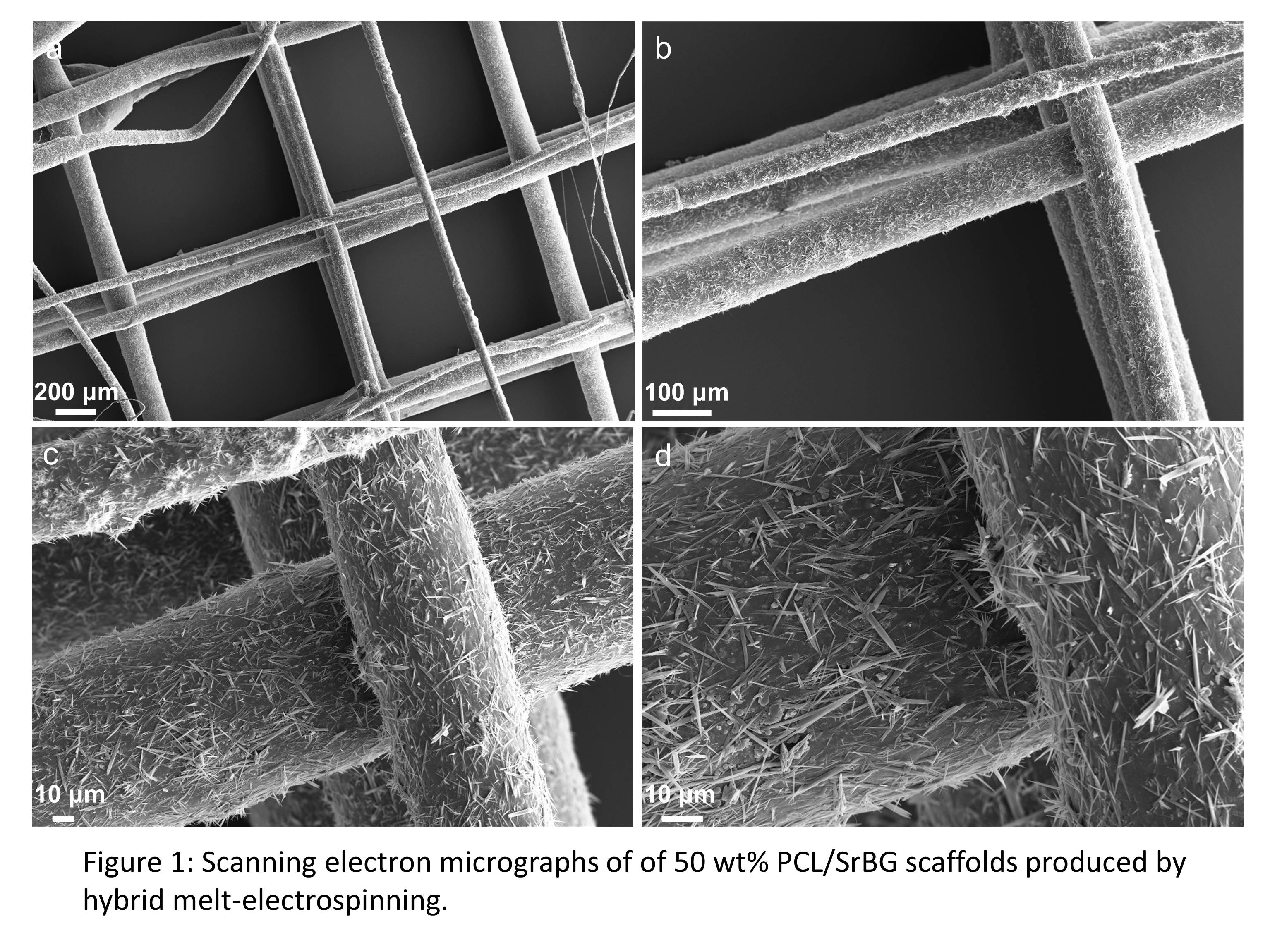
Dr Henrietta Cathey (Electron microscope probe analysis); Dr Charlotte Allen, Dr Sunny Hu and Mr. Mitchell De Bruyn (Inductively coupled plasma analysis); Dr Marie-Luise Wille (micro-CT scanning and analysis); Dr Christina Theodoropoulos (Confocal microscopy); Dr Tong Li (Atomic force microscopy); ARC Linkage for funding
References:
[1] Brown, T. D. et al. Melt electrospinning of poly(ε-caprolactone) scaffolds: Phenomenological observations associated with collection and direct writing. Mater. Sci. Eng. C (2014). doi:10.1016/j.msec.2014.07.034
[2] Muerza-Cascante, M. L., Haylock, D., Hutmacher, D. W. & Dalton, P. D. Melt Electrospinning and Its Technologization in Tissue Engineering. Tissue Eng. Part B. Rev. 00, 1–16 (2014).
[3] Rezwan, K., Chen, Q. Z., Blaker, J. J. & Boccaccini, A. R. Biodegradable and bioactive porous polymer/inorganic composite scaffolds for bone tissue engineering. Biomaterials 27, 3413–3431 (2006).
[4] Boccaccini, A. R. et al. Polymer/bioactive glass nanocomposites for biomedical applications: A review. Compos. Sci. Technol. 70, 1764–1776 (2010).
[5] Woodruff, M. A. & Hutmacher, D. W. The return of a forgotten polymer—Polycaprolactone in the 21st century. Prog. Polym. Sci. 35, 1217–1256 (2010).
[6] O’Donnell, M. D. et al. Materials characterisation and cytotoxic assessment of strontium-substituted bioactive glasses for bone regeneration. J. Mater. Chem. 20, 8934 (2010).
[7] Gentleman, E. et al. The effects of strontium-substituted bioactive glasses on osteoblasts and osteoclasts in vitro. Biomaterials 31, 3949–3956 (2010).
Keywords:
Bone Regeneration,
in vitro,
biomaterial,
ion release
Conference:
10th World Biomaterials Congress, Montréal, Canada, 17 May - 22 May, 2016.
Presentation Type:
General Session Oral
Topic:
Biomaterials in constructing tissue substitutes
Citation:
Ren
J,
Stevens
MM,
Steck
R,
Blackwood
KA and
Woodruff
MA
(2016). Developing strontium-substituted bioglass and polycaprolactone composite scaffolds for bone repair via hybrid electrospinning in the direct writing mode.
Front. Bioeng. Biotechnol.
Conference Abstract:
10th World Biomaterials Congress.
doi: 10.3389/conf.FBIOE.2016.01.00853
Copyright:
The abstracts in this collection have not been subject to any Frontiers peer review or checks, and are not endorsed by Frontiers.
They are made available through the Frontiers publishing platform as a service to conference organizers and presenters.
The copyright in the individual abstracts is owned by the author of each abstract or his/her employer unless otherwise stated.
Each abstract, as well as the collection of abstracts, are published under a Creative Commons CC-BY 4.0 (attribution) licence (https://creativecommons.org/licenses/by/4.0/) and may thus be reproduced, translated, adapted and be the subject of derivative works provided the authors and Frontiers are attributed.
For Frontiers’ terms and conditions please see https://www.frontiersin.org/legal/terms-and-conditions.
Received:
27 Mar 2016;
Published Online:
30 Mar 2016.