Introduction: Co-based alloys for biomedical applications are usually fabricated by investment casting method and exhibits inherent defects that are cause of concern and can lead to early implant failure[1]. In these alloys, the FCC and the HCP phases co-exist. Thus, there are efforts to obtain a single HCP phase Co-based alloys to exploit its mechanical properties[2]-[4]. The investigated Co- 20 wt. %Cr alloy was as-cast segregation-free and has 88 wt. % and 91 wt. % of ε-martensite in the as-cast and heat treated condition, respectively. The resultant alloy microstructures were characterized and tested for determinations of mechanical properties.
Materials and Methods: Co-20 wt. %Cr alloy was prepared in a vacuum induction furnace with argon atmosphere. The alloy was cast into a copper mold to achieve a cooling rate of 230K/s. The as-cast ingots were mechanically polished and etched using a 60 vol. % HNO3 + 40 vol. % H2O solution. Microstructures were observed with a scanning and transmission electron microscopes (JEOL 7600 and 1200 EX, respectively). X-ray diffraction was carried out using a Siemens D-5000 diffractometer with a Kα-Cu (1.5418 Å) radiation. Ingots sections were heat-treated at 1023.15 K (750°C) for 30, 60 and 180 minutes[5]. Mechanical properties were measured with an Instron 1210 at a travel speed of 0.5 mm/min.
Results: Microstructural features in the as-cast and heat treated condition (1023.15K for 60 min.) are shown in Figure 1a-b. Strain-stress curves and mechanical properties of alloy in both conditions are shown in Figure 2a and Table 1. The amount of ε-martensite was obtained from diffraction patterns employing the equation proposed by Sage and Guillaud[6] (Figure 2b).
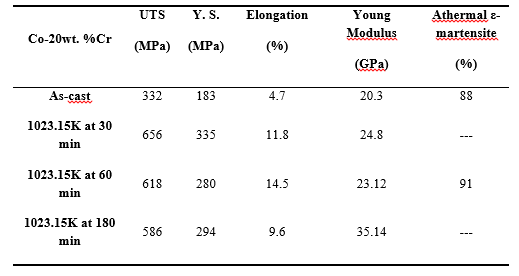
Discussion:
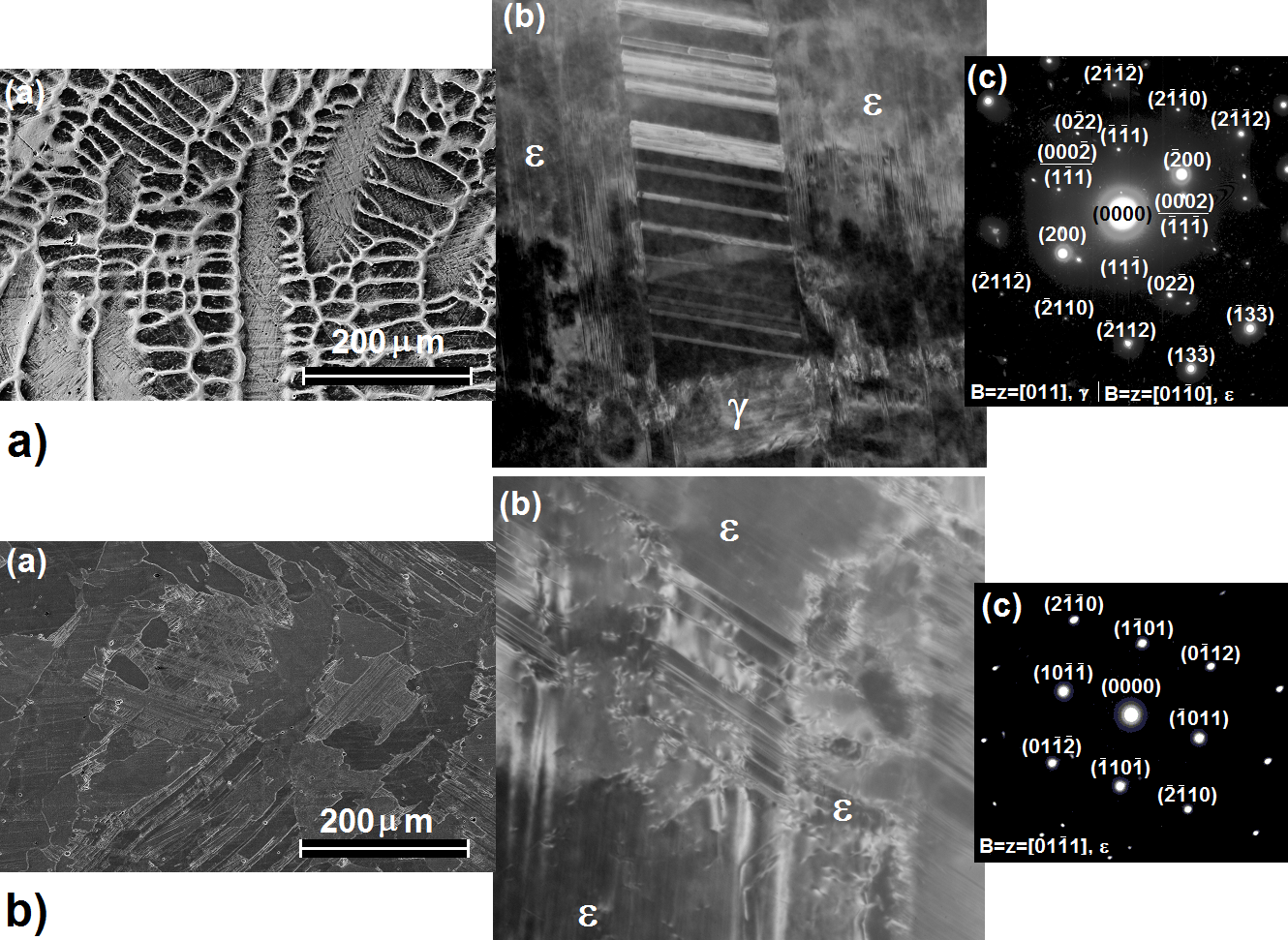
Figure 1a-(a) shown directionally solidified columnar dendrites in the as-cast condition with 88 wt.% of athermal ε-HCP phase. Fine plates of athermal ε-martensite inside columnar dendrites are shown in TEM micrographs (Fig. 1a-(b)) that are consistent with the HCP1 martensite reported by K. Rajan[7] its corresponding pattern is in Fig 1a-(c). For aged alloy, SEM and TEM micrographs (Fig. 1b-(a) and (b)) reveals a kind of recrystallization caused by excess vacancies movement, consequence of rapid solidification[8].
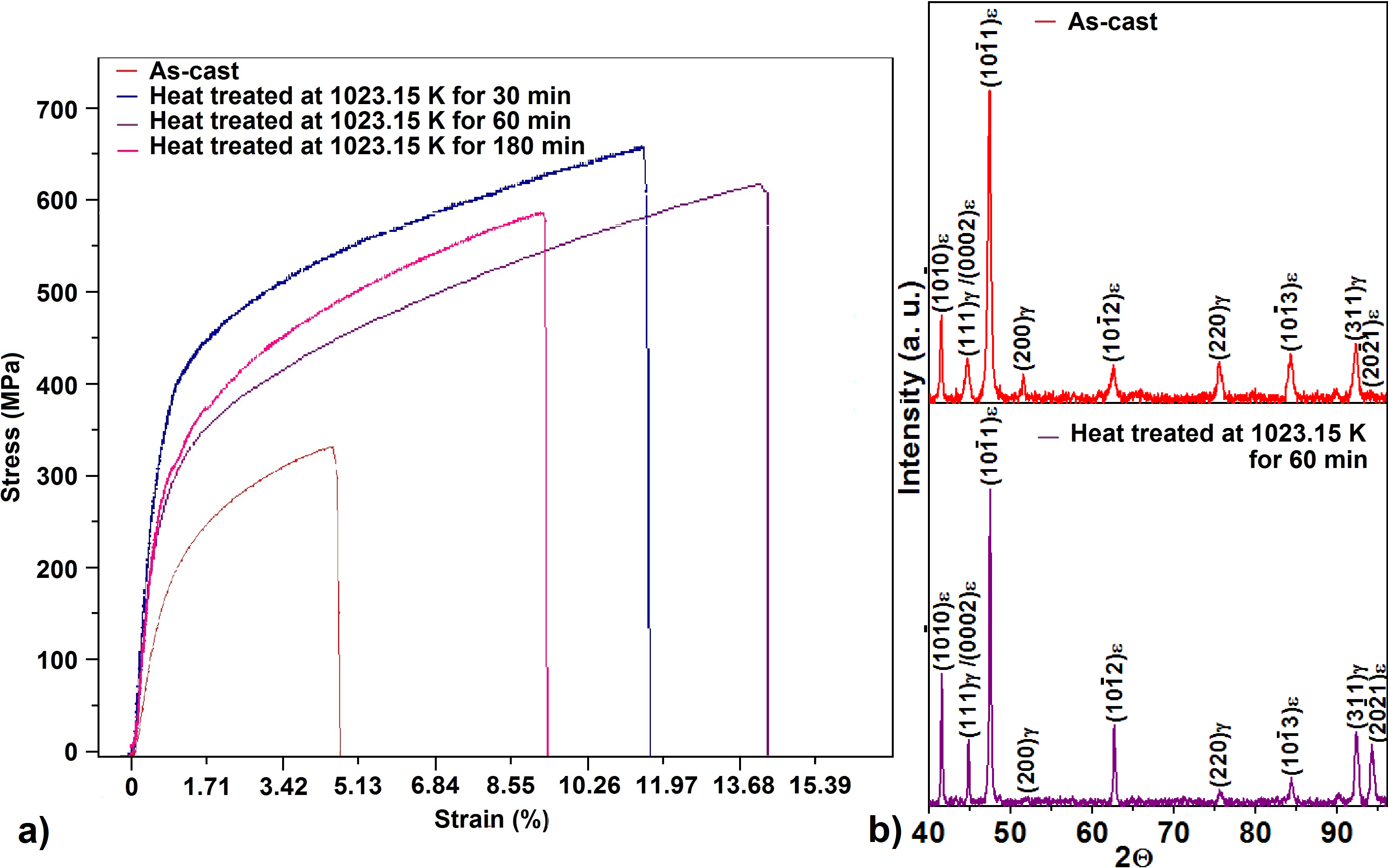
As-cast alloy exhibits yield and ultimate tensile strengths of 183 and 332, respectively, but an elongation of 4.7%. After isothermal alloy aging at 1023.15 K for 60 minutes, the mechanical properties exhibit a dramatic improvement (i.e. 280 MPa yield strength and a UTS of 618 MPa). This confirms that an increases of ε-martensite (from 88 to 91 wt. %) transformed isothermally and recrystallization (grain formation) after aging promotes an increment on the alloy mechanical properties[9]-[11]. Moreover, in this condition the balance between UTS, Young’s modulus and elongation percentage are better than the same properties for the alloy heat-treated at 1023.15K for 30 and 180 minutes as shown in Table 1 and Figure 2a.
Conclusions: In this work, an innovative solidification process is proposed to produce Co-Cr alloys with a high percentage of ε-martensite (HCP phase). In addition, we demonstrated that a heat treatment below martensitic transformation temperature improves significantly its mechanical properties.
A. Tejeda; C. Flores-Morales; C. Zorrilla; CONACYT
References:
[1] Kaiser R, Williamson K, O’Brien C, Browne DJ. Effects of section size, surface cooling conditions, and crucible material on microstructure and as-cast properties of investment cast Co-Cr biomedical alloy. Metall. Mater. Trans. A 2013; 44: 5333-5342
[2] Saldívar-García AJ, Maní-Medrano A, Salinas-Rodríguez A. Effect of solution treatments on the HCP/FCC isothermal martensitic transformation in Co-27Cr-5Mo-0.05C aged at 800°C. Scripta Mater. 1999; 40: 717-722
[3] Balagna C, Spriano S, Faga MG. Characterization of Co-Cr-Mo alloys after a thermal treatment for high wear resistance. Mater. Sci. Eng., C 2012; 32: 1868-1877
[4] Matsumoto H, Kurosu Sh, Lee BS, Li Y, Chiba A. Deformation mode in biomedical Co-27% Cr-5% Mo alloy consisting of a single hexagonal close-packed structure. Scripta Mater. 2010; 63: 1092-1095
[5] Taylor RNJ, Waterhouse RB. A Study of the Ageing Behavior of a Cobalt Based Implant Alloy. J Mater Sci 1983; 18: 3265-3280
[6] Sage M, Gillaud C. Méthode d'analyse quantitative des variétés allotropiques du Cobalt pre les Rayons X. Rev Metall 1950; 49: 139-145
[7] Rajan K. Phase transformations in a wrought Co-Cr-Mo-C alloy. Metall Mater Trans A 1982; 13: 1161-1166
[8] H Jones. “Rapid solidification of metals and alloys”. In: The institution of metallurgists. London;1982
[9] Pu S, Zhang J, Shen YF, Lou LH. Recrystallization in a directionally solidified cobalt-base superalloy. Mater. Sci. Eng., A 2008; 480: 428-433
[10] Saldívar-García AJ, Maní-Medrano A, Salinas-Rodríguez A. Formation of HCP martensite during the isothermal aging of an FCC Co-27Cr-5Mo-0.05C orthopedic implant alloy. Metall Mater Trans A 1999; 30: 1177-1184
[11] Zangeneh Sh, Lashgari HR, Saghafi M, Karshenas M. Effect of isothermal aging on the microstructural evolution of Co-Cr-Mo-C alloy. Mater. Sci. Eng., A 2010; 527: 6494-6500