Introduction: Low intensity pulsed ultrasound (LIPUS) has been shown to be clinically effective for fracture and non-union repair but its mechanism of action is poorly understood. We believe that LIPUS functions to heal bony defects partly through the application of low-level acoustic radiation force and have confirmed the existence of these forces. The focus of this work was to integrate the beneficial effects of LIPUS with hydrogel-based cell therapy for bone regeneration. Our goal was to develop a hydrogel-based cell therapy system in which hydrogel-encapsulated cells that are injected and retained within a bony defect, can then be mechanically loaded in situ via transdermally-applied acoustic radiation force (figure 1).
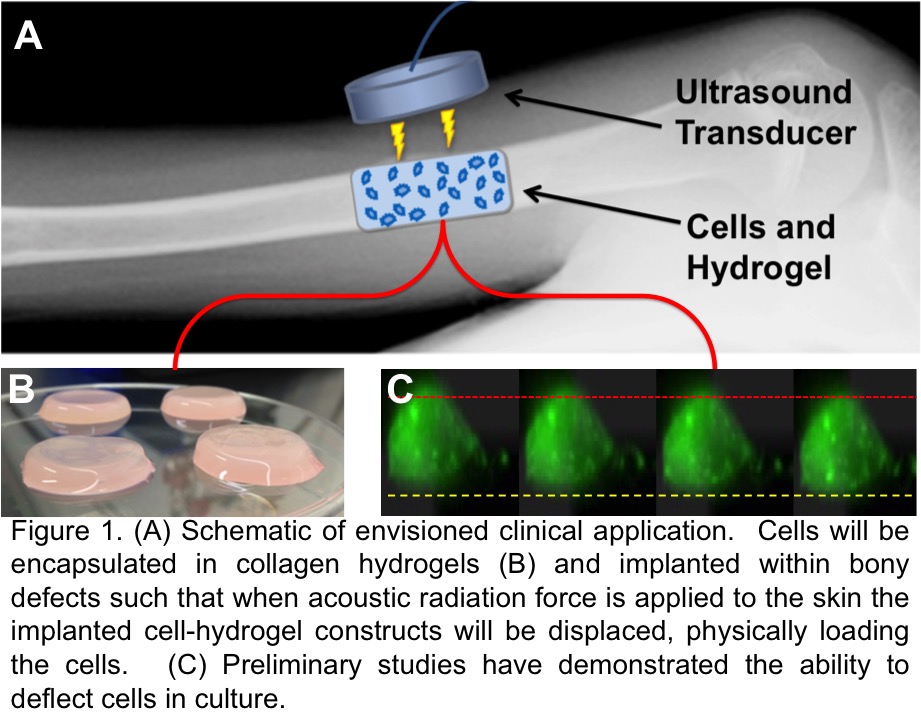
This would allow the encapsulated cells to be mechanically loaded immediately after implantation and throughout the healing process. Here we show the existence of that force, its impact on the hydrogel, and how that same force influences osteoblasts differently depending on the mechanical nature of the substrate.
Materials and Methods: Hydrogel Deformation: Ultrasound intensity was generated using a waveform generator through an RF power amplifier. A 1 MHz carrier frequency with a 1 kHz pulse repetition frequency, and either 20, 50 or 100% duty cycle were generated for output using an amplitude sweep in order to find the proper amplitude to produce a 30 mW/cm2 spatial intensity (clinically approved intensity for fracture healing).
Hydrogel deformation was measured to provide an indication of how cells would be loaded under LIPUS once encapsulated within the hydrogel. Deformation was mapped using encapsulated fluorescent beads whose movement was tracked 3-dimensionally in real time under LIPUS using epifluorescence microscopy with automated z-stack acquisition. Multiple images were captured and compiled, from which particle displacement was quantified and mapped.
Cell Studies: MC3T3-E1 osteoblasts were seeded onto tissue culture polystyrene (TCP) or type 1 collagen hydrogels and exposed to different LIPUS intensities for 20 minutes. Cells were cultured for 1 hour post-LIPUS and then stained for actin filament organization.
Results: Displacement of fluorescent beads encapsulated within hydrogels before, during, and after LIPUS exposure demonstrates the presence of a measurable force and how hydrogel deformation is dependent on both collagen content and LIPUS intensity.
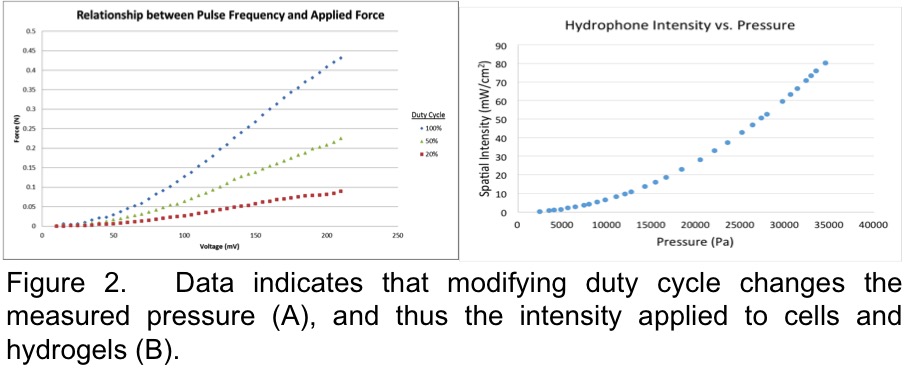
Cellular actin filament polymerization 1 hour after ultrasound application was more robust on 2-D TCP vs hydrogels, which may have absorbed the acoustic force vs transmitted it to cells, suggesting cells may benefit from stiffer gels. This also suggests that studying cells on rigid surfaces may not accurately reflect the clinical scenario.

Discussion: The focus of this work was to integrate hydrogel-based cell therapy for bone regeneration with the beneficial effects of ultrasound-induced acoustic radiation force. We have shown that low intensity pulsed ultrasound produces a measurable acoustic radiation force that can be manipulated to deflect hydrogels. The same force can influence the behavior of gel-encapsulated cells after short-term or long-term exposure, although the effect differs depending on the time. The system designed here is highly tunable by either changing the intensity of the impulse or by changing the composition of the hydrogel, each enabling a range of mechanical interactions, depending on the specific clinical need.
Research reported in this abstract was supported by the National Institute of Arthritis and Musculoskeletal and Skin Diseases of the National Institutes of Health under award number R21AR064432.; The content is solely the responsibility of the authors and does not necessarily represent the official views of the National Institutes of Health.