Introduction: Hyperbranched polymers (HBPs) are a subclass of the family of dendritic macromolecules with 3D globular nanostructures. Due to their unique properties (high solubility, low molecular entanglement, self-assembly, etc.), HBPs are of particular interest in diverse areas including drug delivery and tissue engineering [1]-[3]. When functionalized with biomolecules (drugs [4], ligands for cell targeting [5],etc.), HBPs can exhibit biological activity. Most bioactive HBPs, based on polyesters [6], polyphosphates [7], and polypeptides [8], have been synthesized by postgrafting strategies where biological molecules have been added or encapsulated in the final step. Hyperbranched polypeptides seem promising due to the specific biological responses that peptide sequences can elicit. However, existing HBPs are only based on a single amino-acid (i.e., polylysine [9]), and they do not exhibit any biological activity without postgrafting additional biological molecules. Here, we describe 2 new strategies allowing the synthesis of peptide-based HBPs as scaffolds for tissue engineering. The first strategy consists of postgrafting a peptide sequence (RGDS) on a HBP, and the second strategy is the direct polymerization of peptide-based monomers. Both strategies are expected to give peptide-based HBPs with different degrees of functionalization.
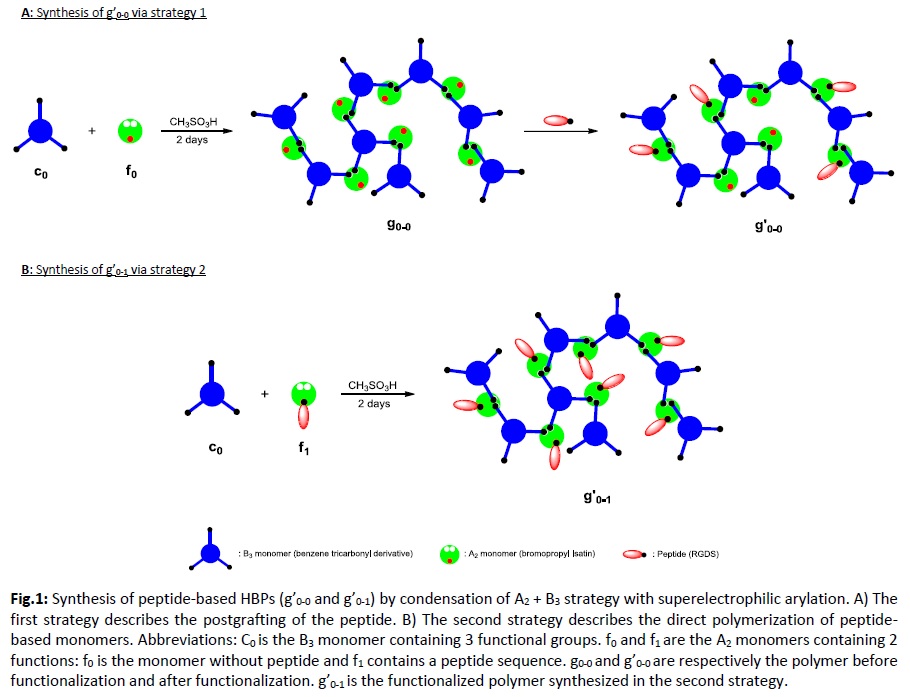
Methods:
Synthesis
Strategy 1 (Fig.1A): Inspired by the work of Smet et al.,[10] HBPs were synthesized by integration of the A2 (bromopropyl Isatin: f0) + B3 (benzene tricarbonyl derivative: c0) strategy with superelectrophilic arylation. The peptide, synthesized by SPPS, was then postgrafted on the HBP by nucleophilic substitution. The resulting HBP (g’0-0) was precipitated and characterized by GPC and NMR.
Strategy 2 (Fig.1B): The peptide sequence was incorporated in the A2 monomer during peptide synthesis, yielding a peptide-based isatin. Condensation of the peptide-based A2 + B3 was performed using the same conditions.
Biocompatibility assays
Samples were coated on cover slips, and biocompatibility assays was carried out using the 10T1/2 fibroblast cell line in standard culture conditions. The Live/Dead assay was used to evaluate cell viability.
Results and Discussion: Regarding the first strategy, condensation of A2 (f0) + B3 (c0) resulted in random highly branched structures (A2B3: g0-0) with high molecular weight (Mw = 10 321) and good polydispersity index (1.89). The peptide was then postgrafted, and the resulting HBP (g’0-0) had a higher molecular weight (Mw = 11 576; PDI = 1.73). The NMR spectrum of g’0-0 confirmed the modification by showing additional shifts missing in the NMR spectrum of g0-0 (Fig.2). Comparing films of this peptide-based HBP with those of the B3 monomer, we could clearly see that fibroblasts were able to grow on the HBP while, for the B3 monomer film, cells were growing only in the areas without any material meaning that the unfunctionalized monomer does not support cell attachment (Fig.3). For the second strategy, the HBP obtained had different solubility properties. Therefore, further studies are ongoing to isolate and characterize this HBP.
Conclusion: In this study, we developed a new strategy to synthesize a RGD-based HBP that supports cell growth. This new polymer is highly tunable and can be considered as new scaffold for tissue engineering.
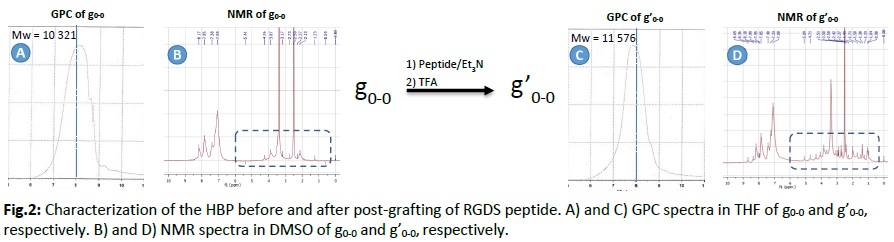
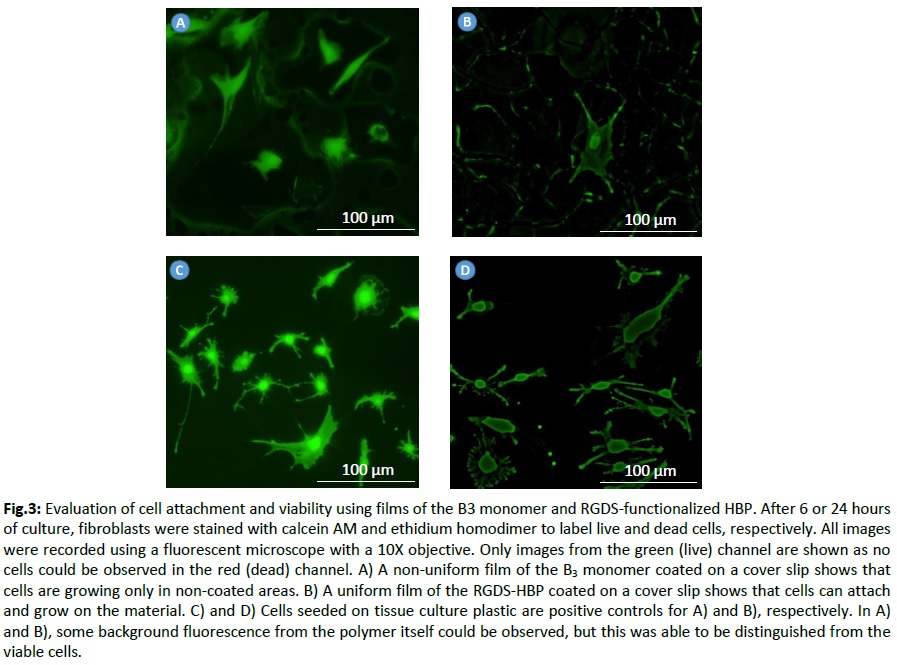
This work was supported by the special research fund of the KU Leuven, grant number CREA/13/017.; This work is part of Prometheus, the R&D Division of Skeletal Tissue Engineering of the KU Leuven: http://www.kuleuven.be/prometheus.; We acknowledge Dr. Susanna Piluso and Abhijith Kudva for assistance with cell culture and microscopy.
References:
[1] Gao, C. & Yan, D. Prog. Polym. Sci. 29, 183–275 (2004).
[2] Wang, D., Zhao, T., Zhu, X., Yan, D. & Wang, W. Chem. Soc. Rev. 44, 4023–4071 (2015).
[3] Zhou, Y., Huang, W., Liu, J., Zhu, X. & Yan, D. Adv. Mater. 22, 4567–4590 (2010).
[4] Jiang, W., Zhou, Y. & Yan, D. Chem Soc Rev 44, 3874–3889 (2015).
[5] Carlmark, A., Hawker, C., Hult, A. & Malkoch, M. Chem. Soc. Rev. 38, 352–362 (2009).
[6] Zhang, H., Patel, A., Gaharwar, A., Mihaila, S., Iviglia, G., Mukundan, S., Bae, H., Yang, H., Khademhosseini, A.,. Biomacromolecules, 14 (5), pp 1299–1310 (2013).
[7] Liu, J., Huang, W., Pang, Y. & Yan, D. Chem Soc Rev 44, 3942–3953 (2015).
[8] Chang, C. and Dong, C., Biomacromolecules, 14 (9), pp 3329–3337 (2013).
[9] Kadlecova, Z., Baldi, L., Hacker, D., Wurm, F. M. & Klok, H. Biomacromolecules 13, 3127–3137 (2012).
[10] Smet, M., Fu, Y., Zhang, X., Schacht, E. H. & Dehaen, W. Rapid Commun. 26, 1458–1463 (2005).