Introduction: Extracellular vesicles (EVs) in bodily fluids are promising novel cancer biomarkers because they carry molecular information about the origin cells[1,][2]. However, rapid analysis of EVs is challenging, and hence conventional detection methods, such as Western blot and enzyme-linked immunosorbent assays, require large sample volumes and long detection times. For point-of-care (POC) diagnosis, the microchip-based analytical system has attracted attention because of its cost effectiveness, i.e., small sample sizes with short analysis times. In RIKEN, various biomarker detection methods on the power-free poly(dimethylsiloxane) (PDMS) microchip[3] have been developed[4],[5]. The power-free technique eliminates the need for an external power source for supplying the sample solution into the microchannels and makes the microchip portable.
Recently, we proposed a graft-type PDMS microchip, in which the inner surface of the microchip was chemically modified by electron beam-induced graft polymerization (EIGP), as a platform for analysis. Unlike other grafting techniques, EIGP enables simple surface modification of PDMS without initiator immobilization or monomer purification, and is applicable to the power-free PDMS microchip. In this study, we apply the technique and prepare a novel portable graft-type PDMS microchip that enables rapid and easy EV detection toward POC cancer diagnosis.
Materials and Methods: Microchip fabrication: The graft-type power-free microchip was prepared as follows: First, a PDMS component with a pair of Y-shaped microchannels (100 μm width, 25 μm height) was fabricated. The left and right Y-shaped microchannels are for detection of the target EV and for a control blank, respectively. Next, poly(glycidyl methacrylate) (PGMA) was grafted onto the surface of the microchannels by EIGP. Lastly, anti-human CD63 antibody was immobilized on the surface and the PDMS component was attached onto the glass slide. Finally, the microchip was degassed.
EV isolation: One mammary epithelial cell line (MCF10A) and three breast cancer cell lines (MCF7, MDA-MB-453, MDA-MB-231) were cultured. From the supernatants of these cell lines, EVs were isolated by differential ultracentrifugation following a previously reported protocol[6].
Results and Discussion: Autofluorescence of irradiated PDMS increased on increasing the dose of the electron beam. For GMA polymerization, a dose of 20 kGy was selected to reduce autofluorescence noise during EV detection. Isolated EVs were confirmed and characterized by dynamic light scattering (DLS) and using a nanoparticle tracking device (NanoSight). Anti-CD63 antibody was immobilized for EV capturing. The amount of anti-CD63 antibody immobilized on the surface of the microchannels was evaluated by fluorescent-labeled secondary antibody adsorption. After preparation of the anti-CD63 antibody-immobilized graft-type microchip, EVs were detected after the following three steps: (1) blocking, (2) capture of EV and immobilization of biotinylated anti-CD63 antibody onto the EV, (3) fluorescence signal amplification by laminar flow-assisted dendritic amplification[7] (Fig. 1). The solutions were injected into the grafted microfluidic device by power-free sequential injection. With increasing amplification time, a bright line appeared at the expected position; it appeared only on the border between the amplification reagents in the left microchannel in which the target EV had been supplied (Fig. 2). The required sample volume was 0.5 μL and the total analysis time was 20 min.
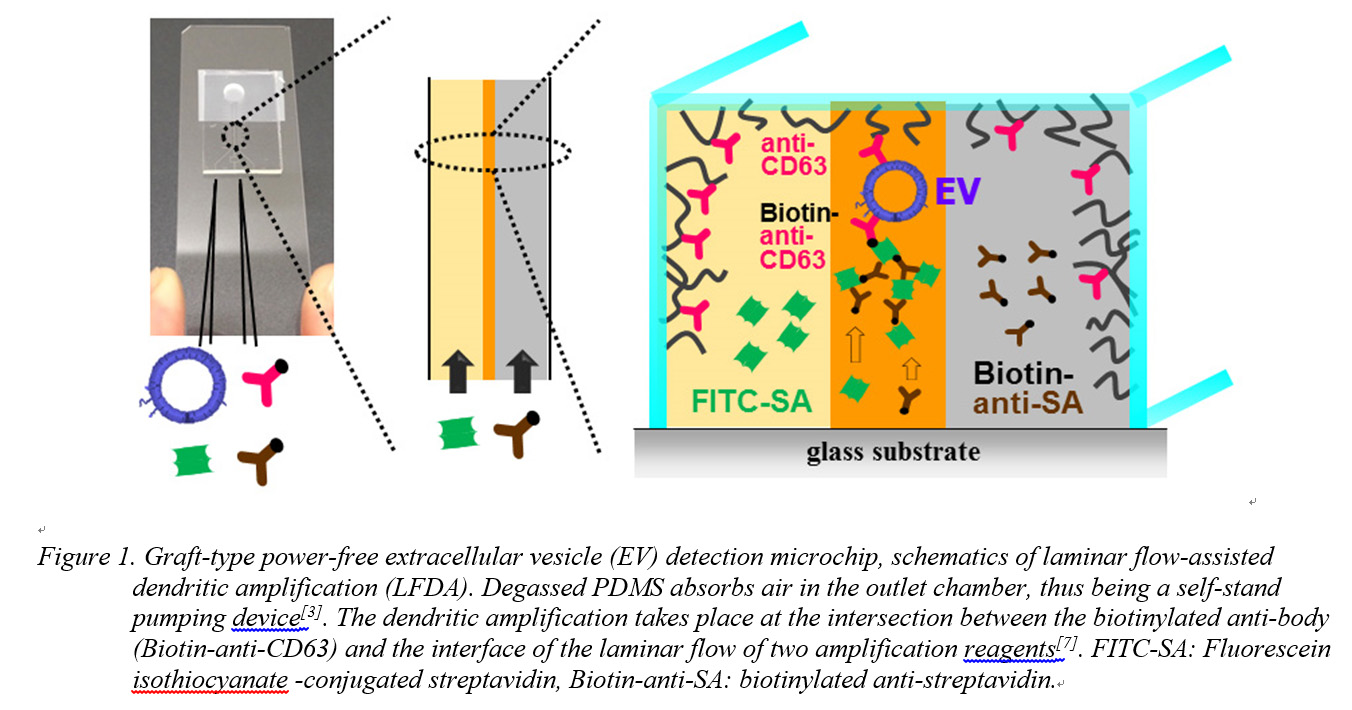
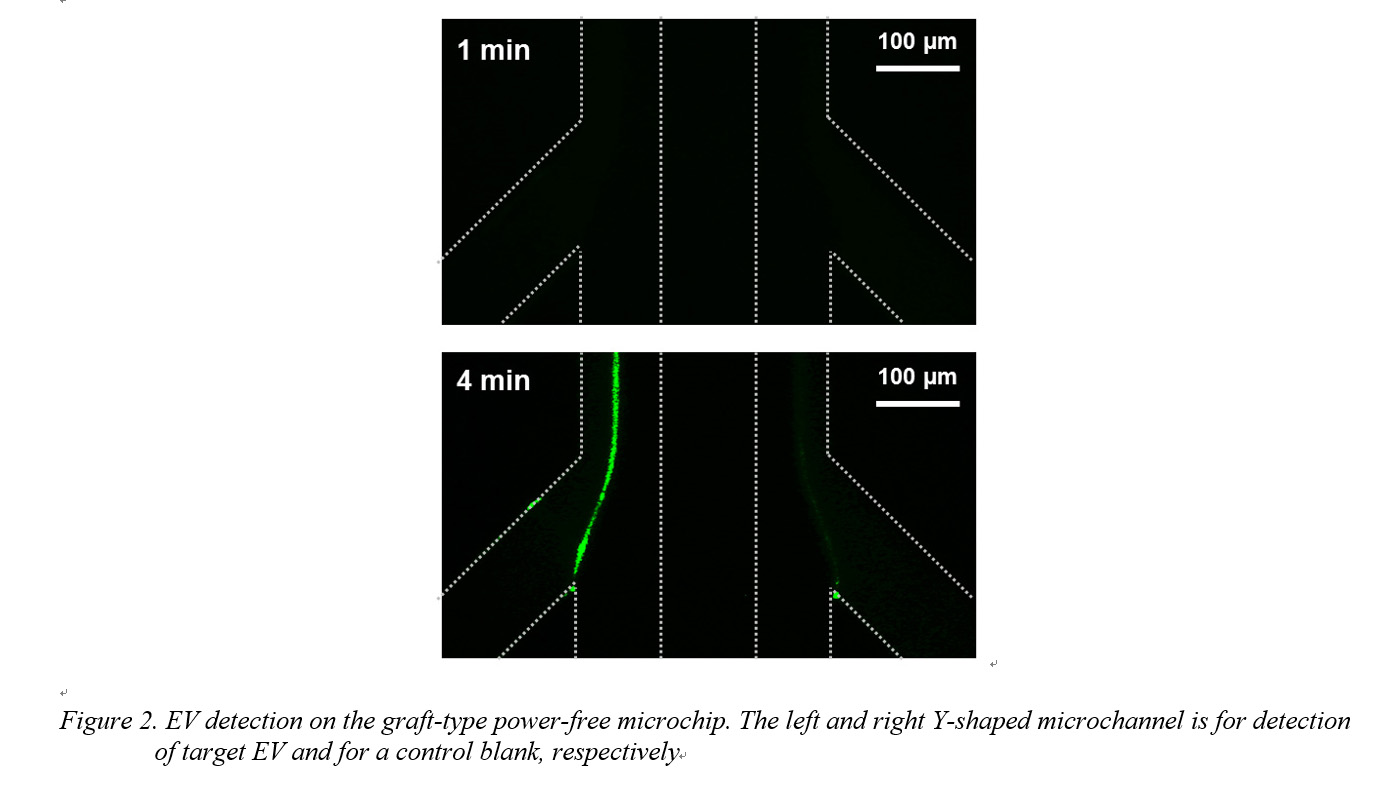
Conclusions: We proposed a simple inner-surface modification method utilizing EIGP and applied the method to a power-free microchip. The graft-type power-free PDMS microchip demonstrated EV detection from a 0.5 µL sample solution within 20 min. Since most existing methods take more than a few hours, the rapidity, small sample volumes, and device portability of this method are advantageous for POC cancer diagnosis.
This work was partly supported by KAKENHI (25871118).; We thank prof. Kohei Soga and Dr. Masao Kamimura at Tokyo University of Science for advising DLS data acquisition.
References:
[1] H. Im, H. Shao,Y. I. Park, V. M. Peterson, C.M. Castro, R. Weissleder, and H. Lee, Nat. Biotechnol., 32, 490-495, 2014.
[2] Y. Yoshioka, N. Kosaka, Y. Konishi, H. Ohta, H. Okamoto, H. Sonoda, Y. Nonaka, H. Yamamoto, H. Ishii, M. Mori, K. Furuta, T. Nakajima, H. Hayashi, H. Sugisaki, H. Higashimoto, T. Kato, F. Takeshita, and T. Ochiya, Nat. Commun., 5, 3591, 2014.
[3] K. Hosokawa, K. Sato, N. Ichikawa, M. Maeda, Lab Chip, 4, 181-185, 2004.
[4] H. Okada, K. Hosokawa, M. Maeda, Anal. Sci., 27, 237-241, 2011.
[5] R. Ishihara, K. Hasegawa, K. Hosokawa, M. Maeda, Anal. Sci., 31, 573-576, 2015.
[6] C. Thery, S. Amigorena, G. Raposo, A. Clayton, Current Protocols in Cell Biology, 3.22 Isolation and Characterization of Exosomes from Cell Culture Supernatants and Biological Fluids, John Wiley & Sons, Ltd: New York, 2006.
[7] K. Hosokawa, M. Omata, M. Maeda, Anal. Chem., 79, 6000-6004, 2007.