- 1Department of Pharmacy, Huadu District People’s Hospital, Southern Medical University, Guangzhou, China
- 2Department of Gastroenterology, Huadu District People’s Hospital, Southern Medical University, Guangzhou, China
Icariin is a major bioactive pharmaceutical constituent isolated from Chinese medicine Horny Goat Weed (Ying Yang Huo) with potent cardiovascular protective functions. Emerging evidence in the past decade has shown that Icariin possesses multiple atheroprotective functions, through multiple mechanisms, including attenuating DNA damage, correcting endothelial dysfunction, inhibiting the proliferation and migration of smooth muscle cells, repressing macrophage-derived foam cell formation and inflammatory responses, as well as preventing platelet activation. All of these protective effects, combined with its lipid-modulatory effects, contribute to the broad atheroprotective effects of Icariin. In this review, we will summarize the anti-atherosclerotic properties of Icariin and highlight future perspectives in developing Icariin as a promising anti-atherosclerotic drug.
Introduction
Cardiovascular diseases (CVDs) represent one of the leading causes of death in the modern society worldwide (Benjamin et al., 2017). At present, due to the complexity of the pathogenesis of atherosclerosis, therapeutic drugs that can prevent or treat atherosclerosis remain very limited (Orekhov et al., 2015). Currently, in light of the fact that atherosclerosis is a lipid disorder; lipid-lowering statins are the main category of anti-atherosclerotic drugs. Recently, several new therapeutic options are showing promising prospect in treating atherosclerosis, such as PCSK9 monoclonal antibodies (Pedersen, 2016). Despite the wide application of statins, the mortality rate of CVD remains very high, suggesting the need to develop new drugs that can treat atherosclerosis.
Numerous studies have shown that atherosclerosis is a chronic inflammatory disease with the characteristics of persistent inflammatory response, inflammasome activation, and impaired inflammation resolution (Ross, 1993; Rader and Daugherty, 2008; Libby et al., 2011; Tabas et al., 2015). Many vascular cells, including endothelial cells, smooth muscle cells, monocytes, macrophages, and platelets are involved in the inflammatory responses of vessel wall in response to pro-atherogenic stimuli (Rader and Daugherty, 2008). It has been reported that the current “gold standard” anti-atherosclerotic drugs such as statins can also produce anti-inflammatory effects independent of lipid-lowering effects (Rosenson, 2004). Therefore, anti-inflammatory therapy is a good strategy for the prevention and treatment of atherosclerosis. As we are entering a golden era of developing drugs from natural products (Shen, 2015), natural products especially Chinese medicinal herbs emerges as important sources of cardiovascular protective medicine that target multiple cellular processes of atherosclerosis. These natural products would probably translate into effective novel cardiovascular therapeutics (Amirkia and Heinrich, 2015; Fang et al., 2017).
Horny Goat Weed, an eminent Chinese herbal medicine (also known as Epimedii Herba or Ying Yang Huo), is widely used to treat various diseases such as coronary heart disease, impotence and osteoporosis, and rheumatism (Ye and Chen, 2001). Among the pharmacologically active constituents, Icariin (Figure 1A) is a major bioactive pharmaceutical constituent that has been reported to treat multiple CVDs (He et al., 1995; Xu and Huang, 2007) via anti-oxidant, anti-inflammatory, and lipid-modulatory effects. The excellent cardiovascular protective profiles of Icariin make it an excellent drug candidate for cardiovascular therapeutics. In the following sections, we will present an overview of the cardiovascular protective effects and molecular mechanisms of Icariin in atherosclerosis.
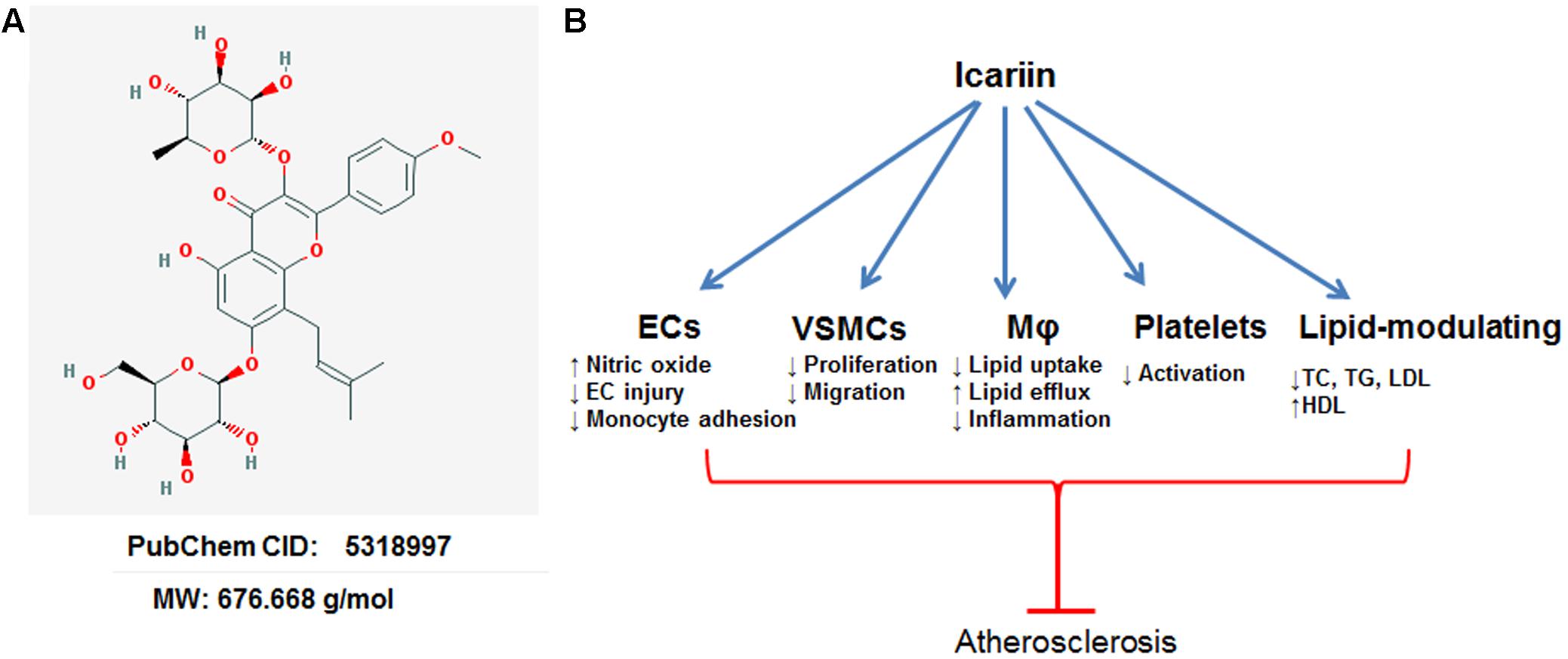
FIGURE 1. Anti-atherosclerotic effects of Icariin. EC, endothelial cell; VSMC, vascular smooth muscle cell; Mφ, macrophage. (A) The chemical structure of Icariin obtained from PubChem database. (B) Atheroprotective effects of Icariin.
Anti-Atherosclerotic Effects of Icariin
Previous studies have suggested that Icariin attenuated the development of atherosclerosis in several animal models, including ApoE-/- mice (Xiao et al., 2015, 2017; Wang et al., 2016), rats (Hu et al., 2015b), and rabbits (Zhang et al., 2013). The atheroprotective mechanisms of Icariin are exerted through anti-oxidative, anti-inflammatory, anti-thrombotic, and lipid-modulatory effects. Cellular models are very useful in evaluating the therapeutic effects of disease-modulating drugs in cultured cell systems (Orekhov et al., 2015). Icariin has demonstrated eminent protects against atherosclerosis in multiple cellular models, including: endothelial dysfunction, macrophage-derived foam cell formation, aberrant proliferation and migration of smooth muscle cells and platelet activation (Figure 1B).
Anti-DNA Damage Effects
The generation of reactive oxygen species (ROS) contributes to the development of many diseases, including atherosclerosis. Icariin has polyphenol structure which shares similar antioxidant properties as other polyphenols. Liu et al. (2004) evaluated the antioxidative effects of icariin on free-radical [AAPH, 2,2′-azobis(2-amidinopropane hydrochloride)]-induced hemolysis of erythrocytes. The authors observed that Icariin has protective effects against free-radical-induced peroxidation in erythrocytes, although much weaker than its structural analogs norwogonin and acacetin. Zhao et al. (2007) followed to examine the anti-oxidant effects of Icariin in a cell-free system and the authors found that Icariin reduced AAPH-induced DNA damage. The observed anti-DNA damage effects could contribute to the reduced DNA damage marker γ-H2AX expression and lifespan-extension effect by Icariin in C57BL/6J mice (Zhang et al., 2015; Shen et al., 2017).
Preventing Endothelial Dysfunction
The endothelium is important in keeping the health and function of the vessel since it is the direct cell type that closely contacts the circulating blood. Main functions of endothelium include the regulation of vascular tone, barrier function, anti-oxidative, anti-inflammatory, and anti-thrombotic status (Gimbrone and Garcia-Cardena, 2016). However, endothelial function impairment by pathological stimuli causes endothelial dysfunction, an initiating event in the development of atherosclerosis. Endothelial dysfunction is a complex and broad term that encompass several aspects, such as impaired NO production, eNOS uncoupling, monocyte adhesion to endothelial cells, endothelial senescence, and apoptosis, etc. (Xu et al., 2016b). In terms of NO production, endothelium is the major cell type that produces NO through the endothelium nitric synthase (eNOS) pathway. In this regard, Icariin protects against H2O2 induced apoptosis in endothelial cell line ECV304 by decreasing the activation of caspase 3 and boosting the production of vasodilatory NO derived from eNOS (Wang and Huang, 2005). Further studies showed that Icariin increases the production of NO by activating PI3K/pAkt/p-eNOS (S1177) and EGF/EGFR pathways (Chung et al., 2008; Liu et al., 2011). In agreement with this finding, another study congruently shows that androgen receptor (AR), PI3K/Akt, and ERK pathway are involved in increased release of NO via eNOS activation (Koizumi et al., 2010). Also, through the Akt/eNOS/NO pathway, Icariin prevents endothelial senescence induced by homocyteine both in vitro and in vivo (Xiao-Hong et al., 2013) as well as s-nitrosogultathione induced endothelial apoptosis (Huang et al., 2016). In addition to the upregulation of eNOS phosphorylation at Ser1177, Icariin also increases eNOS mRNA and protein expression, suggesting Icariin could also regulate eNOS level at the transcriptional and translational level (Xu and Huang, 2007). Furthermore, Icariin also increase eNOS activity by blocking eNOS uncoupling through decreasing the level of ROS and asymmetric dimethylarginine (ADMA) by increasing the level of dimethylarginine dimethylaminohydrolase (DDAH) in ApoE-/- mice. The resulted effect is increasing endothelium-dependent vasorelaxation (Xiao et al., 2015). Recent evidence has also suggested that Icariin reduces oxLDL-induced monocyte adhesion to endothelial cells, the initiating event in atherosclerosis. The mechanism is linked to decreasing the expression of pro-adhesive cytokines ICAM-1, VCAM-1 and E-selectin, probably through NF-κB pathway (Hu et al., 2015a). In terms of the important role of SIRT6 in regulating endothelial dysfunction and atherosclerosis (Liu et al., 2016; Xu et al., 2016a,c; Zhang et al., 2016), Icariin has recently been reported as a SIRT6 pharmacological activator in vitro and in vivo (Chen et al., 2015), pinpointing the potential contribution of SIRT6 activation to the atheroprotective effects of Icarrin. The endothelial protective effects of Icariin contributed to upregulation of eNOS-dependent NO production in ApoE-/- mice fed a high fat diet (Xiao et al., 2017).
Inhibiting the Proliferation and Migration of Smooth Muscle Cells
After endothelial injury, the vascular smooth muscle cells (VSMCs) will migrate from the media layer to the sub-endothelial space and proliferate very quickly, forming the neointima, the early form of vascular remodeling associated with atherosclerosis. Markers for VSMC proliferation include proliferating cell nuclear antigen (PCNA), Ki67 and cell cycle progression. A recent study by Hu colleagues has shown that Icariin dose-dependently (10–40 μM) suppressed oxidized LDL induced proliferation of VSMCs by inhibiting the ERK1/2 pathway and PCNA expression. Icariin treatment also arrests cell cycle at S and G2/M phase (Hu et al., 2016). Accelerating the death of lesional smooth muscle cells is another way of inhibiting the proliferation and migration of VSMCs. In this regards, Icariin decreases VSMC turnover by promoting the apoptosis through glucose regulated protein 78 (GRP78) in homocysteine-treated VSMCs (Shen and He, 2009). All these effects contribute to Icariin-mediated anti-proliferative effects in VSMCs.
Inhibiting Inflammation and Macrophage-Derived Foam Cell Formation
Inflammation is a key process involved in all phases of atherosclerosis (Ross, 1993; Rader and Daugherty, 2008; Libby et al., 2011; Xu et al., 2014a,b; Tabas et al., 2015). Icariin has been shown to inhibit inflammation in lipopolysaccharide (LPS)-stimulated macrophages by suppressing the expression of TNFα, COX2, iNOS, and MPO activity (Chen et al., 2010; Xu et al., 2010) through activating PI3K/Akt pathway and inhibiting p38 MAPK and NF-κB p65 pathways. Transcriptome profiling is a useful method to dissect the molecular mechanisms of pharmaceutical compounds (Xu, 2017). By using transcriptomic profiling, a recent study has shown that Icariin reduces the expression of pro-migratory chemokine CX3CR1 in LPS-stimulated macrophages as well as CX3CL1 induced macrophage chemotaxis (Wang et al., 2016). As a result, Icariin significantly reduces macrophage infiltration in aortic plaques from ApoE-/- mice fed a high fat diet (Wang et al., 2016).
In addition to inflammation in macrophage, macrophage-derived foam cell formation is the hallmark of atherosclerosis. It is a complex cellular event determined by the balance of cholesterol uptake and efflux (Liu et al., 2015). Both cholesterol uptake and efflux are mediated by several scavenger receptors and transporters. Specifically, cholesterol uptake is mediated by CD36, SRA and LOX-1, whole efflux is mediated by ABCA1, ABCG1, and SRBI (Xu et al., 2013). The intracellular level of cholesterol is determined by the net effect of both processes. In this regard, Icariin has been shown to reduce CD36 expression and upregulate SRBI expression through p38 MAPK pathway in macrophages stimulated with LPS and oxidized LDL (Yang et al., 2015).
Icariin Modulates Lipid Metabolism
In a rat model of atherosclerosis by feeding a high cholesterol diet and Vitamin D3, 4 weeks of treatment with Icariin (30 and 60 mg/kg/d, intragastric administration) significantly decreased the levels of TC, TG, LDL-C, while increase that of HDL-C (Hu et al., 2015b). In ApoE-/- mice fed a high fat diet, Icariin reduces atherosclerosis by reducing the concentrations of TC and TG (Xiao et al., 2017). Icariin also reduced the levels of serum TC and LDL-C, as well as the atherosclerotic burden in rabbits (Zhang et al., 2013). These findings suggest the broad lipid-modulatory effects of Icariin are accountable for its atheroprotective effects observed in animal models.
Inhibiting Platelet Activation
Platelet activation and ensuing thrombus formation is a key event in the late stage of atherosclerosis, and drugs that inhibit platelet activation are promising candidates of treating atherosclerosis. Icariin has been found to improve the imbalance between plasminogen activator inhibitor-1 (PAI-1) and tissue-type plasminogen activator (t-PA) activities, thereby reducing platelet activation in hypercholesterolemia in a rabbit model of atherosclerosis (Zhang et al., 2013).
Potential Molecular Targets of Icariin
Icariin has potent anti-oxidative, anti-inflammatory, anti-proliferative, anti-thrombotic, and lipid-modulatory effects in vascular cells. The potential molecular targets are summarized in Figure 2. (i) Molecular targets of anti-oxidant effects: icariin has direct free-radical scavenging effects, which constitute the basis for its preventive effects against DNA damage. Another potential anti-oxidant mechanism could be the activation of Nrf2 in light of the recent observation (Wu et al., 2014) showing that Icariin derivative Icaritin activates Nrf2 in cigarette smoke extract treated-human lung epithelial cells. Further studies are needed to support the Nrf2 activation effects of Icariin in vascular cells; (ii) molecular targets of anti-inflammatory effects: inflammation in vascular cells, macrophage in particular, is mainly driven by transcriptional factor NF-κB (Wu et al., 2010). Icariin has been demonstrated to reduce the expression of COX2, iNOS, and CX3CR1 (Chen et al., 2010; Xu et al., 2010), which are NF-κB direct target genes. Therefore, inhibition of NF-κB could be the primary target of Icariin-mediated anti-inflammatory effects; (iii) molecular targets of anti-proliferative effects: the anti-proliferative targets of Icariin are PCNA (Hu et al., 2016) and GRP78 (Shen and He, 2009); (iv) molecular targets of anti-thrombotic effects: icarrin exhibits protective effects against platelet activation, and the molecular targets are pro-thrombotic PAI-1 and anti-thrombotic t-PA (Zhang et al., 2013); (v) molecular targets of lipid-modulatory effects: the molecular mechanisms of Icariin in modulating lipid metabolism has not been fully explored. Previous studies in experimental animal models have suggested that Icariin reduces bad LDL-cholesterol, while increase good HDL-cholesterol (Hu et al., 2015b). The potential mechanisms could be twofold. On the one hand, Icariin could inhibit cholesterol synthesis and uptake via sterol regulatory element-binding protein (SREBP) (Han et al., 2016) and scavenger receptor (CD36) (Yang et al., 2015), respectively. On the other hand, Icariin could promote SR-BI-mediated cholesterol efflux (Yang et al., 2015) and facilitate cholesterol excretion. The potential effects of Icariin on HDL biogenesis warrant further studies.
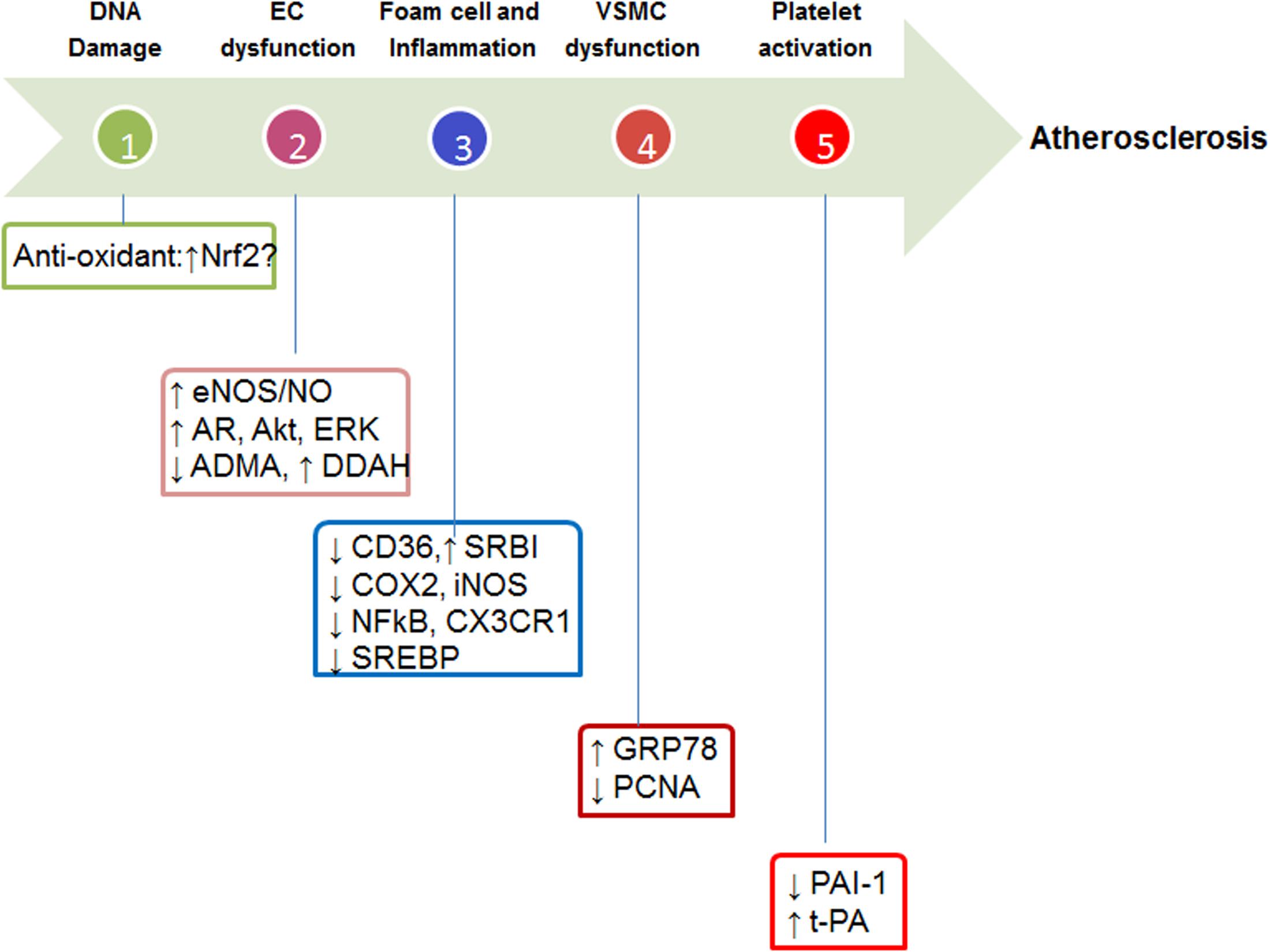
FIGURE 2. Potential molecular targets of Icariin. Nrf2, eNOS, endothelial nitric oxide synthase; NO, nitric oxide; AR, androgen receptor; ADMA, asymmetric dimethylarginine; DDAH, dimethylarginine dimethylaminohydrolase; CD36, cluster of differentiation 36; SR-BI, scavenger receptor B1; COX2, cyclooxygenase 2; iNOS, inducible NO synthase; NF-κB, nuclear factor-kappa B; CX3CR1, SREBP, sterol regulatory element-binding protein; GRP78, glucose regulated protein 78; PCNA, proliferating cell nuclear antigen; PAI-1, plasminogen activator inhibitor-1; t-PA, tissue-type plasminogen activator.
Concluding Remarks and Future Perspective
Emerging evidence from the past several years has indicated that Icariin is a promising naturally occurring compound that possesses multiple atheroprotective functions. The molecular mechanism involves anti-inflammatory, anti-oxidative and lipid-modulatory effects. Since Icariin is identified as a PDE5 inhibitor (Xin et al., 2003) and SIRT6 activator (Chen et al., 2015), it remains to be investigated in future whether the atheroprotective actions of Icariin are dependent on PDE5 inhibition and SIRT6 activation. There are also several aspects that need to be investigated in future studies: (1) the effect of Icariin on VSMC plasticity, i.e., the phenotypic switch from contractile to synthetic phenotype; (2) the effect of Icariin on macrophage transmigration to endothelium, the key initiating events in atherosclerosis; (3) the effect of Icariin on macrophage polarization, i.e., M1 to M2 switch; (4) Icariin has broad lipid-modulatory effects, while, the effects of Icariin on hepatic cholesterol synthesis and metabolism remains to be determined; (5) Icariin derivatives has showed potent anti-inflammatory effects in macrophages (Chen et al., 2010), it is also important to design and test the therapeutic efficacy of Icariin derivatives in treating atherosclerosis in experimental models. With the help from biotechnological advances such as RNA-sequencing, transcriptomic studies in Icariin treated vascular cells under disease settings will help identify novel therapeutic targets of Icariin. Further understanding of mechanism of action of Icariin will help us gain a comprehensive understanding of the anti-atherosclerotic profile of Icariin.
Author Contributions
JF and YZ contributed to the conception, drafting, and revision of the manuscript and approved the final version.
Conflict of Interest Statement
The authors declare that the research was conducted in the absence of any commercial or financial relationships that could be construed as a potential conflict of interest.
References
Amirkia, V., and Heinrich, M. (2015). Natural products and drug discovery: a survey of stakeholders in industry and academia. Front. Pharmacol. 6:237. doi: 10.3389/fphar.2015.00237
Benjamin, E. J., Blaha, M. J., Chiuve, S. E., Cushman, M., Das, S. R., Deo, R., et al. (2017). Heart disease and stroke statistics-2017 update: a report from the American Heart Association. Circulation 135, e146–e603. doi: 10.1161/CIR.0000000000000485
Chen, S. R., Xu, X. Z., Wang, Y. H., Chen, J. W., Xu, S. W., Gu, L. Q., et al. (2010). Icariin derivative inhibits inflammation through suppression of p38 mitogen-activated protein kinase and nuclear factor-kappaB pathways. Biol. Pharm. Bull. 33, 1307–1313. doi: 10.1248/bpb.33.1307
Chen, Y., Sun, T., Wu, J., Kalionis, B., Zhang, C., Yuan, D., et al. (2015). Icariin intervenes in cardiac inflammaging through upregulation of SIRT6 enzyme activity and inhibition of the NF-kappa B pathway. BioMed Res. Int. 2015:895976. doi: 10.1155/2015/895976
Chung, B. H., Kim, J. D., Kim, C. K., Kim, J. H., Won, M. H., Lee, H. S., et al. (2008). Icariin stimulates angiogenesis by activating the MEK/ERK- and PI3K/Akt/eNOS-dependent signal pathways in human endothelial cells. Biochem. Biophys. Res. Commun. 376, 404–408. doi: 10.1016/j.bbrc.2008.09.001
Fang, J., Little, P. J., and Xu, S. (2017). Atheroprotective effects and molecular targets of tanshinones derived from herbal medicine danshen. Med. Res. Rev. doi: 10.1002/med.21438
Gimbrone, M. A. Jr., and Garcia-Cardena, G. (2016). Endothelial cell dysfunction and the pathobiology of atherosclerosis. Circ. Res. 118, 620–636. doi: 10.1161/CIRCRESAHA.115.306301
Han, Y. Y., Song, M. Y., Hwang, M. S., Hwang, J. H., Park, Y. K., and Jung, H. W. (2016). Epimedium koreanum Nakai and its main constituent icariin suppress lipid accumulation during adipocyte differentiation of 3T3-L1 preadipocytes. Chin. J. Nat. Med. 14, 671–676. doi: 10.1016/S1875-5364(16)30079-6
He, W., Sun, H., Yang, B., Zhang, D., and Kabelitz, D. (1995). Immunoregulatory effects of the herba Epimedium glycoside icariin. Arzneimittelforschung 45, 910–913.
Hu, Y., Liu, K., Yan, M., Zhang, Y., Wang, Y., and Ren, L. (2015a). Effects and mechanisms of icariin on atherosclerosis. Int. J. Clin. Exp. Med. 8, 3585–3589.
Hu, Y., Sun, B., Liu, K., Yan, M., Zhang, Y., Miao, C., et al. (2015b). Icariin attenuates high-cholesterol diet induced atherosclerosis in rats by inhibition of inflammatory response and p38 MAPK signaling pathway. Inflammation 39, 228–236. doi: 10.1007/s10753-015-0242-x
Hu, Y., Liu, K., Yan, M., Zhang, Y., Wang, Y., and Ren, L. (2016). Icariin inhibits oxidized low-density lipoprotein-induced proliferation of vascular smooth muscle cells by suppressing activation of extracellular signal-regulated kinase 1/2 and expression of proliferating cell nuclear antigen. Mol. Med. Rep. 13, 2899–2903. doi: 10.3892/mmr.2016.4813
Huang, X., Xiang, T., Xu, S., Lu, S., Liu, H., Zhang, W. C., et al. (2016). [Icariin reduces S-nitrosogultathione induced endothelial cell apoptosis through modulating AKT/P53 pathway]. Zhonghua Xin Xue Guan Bing Za Zhi 44, 707–713. doi: 10.3760/cma.j.issn.0253-3758.2016.08.013
Koizumi, H., Yu, J., Hashimoto, R., Ouchi, Y., and Okabe, T. (2010). Involvement of androgen receptor in nitric oxide production induced by icariin in human umbilical vein endothelial cells. FEBS Lett. 584, 2440–2444. doi: 10.1016/j.febslet.2010.04.049
Libby, P., Ridker, P. M., and Hansson, G. K. (2011). Progress and challenges in translating the biology of atherosclerosis. Nature 473, 317–325. doi: 10.1038/nature10146
Liu, T., Qin, X. C., Li, W. R., Zhou, F., Li, G. Y., Xin, H., et al. (2011). [Effects of icariin and icariside II on eNOS expression and NOS activity in porcine aorta endothelial cells]. Beijing Da Xue Xue Bao 43, 500–504.
Liu, Z., Wang, J., Huang, X., Li, Z., and Liu, P. (2016). Deletion of sirtuin 6 accelerates endothelial dysfunction and atherosclerosis in apolipoprotein E-deficient mice. Transl. Res. 172, 18–29.e12. doi: 10.1016/j.trsl.2016.02.005
Liu, Z., Xu, S., Huang, X., Wang, J., Gao, S., Li, H., et al. (2015). Cryptotanshinone, an orally bioactive herbal compound from Danshen, attenuates atherosclerosis in apolipoprotein E-deficient mice: role of lectin-like oxidized LDL receptor-1 (LOX-1). Br. J. Pharmacol. 172, 5661–5675. doi: 10.1111/bph.13068
Liu, Z. Q., Luo, X. Y., Sun, Y. X., Wu, W., Liu, C. M., Liu, Z. Q., et al. (2004). The antioxidative effect of icariin in human erythrocytes against free-radical-induced haemolysis. J. Pharm. Pharmacol. 56, 1557–1562. doi: 10.1211/0022357044869
Orekhov, A. N., Sobenin, I. A., Revin, V. V., and Bobryshev, Y. V. (2015). Development of antiatherosclerotic drugs on the basis of natural products using cell model approach. Oxid. Med. Cell. Longev. 2015:463797. doi: 10.1155/2015/463797
Pedersen, T. R. (2016). The success story of LDL cholesterol lowering. Circ. Res. 118, 721–731. doi: 10.1161/CIRCRESAHA.115.306297
Rader, D. J., and Daugherty, A. (2008). Translating molecular discoveries into new therapies for atherosclerosis. Nature 451, 904–913. doi: 10.1038/nature06796
Rosenson, R. S. (2004). Statins in atherosclerosis: lipid-lowering agents with antioxidant capabilities. Atherosclerosis 173, 1–12. doi: 10.1016/S0021-9150(03)00239-9
Ross, R. (1993). The pathogenesis of atherosclerosis: a perspective for the 1990s. Nature 362, 801–809. doi: 10.1038/362801a0
Shen, B. (2015). A new golden age of natural products drug discovery. Cell 163, 1297–1300. doi: 10.1016/j.cell.2015.11.031
Shen, C. Y., Jiang, J. G., Yang, L., Wang, D. W., and Zhu, W. (2017). Anti-ageing active ingredients from herbs and nutraceuticals used in traditional Chinese medicine: pharmacological mechanisms and implications for drug discovery. Br. J. Pharmacol. 174, 1395–1425. doi: 10.1111/bph.13631
Shen, X., and He, H. (2009). [Effects of icariin on expression of glucose regulated protein 78 in vascular smooth muscle cell induced by homocysteine]. Zhongguo Zhong Yao Za Zhi 34, 1964–1967.
Tabas, I., Garcia-Cardena, G., and Owens, G. K. (2015). Recent insights into the cellular biology of atherosclerosis. J. Cell Biol. 209, 13–22. doi: 10.1083/jcb.201412052
Wang, Y., Wang, Y. S., Song, S. L., Liang, H., and Ji, A. G. (2016). Icariin inhibits atherosclerosis progress in Apoe null mice by downregulating CX3CR1 in macrophage. Biochem. Biophys. Res. Commun. 470, 845–850. doi: 10.1016/j.bbrc.2016.01.118
Wang, Y. K., and Huang, Z. Q. (2005). Protective effects of icariin on human umbilical vein endothelial cell injury induced by H2O2 in vitro. Pharmacol. Res. 52, 174–182. doi: 10.1016/j.phrs.2005.02.023
Wu, J., Xu, H., Wong, P. F., Xia, S., Xu, J., and Dong, J. (2014). Icaritin attenuates cigarette smoke-mediated oxidative stress in human lung epithelial cells via activation of PI3K-AKT and Nrf2 signaling. Food Chem. Toxicol. 64, 307–313. doi: 10.1016/j.fct.2013.12.006
Wu, X., Huang, H., Tang, F., Le, K., Xu, S., and Liu, P. (2010). Regulated expression of endothelial lipase in atherosclerosis. Mol. Cell. Endocrinol. 315, 233–238. doi: 10.1016/j.mce.2009.11.003
Xiao, H. B., Liu, Z. K., Lu, X. Y., Deng, C. N., and Luo, Z. F. (2015). Icariin regulates PRMT/ADMA/DDAH pathway to improve endothelial function. Pharmacol. Rep. 67, 1147–1154. doi: 10.1016/j.pharep.2015.04.017
Xiao, H. B., Sui, G. G., and Lu, X. Y. (2017). Icariin improves eNOS/NO pathway to prohibit the atherogenesis of apolipoprotein E-null mice. Can. J. Physiol. Pharmacol. 95, 625–633. doi: 10.1139/cjpp-2016-0367
Xiao-Hong, D., Chang-Qin, X., Jian-Hua, H., Wen-Jiang, Z., and Bing, S. (2013). Icariin delays homocysteine-induced endothelial cellular senescence involving activation of the PI3K/AKT-eNOS signaling pathway. Pharm. Biol. 51, 433–440. doi: 10.3109/13880209.2012.738332
Xin, Z. C., Kim, E. K., Lin, C. S., Liu, W. J., Tian, L., Yuan, Y. M., et al. (2003). Effects of icariin on cGMP-specific PDE5 and cAMP-specific PDE4 activities. Asian J. Androl. 5, 15–18.
Xu, C. Q., Liu, B. J., Wu, J. F., Xu, Y. C., Duan, X. H., Cao, Y. X., et al. (2010). Icariin attenuates LPS-induced acute inflammatory responses: involvement of PI3K/Akt and NF-kappaB signaling pathway. Eur. J. Pharmacol. 642, 146–153. doi: 10.1016/j.ejphar.2010.05.012
Xu, H. B., and Huang, Z. Q. (2007). Icariin enhances endothelial nitric-oxide synthase expression on human endothelial cells in vitro. Vascul. Pharmacol. 47, 18–24. doi: 10.1016/j.vph.2007.03.002
Xu, S. (2017). Transcriptome profiling in systems vascular medicine. Front. Pharmacol. 8:563. doi: 10.3389/fphar.2017.00563
Xu, S., Bai, P., and Jin, Z. G. (2016a). Sirtuins in cardiovascular health and diseases. Trends Endocrinol. Metab. 27, 677–678. doi: 10.1016/j.tem.2016.07.004
Xu, S., Liu, B., Yin, M., Koroleva, M., Mastrangelo, M., Ture, S., et al. (2016b). A novel TRPV4-specific agonist inhibits monocyte adhesion and atherosclerosis. Oncotarget 7, 37622–37635. doi: 10.18632/oncotarget.9376
Xu, S., Yin, M., Koroleva, M., Mastrangelo, M. A., Zhang, W., Bai, P., et al. (2016c). SIRT6 protects against endothelial dysfunction and atherosclerosis in mice. Aging 8, 1064–1082. doi: 10.18632/aging.100975
Xu, S., Bai, P., Little, P. J., and Liu, P. (2014a). Poly(ADP-ribose) polymerase 1 (PARP1) in atherosclerosis: from molecular mechanisms to therapeutic implications. Med. Res. Rev. 34, 644–675. doi: 10.1002/med.21300
Xu, S., Liu, Z., and Liu, P. (2014b). Targeting hydrogen sulfide as a promising therapeutic strategy for atherosclerosis. Int. J. Cardiol. 172, 313–317. doi: 10.1016/j.ijcard.2014.01.068
Xu, S., Ogura, S., Chen, J., Little, P. J., Moss, J., and Liu, P. (2013). LOX-1 in atherosclerosis: biological functions and pharmacological modifiers. Cell. Mol. Life Sci. 70, 2859–2872. doi: 10.1007/s00018-012-1194-z
Yang, H., Yan, L., Qian, P., Duan, H., Wu, J., Li, B., et al. (2015). Icariin inhibits foam cell formation by down-regulating the expression of CD36 and up-regulating the expression of SR-BI. J. Cell. Biochem. 116, 580–588. doi: 10.1002/jcb.25009
Ye, L. C., and Chen, J. M. (2001). [Advances in study on pharmacological effects of Epimedium]. Zhongguo Zhong Yao Za Zhi 26, 293–295.
Zhang, S. Q., Cai, W. J., Huang, J. H., Wu, B., Xia, S. J., Chen, X. L., et al. (2015). Icariin, a natural flavonol glycoside, extends healthspan in mice. Exp. Gerontol. 69, 226–235. doi: 10.1016/j.exger.2015.06.020
Zhang, W. P., Bai, X. J., Zheng, X. P., Xie, X. L., and Yuan, Z. Y. (2013). Icariin attenuates the enhanced prothrombotic state in atherosclerotic rabbits independently of its lipid-lowering effects. Planta Med. 79, 731–736. doi: 10.1055/s-0032-1328551
Zhang, Z. Q., Ren, S. C., Tan, Y., Li, Z. Z., Tang, X., Wang, T. T., et al. (2016). Epigenetic regulation of NKG2D ligands is involved in exacerbated atherosclerosis development in Sirt6 heterozygous mice. Sci. Rep. 6:23912. doi: 10.1038/srep23912
Keywords: icariin, atherosclerosis, endothelial function, foam cell, smooth muscle cell
Citation: Fang J and Zhang Y (2017) Icariin, an Anti-atherosclerotic Drug from Chinese Medicinal Herb Horny Goat Weed. Front. Pharmacol. 8:734. doi: 10.3389/fphar.2017.00734
Received: 29 August 2017; Accepted: 29 September 2017;
Published: 12 October 2017.
Edited by:
Changhua Wang, Wuhan University, ChinaReviewed by:
Yanwu Hu, Tonghua Normal University, ChinaWolfgang F. Graier, Medical University of Graz, Austria
Copyright © 2017 Fang and Zhang. This is an open-access article distributed under the terms of the Creative Commons Attribution License (CC BY). The use, distribution or reproduction in other forums is permitted, provided the original author(s) or licensor are credited and that the original publication in this journal is cited, in accordance with accepted academic practice. No use, distribution or reproduction is permitted which does not comply with these terms.
*Correspondence: Jian Fang, bGlmZWd6QHFxLmNvbQ==