- 1Guangdong Provincial Key Laboratory of Veterinary Pharmaceutics Development and Safety Evaluation, South China Agricultural University, Guangzhou, China
- 2National Risk Assessment Laboratory for Antimicrobial Resistance of Animal Original Bacteria, South China Agricultural University, Guangzhou, China
- 3Joint International Research Laboratory of Agriculture and Agri-Product Safety, Institutes of Agricultural Science and Technology Development, Yangzhou University, Yangzhou, China
Objectives: Carbapenems, colistin, and tigecycline are critically important antibiotics in clinics. After the global appearance of blaNDM and mcr mediating the resistance to carbapenems and colistin, respectively, tigecycline becomes the last-resort drug against severe human infections caused by multidrug-resistant bacteria. Recently, a mobile tigecycline resistance gene tet(X4) has been identified in Escherichia coli, Klebsiella pneumoniae, and Acinetobacter baumannii that causes high resistance to tigecycline and other tetracyclines. In this study, the prevalence of tet(X4) in E. coli isolates from duck and goose farms in Southeast China was identified and characterized.
Methods: Feces, soil, sewage, and dust samples were collected from duck and goose farms along with the southeast coast provinces of China. Antimicrobial susceptibility testing and polymerase chain reaction screening were performed to investigate the phenotype and genotype of tigecycline resistance. Conjugation, S1 pulsed-field gel electrophoresis (PFGE), and whole-genome sequencing were used to determine the transferability, genetic location, and the genomic characteristics of tet(X4).
Results: In total, 1,716 samples were collected, and 16 isolates (0.9%) recovered from Guangdong, Shandong, and Jiangsu were positive for tet(X4) gene with tigecycline minimum inhibitory concentrations ≥16 mg/L. Notably, among these tet(X4)-positive E. coil isolates, seven of them were from the environment samples (soil and sewage). PFGE and multilocus sequence typing demonstrated that ST3997 was the most prevalent sequence type (eight isolates, 50%) in Jiangsu province. By conjugation assays, 11 isolates were able to transfer tet(X4) plasmid to E. coli C600 recipient, and these plasmids belonged to IncHI1 and IncX1 detected by sequence analysis. tet(X4) was found adjacent to an insertion sequence ISCR2 downstream and a catD gene upstream for all isolates. In addition, multiple-drug resistance to tigecycline, chlortetracycline, ampicillin, florfenicol, ciprofloxacin, gentamicin, trimethoprim/sulfamethoxazole, and fosfomycin was profiled in most of the tet(X4)-positive isolates.
Conclusion: The identification of tet(X4) harboring E. coli strains in duck farms and their surrounding environment enlarges our knowledge of the variety and prevalence of tigecycline resistance. The prevalence of tet(X4) raises concern for the use of tetracyclines in animal farming, and the tet(X4) gene should be listed as primary gene for resistance surveillance.
Introduction
Carbapenems, colistin, and tigecycline are considered as the last-resort antibiotics against severe human infections caused by multidrug-resistant (MDR) bacteria. However, the discovery of a series of carbapenems resistance genes, such as blaOXA, blaNDM, blaVIM, and blaKPC, as well as the mobile colistin resistance mcr gene, has compromised the effectiveness of carbapenems and colistin in clinics (Gangcuangco et al., 2016; Lunha et al., 2016; Wang et al., 2017; Gaibani et al., 2020). Under such circumstances, tigecycline was considered as the last chance for treatment of extensively drug-resistant pathogens. Unfortunately, the effectiveness of tigecycline was compromised by the new plasmid-borne variants of the tet(X) family genes tet(X3) and tet(X4) that mediate the resistance to tigecycline, as well as to the newly approved eravacycline and omadacycline (He et al., 2019). The identification of tigecycline resistance has again raised challenges for clinical treatment of critical infections, especially caused by carbapenem-resistant Enterobacteriaceae (CRE) in public health.
The tetracycline family of therapeutic agents has been in commercial use since 1940s, but the increasing incidence of bacterial resistance has relegated older tetracyclines to a limited role for treating common infectious diseases (Villano et al., 2016). Three new tetracyclines generations (tigecycline, omadacycline, and eravacycline) have been discovered that circumvent the common tetracycline resistance mechanisms. Tigecycline, a glycylcycline tetracycline, has demonstrated antibacterial activity across a broad spectrum of Gram-positive, Gram-negative, anaerobic, and atypical bacteria (Peterson, 2008). It was approved for complicated skin and intra-abdominal infections by both the Food and Drug Administration (FDA) in the United States and the European Medicines Agency (European medicines agency, 2013). It can be applied for the community-acquired bacterial pneumonia approved by FDA as well (FDA, 2005). Tigecycline exhibits antimicrobial susceptibility against broad-spectrum pathogens including the CRE and even colistin-meropenem–coresistant Escherichia coli (Yu et al., 2019).
However, tigecycline resistance has been reported to be mediated by different resistant mechanisms. Evolution of the tetA gene decreases tigecycline susceptibility with minimum inhibitory concentrations (MICs) from 1 rising to 32 mg/L and leads to treatment failure in carbapenem-resistant Klebsiella pneumoniae infections (Du et al., 2018). Overexpression of the resistance–nodulation–cell division–type efflux system plays a major role in tigecycline resistance in clinical Acinetobacter nosocomialis (Yang et al., 2019), and the mutations at the tip of the extended loop of the ribosomal S10 protein have been associated with tigecycline resistance in different bacterial species (Angeles Argudin et al., 2018). The latest identification of the plasmid-mediated high-level tigecycline resistance gene, tet(X4), has again decreased the promised prospect of using tigecycline in the clinic (He et al., 2019; Sun et al., 2019a). For treating common community- and hospital-acquired infections, omadacycline, a first-in-class aminomethylcycline antibiotic, has been newly approved by FDA and is active against extended-spectrum beta-lactamase–producing bacteria, methicillin-resistant Staphylococcus aureus, and even vancomycin-resistant enterococcus (Macone et al., 2014). Eravacycline, also approved by FDA, is a fully synthetic fluorocycline antibiotic and active against clinically important pathogens mostly resistant to cephalosporins, fluoroquinolones, beta-lactams, and carbapenems (Zhanel et al., 2016). The severely important aspect of the tet(X4) gene is that it also causes the resistance to both omadacycline and eravacycline (Sun et al., 2019a).
Aquaculture is a diversified production sector with different production systems and practices. Although centralization-breeding factory are increasingly popular in China, one of the integrated farming systems (duck or goose–fish production) still plays a significant role in southeast China (Miao, 2010). In this study, we investigated the prevalence of tet(X4) in duck and goose farms belonging to different breeding patterns in Southeast China. Isolation ratio for tet(X4) gene was analyzed, and the genomic profiling was conducted for a comprehensive understanding of the genomic background of tet(X4) gene. Furthermore, conjugation assays were tested to determine the dissemination and transferability of the tet(X4) gene.
Materials and Methods
Sampling Information and Bacterial Strains
From May 1, 2017, to January 1, 2019, we collected 1,716 consecutive, non-duplicate samples, including fecal (1211), soil (259), sewage (228), and dust (18) samples, from 25 duck farms and 3 goose farms in six provinces (Shandong, Jiangsu, Fujian, Guangdong, Hainan, and Guangxi) along with the southeast coast of China (Figure 1 and Supplementary Table 1). All duck and goose farms investigated in this study were divided into three different breeding patterns (Supplementary Figure 1): (A) on filter net and shelves, (B) along the river without shed, and (C) duck–fish production system. Feces samples were collected freshly from the dropping trays (breeding pattern A) or the grounds (B and C), and dust samples were scrubbed from the windows or doors of sheds in patterns A and C. The fecal samples were randomly collected from ducks and geese, with approximately 60 samples per farm. The dust samples were collected with cotton swab and transferred into the 2-mL centrifuge tubes with normal saline. Soil samples were collected around the farms, and sewage samples were collected from the river near the farms and downstream as well. The soil, dust, and sewage samples were collected at least in triplicate per farm. Tigecycline non-susceptible isolates were selected on MacConkey agar plates containing tigecycline (4 mg/L), and all plates were incubated at 37°C for 20 to 22 h. Then, one to three well-formed tigecycline non-susceptible colonies were randomly selected for polymerase chain reaction (PCR) screening of tet(X), and the tet(X4) subtypes were further confirmed by full-length amplification and Sanger sequencing, as previously described (Sun et al., 2019a). The bacterial species was identified by MOLDI-TOF MS AximaTM (Shimadzu-Biotech Corp., Kyoto, Japan) and 16S rRNA sequencing. Then, for multiple isolates separated from the same sample, enterobacterial repetitive intergenic consensus (ERIC)–PCR was performed for preliminary typing using previous primers (Sun et al., 2019a), and different clones were kept.
Antimicrobial Susceptibility Testing
Against the tet(X4)-positive strains, MICs of tigecycline, chlortetracycline, amikacin, gentamicin, meropenem, colistin, ceftazidime, cefotaxime, fosfomycin, ciprofloxacin, ampicillin, and sulfamethoxazole–trimethoprim were determined and interpreted according to the Clinical & Laboratory Standards Institute (CLSI) guidelines (CLSI, 2019). In particular, the breakpoint of colistin was interpreted in accordance with the EUCAST breakpoint (EUCAST, 2021). Florfenicol breakpoints were regarding the previous report (Michigan State University, 2014). E. coli ATCC 25922 served as the quality control strain.
Whole-Genome Sequencing
Genomic DNA was extracted from E. coli strains using the Genomic DNA Purification Kit (Tiangen, China). DNA purity and concentration were determined using a NANODROP 2000c spectrophotometer. Whole-genome sequencing (WGS) was performed with the Illumina HiSeq 2500 system (Bionova Biotech Co., China) using the paired-end 2% 150-bp sequencing protocol. The draft genome was de novo assembled using SPAdes version 3.12.0. The putative coding sequences of the flanking regions of tet(X4) were obtained using RAST1. Multilocus sequence typing (MLST), plasmid in-compatibility (Inc) groups, antibiotic resistance genes (ARGs), and mobile elements were analyzed by Center for Genomic Epidemiology2 and ISfinder3.
Molecular Typing
All tet(X4)-positive E. coli strains were classified by XbaI digested (Takara, Dalian, China) pulsed-field gel electrophoresis (PFGE) according to the PulseNet protocol4 using a CHEF Mapper System (Bio-Rad, Hercules, CA, United States). PFGE patterns were compared using BioNumerics version 6.6 (Applied Maths, Sint-Martens-Latem, Belgium) under appropriate optimization (1.5%) and tolerance (1.5%) settings and a cutoff at 85% similarity to delineate PFGE clusters. MLST was performed by the primers and protocol specified on the E. coli MLST database website5.
Plasmid Characterization
The tet(X4) gene locations were identified using S1 nuclease-PFGE and Southern blot analysis. Briefly, DNA from donor strains and the transconjugants harboring tet(X4) were extracted and embedded in agarose gel plugs and then treated with S1 nuclease (Takara), and the DNA fragments were separated by PFGE. Southern blot hybridization was then performed with DNA probes specific for the tet(X4) gene that was non-radioactively labeled with a DIG High Prime DNA labeling and detection kit (Roche Diagnostics, Mannheim, Germany) (Versalovic et al., 1991).
Conjugation and Transformation Analyses
To investigate the transferability of tet(X4)-bearing plasmid, conjugation assays were performed using streptomycin-resistance E. coli C600 as the recipient strain. Briefly, overnight cultures of donor and the recipient strains were 1:1 mixed and incubated at 37°C for 16 to 20 h. After incubation, 10-fold serial dilutions were mixed in sterile saline, and 100-μL samples were spread onto LB agar plates containing 4 mg/L tigecycline and 1,500 mg/L streptomycin. The tet(X4)-positive transconjugants were confirmed by PCR and ERIC-PCR (Versalovic et al., 1991; Sun et al., 2019b). Susceptibility of transconjugants was detected as mentioned previously. Plasmid analysis was performed using whole-genome sequence as described previously. Plasmid DNA was extracted using a Qiagen Prep Plasmid Midi Kit (Hilden, Germany).
Results
With the detection ratio of 0.9%, 16 E. coli strains were identified harboring tet(X4) gene, among which nine were isolated from feces, six from soil, and one from sewage (Supplementary Table 1). The 16 tet(X4)-positive strains were detected from three provinces: Shandong, Jiangsu, and Guangdong (Figure 1).
Antimicrobial susceptibility testing identified that all tet(X4)-positive E. coli strains were resistant to tigecycline showing MICs over 16 mg/L (8 mg/L of FDA breakpoint) (FDA, 2019). In addition, most of these strains were multiply resistant to ampicillin, chlortetracycline, florfenicol, fosfomycin, sulfamethoxazole-trimethoprim, and ciprofloxacin (Figure 2 and Supplementary Table 2). The MICs for colistin and meropenem for all E. coli strains were lower than 0.25 and 0.03 mg/L, respectively. In total, 9 of 16 isolates were resistant to gentamicin, with MICs ranging from 0.5 to 128 mg/L, whereas no isolates were resistant to amikacin (Supplementary Table 2).

Figure 2. Antimicrobial resistance profiles of tet(X4)-positive E. coli isolates and the presence or lack of AMR genes are colored in blue or gray, respectively. The red squares indicate the presence of tet(X4) gene. The green squares indicate the plasmid type. TGC, tigecycline; CETE, chlortetracycline; MEM, meropenem; CAZ, ceftazidime; CTX, cefotaxime; AMP, ampicillin; CIP, ciprofloxacin; S/T, trimethoprim/sulfamethoxazole; GEN, gentamicin; AMK, amikacin; FOS, fosfomycin; CS, colistin. The breakpoint of CETE is not available, so resistance to CETE was defined regarding the resistant breakpoint of tetracycline (≥16 mg/L) (CLSI, 2019).
We further characterized the molecular structures of the tet(X4)-positive isolates and tet(X4)-bearing plasmids. The distinct tet(X4)-positive isolates belonged to seven sequence types (STs): ST3997 (n = 8), ST2325 (n = 1), ST48 (n = 1), ST3944 (n = 1), and ST746 (n = 1) and another two new STs labeling as New-1 (n = 3) and New-2 (n = 1) in Figure 3. Interestingly, most isolates from Jiangsu province belonged to ST3997 sharing the same PFGE pattern suggesting clonal dissemination. Three tet(X4)-positive E. coli isolates from Guangdong province belonged to a new ST type (Figure 3).
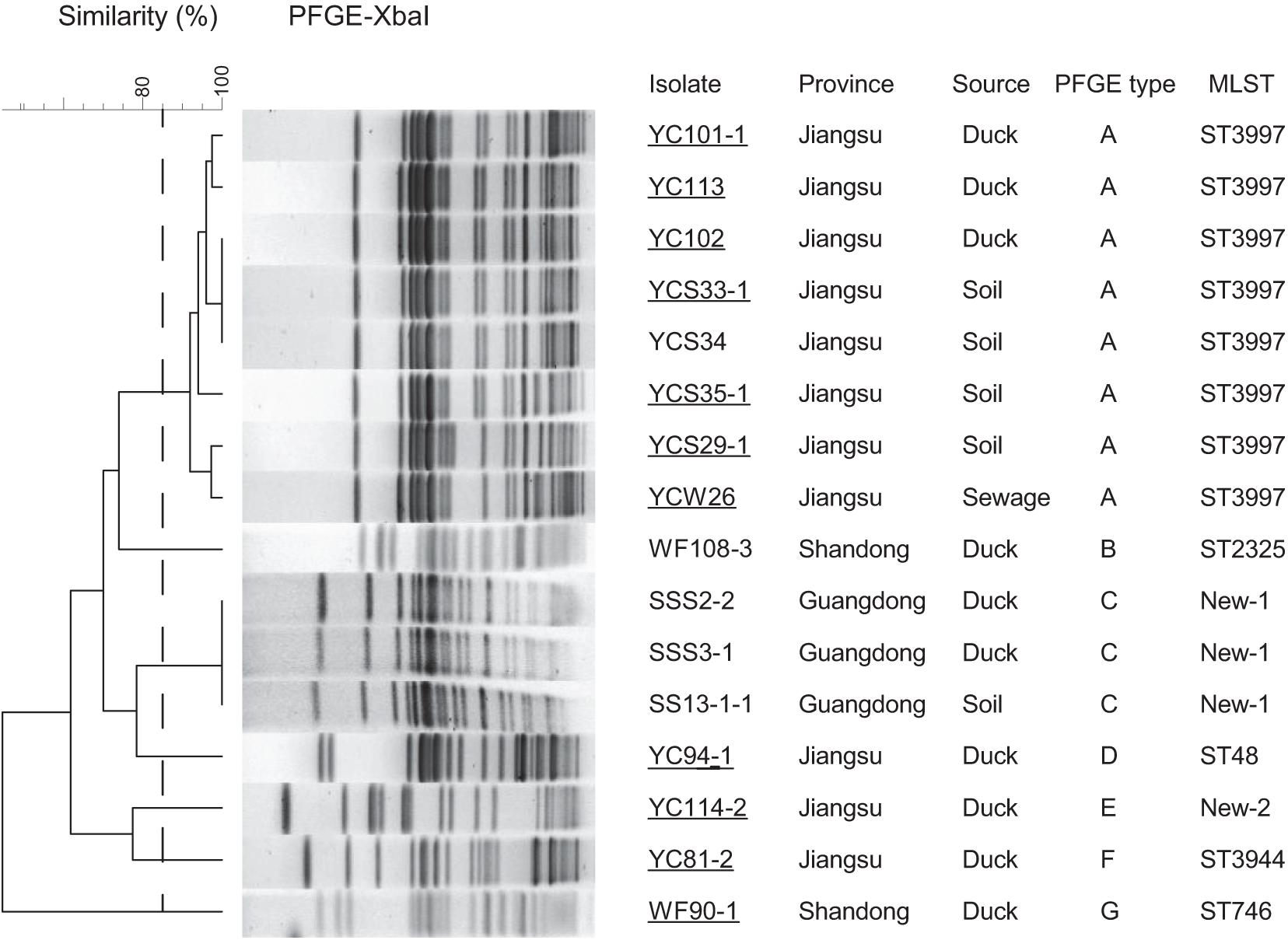
Figure 3. PFGE-Xbal dendrogram and details about tet(X4)-positive E. coli isolates, PFGE patterns with a cutoff at 85% similarity were considered to be the same cluster and are included as groups A–G. Sampling details are listed in the right table, as well as the MLST analysis results of WGS. Conjugation assays to C600 succeeded for 11 isolates labeling with underline. Duck, duck feces.
In the mating tests, 11 tet(X4) genes were successfully transferred to E. coli C600, and S1-PFGE of both donors and transconjugants suggested that these genes were located on plasmids. The tigecycline MICs of the transconjugants were increased by at least 32-fold over the C600 recipient strains (Supplementary Table 2). Further, S1-nuclease digestion and Southern blot analysis showed that the tet(X4) genes were mostly located on plasmids in sizes of 200, 230, or 330 kb (Supplementary Figures 2A,B). Different incompatibility groups of IncHI1 and IncX1 plasmids carrying tet(X4) genes were confirmed by product enhanced reverse transcriptase testing of the corresponding transconjugants. During the mating assay, the cotransfer of IncHI1 and IncX1 plasmids was observed for YC101-1, YC113, YC102, and YCS29-1 as donors.
Moreover, WGS showed the tet(X4) gene was located primarily within three similar contigs in size of 2,700 to 9,553 bp bearing the core gene arrangement catD-tet(X4)-terlS-ISCR2-orilS (Figure 4), which is identical to the reference plasmid p47EC (MK 134376.1) from E. coli (He et al., 2019). Mobile elements including △IS1R and IS26 were identified at the upstream of tet(X4) gene in IncHI1 plasmid from 11 isolates and IncX1 plasmid from three isolates, respectively. This indicated that the tet(X4) genes are highly active and possibly will further transfer to other plasmids or isolates. For another two isolates, only orilS was found at the upstream of tet(X4) gene. Additionally, resistance genes of tet(A), aph, aadA, aadA2, blaCTX, blaTEM–1B, blaOXA, blaSHV, oqxAB, qnrS1, fosA3, mdfA, lnu, erm, cmlA1, floR, sul, and dfrA were detected in these tet(X4)-positive isolates as well (Figure 2).
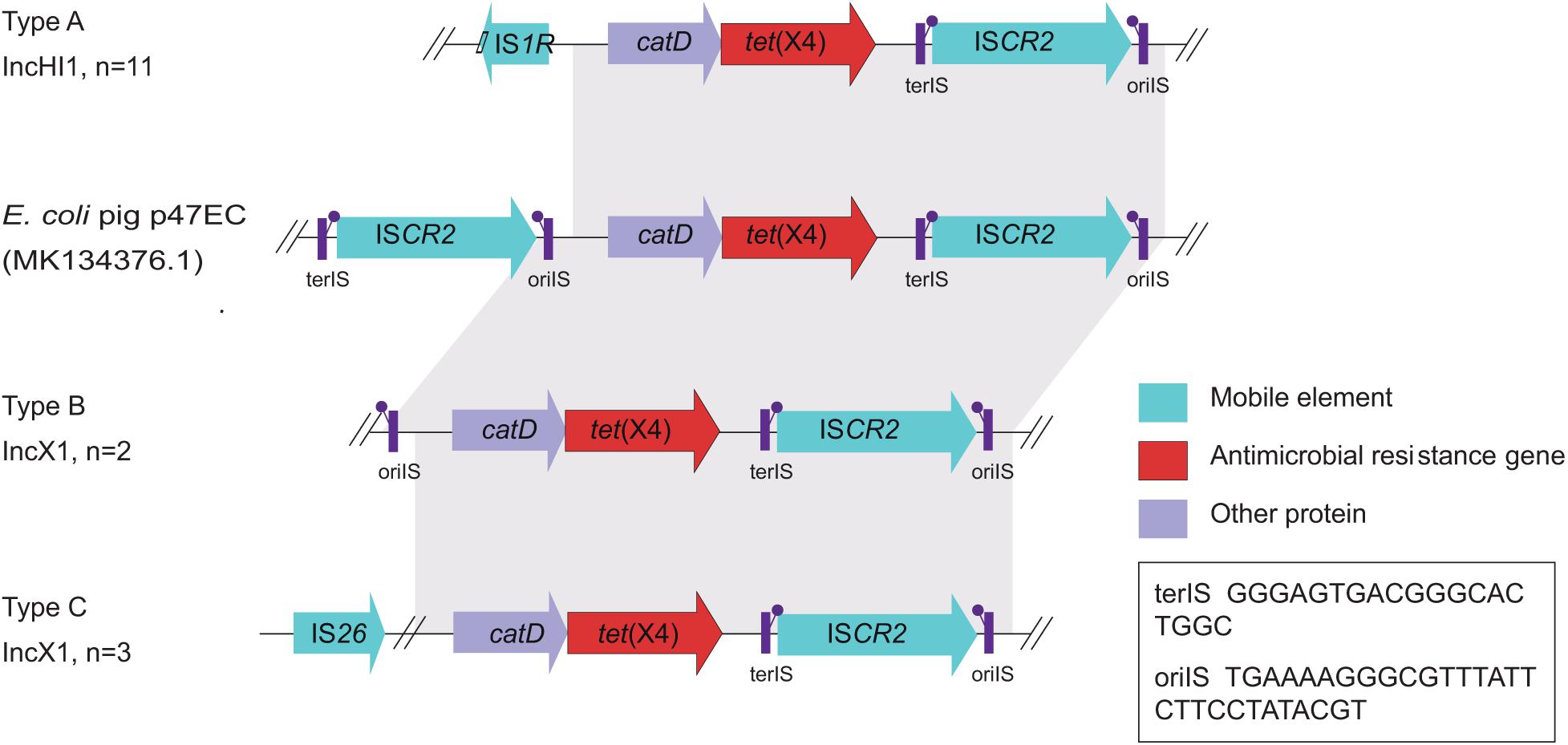
Figure 4. Gene alignments of the tet(X4) in isolates from this study and the reported plasmid p47EC. Mobile elements, antimicrobial resistance genes, and other proteins are shown as blue, red, and purple arrows, respectively. Regions of homology between 73 and 100% are marked by gray shading.
Discussion
For now, the tet(X4)-positive isolates were mostly reported in animals such as pigs, chickens, cows, and ducks. In our study, in the region of Southeast China, 0.7% (9/1211), 2.3% (6/259), and 0.4% (1/228) of studied fecal, soil, and sewage samples possessed tet(X4)-positive E. coli strains originated on duck farms. Although the detection ratio of tet(X4) in humans was low (0.07%), tet(X4) has been previously identified in pigs and chickens at slaughters, from soil and dust in animal farms (Sun et al., 2019a) (also in our study), and even pork from markets (Bai et al., 2019). Given the fact that tigecycline is not approved for veterinary use, the presence of tet(X4) in animals may be due to the high-level use of tetracycline derivatives for livestock (Bai et al., 2019). As one of the countries with the largest amount of antibiotics usage in the world, 12,000 tons (7.4%) of tetracyclines were consumed by China yearly (data from 2013), and most of them eventually entered into environment (Zhang et al., 2015). The tet(X4) gene possibly resulted from the evolution of tetX family members driven by the historical selective pressure due to the large-scale use of tetracyclines and had become the most worrisome resistant determinant to data. In addition, the importance of animals in the dissemination of tigecycline-resistance is only becoming recognized, and it might be just a matter of time when the tet(X4)-producing pathogens become widespread in public.
Whole-genome sequencing analysis showed that 16 E. coli strains belonged to seven different MLSTs (ST3997, ST2325, ST48, ST3944, and ST746 and another two new types). Among STs, ST3977 was the most prevalent type in Jiangsu province and has been reported harboring blaNDM–1 and mcr-1 genes in animals (Novovic et al., 2016). ST48 has been identified in humans from various geographical areas (Nabal et al., 2019), indicating the wide spread of ST48 E. coli strains in public health. Multiple-drug resistance to chlortetracycline, ceftazidime, cefotaxime, ampicillin, florfenicol, ciprofloxacin, trimethoprim/sulfamethoxazole, and fosfomycin was observed in tet(X4) isolates. In accordance with the antimicrobial susceptibility tests, the WGS analysis indicated that more than 20 different ARGs were carried in these isolates. For instance, we identified tet(X4), tet(A), aph(3’), aph(4), aph(6), aacA, blaCTX, blaOXA, blaTEM, oqxAB, qnrS1, fosA3, mdfA, lnu(G), floR, ARR-2, and sul2 genes in all the isolates from Jiangsu province. Notably, eight E. coli strains were detected cocarrying blaCTX–M–55 and blaCTX–M–14 genes, indicating the widespread and the diversity of ESBL resistance. Together with the similar PFGE patterns, it is possible that one epidemic clone has been disseminated in this region. The scenario is alarming as these isolates can act as an abundant reservoir spreading ARGs to both environment and humans.
We detected tet(X4) strains from both patterns A and C breeding system. Duck farming in pattern A system often has high density of animals, and the duck–fish production system (pattern C) is much more economical than most traditional crop farming and poultry husbandry systems, because it is based on the concept that “there is no waste,” and “waste is only a misplaced resource that can be a valuable input for other component” (Krag et al., 2015). There was no enough evidence in this study to demonstrate the connection of dissemination of tet(X4) with the different breeding patterns. However, it is concerning that among all the tet(X4)-positive isolates, 45% (5/11) of the samples were isolated from environments in Shandong and Jiangsu provinces. Additionally, AMR genes including tet(X4) were identified in isolates recovered from sewage, river, and soil of ponds in this study, indicating the possible dissemination of AMR genes in environment.
In conclusion, we identified 16 tet(X4)-positive E. coli isolates from duck farms in southeast China. Notably, this is the first study to report the development of diversity in the population of tet(X4)-positive E. coli isolates from ducks. WGS analysis further determined tet(X4) coexisted with other ARGs mediating multiple-drug resistance to chlortetracycline, ampicillin, florfenicol, ciprofloxacin, gentamicin, trimethoprim/sulfamethoxazole, and fosfomycin. The detection of tet(X4)-bearing IncHI1 and IncX1 plasmids in isolates from feces, soil, and even sewage samples enlarges our understanding of the dissemination of tigecycline-resistant genes as (i) tet(X4)-bearing IncHI1 and IncX1 plasmids were highly transferable and (ii) environmental-isolated strains could pose a greater threat to public health. It is absolutely the time to add the tet(X4) gene into the resistance surveillance.
Data Availability Statement
The datasets presented in this study can be found in online repositories. The names of the repository/repositories and accession number(s) can be found below: NCBI SRA BioProject, accession no: PRJNA743422.
Author Contributions
Y-HL, X-PL, and JS conceived of this study. YY designed the experiment and drafted the manuscript. YY and XK revised the manuscript. YY and C-YC analyzed the sequencing data. C-YC, CC, and XK performed the experiments. M-GW collected all the samples. All authors read and approved the final manuscript.
Funding
This work was supported by the National Key Research and Development Program of China (2016YFD0501300), the Program for Innovative Research Team in the Ministry of Education of China (IRT_17R39), and the Innovation and Strengthening School Project of Guangdong, China (2016KCXTD010PB_171601 and 2019KCXTD001).
Conflict of Interest
The authors declare that the research was conducted in the absence of any commercial or financial relationships that could be construed as a potential conflict of interest.
Publisher’s Note
All claims expressed in this article are solely those of the authors and do not necessarily represent those of their affiliated organizations, or those of the publisher, the editors and the reviewers. Any product that may be evaluated in this article, or claim that may be made by its manufacturer, is not guaranteed or endorsed by the publisher.
Supplementary Material
The Supplementary Material for this article can be found online at: https://www.frontiersin.org/articles/10.3389/fmicb.2021.716393/full#supplementary-material
Footnotes
- ^ https://rast.nmpdr.org/
- ^ https://cge.cbs.dtu.dk/services/
- ^ https://www-is.biotoul.fr
- ^ http://www.pulsenetinternational.org/protocols/
- ^ http://enterobase.warwick.ac.uk
References
Angeles Argudin, M., Roisin, S., Dodémont, M., Nonhoff, C., Deplano, A., and Denisa, O. (2018). Mutations at the ribosomal s10 gene in clinical strains of staphylococcus aureus with reduced susceptibility to tigecycline. Antimicrob. Agents Chemother. 62, e1852–e1817. doi: 10.1128/AAC.01852-17
Bai, L., Du, P., Du, Y., Sun, H., Zhang, P., Wan, Y., et al. (2019). Detection of plasmid-mediated tigecycline-resistant gene tet(X4) in Escherichia coli from pork, Sichuan and Shandong Provinces, China, February 2019. Euro Surveill 24:1900340. doi: 10.2807/1560-7917.ES.2019.24.25.1900340
CLSI (2019). Performance Standards for Antimicrobial Susceptibility Testing, 29th Edn. Annapolis Junction, MD: Clinical and Laboratory Standards Institute.
Du, X., He, F., Shi, Q., Zhao, F., Xu, J., Fu, Y., et al. (2018). The Rapid Emergence of Tigecycline Resistance in blaKPC–2 Harboring Klebsiella pneumoniae, as Mediated in Vivo by Mutation in tetA During Tigecycline Treatment. Front. Microbiol. 9:648.
EUCAST (2021). European Committee on Antimicrobial Susceptibility Testing Breakpoint tables for interpretation of MICs and zone diameters European Committee on Antimicrobial Susceptibility Testing Breakpoint tables for interpretation of MICs and zone diameters, Version 11.0. Sweden: EUCAST.
European medicines agency (2013). European Medicines Agency. Tygacil® SmPC. Available online at: http://www.ema.europa.eu/docs/en_GB/document_library/EPAR_-_Product_Information/human/000644/WC500044508.pdf (accessed March 28, 2019).
FDA (2005). TIGECYCLINE for injection, for intravenous use Initial U.S. Approval: 2005. Silver Spring: FDA, 1–31.
FDA (2019). Tigecycline – Injection products, FDA-Identified Interpretive Criteria. Silver Spring: FDA.
Gaibani, P., Re, M. C., Campoli, C., Viale, P. L., and Ambretti, S. (2020). Bloodstream infection caused by KPC-producing Klebsiella pneumoniae resistant to ceftazidime/avibactam: epidemiology and genomic characterization. Clin. Microbiol. Infect. 26, e1–.e516. doi: 10.1016/j.cmi.2019.11.011
Gangcuangco, L. M., Clark, P., Stewart, C., Miljkovic, G., and Saul, Z. K. (2016). Persistent bacteremia from pseudomonas aeruginosa with in vitro resistance to the novel antibiotics ceftolozane-tazobactam and ceftazidime-avibactam. Case Rep. Infect. Dis. 2016:1520404. doi: 10.1155/2016/1520404
He, T., Wang, R., Liu, D., Walsh, T. R., Zhang, R., Lv, Y., et al. (2019). Emergence of plasmid-mediated high-level tigecycline resistance genes in animals and humans. Nat. Microbiol. 2019:445. doi: 10.1038/s41564-019-0445-2
Krag, L. A., Herrmann, B., Karlsen, J. D., and Mieske, B. (2015). The State of and Aquaculture World Fisheries. Rome: FAO.
Lunha, K., Chanawong, A., Lulitanond, A., Wilailuckana, C., Charoensri, N., Wonglakorn, L., et al. (2016). High-level carbapenem-resistant OXA-48-producing Klebsiella pneumoniae with a novel OmpK36 variant and low-level, carbapenem-resistant, non-porin-deficient, OXA-181-producing Escherichia coli from Thailand. Diagn. Microbiol. Infect. Dis. 85, 221–226. doi: 10.1016/j.diagmicrobio.2016.03.009
Macone, A. B., Caruso, B. K., Leahy, R. G., Donatelli, J., Weir, S., Draper, M. P., et al. (2014). In vitro and in vivo antibacterial activities of omadacycline, a novel aminomethylcycline. Antimicrob. Agents Chemother. 58, 1127–1135. doi: 10.1128/aac.01242-13
Miao, W. (2010). Distribution and characteristics of duck-fish farming systems in eastern china. Rome: FAO.
Michigan State University, D. C. P. A. H. (2014). Resistance Breakpoints for Antimicrobials Used in Animals. Michigan: Michigan State University, 1–8.
Nabal, S., Mormeneo, S., and García-Lechuz, J. M. (2019). Highlights at the 28th Congress of the European Society of Clinical Microbiology and Infectious Diseases (ESCMID), 2018. Rev. Esp. Quimioter. 32, 16–21.
Novovic, K., Vasiljevic, Z., Kuzmanovic, M., Lozo, J., Begovic, J., Kojic, M., et al. (2016). Novel E. coli ST5123 Containing blaNDM-1 Carried by IncF Plasmid Isolated from a Pediatric Patient in Serbia. Microb. Drug Resist. 22, 707–711. doi: 10.1089/mdr.2015.0264
Peterson, L. R. (2008). A review of tigecycline - the first glycylcycline. Int. J. Antimicrob. Agents 32, S215–S222. doi: 10.1016/S0924-8579(09)70005-6
Sun, J., Chen, C., Cui, C.-Y., Zhang, Y., Liu, X., Cui, Z.-H., et al. (2019a). Plasmid-encoded tet(X) genes that confer high-level tigecycline resistance in Escherichia coli. Nat. Microbiol. 2019, 1–8. doi: 10.1038/s41564-019-0496-4
Sun, J., Chen, C., Cui, C. Y., Zhang, Y., Liu, X., Cui, Z. H., et al. (2019b). Plasmid-encoded tet(X) genes that confer high-level tigecycline resistance in Escherichia coli. Nat. Microbiol. 4, 1457–1464.
Versalovic, J., Koeuth, T., and Lupski, R. (1991). Distribution of repetitive DNA sequences in eubacteria and application to finerpriting of bacterial enomes. Nucleic Acids Res. 19, 6823–6831. doi: 10.1093/nar/19.24.6823
Villano, S., Steenbergen, J., and Loh, E. (2016). Omadacycline: development of a novel aminomethylcycline antibiotic for treating drug-resistant bacterial infections. Future Microbiol. 11, 1421–1434. doi: 10.2217/fmb-2016-0100
Wang, Y., Zhang, R., Li, J., Wu, Z., Yin, W., Schwarz, S., et al. (2017). Comprehensive resistome analysis reveals the prevalence of NDM and MCR-1 in Chinese poultry production. Nat. Microbiol. 2:16260. doi: 10.1038/nmicrobiol.2016.260
Yang, Y.-S., Chen, H.-Y., Hsu, W.-J., Chou, Y.-C., Perng, C.-L., Shang, H.-S., et al. (2019). Overexpression of AdeABC efflux pump associated with tigecycline resistance in clinical Acinetobacter nosocomialis isolate. Clin. Microbiol. Infect. 25, e1–.e512. doi: 10.1016/j.cmi.2018.06.012
Yu, Y., Walsh, T. R., Yang, R. S., Zheng, M., Wei, M. C., Tyrrell, J. M., et al. (2019). Novel partners with colistin to increase its in vivo therapeutic effectiveness and prevent the occurrence of colistin resistance in NDM- and MCR-co-producing Escherichia coli in a murine infection model. J. Antimicrob. Chemother. 74, 87–95. doi: 10.1093/jac/dky413
Zhanel, G. G., Cheung, D., Adam, H., Zelenitsky, S., Golden, A., Schweizer, F., et al. (2016). Review of Eravacycline, a Novel Fluorocycline Antibacterial Agent. Drugs 76, 567–588. doi: 10.1007/s40265-016-0545-8
Zhang, Q. Q., Ying, G. G., Pan, C. G., Liu, Y. S., and Zhao, J. L. (2015). Comprehensive evaluation of antibiotics emission and fate in the river basins of China: source analysis, multimedia modeling, and linkage to bacterial resistance. Environ. Sci. Technol. 49, 6772–6782. doi: 10.1021/acs.est.5b00729
Keywords: antimicrobial resistance (AMR), tet(X4), ducks, feces, the environment
Citation: Yu Y, Cui C-Y, Kuang X, Chen C, Wang M-G, Liao X-P, Sun J and Liu Y-H (2021) Prevalence of tet(X4) in Escherichia coli From Duck Farms in Southeast China. Front. Microbiol. 12:716393. doi: 10.3389/fmicb.2021.716393
Received: 28 May 2021; Accepted: 23 July 2021;
Published: 23 August 2021.
Edited by:
Zulqarnain Baloch, Kunming University of Science and Technology, ChinaReviewed by:
Masaru Usui, Rakuno Gakuen University, JapanJasna Hrenovic, University of Zagreb, Croatia
Copyright © 2021 Yu, Cui, Kuang, Chen, Wang, Liao, Sun and Liu. This is an open-access article distributed under the terms of the Creative Commons Attribution License (CC BY). The use, distribution or reproduction in other forums is permitted, provided the original author(s) and the copyright owner(s) are credited and that the original publication in this journal is cited, in accordance with accepted academic practice. No use, distribution or reproduction is permitted which does not comply with these terms.
*Correspondence: Ya-Hong Liu, lyh@scau.edu.cn
†These authors have contributed equally to this work