- 1Department of Biology, University of Naples Federico II, Naples, Italy
- 2Consorzio Nazionale Interuniversitario per le Scienze del Mare, Rome, Italy
- 3Stazione Zoologica Anton Dohrn, Naples, Italy
- 4UN Environment Programme World Conservation Monitoring Centre, Cambridge, United Kingdom
- 5Department of Biological and Environmental Sciences and Technologies, University of Salento, Lecce, Italy
- 6Hellenic Centre for Marine Research, Heraklion, Greece
- 7Instituto Do Mar, Universidade dos Açores, Horta, Portugal
- 8Environmental and Marine Biology, Åbo Akademi University, Turku, Finland
- 9Department of Evolutionary Biology, Ecology and Environmental Sciences, Institut de Recerca de la Biodiversitat, University of Barcelona, Barcelona, Spain
- 10Department of Life and Environmental Science, Polytechnic University of Marche, Ancona, Italy
- 11Facultat de Ciències, Institut d'Ecologia Aquàtica, Universitat de Girona, Girona, Spain
- 12Institut Ciències del Mar, Barcelona, Spain
- 13Helmholtz Institute for Functional Marine Biodiversity at the University of Oldenburg (HIFMB), Oldenburg, Germany
- 14Alfred-Wegener-Institut, Helmholtz Center for Polar and Marine Research, Bremerhaven, Germany
- 15Department of Biology, Faculty of Science, University of Zagreb, Zagreb, Croatia
- 16Estonian Marine Institute, University of Tartu, Tallinn, Estonia
- 17Department of Life and Environmental Sciences, University of Cagliari, Cagliari, Italy
Restoration is considered an effective strategy to accelerate the recovery of biological communities at local scale. However, the effects of restoration actions in the marine ecosystems are still unpredictable. We performed a global analysis of published literature to identify the factors increasing the probability of restoration success in coastal and marine systems. Our results confirm that the majority of active restoration initiatives are still concentrated in the northern hemisphere and that most of information gathered from restoration efforts derives from a relatively small subset of species. The analysis also indicates that many studies are still experimental in nature, covering small spatial and temporal scales. Despite the limits of assessing restoration effectiveness in absence of a standardized definition of success, the context (degree of human impact, ecosystem type, habitat) of where the restoration activity is undertaken is of greater relevance to a successful outcome than how (method) the restoration is carried out. Contrary to expectations, we found that restoration is not necessarily more successful closer to protected areas (PA) and in areas of moderate human impact. This result can be motivated by the limits in assessing the success of interventions and by the tendency of selecting areas in more obvious need of restoration, where the potential of actively restoring a degraded site is more evident. Restoration sites prioritization considering human uses and conservation status present in the region is of vital importance to obtain the intended outcomes and galvanize further actions.
Introduction
Despite increasing efforts to conserve marine-coastal ecosystems, global analyses show unprecedented rates of loss and change at all levels of biological diversity (Butchart et al., 2010; Cardinale et al., 2012). Natural recovery of ecosystems can be difficult in a reasonable time frame (Dobson et al., 1997; Lotze et al., 2006). Once a source of impact has been removed, the return to pristine conditions can vary from decades to centuries (Lotze et al., 2011) since based on both intrinsic (e.g., life-histories traits, ecosystem-specific features) and extrinsic (e.g., type and magnitude of disturbance) factors (Worm et al., 2006). Thus, current conservation initiatives aimed at mitigating human threats (i.e., termed as passive or unassisted restoration) can be insufficient to halt or reverse trajectories of change (Perrow and Davy, 2002; McCrackin et al., 2017; Jones et al., 2018; Lindegren et al., 2018). To this end, there is a need to diversify our approaches and active restoration (i.e., the process of actively assisting the recovery of an ecosystem that has been degraded, damaged, or destroyed; Society for Ecological Restoration International Science Policy Working Group, 2004) is considered an effective strategy to supplement current conservation and management actions when the natural recovery of ecosystems is precluded (Perrow and Davy, 2002; Perring et al., 2015; Jones et al., 2018). Recognizing this, the Convention on Biological Diversity and the European Union have dedicated restoration targets (EU, 2011; CBD, 2014), and in 2019 the “United Nation Decade on ecosystem restoration 2021–2030” has been declared (Waltham et al., 2020). In order to capitalize on the current political and societal will and deliver the changes required, it is imperative that restoration actions are efficient and evidence based.
When successful, restoration of marine-coastal systems can provide a myriad of benefits, relating to climate, biodiversity, economic growth, and physical and mental well-being (Aronson and Alexander, 2013). However, the effects of restoration are still unpredictable in the marine realm (Bayraktarov et al., 2016). As restoration is a costly activity, it is vital that limited conservation funds are spent effectively and the potential is realized in practice to obtain the intended outcomes and galvanize further action. Nevertheless, whilst there is a relatively good understanding of what works in terrestrial systems (Wortley et al., 2013) similar knowledge is lacking in coastal and marine systems (Blignaut et al., 2013). Besides differences in the nature and scale of degrading processes, which historically affect the way we manage biotic resources on land and in the sea (Carr et al., 2003), this discrepancy is also due to marine ecosystems being largely “out-of-view,” leading to a lack of understanding of the degree of degradation and the needs for restoration practices (Parsons et al., 2014), as well as the intrinsic difficulties and elevated costs associated with working in the marine environment (Bayraktarov et al., 2016), especially in remote areas (Van Dover et al., 2014). As a result, several fundamental gaps including current implementation methods and the supporting science still limit the systematic use of ecological restoration in the marine realm (e.g., Elliott et al., 2007; Suding, 2011; Duarte et al., 2015).
Recent scientific reviews have highlighted several challenges and perspectives in marine restoration (e.g., Bayraktarov et al., 2016; Swan et al., 2016; Jacob et al., 2018), starting from a revision of concepts and definitions (Elliott et al., 2007; Abelson et al., 2016). An array of approaches to restoring coastal systems is emerging, falling under four broad categories: transplanting fauna and flora from one site to another, removing invasive species, creating artificial habitat to promote range expansion and recolonization, and inducing changes in hydrological and physical settings each with their own cost and probability of success. However, the method used is only one part of the puzzle: focal species/ecosystem (Montero-Serra et al., 2018), duration of the activity (Bayraktarov et al., 2016), geographical location (Darwiche-Criado et al., 2017), and local factors such as pressures present and conservation level have been identified as relevant in restoration (Keenleyside et al., 2012). Finally, the evaluation of restoration outcomes is not an easy task (Wortley et al., 2013). The SER (Gann et al., 2019) provides a list of key attributes to support the identification of appropriate indicators of restoration success, including six key ecosystem attributes to measure progress along a trajectory of recovery. However, to date, even due to the early developmental stage of marine restoration, restoration success is still typically reported in terms of item-based success (Bayraktarov et al., 2016). Acknowledging limits of assessing restoration effectiveness in absence of a standardized definition of success (e.g., Ruiz-Jaen and Mitchell Aide, 2005), here, we performed a global analysis of published literature aimed to identify the factors increasing the probability of success in restoration actions of coastal and marine systems. Understanding their relative importance is vital for site prioritization and to aid the development of science-based operational protocols.
Materials and Methods
Data Collection, Screening, and Extraction
A systematic literature review was conducted to document active restoration practices (sensu Elliott et al., 2007) across marine and coastal habitats. The review included strictly marine ecosystems (including the intertidal, from shallow habitats to deeper ones) as well as semi-terrestrial systems, such as transitional water bodies (i.e., estuaries, wetlands) and typical interface ecosystems (i.e., mangroves). Two databases (ISI Web of Science and Scopus) were searched using the terms “Restor* OR Rehab*” combined with specific habitat and/or ecosystem types “habitat/ecosystem 1* OR habitat/ecosystem 2*, … OR habitat/ecosystem n”. Searches were undertaken within article titles, abstracts, and keywords. The review was global in scale, and restricted to 1985–2017. This review builds on activities carried out during the first year of the EU MERCES project (http://www.merces-project.eu/) (see also the associated deliverable https://cordis.europa.eu/project/id/689518/results). A total of 4,066 publications were identified, with 498 retained for analysis (see Supplementary Material 1 for details on search terms and eligible criteria). From each article a range of contextual and methodological information was extracted (see Supplementary Material 1, Supplementary Table 1, and the entire catalog in the Supplementary Material 2). However, it has to be stressed that there are major biases in the information that is presented in the literature from the lack of communication and collaboration between practitioners, managers, and scientists, with a large proportion of restoration efforts to date undertaken with little or no scientific input or detailed monitoring. The consequence is that marine restoration projects are often not usually communicated in the scientific literature (Boström-Einarsson et al., 2020).
There is no commonly agreed definition of restoration success. The level of failure, partial success, or success was inferred from each study using the same rationale of Bayraktarov et al. (2016) based on the assessment of item-based metrics such as the survival of manipulated organisms. For instance, we defined a highly successful ecological restoration project/study as one where the restoration goals achieved ≥50% survival of restored organisms for the entire intervention area. We define restoration failure as projects with an outcome of ≤ 10% survival of restored organisms. Partial success was assigned if the outcomes of the intervention were not consistent (i.e., each one ≥50%) across the different item-based metrics (or target species) considered in a study. In those cases where the rationale of Bayraktarov et al. (2016) was not applicable, restoration outcomes were classified as reported by authors in the original study, according to the target set by authors (e.g., Gittman et al., 2016).
Also, the classification of success, partial success, and failure was impracticable in case studies where the criteria to evaluate restoration success were not based on a formal comparison between restored and multiple controls, following the rationale of Before After Control Impact (BACI) design (e.g., Craft et al., 2002; Mora and Burdick, 2013; Flores-Verdugo et al., 2015) as suggested by the SER (Gann et al., 2019). In this case, NA was reported due to the lack in providing the temporal and/or spatial dynamics of target systems.
Retained articles were georeferenced in order to determine the distance between restoration action and documented forms of protection. To do this, we downloaded the public version of the World Database on Protected Areas (WDPA) for April 2018 as a file geodatabase from Protected Planet (http://www.protectedplanet.net/) (IUCN UNEP-WCMC, 2018). We considered all Protected Area (PA) types, except those with a “proposed” or “not reported” status and sites reported as points without an associated reported area. Protected area polygons were dissolved to avoid double counting. The minimum straight distance between the centroid of the restoration action and the boundary of the nearest protected polygon was calculated in kilometers using ArcGIS 10.1. The same software has been used to determine the cumulative human impact score of the location where the study was conducted. In this case, the cell values of the raster based on the map (https://knb.ecoinformatics.org/view/10.5063/F12B8WBS) provided by Halpern et al. (2015) were employed. Where location of the study had no score, due to source raster coverage, the value in the point has been calculated from the adjacent cells with valid values using bilinear interpolation. In Figure 1, the density of records occurrence was obtained by mean of the ArcGIS tool “Point Density [Spatial Analyst] which calculates a magnitude per unit area from point features that fall within a neighborhood around each cell. The cell size for the output raster dataset is 0.42 decimal degrees (DD). The shape of the area around each cell that is used to calculate the density value is circular with the given radius 3.47 (the units of the selected neighborhood measurements in map units). This is default where the radius is the shortest of the width or height of the extent of the input point features, in the output spatial reference, divided by 30.
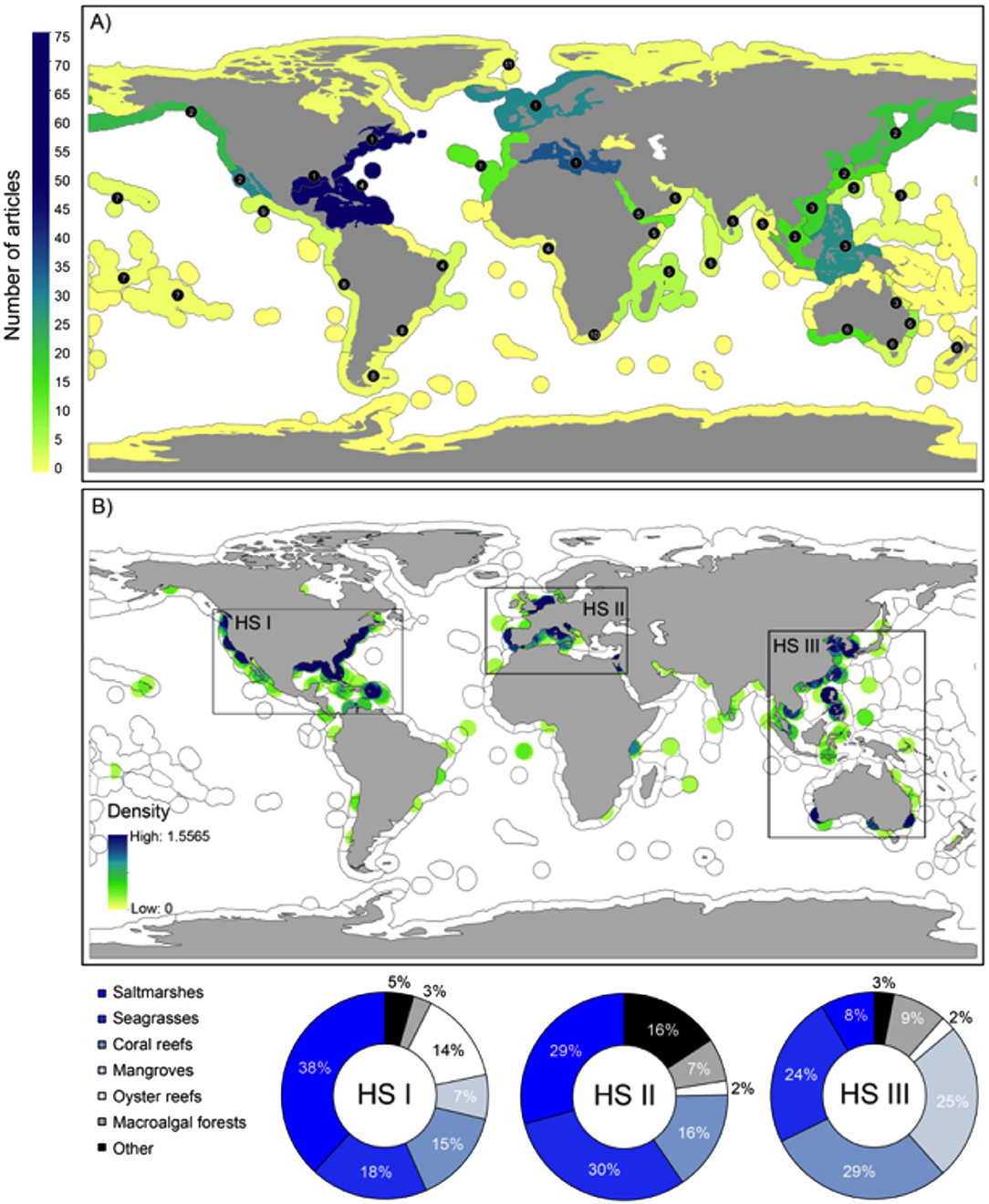
Figure 1. Distributional maps of peer-reviewed studies on active restoration (n = 498) across marine “provinces” from Spalding et al. (2007) showing (A) the total amount of articles; (B) hotspot areas (HS) of restoration efforts in term of articles occurrence density (details provided below). In (A) the numbers indicate for each province the realm they belong to (1. Temperate Northern Atlantic, 2. Temperate Northern Pacific, 3. Central Indo-Pacific, 4. Tropical Atlantic, 5. Western Indo-Pacific, 6. Temperate Australasia, 7. Eastern Indo-Pacific, 8. Temperate South America, 9. Tropical Eastern Pacific, 10. Temperate Southern Africa, 11.Arctic). In (B) the relative frequency of occurrence across habitats for each hotspot area identified (i.e., HSI, HSII, HSIII) is also reported as donut plots. Habitats showing a sporadic occurrence (i.e., <3%) across the overall database (e.g., mudflats, mussel beds, scallops) were grouped in “Other”.
Statistical Analysis
Ordered multinomial logistic regressions were used to identify the most parsimonious models that explain restoration success and determine the relative importance of the potential correlates. This model type was selected as the response variable is categorical and has multiple levels (failure, partial success, and success) and was implemented using the “multinom” function of the R package nnet (Venables and Ripley, 2002).
As there was not a-priori hypothesis, an exhaustive modeling approach was used and all possible interactions between potential explanatory variables and higher order terms considered (Supplementary Material 1, Supplementary Table 2). Often a single “best” model is selected, based on a variety of methods and thresholds including model fit and p-values, however, there is often substantial uncertainty over this selection, with important information contained in the second, third, fourth “best” models that is dismissed. Therefore, arriving at and basing inferences on a single model fails to account for uncertainty. Rather than arriving at, and drawing inferences from, a single “best model,” we used a model averaging approach to generate model estimates that are based on a set of highly probable candidate models and therefore account for and include parameter uncertainty in our results.
Model comparisons were based on the small-sample-size-corrected Akaike's information criterion (AICc). The highest-ranked models according to AICc are those that explain the most substantial proportion of variance in the data yet exclude unnecessary parameters that cannot be justified for inference on the basis of the data. Model competitiveness was determined by the AICc differences (Δi) between each model (i) and the AICc-value of the top-ranked model, with models with Δi > 2 dismissed (Burnham and Anderson, 2002).
Then, for the retained set of models (i.e., Δi < 2), Akaike weights (wi) were calculated to represent the relative likelihood of each model and a 95% confidence set of models was then constructed beginning with the model with the highest weight and then continuously adding the model with the next highest weight, until the cumulative sum of weights exceeded 0.95.
The potential explanatory variables were then averaged across the 95% candidate set of models in order to derive model-averaged results which take into account the uncertainty in the modeled estimates. Finally, the relative importance of each potential explanatory variables was determined by summing the Akaike weights for all models containing the variable. Variables with a summed wi < 0.5 were considered relatively unimportant.
Results
Overview of Literature Analyzed
Since 2000, active restoration initiatives have been carried out across all ecoregional realms, except “Southern Ocean” (sensu Spalding et al., 2007) with the majority (58%) of studies concentrated in the northern hemisphere (i.e., the Temperate Northern Atlantic and Pacific: 41 and 17% of studies, respectively) (Figure 1A). The Tropical Atlantic and the Central Indo-Pacific also show a relevant number of restoration efforts (16 and 14% of studies, respectively). Crucially, the majority of studies were recorded in estuarine/wetland systems (42%), followed by rocky reefs (30% including both intertidal and subtidal), and soft-bottom environments (28%). In particular, studies strictly dealing with marine environments have exceeded those on estuarine/wetland systems since 2008, due to the increasing number of studies carried out in the rocky subtidal (mainly focused on coral reefs) and in soft-bottom systems (mainly seagrass and mangrove habitats).
The global map shows that these efforts are not homogeneously distributed across provinces in each biogeographic realm and several hot spots of restoration initiatives have been identified (Figure 1B). Most studies along the coasts of USA (i.e., HS I) focused on saltmarshes (38%), whilst, across European countries (HS II), seagrass restoration is more represented (30%). Instead, a prevalent number of studies regarded coral reefs (29%) and mangroves (25%) in the central Indo-Pacific (HS III, Figure 1B). It should be noted that all restoration case studies evaluated targeted coastal or shallow water ecosystems with no records for open-ocean or deep-water restoration projects.
A total of 228 species belonging to 118 genera have been targeted by active restoration interventions, the majority being saltmarsh plants and corals (Figure 2A). Most of information gathered from restoration efforts derives from a relatively small subset of species (Supplementary Material, Figures 2B,C), in particular Zostera marina and Posidonia oceanica for seagrasses, Spartina alterniflora and Phragmites australis for saltmarshes, Rhizophora spp. for mangroves, Crassostrea virginica for oyster reefs, Acropora cervicornis and Acropora palmata for coral reefs were the most targeted species (i.e., ≥10 records in the catalog).
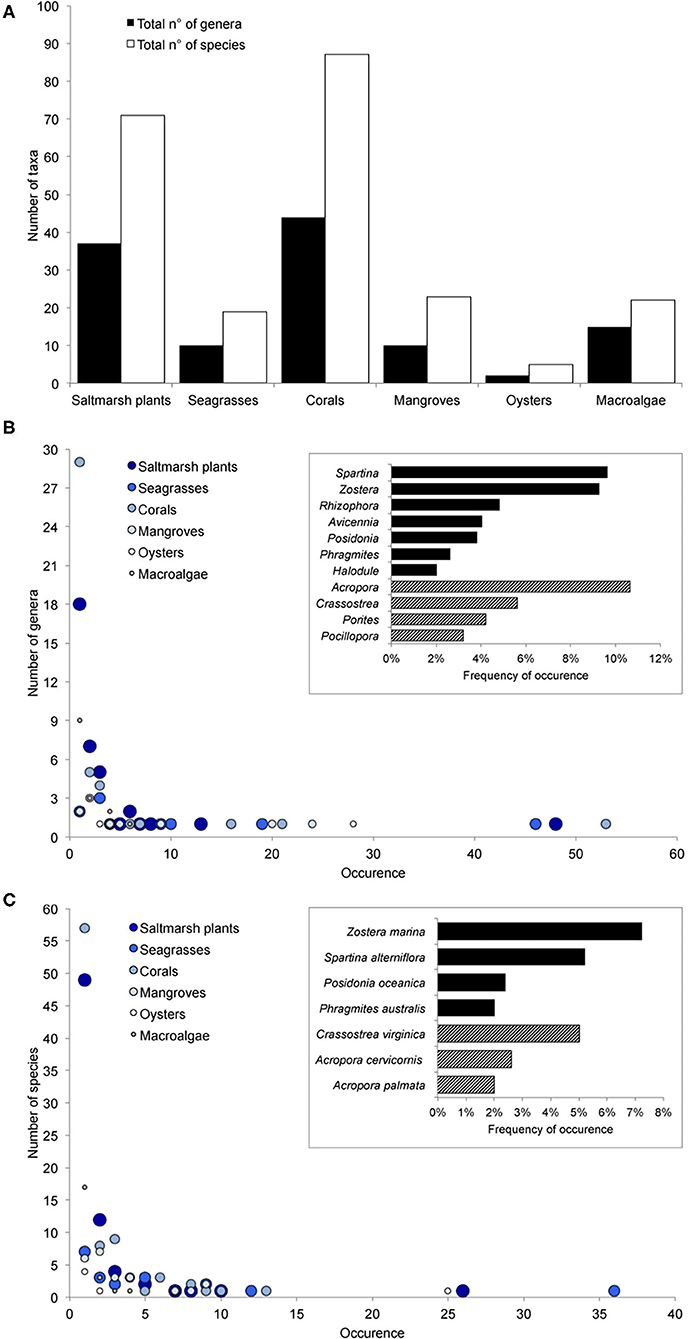
Figure 2. Summary of targeted organisms across studies. (A) Total number of genera and species across the main habitats identified. Rates of occurrence across (B) genera and (C) species for each habitat category. Different colors represent the different hosting habitats. Symbols (circles) have varying dimension so that overlapping is visible. In (B,C) genera and species showing an occurrence ≥2% (i.e., ≥10 records in the catalog) were also reported in the bar plots. Black bars = plants; striped bars = invertebrates.
Across all habitats, 60% of the studies were classified as successful and a further 15% as partially successful (Figure 3). This pattern was consistent across habitats independently from the number of studies. The majority of studies (~60%) last 1–2 years; 30% of saltmarsh, seagrass, and mangrove studies are over 2 years; and 4% (saltmarsh) exceeded 16 years. The majority (54%) cover < 1,000 m2, with a large number between 100 and 1,000 m2, however, some (corals, seagrasses) are at a very small scale (1–10 m2). Saltmarsh habitats have the highest number of records larger than one hectare (13%) (Figures 4A,B). Most studies use transplantation techniques in combination with the establishment of nurseries and the use of artificial structures (~40%). Planting and the modification of physical/hydrological settings (e.g., damming and channeling) are also common (20 and 25%, respectively) (Supplementary Figure 1A).
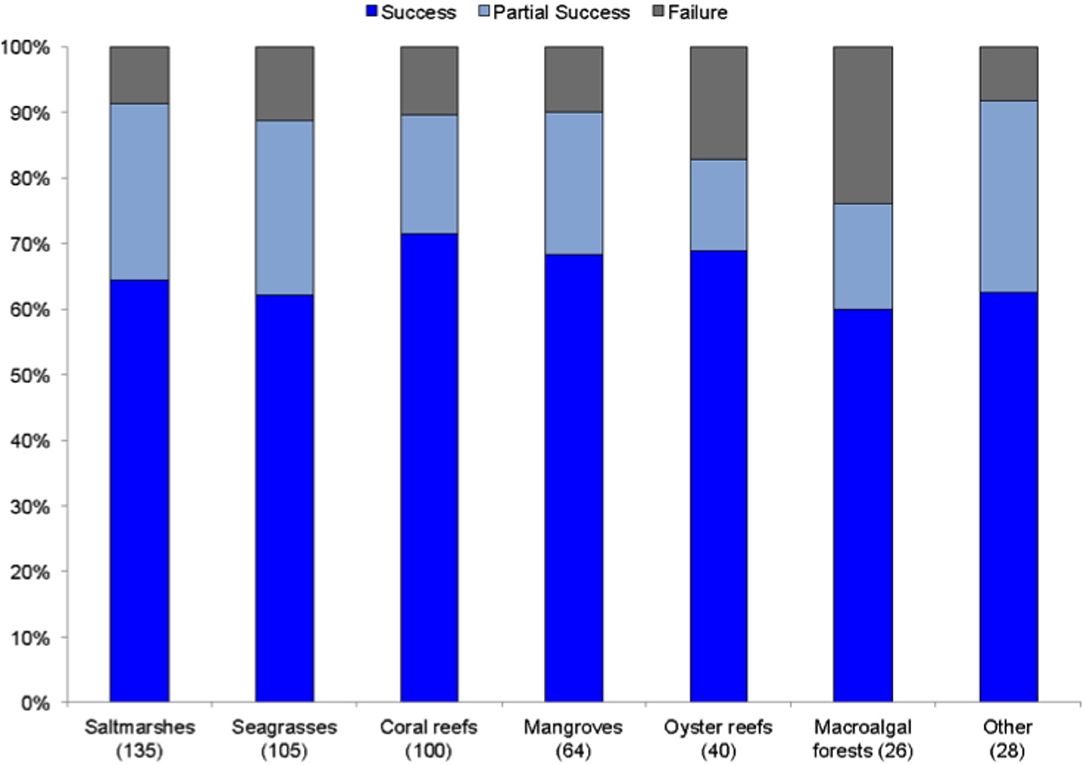
Figure 3. Partitioning (i.e., percentage representativeness) of the restoration outcomes (i.e., from success to failure) across habitats. In brackets the total number of studies for each habitat is also reported. Habitats showing a sporadic occurrence of records (i.e., <3%) across the overall database (e.g., mudflats, mussel beds, scallops) were grouped in “Other”.
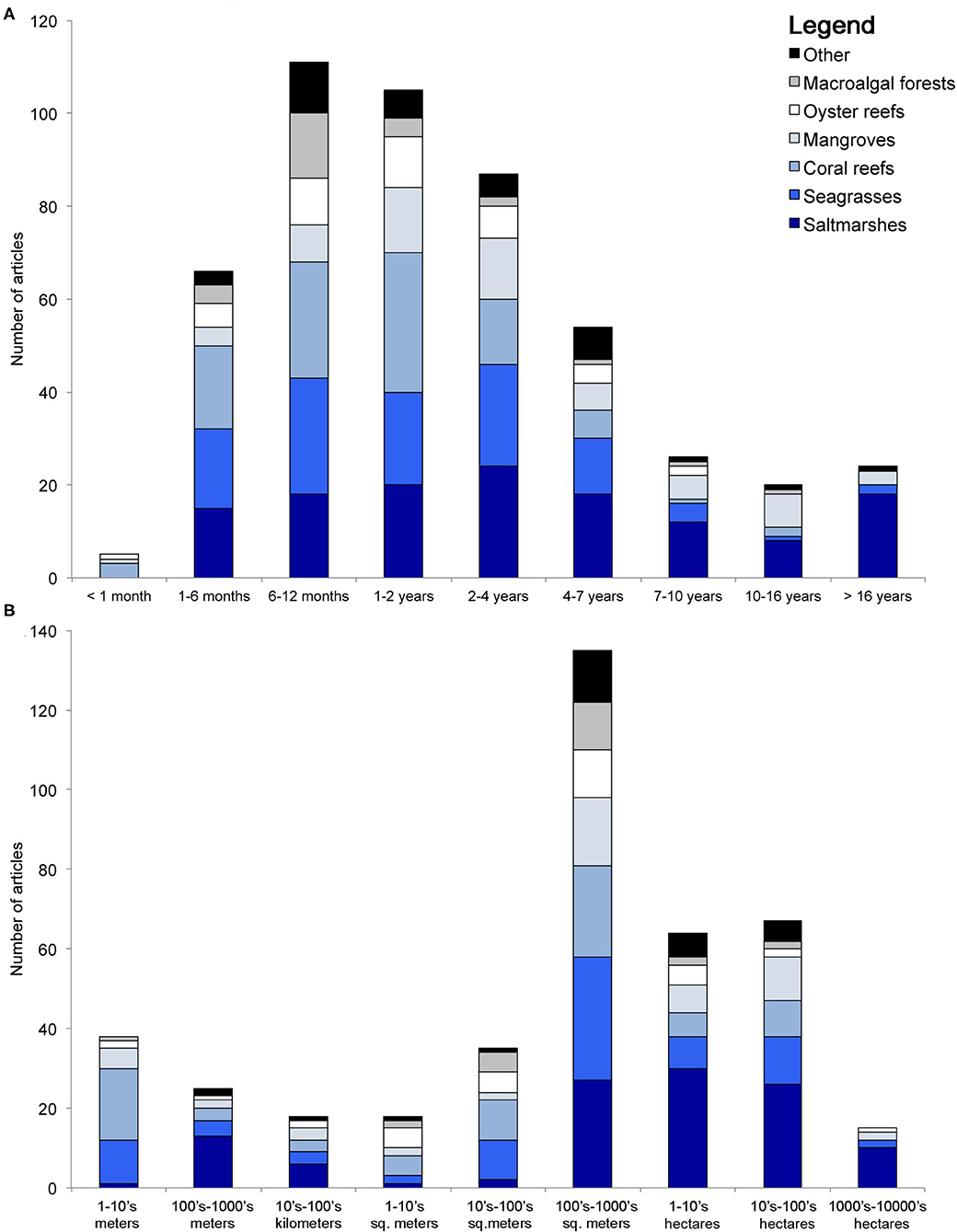
Figure 4. (A) Duration and (B) area covered (i.e., extent) by each study across habitats. Habitats showing a sporadic occurrence (i.e., <3%) in the database (e.g., mudflats, mussel beds, scallops) were grouped in “Other”.
Generally, the most common response variables used to assess the outcomes of the restoration action were item-based. The survival of transplanted organisms, followed by growth measurements were the most commonly-used metrics across studies (i.e., 36 and 28% of occurrence, respectively, Supplementary Figure 1B). Ecological processes like productivity are not measured as frequently as measures of structure or diversity.
Explanatory Variables to Support Restoration Success
The probability of a restorative action being partially or fully successful was found to be predominately influenced by the contextual setting of the action and the habitat being restored rather than the method used (Table 1). For example, the likelihood of success increases in seagrass beds, saltmarsh and macroalgal forests and in areas with higher levels of human impact. However, the importance of methodological factors increased in studies reporting full restoration success, with the re-establishment of habitats in locations they were previously found and the mitigation of pressures being of importance (Figures 5, 6). Considering the wider set of variables in the 95% candidate set, there was considerable variability and uncertainty regarding the influence of the restoration actions, their duration and the geographic realm.
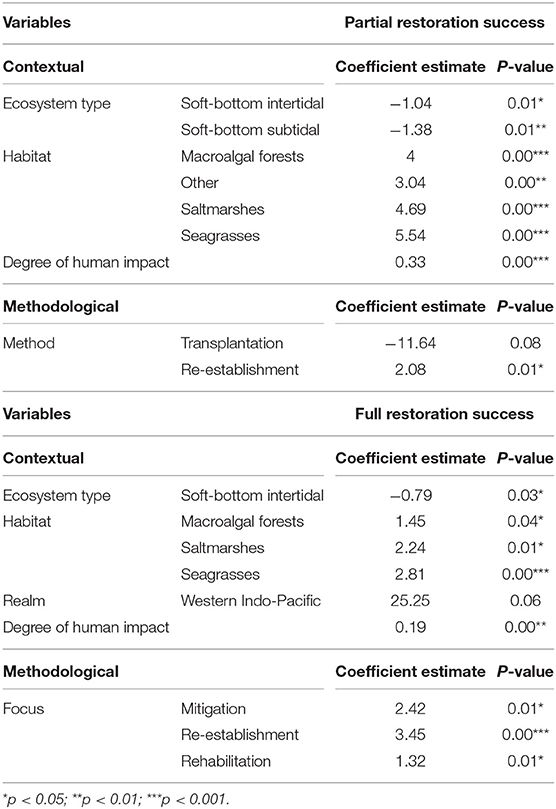
Table 1. The model averaged coefficients of the most informative variables in the 95% confidence set of models for partial and full restoration success.
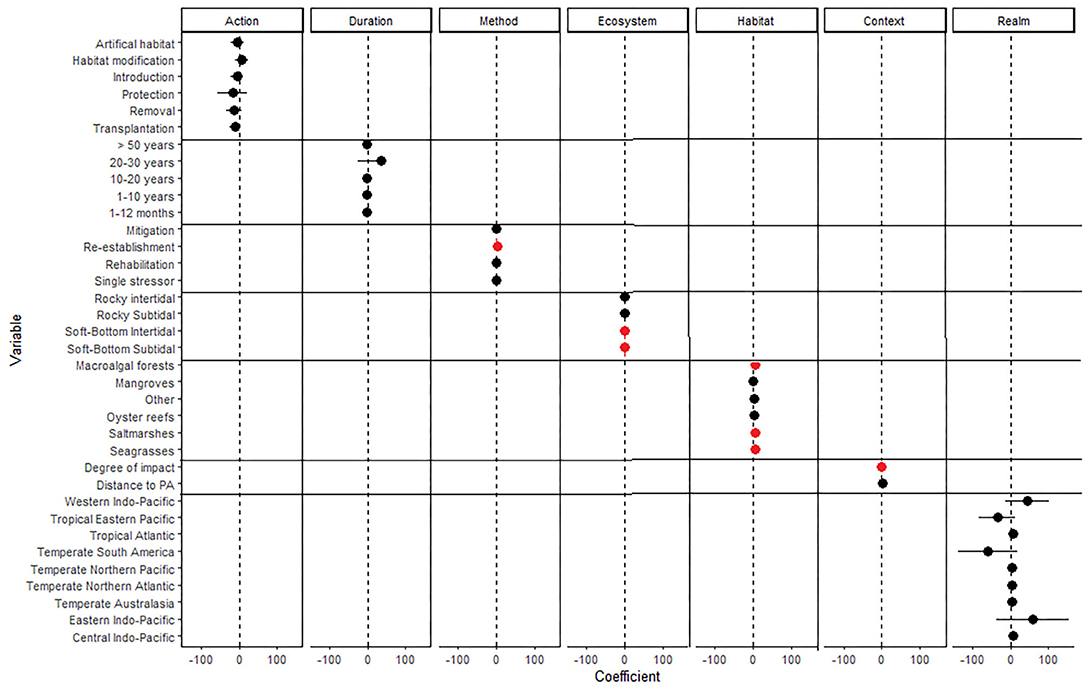
Figure 5. The model averaged coefficients in the 95% confidence set of models for partial restoration success. Red dots indicate a significant effect of that variable on the outcome.
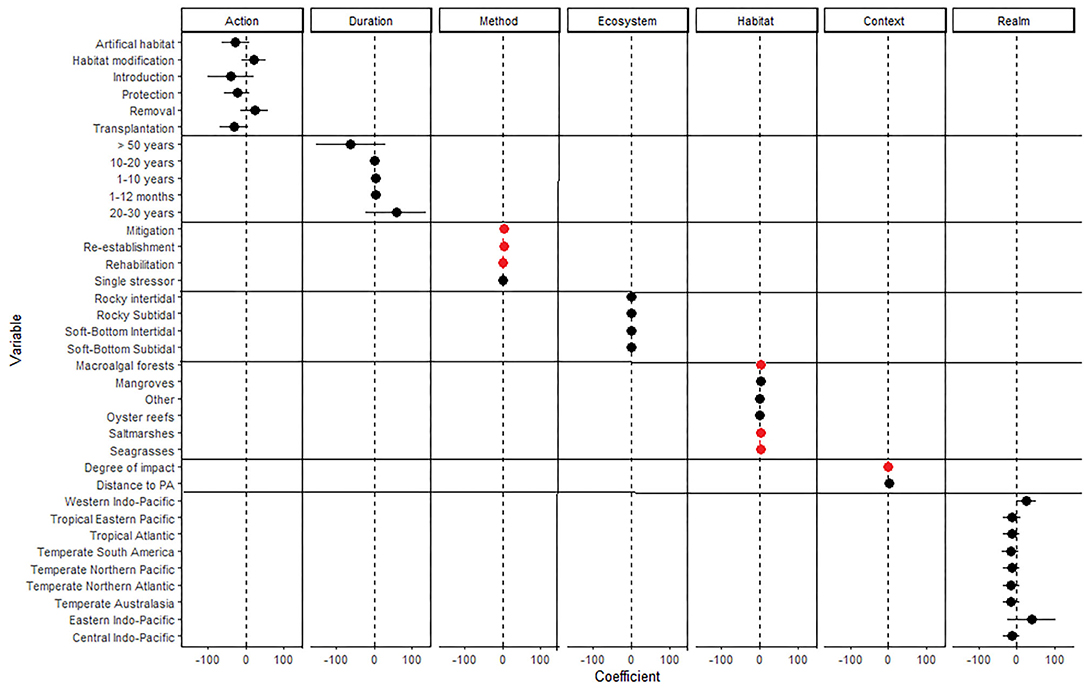
Figure 6. The model averaged coefficients in the 95% confidence set of models for full restoration success. Red dots indicate a significant effect of that variable on the outcome.
Discussion
Overall, the results from this analysis suggest that the context of where a restoration activity is undertaken is generally of greater relevance to a successful outcome than how the restoration is carried out. Where methodological factors were of importance, they related to the re-establishment of lost and the rehabilitation of existing habitats, including the removal of stressors, rather than the creation of novel areas.
The importance of contextual factors, such as the ecosystem type and geographic location in determining restoration success is in accordance with previous findings (e.g., Suding, 2011; Bayraktarov et al., 2016) and demonstrates the vital importance of site selection, for example, in terms of knowledge of the pressures present within the region, its historical context, and the wider seascape. For example, Suding (2011) found that the recovery of a site can often be attributed to a mixture of local and landscape constraints, including shifts in species distribution and legacies of past land use, whilst Bayraktarov et al. (2016) highlighted the importance of site selection and techniques as determinants of restoration success. We also found that methodologies related to “protecting” the restored site, for example through the removal of invasive species (Patten and O'Casey, 2007) or the use of protective mesh cages (Yoon et al., 2014), had a significant positive influence on restoration success.
Despite the evidence that the most damaged ecosystems are the hardest to recover, since they have crossed various thresholds or lost key processes that reinforce the impacted state (Lotze et al., 2011; Ling et al., 2015; Guarnieri et al., 2020), we found that restoration is not necessarily more successful closer to PAs and in areas of moderate human impact. This is perhaps counter-intuitive, however, different reasons could explain the observed results. First, as our measure of success was not objective, and instead based on the view of the authors of the study, it is likely that change (and hence success) is quicker and more obvious in areas which have previously received relatively little protection and have been subjected to higher human impact. Secondly, it is possible that researchers will be more likely to select areas in more obvious need of restoration, where the potential of actively restoring a degraded site is more evident. Third, most existing MPAs are partially protected and partially PAs tend to attract uses rather than decrease them (Dureuil et al., 2018; Zupan et al., 2018) elevating recent concerns about growing human pressure on PAs worldwide. Finally, these patterns are likely to be strongly biased due to the literature reporting more successful rather than failed restoration activities (Hobbs, 2009; Knight, 2009; Suding, 2011). To address this issue, (Boström-Einarsson et al. 2020) produced an online interactive database to act as a resource for practitioners, managers, and scientists to learn from both past successes and failures in coral restoration. However, these attempts are still very limited and how this publication bias might affect the results and their interpretation is still under evaluated.
The restoration of lost and degraded habitats is a long-term process, however, we found no significant influence of the duration of the action and its subsequent monitoring and the probability of success, likely because the outcome of restoration (success or failure) is directly related to the period of observation, which typically lasts as long as the research grant (Bayraktarov et al., 2016, and references therein).
The main reasons of failure (10% of studies) are very heterogeneous going from inadequate site selection (Ferse, 2010; de Paoli et al., 2015), stochastic events (Coates et al., 2014), and methodology (Cooper et al., 2014). A substantial lack of knowledge on the factors driving restoration success together with the absence of protocols and best practices for supporting the recovery of ecosystems are major issues across studies. Typically, with the exception of wetlands and estuarine systems (i.e., mostly saltmarshes and mangroves), our review shows that most restoration projects are still carried out over relatively small areas (<1 ha), failing to match the scale of human disturbance. Whilst small-scale restoration actions can be informative in testing a technique or theory, success should be carried out, and evaluated at multiple spatial scales. This result indicates that efforts should be directed at upscaling marine restoration into the future, in space and time. Several years ago, Zimmer (2006), reported that restoration research on coral reefs had been focused on the development of techniques, rather than on assessing the application of established methodologies in large-scale restoration projects. Unfortunately, the present review indicates that little has changed to date, and many studies are still experimental in nature, covering small spatial and temporal scales.
Whilst the results presented show the great importance of considering where the restoration action takes place, perhaps more than how it will be done, it is important to recognize that there is no single or simple reason why restoration actions fail or success. This will vary significantly over spatial scale, be influenced by a wide range of processes operating at very local to regional scales and relate to socio-economic, institutional, and policy factors. However, while the scientific literature on marine ecosystem restoration is rich with studies comparing alternative restoration techniques within a single site (West et al., 2000; Dizon et al., 2008; Busch et al., 2010; Verdura et al., 2018), less attention has been paid to the potentially equally important impacts of decisions about where to conduct restoration in the first place (Lester et al., 2020). Finding suitable areas where to plan active restoration actions is a crucial step in this process and requires the adoption of the principles and tools generally adopted in the framework of marine spatial planning, conservation planning, and spatial ecology. It is not an easy task since it requires fine-scale data about past and present distribution of the target of restoration and of human uses together with the knowledge of environmental variables and local socio-economical features potentially affecting the outcomes of restoration. In this sense, a limit of the study is that our attempts to include the consideration of the degree of human impact was estimated from a single snapshot in time, which may not be temporally consistent with the start of the restoration effort given that duration of studies compiled in the analysis range from less than a month to >50 years (see Figures 5, 6).
In order to evaluate the success of a restoration effort, the criteria for success must first be set. Another limitation and a recurring theme (Ruiz-Jaen and Mitchell Aide, 2005) in the analysis of restoration outcome across studies is the lack of a standardized approach for defining “success” and the subjective nature by which it is assessed. Measures of “success,” “partial success,” and “failure” are highly heterogeneous and vaguely reported, the majority using measures of survival or growth without defined and measurable a priori targets, or reference sites (e.g., Correa et al., 2006; Garrison and Ward, 2008; Balestri and Lardicci, 2012; Irving et al., 2014; Zhang et al., 2015), a problem that continues to persist (Suding, 2011) representing thus a potential crucial bias in the interpretation of the results. In addition, the lack of knowledge of pre-disturbance baselines, which may have shifted along with climate change (Pauly, 1995; Bekkby et al., 2020), is also a challenge. Ultimately, this hampers a proper evaluation of the impact of anthropogenic activities, the actual degree of degradation, and therefore the choice of the restoration goals.
Where criteria for success were stated explicitly, they typically referred to ecological attributes related mostly to simple metrics such as survival, cover, and abundance (up to 50%) of a target species, rarely considering the recovery of the wider community, and ecosystem functions or services (up to 20%), as also documented in similar analyses (e.g., Wortley et al., 2013). Furthermore, whilst a number of studies have provided guidance for the use of consistent and standardized terminology (e.g., Elliott et al., 2007; Duarte et al., 2015), they remain inconsistent, with conflicting and sometimes overlapping terms which further hamper attempts to provide objective and comparative information concerning the success rates of restoration interventions (Ruiz-Jaen and Mitchell Aide, 2005; Elliott et al., 2007; Abelson et al., 2016).
Our analysis also highlights a bias in the species being restored and the regions in which this is taking place. Therefore, the generality of our findings and impact on our ability to develop effective restoration actions in certain regions or habitats were limited. For example, there is a bias toward studies carried out in the USA and Europe, which generate results that are context dependent and, thus, not extendable to other socio-ecological contexts. The bias is likely due to the fact that coastal waters in the USA and Europe are amongst the most globally impacted ones (Halpern et al., 2015), and there is significant funding available to undertake restoration; the “restoration economy” contributes annually over $24 billion and 221,000 jobs in the USA (BenDor et al., 2015), which is not the case in other regions and countries.
Furthermore, there is also a substantial focus on semi-terrestrial systems (predominantly saltmarshes), compared to “strictly marine” environments (e.g., seagrasses), which poses similar problems to those outlined above and hampers our ability to make generalizations to novel regions and habitats such as the deep sea. This bias likely stems from the higher costs of restoration in the marine environment (Bayraktarov et al., 2016), coupled with the intrinsic features of this system (e.g., being less stable/manageable compared to confined environments).
Finally, restoration actions could be reliable and profitable strategies to return ecosystems to their original state in a reasonable time frame (Rohr et al., 2018), while representing a smart economic objective to achieve a sustainable future (Duarte et al., 2020). However, our knowledge of how restoration protocols will work under different scenarios of global change is practically nil. We could expect that the reliability and efficacy of restoration protocols could be undermined by increasing temperature, ocean acidification, nutrient, and oxygen availability shifts. In this sense, we need to enhance our ability to use restoration to foster marine ecosystem resilience to global change (Timpane-Padgham et al., 2017).
Recommendations
Under the UN Decade of Ecosystem Restoration, there are several issues and questions whose understanding is indispensable in order to drive future research and policies of restoration, and assist funders and programme managers in allocating funds and planning projects (Ockendon et al., 2018; Waltham et al., 2020). The following recommendations emerge from our analysis:
• The role of contextual variables (e.g., the type and state of the ecosystem being restored, the relative degree of human impact in the area) rather than methodological variables (e.g., how the restoration activity can be conducted) should be carefully assess in determining restoration outcome. Restoration needs fine scale knowledge of both human uses and ecosystem status to be successful.
• A process of spatial prioritization is critical for marine ecosystem restoration, this suggesting a need to explore how spatial planning tools and principles could be applied to restoration practice, to identify the areas with the highest potential for recovery.
• Revise and standardize concepts of restoration, including what constitutes “success” and what the desired target state is for restoration projects. However, without baseline ecological information on species and habitats combined with the knowledge about their status and causes of degradation, providing measures of success, and identify restoration targets will be always difficult.
• There is a need to follow the approaches taken in conservation, and shift from single species restoration actions, and targets to an ecosystem level restoration, recognizing the importance of addressing the complexity of the ecological interaction across systems.
• Most restoration projects were carried out over relatively small areas (<1 ha) which fails to match the scale of human disturbance. Whilst small-scale restoration actions can be informative in testing a technique, success should be evaluated at multiple spatial scales.
• In this respect, it is imperative to create opportunities for public-private partnerships and market-based incentives for businesses and individuals within restoration initiatives. Scaling up will be impossible without a concrete convergence among scientists, private sectors, and stakeholders leading to a share vision in order to maximize synergies and avoid trade-offs between priorities for restoring biodiversity, mitigating threats, and adapting to climate change.
Data Availability Statement
The datasets presented in this study can be found in online repositories. The names of the repository/repositories and accession number(s) can be found in the article/Supplementary Material.
Author Contributions
SF conceived the study. SF, GG, and LP developed criteria for the literature review. CM analyzed the data. SF, GG, CM, and NP wrote a first draft of the manuscript. All authors contributed to data collection and manuscript revision.
Funding
Research funded by the EU project MERCES of the European Union's Horizon 2020 research (Grant agreement No. 689518, http://www.merces-project.eu).
Conflict of Interest
The authors declare that the research was conducted in the absence of any commercial or financial relationships that could be construed as a potential conflict of interest.
Publisher's Note
All claims expressed in this article are solely those of the authors and do not necessarily represent those of their affiliated organizations, or those of the publisher, the editors and the reviewers. Any product that may be evaluated in this article, or claim that may be made by its manufacturer, is not guaranteed or endorsed by the publisher.
Supplementary Material
The Supplementary Material for this article can be found online at: https://www.frontiersin.org/articles/10.3389/fmars.2021.626843/full#supplementary-material
References
Abelson, A., Halpern, B., Reed, D. C., Orth, R. J., Kendrick, G. A., Beck, M. W., et al. (2016). Upgrading marine ecosystem restoration using ecological-social concepts. Bioscience 66, 156–163. doi: 10.1093/biosci/biv171
Aronson, J., and Alexander, S. (2013). Ecosystem restoration is now a global priority: time to roll up our sleeves. Restor. Ecol. 21, 293–296. doi: 10.1111/rec.12011
Balestri, E., and Lardicci, C. (2012). Nursery-propagated plants from seed: a novel tool to improve the effectiveness and sustainability of seagrass restoration. J. Appl. Ecol. 49, 1426–1435. doi: 10.1111/j.1365-2664.2012.02197.x
Bayraktarov, E., Saunders, M. I., Abdullah, S., Mills, M., Beher, J., Possingham, H. P., et al. (2016). The cost and feasibility of marine coastal restoration. Ecol. Appl. 26, 1055–1074. doi: 10.1890/15-1077
Bekkby, T., Papadopoulou, N., Fiorentino, D., McOwen, C., Rinde, E., Boström, C., et al. (2020). Habitat features and their influence on the restoration potential of marine habitats in Europe. Front. Mar. Sci. 7:184. doi: 10.3389/fmars.2020.00184
BenDor, T., Lester, T. W., Livengood, A., Davis, A., and Yonavjak, L. (2015). Estimating the size and impact of the ecological restoration economy. PLoS ONE 10:e0128339 doi: 10.1371/journal.pone.0128339
Blignaut, J., Esler, K. J., de Wit, M. P., Le Maitre, D., Milton, S. J., and Aronson, J. (2013). Establishing the links between economic development and the restoration of natural capital. Curr. Opin. Environ. Sustain. 5, 94–101. doi: 10.1016/j.cosust.2012.12.003
Boström-Einarsson, L., Babcock, R. C., Bayraktarov, E., Ceccarelli, D., Cook, N., Ferse, S. C. A., et al. (2020). Coral restoration - a systematic review of current methods, successes, failures and future directions. PLoS ONE 15:e0226631 doi: 10.1371/journal.pone.0226631
Burnham, K. P., and Anderson, D. R. (2002). Model Selection and Multimodel Inference: A Practical Information-Theoretic Approach. 2nd Edn. New York, NY: Springer.
Busch, K. E., Golden, R. R., Parham, T. A., Karrh, L. P., Lewandowski, M. J., and Naylor, M. D. (2010). Large-scale Zostera marina (eelgrass) restoration in Chesapeake Bay, Maryland, USA. Part I: a comparison of techniques and associated costs. Restor. Ecol. 18, 490–500. doi: 10.1111/j.1526-100X.2010.00690.x
Butchart, S. H. M., Walpol, M., Collen, B., Van Strien, A., Scharlemann, J. P. W., Almond, R. E. A., et al. (2010). Global biodiversity: indicators of recent declines. Science 328, 1164–1168. doi: 10.1126/science.1187512
Cardinale, B. J., Duffy, J. E., Gonzales, A., Hooper, D. U., Perrings, C., Venail, P., et al. (2012). Biodiversity loss and its impact on humanity. Nature 486, 59–67. doi: 10.1038/nature11148
Carr, M. H., Neigel, J. E., Estes, J. A., Andelman, S., Warner, R., and Largier, J. L. (2003). Comparing marine and terrestrial ecosystems: implications for the design of coastal marine reserves. Ecol. Appl. 13, 90–107. doi: 10.1890/1051-0761(2003)0130090:CMATEI2.0.CO
CBD. (2014). Strategic Plan for Biodiversity 2011–2020 and the Aichi Targets. Secretariat of the Convention on Biological Diversity, Quebec.
Coates, J. H., Hovel, K. A., Butler, J. L., and Bohonak, A. J. (2014). Recruitment and recovery of pink abalone (Haliotis corrugata) in a historically overexploited kelp forest: are local populations self-sustaining? J. Exp. Mar. Biol. Ecol. 460, 184–192. doi: 10.1016/j.jembe.2014.07.004
Cooper, W. T., Lirman, D., VanGroningen, M. P., Parkinson, J. E., Herlan, J., and McManus, J. W. (2014). Assessing techniques to enhance early post-settlement survival of corals in situ for reef restoration. Bull. Mar. Sci. 90, 651–664. doi: 10.5343/bms.2013.1020
Correa, J. A., Lagos, N. A., Medina, M. H., Castilla, J. C., Cerda, M., Ramírez, M., et al. (2006). Experimental transplants of the large kelp Lessonia nigrescens (Phaeophyceae) in high-energy wave exposed rocky intertidal habitats of northern Chile: experimental, restoration and management applications. J. Exp. Mar. Biol. Ecol. 335, 13–18. doi: 10.1016/j.jembe.2006.02.010
Craft, C., Broome, S., and Campbell, C. (2002). Fifteen years of vegetation and soil development after brackish-water marsh creation. Restor. Ecol. 10, 248–258. doi: 10.1046/j.1526-100X.2002.01020.x
Darwiche-Criado, N., Sorando, R., Eismann, S. G., and Comín, F. A. (2017). Comparing two multi-criteria methods for prioritizing wetland restoration and creation sites based on ecological, biophysical and socio-economic factors. Water Resour. Manage. 31, 1227–1241. doi: 10.1007/s11269-017-1572-2
de Paoli, H., van de Koppel, J., van der Zee, E., Kangeri, A., van Belzen, J., Holthuijsen, S., et al. (2015). Processes limiting mussel bed restoration in the Wadden-Sea. J. Sea Res. 103, 42–49. doi: 10.1016/j.seares.2015.05.008
Dizon, R. M., Edwards, A. J., and Gomez, E. D. (2008). Comparison of three types of adhesives in attaching coral transplants to clam shell substrates. Aquat. Conserv. Mar. Freshwater Ecosyst. 18, 1140–1148. doi: 10.1002/aqc.944
Dobson, A. P., Bradshaw, A. D., and Baker, A. J. M. (1997). Hopes for the future: restoration ecology and conservation biology. Science 277, 515–522. doi: 10.1126/science.277.5325.515
Duarte, C. M., Agusti, S., Barbier, E., Britten, G. L., Castilla, J. C., Gattuso, J. P., et al. (2020). Rebuilding and restoring marine life represents a smart economic objective to achieve a sustainable future. Nature 580, 39–51. doi: 10.1038/s41586-020-2146-7
Duarte, C. M., Borja, A., Carstensen, J., Elliott, M., Krause-Jensen, D., and Marbà, N. (2015). Paradigms in the recovery of estuarine and coastal ecosystems. Estuar. Coasts 38, 1202–1212. doi: 10.1007/s12237-013-9750-9
Dureuil, M., Boerder, K., Burnett, K. A., Froese, R., and Worm, B. (2018). Elevated trawling inside protected areas undermines conservation outcomes in a global fishing hot spot. Science 362, 1403–1407. doi: 10.1126/science.aau0561
Elliott, M., Burdon, D., Hemingway, K. L., and Apitz, S. E. (2007). Estuarine, coastal and marine ecosystem restoration: confusing management and science – a revision of concepts. Estuar. Coast Shelf Sci. 74, 349–366. doi: 10.1016/j.ecss.2007.05.034
Ferse, S. C. A. (2010). Poor performance of corals transplanted onto substrates of short durability. Restor. Ecol. 18, 399–407. doi: 10.1111/j.1526-100X.2010.00682.x
Flores-Verdugo, F., Zebadua-Penagos, F., and Flores-de-Santiago, F. (2015). Assessing the influence of artificially constructed channels in the growth of afforested black mangrove (Avicennia germinans) within an arid coastal region. J. Environ. Manage. 160, 113–120. doi: 10.1016/j.jenvman.2015.06.024
Gann, G. D., McDonald, T., Walder, B., Aronson, J., Nelson, C. R., Jonson, J., et al. (2019). International principles and standards for the practice of ecological restoration. Second edition. Restor. Ecol. 27, S1–S46. doi: 10.1111/rec.13035
Garrison, V., and Ward, G. (2008). Storm-generated coral fragments - a viable source of transplants for reef rehabilitation. Biol. Conserv. 141, 3089–3100. doi: 10.1016/j.biocon.2008.09.020
Gittman, R. K., Peterson, C. H., Currin, C. A., Joel Fodrie, F., Piehler, M. F., and Bruno, J. F. (2016). Living shorelines can enhance the nursery role of threatened estuarine habitats. Ecol. Appl. 6, 249–263. doi: 10.1890/14-0716
Guarnieri, G., Bevilacqua, S., Figueras, N., Tamburello, L., and Fraschetti, S. (2020). Large-scale sea urchin culling drives the reduction of subtidal barren grounds in the Mediterranean Sea. Front. Mar. Sci. 7:519. doi: 10.3389/fmars.2020.00519
Halpern, B. S., Frazier, M., Potapenko, J., Casey, K. S., Koening, K., Longo, C., et al. (2015). Spatial and temporal changes in cumulative human impacts on the world's ocean. Nat. Commun. 6:7615 doi: 10.1038/ncomms8615
Hobbs, R. J. (2009). Looking for the silver lining: making the most of failure. Restor. Ecol. 17, 1–3. doi: 10.1111/j.1526-100X.2008.00505.x
Irving, A. D., Tanner, J. E., and Collings, G. J. (2014). Rehabilitating seagrass by facilitating recruitment: improving chances for success. Restor. Ecol. 22, 134–141. doi: 10.1111/rec.12036
IUCN UNEP-WCMC. (2018). The World Database on Protected Areas (WDPA). Available online at: www.protectedplanet.net (accessed April 1, 2018).
Jacob, C., Buffard, A., Pioch, S., and Thorin, S. (2018). Marine ecosystem restoration and biodiversity offset. Ecol. Eng. 120, 585–594. doi: 10.1016/j.ecoleng.2017.09.007
Jones, H. P., Jones, P. C., Barbier, E. B., Blackburn, R. C., Benayas, J. M. R., Holl, K. D., et al. (2018). Restoration and repair of Earth's damaged ecosystems. Proc. Roy. Soc. B 285:20172577. doi: 10.1098/rspb.2017.2577
Keenleyside, K., Dudley, N., Cairns, S., Hall, C. M., and Stolton, S. (2012). Ecological Restoration for Protected Areas: Principles, Guidelines and Best Practices. Gland: IUCN. p. 120. Available online at: https://portals.iucn.org/library/sites/library/files/documents/PAG-018.pdf (accessed September 20, 2021).
Knight, A. T. (2009). Is conservation biology ready to fail? Conserv. Biol. 23:517. doi: 10.1111/j.1523-1739.2009.01222.x
Lester, S. E., Dubel, A. K., Hernán, G., McHenry, J., and Rassweiler, A. (2020). Spatial planning principles for marine ecosystem restoration. Front. Mar. Sci. 7:328. doi: 10.3389/fmars.2020.00328
Lindegren, M., Holt, B. G., MacKenzie, B. R., and Rahbek, C. (2018). A global mismatch in the protection of multiple marine biodiversity components and ecosystem services. Sci. Rep. 8:4099. doi: 10.1038/s41598-018-22419-1
Ling, S. D., Scheibling, R. E., Rassweiler, A., Johnson, C. R., Shears, N., Connell, S. D., et al. (2015). Global regime shift dynamics of catastrophic sea urchin overgrazing. Philos. Trans. Roy. Soc. B 370:20130269. doi: 10.1098/rstb.2013.0269
Lotze, H. K., Coll, M., Magera, A. M., Ward-Paige, C., and Airoldi, L. (2011). Recovery of marine animal populations and ecosystems. Trends Ecol. Evol. 26, 595–605. doi: 10.1016/j.tree.2011.07.008
Lotze, H. K., Lenihan, H. S., Bourque, B. J., Bradbury, R. H., Cooke, R. G., Kay, M. C., et al. (2006). Depletion, degradation, and recovery potential of estuaries and coastal seas. Science 312, 1806–1809. doi: 10.1126/science.1128035
McCrackin, M., Jones, H. P., Mateo, D. M., and Jones, P. C. (2017). Incomplete recovery of lakes and coastal marine ecosystems from eutrophication: a global metaanalysis. Limnol. Oceanogr. 62, 507–518. doi: 10.1002/lno.10441
Montero-Serra, I., Garrabou, J., Doak, D. F., Figuerola, L., Hereu, B., Ledoux, J. B., et al. (2018). Accounting for life-history strategies and timescales in marine restoration. Conserv. Lett. 11:e12341. doi: 10.1111/conl.12341
Mora, J. W., and Burdick, D. M. (2013). Effects of man-made berms upon plant communities in New England salt marshes. Wetlands Ecol. Manage. 21, 131–145. doi: 10.1007/s11273-013-9285-7
Ockendon, N., Thomas, D. H. L, Cortina, J., Adams, W. M., Aykroyd, T., Barov, B., et al. (2018). One hundred priority questions for landscape restoration in Europe. Biol. Conserv. 221, 198–208. doi: 10.1016/j.biocon.2018.03.002
Parsons, E. C. M., Favaro, B., Aguirre, A. A., Bauer, A. L., Blight, L. K., Cigliano, J. A., et al. (2014). Seventy-one important questions for the conservation of marine biodiversity. Conserv. Biol. 28, 1206–1214. doi: 10.1111/cobi.12303
Patten, K., and O'Casey, C. (2007). Use of Willapa Bay, Washington, by shorebirds and waterfowl after Spartina control efforts. J. Field Ornithol. 78, 395–400. doi: 10.1111/j.1557-9263.2007.00128.x
Pauly, D. (1995). Anecdotes and the shifting baseline syndrome of fisheries. Trends Ecol. Evol. 10:430. doi: 10.1016/S0169-5347(00)89171-5
Perring, M. P., Standish, R. J., Price, J. N., Craig, M. D., Erickson, T. E., Ruthrof, K. X., et al. (2015). Advances in restoration ecology: rising to the challenges of the coming decades. Ecosphere 6:131. doi: 10.1890/ES15-00121.1
Perrow, M., and Davy, A. (2002). Handbook of Ecological Restoration. Cambridge: Cambridge University Press. doi: 10.1017/CBO9780511549984
Rohr, J. R., Bernhardt, E., Cadotte, M. W., and Clements, W. (2018). The ecology and economics of restoration: when, what, where, and how to restore ecosystems. Ecol. Soc. 23:15 doi: 10.5751/ES-09876-230215
Ruiz-Jaen, M. C., and Mitchell Aide, T. (2005). Restoration success: how is it being measured? Restor. Ecol. 13, 569–577. doi: 10.1111/j.1526-100X.2005.00072.x
Society for Ecological Restoration International Science Policy Working Group. (2004). The SER International Primer on Ecological Restoration. Tucson: Society for Ecological Restoration. Available online at: https://www.ser-rrc.org/resource/the-ser-international-primer-on/
Spalding, M. D., Fox, H. E., Allen, G. R., Davidson, N., Ferdaña, Z. A., Finlayson, M., et al. (2007). Marine ecoregions of the world: a bioregionalization of coastal and shelf areas. Bioscience 57, 573–583. doi: 10.1641/B570707
Suding, K. N. (2011). Toward an era of restoration in ecology: successes, failures, and opportunities ahead. Annu. Rev. Ecol. Evol. Syst. 42, 465–487. doi: 10.1146/annurev-ecolsys-102710-145115
Swan, K. D., McPherson, J. M., Seddon, P. J., and Moehrenschlager, A. (2016). Managing marine biodiversity: the rising diversity and prevalence of marine conservation translocations. Conserv. Lett. 9, 239–251. doi: 10.1111/conl.12217
Timpane-Padgham, B. L., Beechie, T., and Klinger, T. (2017). A systematic review of ecological attributes that confer resilience to climate change in environmental restoration. PLoS ONE 12:e0173812. doi: 10.1371/journal.pone.0173812
Van Dover, C. L., Aronson, J., Pendleton, L., Smith, S., Arnaud-Haond, S., Moreno-Mateos, D., et al. (2014). Ecological restoration in the deep sea: desiderata. Mar. Policy 44, 98–106. doi: 10.1016/j.marpol.2013.07.006
Venables, W. N., and Ripley, B. D. (2002). Modern Applied Statistics with S. 4h Edn. New York, NY: Springer. doi: 10.1007/978-0-387-21706-2
Verdura, J., Sales, M., Ballesteros, E., Cefalì, M. E., and Cebrian, E. (2018). Restoration of a canopy-forming alga based on recruitment enhancement: methods and long-term success assessment. Front. Plant Sci. 9:1832. doi: 10.3389/fpls.2018.01832
Waltham, N. J. M, Elliott, S. Y., Lee, C., Lovelock, C. M., Duarte, C., et al. (2020). UN Decade on Ecosystem Restoration 2021–2030 - what chance for success in restoring coastal ecosystems? Front. Mar. Sci. 7:71. doi: 10.3389/fmars.2020.00071
West, T. L., Clough, L. M., and Ambrose, W. G. (2000). Assessment of function in an oligohaline environment: lessons learned by comparing created and natural habitats. Ecol. Eng. 15, 303–321. doi: 10.1016/S0925-8574(00)00083-5
Worm, B., Barbier, E. B., Beaumont, N., Duffy, J. E., Folke, C., Halpern, B. S., et al. (2006). Impacts of biodiversity loss on ocean ecosystem services. Science 314, 787–790. doi: 10.1126/science.1132294
Wortley, L., Hero, J. M., and Howes, M. (2013). Evaluating ecological restoration success: a review of the literature. Restor. Ecol. 5, 537–543. doi: 10.1111/rec.12028
Yoon, J. T., Sun, S. M., and Chung, G. (2014). Sargassum bed restoration by transplantation of germlings grown under protective mesh cage. J. Appl. Phycol. 26, 505–509. doi: 10.1007/s10811-013-0058-8
Zhang, P. D., Fang, C., Liu, J., Xu, Q., Li, W. T., and Liu, Y. S. (2015). An effective seed protection method for planting Zostera marina (eelgrass) seeds: implications for their large-scale restoration. Mar. Pollut. Bull. 95, 89–99. doi: 10.1016/j.marpolbul.2015.04.036
Zimmer, B. (2006). “Coral reef restoration: an overview,” in Coral Reef Restoration Handbook, ed W. F. Precht (Boca Raton, FL: CRC Press), pp. 39–59. doi: 10.1201/9781420003796.ch3
Keywords: active restoration, marine habitats, restoration effectiveness, restoration success and failure, restoration site prioritization
Citation: Fraschetti S, McOwen C, Papa L, Papadopoulou N, Bilan M, Boström C, Capdevila P, Carreiro-Silva M, Carugati L, Cebrian E, Coll M, Dailianis T, Danovaro R, De Leo F, Fiorentino D, Gagnon K, Gambi C, Garrabou J, Gerovasileiou V, Hereu B, Kipson S, Kotta J, Ledoux J-B, Linares C, Martin J, Medrano A, Montero-Serra I, Morato T, Pusceddu A, Sevastou K, Smith CJ, Verdura J and Guarnieri G (2021) Where Is More Important Than How in Coastal and Marine Ecosystems Restoration. Front. Mar. Sci. 8:626843. doi: 10.3389/fmars.2021.626843
Received: 06 November 2020; Accepted: 13 September 2021;
Published: 12 October 2021.
Edited by:
Anna Metaxas, Dalhousie University, CanadaReviewed by:
Karen Filbee-Dexter, Laval University, CanadaRachel Kelley Gittman, East Carolina University, United States
Copyright © 2021 Fraschetti, McOwen, Papa, Papadopoulou, Bilan, Boström, Capdevila, Carreiro-Silva, Carugati, Cebrian, Coll, Dailianis, Danovaro, De Leo, Fiorentino, Gagnon, Gambi, Garrabou, Gerovasileiou, Hereu, Kipson, Kotta, Ledoux, Linares, Martin, Medrano, Montero-Serra, Morato, Pusceddu, Sevastou, Smith, Verdura and Guarnieri. This is an open-access article distributed under the terms of the Creative Commons Attribution License (CC BY). The use, distribution or reproduction in other forums is permitted, provided the original author(s) and the copyright owner(s) are credited and that the original publication in this journal is cited, in accordance with accepted academic practice. No use, distribution or reproduction is permitted which does not comply with these terms.
*Correspondence: Simonetta Fraschetti, simonetta.fraschetti@unina.it