- 1Complement Biology Laboratory, National Centre for Cell Science, Savitribai Phule (S. P.) Pune University Campus, Pune, India
- 2Dr. D.Y. Patil Biotechnology & Bioinformatics Institute, Dr. D.Y. Patil Vidyapeeth, Pune, India
- 3Institut Pasteur, Université Paris Cité, CNRS UMR2000, Unité Mycologie Moléculaire, Department of Mycology, Paris, France
- 4Laboratory of Autoimmunity and Tolerance, National Centre for Cell Science, Savitribai Phule (S. P.) Pune University Campus, Pune, India
- 5Department of Innate Immunity, ICMR – National Institute for Research in Reproductive and Child Health, Mumbai, India
Invasive aspergillosis (IA) is a life-threatening fungal infection for immunocompromised hosts. It is, therefore, necessary to understand the immune pathways that control this infection. Although the primary infection site is the lungs, aspergillosis can disseminate to other organs through unknown mechanisms. Herein we have examined the in vivo role of various complement pathways as well as the complement receptors C3aR and C5aR1 during experimental systemic infection by Aspergillus fumigatus, the main species responsible for IA. We show that C3 knockout (C3-/-) mice are highly susceptible to systemic infection of A. fumigatus. Intriguingly, C4-/- and factor B (FB)-/- mice showed susceptibility similar to the wild-type mice, suggesting that either the complement pathways display functional redundancy during infection (i.e., one pathway compensates for the loss of the other), or complement is activated non-canonically by A. fumigatus protease. Our in vitro study substantiates the presence of C3 and C5 cleaving proteases in A. fumigatus. Examination of the importance of the terminal complement pathway employing C5-/- and C5aR1-/- mice reveals that it plays a vital role in the conidial clearance. This, in part, is due to the increased conidial uptake by phagocytes. Together, our data suggest that the complement deficiency enhances the susceptibility to systemic infection by A. fumigatus.
Introduction
Aspergillus fumigatus is an opportunistic airborne fungal pathogen that causes invasive infection in immunocompromised individuals, such as those with hematological disorders, undergoing prolonged corticosteroid treatment (patients with organ transplantation), acquired-immunodeficiency syndrome, and autoimmune diseases (1). In immunocompetent hosts, inhaled A. fumigatus spores (conidia, the infective propagules) are cleared by the innate immune system. A significant body of knowledge is available on the clearance of conidia by the cellular immune system of the host (2–4). Still, the role of the humoral immune system, particularly the complement system, is poorly understood.
The complement system is a cascade of proteases that efficiently recognizes pathogens and kills them by lysis or tags them for removal by phagocytic cells. Besides, activation products of the complement system are capable of priming the acquired immune system by directly acting on T cell, B cell, and antigen-presenting cells (5–8). A. fumigatus is recognized by various complement activation pathway components such as C1q (9), MBL (10), ficolin-2 (11), and pentraxin-3 (PTX-3) (12). Therefore, it is not surprising that this pathogen, when incubated with serum in vitro, can activate all the three major (classical, alternative, and lectin) complement pathways (13–15). Initial studies on complement activation by various morphotypes of A. fumigatus showed that dormant conidia primarily activate the alternative pathway, while germinating morphotype activates the classical pathway (13, 14). A recent study showed that in normal serum, conidia initiate complement activation by the classical pathway, which then kicks off the alternative pathway amplification loop (16). However, when there is a lack of immunoglobulins (as in immunosuppressed hosts), the lectin pathway compensates for the classical pathway (17). Because A. fumigatus contains a thick cell wall, it is unlikely to be killed by the complement system by forming a membrane attack complex (MAC; C5b-9) in its cell membrane (13, 18). On the other hand, the complement system facilitates conidial clearance by promoting phagocytosis through conidial opsonization and/or by generating C5a, an anaphylatoxin (19). Indeed, the complement receptors CR3 and CR4 have been demonstrated to be important in the phagocytosis of opsonized A. fumigatus conidia (20).
To establish infection, A. fumigatus conidia employ a multitude of complement evasion mechanisms. For example, one of the cell wall components, melanin pigments, limits C3b deposition (21). Besides, conidia evade complement by recruiting host regulatory proteins and secreting proteases. The major soluble regulators of the complement system, such as factor H (FH), factor H-like protein (FHL-1), and C4b-binding protein (C4BP), have been shown to bind to the pathogen and regulate complement activation (22, 23). Two proteases associated with A. fumigatus, one released by its conidial morphotype (Mep1p) and another secreted by mycelia (Alp1p), have been shown to cleave major complement proteins C3, C4, and C5, and thereby inhibit phagocytosis (24, 25). Therefore, it is likely that these complement subversion strategies are enough to protect airborne A. fumigatus conidia from complement attack in the lung alveoli, where complement components are present in relatively lower amounts compared to blood. However, what role complement plays when the infection is disseminated and whether A. fumigatus can survive complement assault during systemic disease remain unclear. An earlier study demonstrated that C5-deficient mice (DBA/2N) display decreased resistance to systemic A. fumigatus infection compared to C5-sufficient outbred mice (CFW) (26). However, the genetic backgrounds of C5-deficient and -sufficient mice were different, posing difficulty in the data interpretation.
In the present study, we thus asked: (i) whether the complement system plays any protective role during systemic A. fumigatus infection, (ii) if yes, which complement pathways and/or components participate in this process, and (iii) what contribution, if any, do the anaphylatoxins C3a and C5a make in the host defense against systemic A. fumigatus infection? To decipher these questions, we employed a previously reported intravenous infection model to study disseminated aspergillosis (26, 27). Intravenous infection with A. fumigatus conidia in C3-/- or C5-/- mice resulted in significantly higher mortalities compared to that in wild-type mice, suggesting an essential role of the complement system against experimental systemic aspergillosis. In vitro study indicated that the conidia harbor C3 and C5 cleaving proteases. To dissect the role of anaphylatoxins during systemic aspergillosis, we performed studies using C3a receptor (C3aR-/-) and C5a receptor-1 knockout (C5aR1-/-) mice. The data suggested that C5aR1, but not C3aR signaling is required to mount a protective immune response against systemic A. fumigatus infection, which is partly due to enhanced uptake of conidia by phagocytes.
Materials and methods
A. fumigatus strain and growth conditions
A clinical isolate of A. fumigatus (28) was maintained on 2% malt-agar slant at ambient temperature. Conidia from 10-12 days old agar slants were harvested using 0.05% aqueous Tween-80 and passed through a filter (0.45 μm) to remove any hyphal fragments present, centrifuged (10,000 rpm, 10 min), resuspended in sterile water, freeze-dried, and stored at 4°C until use.
Mice
The wild-type (BALB/c and C57BL/6) as well as the complement knockout mice (C3-/- and C4-/- on C57BL/6 background, C3aR-/- and C5aR-/- on BALB/c background, and B10.D2/oSnJ background for both C5-sufficient and C5-deficient) were purchased from the Jackson Laboratory, USA. C3-/- mice on BALB/c background and factor B-/- mice on C57BL/6 background were kind gifts from Prof. Marina Botto, Department of Medicine, Imperial College, London. All mice were bred and housed in a barrier-maintained and controlled animal facility of the National Centre for Cell Science (NCCS), Pune. All the animal experiments were approved by the Institutional animal ethics committee (IAEC) of NCCS, Pune (EAF/2016/B-207).
A. fumigatus infection in mice
Wild-type (BALB/c and C57BL/6) and complement deficient mice (C3-/-, FB-/-, C4-/-, C5-sufficient, C5-deficient, C3aR-/- and C5aR-/-) were used for the infection study. The mice were grouped (4-6 mice per cage) and marked on ear pinna by forming cuts. For infection, conidia were suspended in sterile PBS with 0.05% Tween 80 (PBS-T). A graded dose of conidial suspension (1x105, 1x106or 1x107) in a volume of 100 µl was injected intravenously through the lateral tail vein. These mice were observed daily for survival and signs of illness such as head-tilt and swirling. The body weight of mice was monitored from the day of infection. Mice with body weight loss of ≥30% were sacrificed humanely and considered for mortality calculation.
Complement proteins (C3 and C5), antibodies, sera, and plasma
Human C3 and C5 were obtained from Merck Millipore. Anti-mouse Ly-6G PE (Cat. No.:127614; clone:1A8), anti-mouse Ly-6C APC (Cat. No.:128008; clone:HK1.4), rat anti-mouse CD11b PE-Cy7 (Cat. No.:552850; clone.:M1/70) were purchased from BD Biosciences. Anti-mouse IgM (Cat. No.: 12-5890-82) was purchased from eBioscience. Blood was collected from infected mice by retro-orbital bleeding. For obtaining plasma, blood was collected in a microcentrifuge tube containing EDTA (final concentration, 20 mM) and centrifuged at 3000 rpm for 5 min at 4°C. For serum separation, blood collected in a microcentrifuge tube was kept at 37°C for 30 min and centrifuged at 3000 rpm for 5 min at 4°C. Plasma and sera were stored at -80°C until use.
In vivo interaction of A. fumigatus conidia with neutrophils and monocytes
For measuring in vivo interaction of A. fumigatus conidia with neutrophils, eosinophils and monocyte, conidia were labeled with carboxyfluorescein diacetate succinimidyl ester (CFSE). In brief, dormant conidial suspension (1x107 conidia/ml; 1 ml) in PBS-T was mixed with CFSE (2.5 μM) and incubated at 37°C in a water bath in the dark for 25 min, with vortexing every 5 min. To remove unbound CFSE, 300 µl of PBS-T was added to the suspension and centrifuged at 10,000 rpm. Conidia were then washed and suspended in PBS-T. CFSE-labeled conidia were analyzed with a FACS Calibur flow cytometer (BD Biosciences). The unstained conidia were used as a negative control for FACS analysis.
To examine the interaction of conidia with neutrophils/monocytes, CFSE-labeled conidia (1x106;100 µl) were injected intravenously into WT and C3-/- mice via the lateral tail vein. Next, about 0.5 ml of blood was collected in a sterile tube from each mouse by orbital bleeding at 5 min and 15 min post-infection and kept on ice. Blood was collected in 20 mM EDTA to avoid complement activation. To separate cells, blood was centrifuged at 3000 rpm, and RBCs were lysed by adding 4 ml of chilled lysis buffer (155 mM NH4Cl, 10 mM KHCO3, and 0.1 mM EDTA, pH 7.4) followed by incubation on ice for 4 min. The cell suspension was then mixed with 2 ml of chilled sterile PBS and centrifuged at 1800 rpm for 10 min at 4°C. The cells were washed twice with chilled sterile PBS to remove traces of lysis buffer. Hereafter, cells were suspended in 50 µl of sterile PBS and stained with fluorescently conjugated anti-CD11b, anti-Ly6G, and anti-Ly6C antibodies (0.5 µl of each antibody). The conidial interaction with the specific cell types was studied by flow cytometry, with a gating strategy: mature monocytes: Ly6G- Ly6C-, immature monocytes: Ly6G- Ly6C+, neutrophils: Ly6G+ Ly6C mid, and eosinophils: Ly6G+ Ly6C- (Supplemental Figure 1). Data were analyzed by FlowJo software (TreeStar Inc.), and the total number of cells interacting with the conidia was plotted as a mean ± SD (three independent experiments).
Complement C3 and C5 cleavage activity of A. fumigatus
To assess the complement C3 or C5 cleaving activity, 1x107 conidia were incubated in a 50 µl of Tris buffer with human C3 or C5 (3 µg) for 30 min at 37°C. The reaction mixture was then centrifuged at 10,000 rpm for 5 min, and the supernatant was loaded on SDS-PAGE. The gel was stained with Coomassie blue to visualize the cleaved fragments. The protease activity was blocked by EDTA (10 mM), Chymostatin (100 µg/ml), or heat inactivation of conidia (95°C, 5 min). Conidial C3 cleavage capacity was also analyzed by Western blot at two different time-points: after 15 min and 30 min co-incubation of C3 with conidia. The C5a released upon C5 cleavage activity of conidia was measured by ELISA using the C5a DuoSet detection kit (R&D Systems).
ELISA for mouse elastase, IL-6, and TNF-α and C5a measurements
Elastase, IL-6, and TNF-α were quantified using ELISA kits (R&D Systems). For C5a measurement, a 96-well plate (Greiner Bio-One) was coated with rat anti-mouse C5a antibodies (100 µl; 10 μg/ml in PBS; BD Biosciences Cat. No.: 558027) overnight at 4°C. The coated wells were blocked with 1% fetal bovine serum and 2% skimmed milk in PBS for 2 h at room temperature. After a wash with PBS-0.05% Tween-20, mice plasma (1:10 dilution) in blocking buffer was added to the wells; for the standard curve, different concentrations of C5a standard (BD Biosciences, Cat. No.: 51-9004473) was used. The plate was incubated for 2 h at room temperature and washed thrice with PBS-0.05% Tween-20. Bound C5a was measured by sequential incubations with biotinylated anti-mouse C5a antibody (1 µg/ml; BD Biosciences, Cat. No.: 558028), anti-avidin-HRP antibody (1:1000 diluted; Bio-Rad Cat. No. 170-6528) both suspended in blocking buffer, for 1 h, with PBS-T washes (thrice) between each step, followed by adding substrate (o-phenylenediamine dissolved in 0.1 M citrate buffer (pH 4.2) containing 1µl/ml of 30% H2O2;100 µl/well). After 20 min, the reaction was stopped by adding 3N H2SO4 (100 µl/well), and the color was read at 490 nm using a plate reader.
Passive transfer of naïve sera
Naïve sera were obtained from WT (BALB/c) and C3-/- (BALB/c) mice and incubated at 56°C for 30 min to inactivate complement. To determine the protective ability of the naïve mice sera from WT and C3-/- mice against A. fumigatus infection, C3-/- (BALB/c) mice were administered intraperitoneally with 250 µl of pooled serum on day -1 and day 4 post-infection. These mice were then challenged intravenously on day 0 with conidia (1x105) suspended in PBS-T and examined for weight loss and mortality.
Histopathology
For histopathology analysis, visceral organs (kidney, lung, spleen and liver) were harvested and immediately fixed in 10% neutral-buffered formalin (pH 7.4). The tissues were then processed by alcohol-xylene protocol, embedded in paraffin, and 5 μm size sections were cut using a microtome (Leica, Germany). The cut sections were stained with hematoxylin and eosin (H & E), and analyzed by observing under a light microscope.
Periodic acid-Schiff staining
The sections used for histopathology analysis were also analyzed for PAS staining. For deparaffinization, the slide was flamed on the burner and placed in the xylene till all the wax was removed. Xylene was drained and the tissue section was hydrated by passing through decreasing concentrations of alcohol baths (100%, 90%, 80%, 70%) and water. The section was placed in a periodic acid solution (1%) for 5-10 minutes and thereafter the slide was washed with at least two changes of distilled water. Next, the slide was covered with Schiff’s reagent for 20-30 minutes followed by a rinse in running tap water for 10 minutes. Following this, the slide was covered with hematoxylin for 5 minutes and the section was dehydrated in increasing concentration of alcohols and placed in two xylene baths for clearing. Slides were mounted in DPX and analyzed under a light microscope.
Statistical analysis
For in vivo studies, the percent survival of mice in each group was plotted as Kaplan-Meier plots and analyzed using the Log-Rank test (GraphPad Prism, GraphPad, San Diego, CA). Statistical analyses for in vitro assays were performed by Mann Whitney test (SigmaStat, Systat Software, Inc., San Jose, CA) for comparison of two groups and by one-way ANOVA (Tukey’s multiple comparison test, GraphPad prism) for comparison of multiple groups.
Results
Complement C3-/- mice are highly susceptible to systemic A. fumigatus infection
C3 is the central component of the complement system. Its activation leads to the formation of C3b, which has been demonstrated to be essential for the A. fumigatus conidial opsonization and clearance by phagocytes (29). To explore the role of C3 during systemic A. fumigatus infection, we performed infection studies in C3-/- mice (C57BL/6 background) and compared it with the wild-type (WT) mice. The mice were intravenously injected with high (1x107), moderate (1x106), and low (1x105) doses of conidia (Figure 1A). At a high (1x107) dose, both WT and C3-/- mice failed to survive; however, in the C3-/- mice group, 100% death occurred on the 5th day post-infection (dpi) compared to the 7th dpi in WT mice group. At a moderate (1x106) dose, the C3-/- mice showed 100% mortality, and WT mice showed only 30% mortality. Whereas at a low conidial dose (1x105), C3-/- mice group exhibited 30% mortality and WT mice showed 100% survival. Histopathological sections of different organs of conidia-challenged wild-type/C3-knockout mice were examined for fungal load (PAS staining) and infiltration of immune cells (H&E staining). The data indicated that in the C3-knockout mice, there is an increased fungal burden and inflammation, compared to the wild-type mice (Supplemental Figures 2-4), suggesting that the C3 deficiency contributes to both increased survival of the fungus and dysregulation of inflammation. Together, these results suggested that the C3-/- mice are more susceptible to systemic A. fumigatus infection than the WT mice.
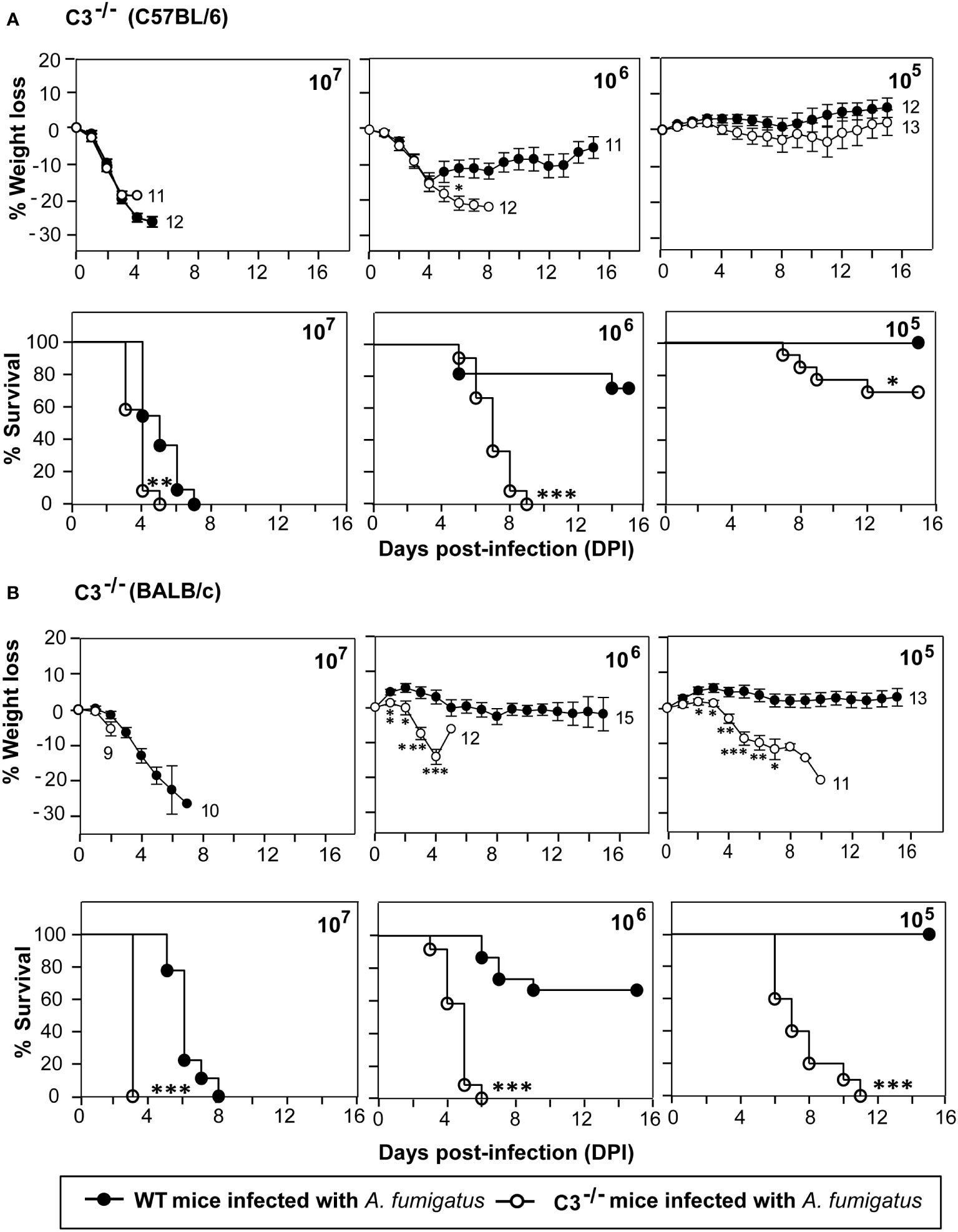
Figure 1 C3 deficiency enhances the susceptibility to systemic A fumigatus infection. Mice were intravenously infected with 1x105, 1x106, or 1x107 conidia suspended in PBS-T. The percent body weight change and survival of mice after the infection was recorded daily for 15 days. (A) Percentage of body weight loss and survival in complement C3 deficient mice (C3-/-; C57BL/6 background) in comparison to the wild-type mice (WT; C57BL/6 background). The body weights were normalized to their initial body weights. The numbers at the end of the line depict the number of mice used in that group. The survival in C3-/- mice group was significantly lower in comparison with WT mice group at 1x105 and 1x106 dose of conidial infection (% weight loss: *p < 0.05; % survival: ***p < 0.0001, **p < 0.001, *p < 0.01). (B) Percentage of body weight loss and survival in complement C3 deficient mice (C3-/-; BALB/c background) in comparison to the wild-type mice (WT; BALB/c background). Similar to C57BL/6 C3-/- mice, the BALB/c C3-/- mice also showed reduced survival at 1 x 105 and 1 x 106 dose of conidial infection in comparison to the WT mice (% weight loss: *p < 0.05, **p < 0.005, ***p < 0.0005; % survival: ***p < 0.0001). Results shown are mean ± SEM of three independent experiments.
C57BL/6 mice preferentially develop a Th1 immune response, while BALB/c mice develop a Th2 immune response (27, 30). We thus sought to determine whether C3 deficiency in different genetic backgrounds affects the susceptibility to systemic infection by A. fumigatus. Therefore, as above, WT and C3-/- mice, both on BALB/c background, were infected intravenously with various doses of A. fumigatus conidia. C3-/- mice on BALB/c background were more susceptible to A. fumigatus systemic infection than that on a C57BL/6 background. Nevertheless, the survival patterns were essentially similar to those observed for C57BL/6 mice (Figure 1B). At the high dose of conidia (1x107), all C3-/-mice succumbed to infection, but the mortality in WT mice was significantly delayed compared to C3-/- mice. At the moderate dose (1x106), all C3-/- mice succumbed to infection compared to only 30% mortality in the WT mice group. Infection with a low conidial dose (1x105) led to no mortality in the WT mice but 100% mortality in the C3-/- mice group. Overall, these data demonstrate that complement component C3 is essentially required for the clearance of conidia from the systemic circulation regardless of the murine genetic background.
Role of C4 and factor B in protection against systemic A. fumigatus infection
Next, we examined the role of individual complement activation pathways during infection. Earlier in vitro studies have shown that A. fumigatus conidia and hyphae can activate all the three pathways of the complement system (13, 16, 17, 31), but which pathway is more critical during in vivo infection, is not known. We, therefore, intravenously infected factor B knockout (FB-/-) and complement C4 knockout (C4-/-) mice with conidia. The complement FB is a component of the alternative pathway; therefore, FB-/- mice are deficient in the alternative pathway. C4, on the other hand, is a component of the classical and lectin pathways; hence, C4-/- mice are deficient in these two pathways. Infection of FB-/-mice with conidia had no significant difference in survival and weight loss compared to WT mice (Figure 2A). C4-/- mice also failed to show any difference in survival compared to WT mice (Figure 2B). This could be because of the redundancy, i.e., the lack of one pathway is compensated by the presence of the other, and therefore, both FB and C4 could be critical for the protection against A. fumigatus infection. It is also likely that during interaction with A. fumigatus, complement activation occurs primarily via a non-canonical pathway that does not require FB or C4.
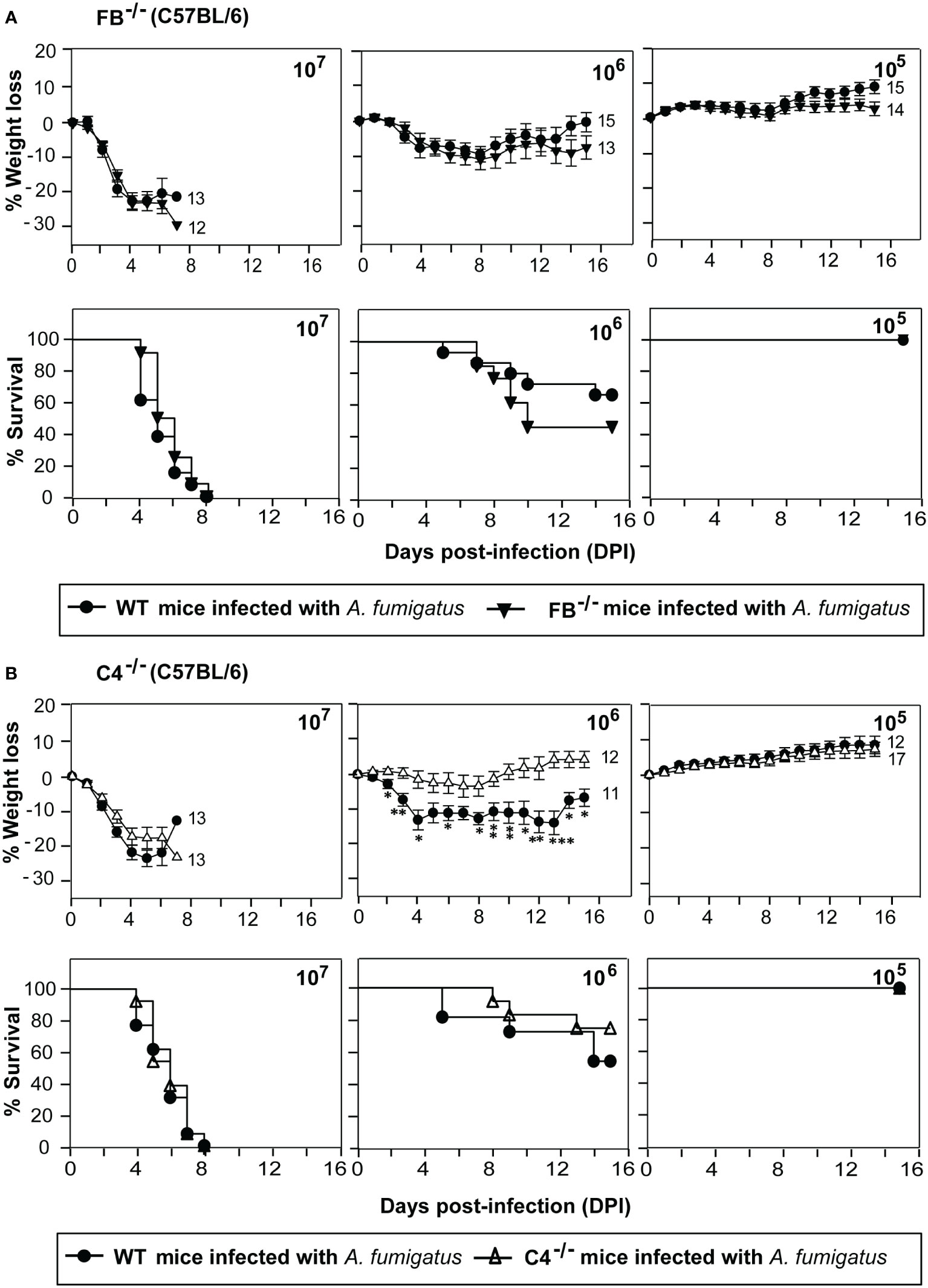
Figure 2 Susceptibility of factor B and C4 deficient mice to systemic A fumigatus infection. Mice were intravenously infected with indicated amounts of conidia (1x105, 1x106, or 1x107) and observed for body weight loss and survival. (A) Percentage of body weight loss and survival in complement factor B deficient mice (FB-/-; C57BL/6 background) in comparison to the wild-type mice (WT; C57BL/6 background). There is no difference in the weight loss and survival of FB-/-mice compared to the WT mice. (B) Percentage of body weight loss and survival in complement C4 deficient mice (C4-/-; C57BL/6 background) in comparison to the wild-type mice (WT; C57BL/6 background). C4-/- mice did not show more weight loss and reduced survival compared to the WT mice. (% weight loss: ***p < 0.0005; **p < 0.005 *p < 0.05). Results shown are mean ± SEM of three independent experiments.
As A. fumigatus is known to encode many secreted proteases (32), we looked if conidia contain any surface protease capable of cleaving complement C3. Incubation of metabolically active conidia with human C3 resulted in the cleavage of C3 into a C3b-like fragment. Western blot indicated the C3b-like fragment formation and also captured further degradation products. Importantly, this cleavage could be blocked by chymostatin and heat-inactivation of conidia but not by EDTA, a metal chelator (Figure 3). The results suggested that conidia have protease(s) capable of activating complement. Further, it is not a metalloprotease, but likely a chymotrypsin-like serine/cysteine protease.
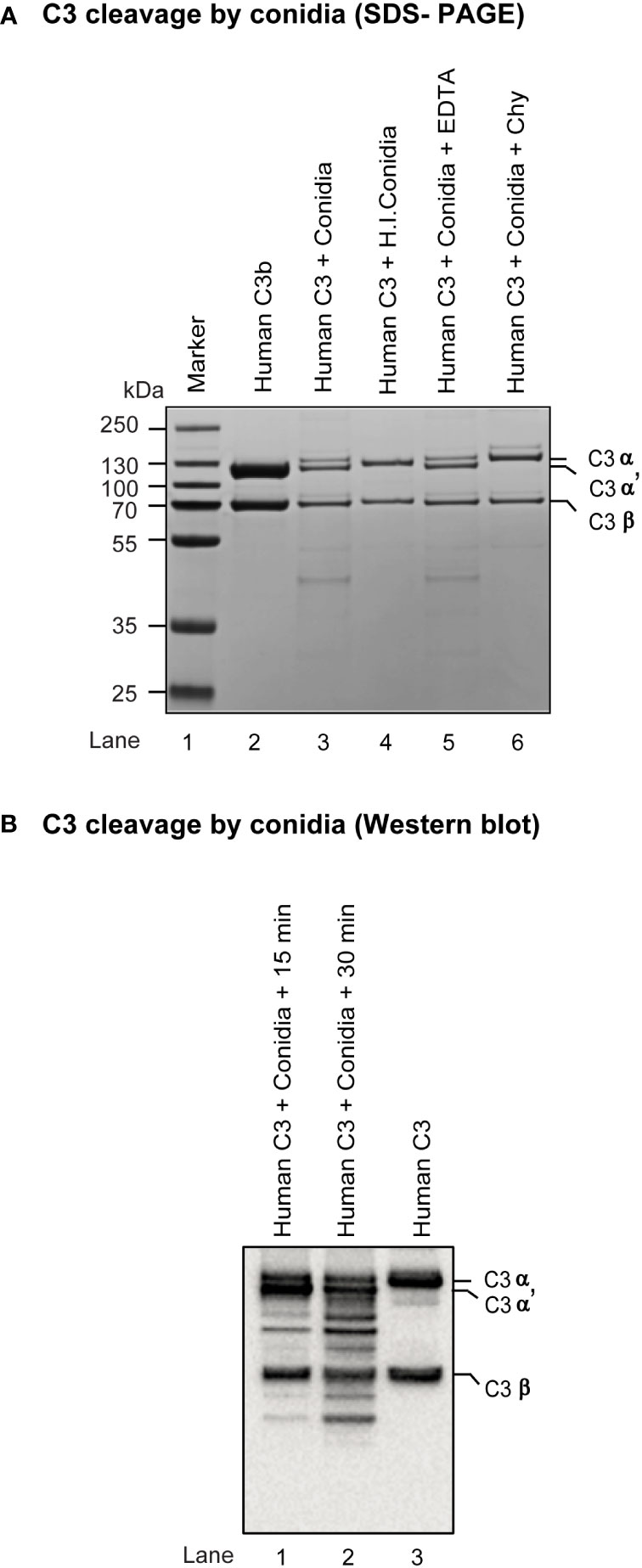
Figure 3 Cleavage of complement protein C3 by A fumigatus conidia. (A) Human C3 (3 µg) was incubated for 30 min at 37°C with 1x107 conidia in a total reaction volume of 50 µl in Tris-buffer pH 7.4. Following incubation, the reaction mix was centrifuged at 10,000 rpm for 5 min. Supernatants were loaded on a 7.5% SDS-PAGE, and the cleaved fragments of C3 were visualized by staining the gel with Coomassie blue. Heat inactivated conidia: heat-inactivated by incubating it at 95°C for 5 min. Cleavage of C3 by A fumigatus conidia was performed in the presence of inhibitors: EDTA, (10 mM); Chy, Chymostatin (100 µg/ml). Conidia effectively cleaved C3, which could be inhibited by Chymostatin, but not by EDTA. The PAGE profile shown is a representative of three independent experiments. (B) C3 cleavage capacity of conidia was also examined by Western blot by incubating human C3 (3 µg) with 1x107 conidia for 15-30 min at 37°C, collecting and subjecting the supernatant of the reaction mixture to SDS-PAGE (gradient gel, 4-12%), blotting separated protein bands on to nitrocellulose membrane, probing the membrane with anti-C3 antibody (Merci Millipore) and revealing the bands using ECL detection kit (Pierce); the experiment was repeated twice.
Complement C5-deficient mice are more susceptible to systemic A. fumigatus infection
Activation of terminal complement component C5 results in the generation of C5a and C5b. C5a is a potent immunomodulatory molecule that mediates the recruitment and activation of neutrophils and monocytes, which play a central role in the clearance of A. fumigatus conidia. C5b, on the other hand, initiates the formation of the terminal complement pathway and forms C5b-9 (MAC) on the pathogen leading to lysis, which is less likely to occur for A. fumigatus owing to its thick cell wall. Thus, if the terminal pathway is important, C5 deficiency is expected to exacerbate the disease. Therefore, the systemic infection was performed in C5-deficient and sufficient mice (Figure 4A). At a moderate dose (1x106) of infection with conidia, the mortality in C5-deficient mice was 66%, whereas there was no mortality in C5-sufficient mice. With a high dose of conidia (1x107), there was a significant delay in the death of C5-sufficient mice compared to C5-deficient mice. This result suggested that the complement C5 also plays an essential role against systemic A. fumigatus infection.
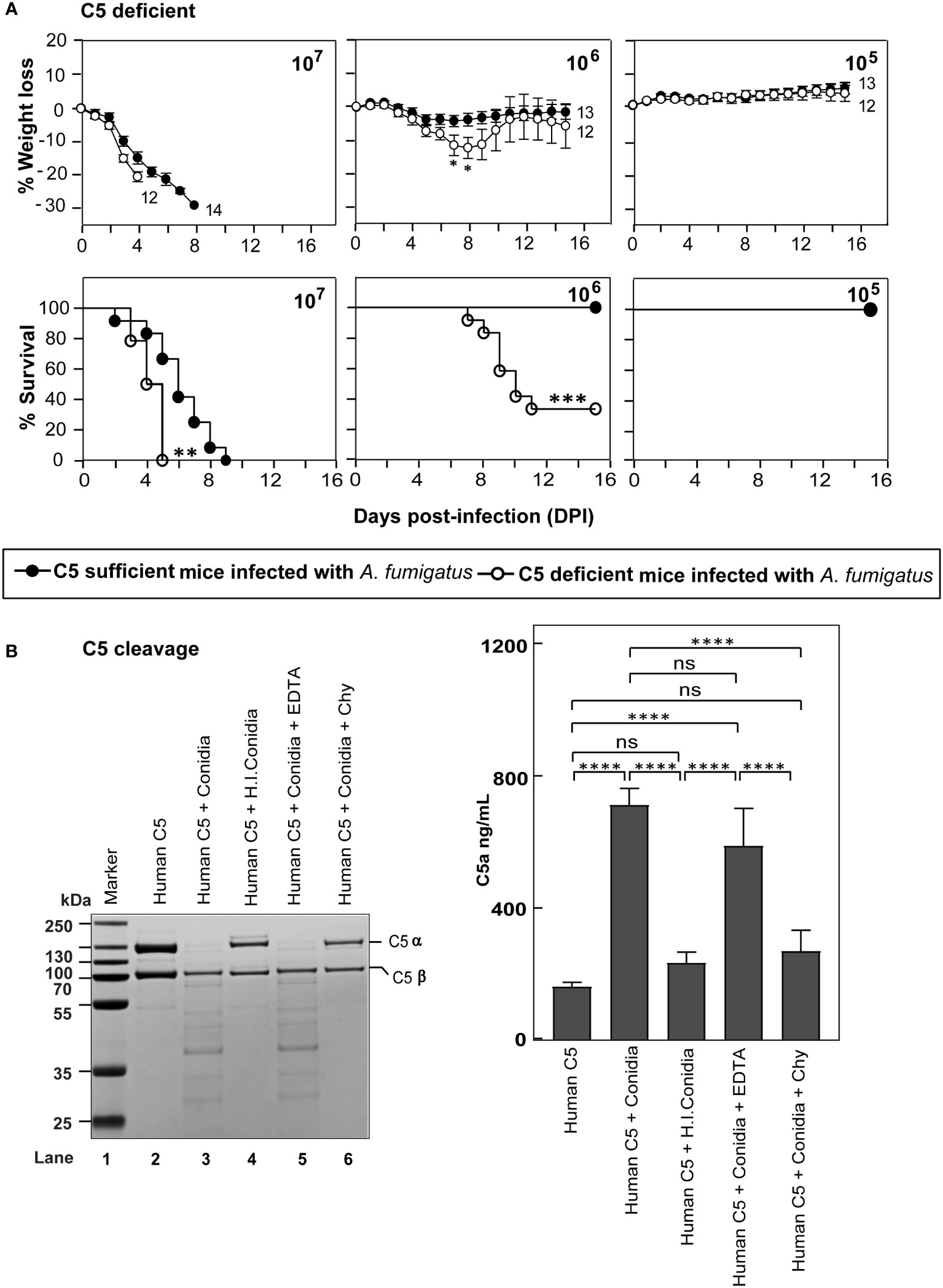
Figure 4 C5 deficiency enhances the susceptibility to systemic A fumigatus infection. (A) C5 sufficient (B10.D2/nSn) and C5 deficient (B10.D2/oSn) mice were infected intravenously with 1x105, 1x106 or 1x107 conidia. The percent body weight loss and survival were monitored for 15 days. C5 deficient mice exhibited more susceptibility to A fumigatus infection than WT mice (***p < 0.0001; **p < 0.001 for survival and *p < 0.05 for weight loss). Results shown are mean ± SEM of three independent experiments. (B) Human C5 (3 µg) was incubated for 30 min at 37°C with 1x107 conidia in a total reaction volume of 50 µl in Tris-buffer pH 7.4. Following incubation, the reaction mix was centrifuged at 10,000 rpm for 5 min. Left panel: Supernatants were loaded on a 12% SDS-PAGE, and the cleaved fragments of C5 were visualized by Coomassie blue staining. Right panel: Supernatants were tested for the presence of C5a by ELISA. Heat inactivated conidia: heat-inactivated by incubating it at 95°C for 5 min. Cleavage of C5 by A fumigatus conidia was performed in the presence of inhibitors: EDTA, (10 mM); Chy, Chymostatin (100 µg/ml). Conidia effectively cleaved C5, which could be inhibited by Chymostatin, but not by EDTA (ns – not significant, *p < 0.05, **p < 0.005, ***p < 0.0005). The statistical significance was evaluated by One way ANOVA, followed by Tukey’s multiple comparison test (****p < 0.01). ns, not significant.
The susceptibility of C5-deficient mice to A. fumigatus infection led us to question whether conidia also have C5 degrading activity. To check this, we incubated human C5 with conidia (Figure 4B). Interestingly, upon incubating C5 with conidia, there was a degradation of the α-chain of C5; this C5 degrading activity was lost when conidia were subjected to prior heat-inactivation or in the presence of chymostatin, but not EDTA, suggesting that the conidial C5 degrading activity is also associated with a chymotrypsin-like serine/cysteine protease. Moreover, when we measured C5a released upon C5 incubation with conidia, there was a significant amount of C5a in the reaction mixture, which was found to decrease upon heat-inactivation of conidia or by the addition of chymostatin into the reaction mixture, but not upon EDTA addition (Figure 4B).
C5a-C5aR axis plays a protective role during systemic A. fumigatus infection
Activation of the complement system should result in the generation of two potent anaphylatoxins – C3a and C5a. These peptides modulate the functioning of various immune cells by binding to their receptors C3aR and C5aR1, respectively. Earlier reports have shown that the requirement of immune signaling via the C3a-C3aR and C5a-C5aR1 axes varies depending on the pathogen (33–36). To dissect the role of signaling through C3aR or C5aR1 axis in protection against systemic A. fumigatus infection, we infected C3aR-/- and C5aR1-/- mice with various doses of conidia (Figure 5A). At a moderate conidial dose (1x106), the mortality in C5aR1-/-, but not C3aR-/- mice, was significantly higher (~45%) than in the WT mice. At a low dose (1x105) of infection, C5aR1-/- mice showed comparatively more mortality than the WT mice, but the difference was not significant. It, therefore, can be concluded that the C5a-C5aR1 axis participates in immune protection against A. fumigatus infection. However, there is a caveat in this interpretation; for the ligand-receptor axis to work, the ligand must be available, and therefore, it is essential to assess the generation of C5a during the infection. Analysis of the serum of WT, C3-/- and C5aR1-/- mice infected with conidia showed that, although not at statistically different levels in different groups, the anaphylatoxin C5a was indeed generated during infection (Figure 5B).
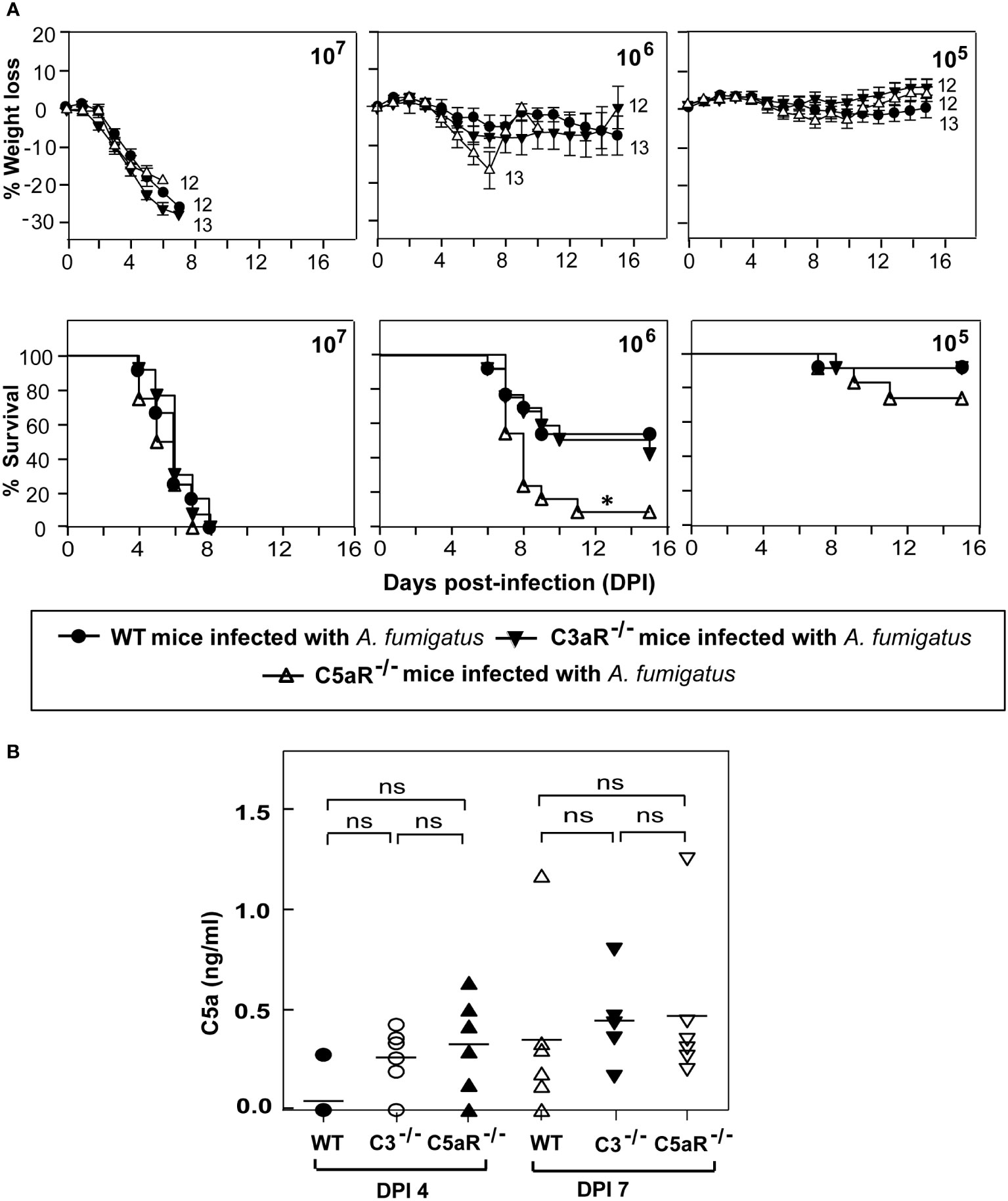
Figure 5 C5a receptor signaling is crucial for protecting against systemic A fumigatus infection. (A) Wild-type (WT), C3aR-/- and C5aR-/- mice (all on BALB/c background) were infected intravenously with 1x105, 1x106, or 1x107 conidia and observed for percent body weight loss and survival till day 15 post-infection. The numbers depicted at the end of each line in the weight loss graph indicate the number of animals used in that group. The C5aR-/- mice showed significantly higher mortality than the WT mice (*p < 0.01). Results represent the mean ± SEM of three independent experiments. (B) BALB/c WT, C3-/- and C5aR-/- mice were infected intravenously with 1x105 conidia. Plasma samples from A fumigatus infected mice were obtained on day four and day seven post-infection. C5a levels were determined by ELISA. Results shown are mean concentrations ± SD obtained from six individual mice samples. The statistical significance was evaluated by One way ANOVA, followed by Tukey’s multiple comparison test. ns, not significant.
Passive administration of naïve sera from WT mice to C3-/- mice extends their survival following systemic A. fumigatus infection
Multiple reports show a convincing role of a natural reservoir of antibodies in protective immunity to bacteria, viruses, and A. fumigatus infection (37–39). Further, deficiency of complement receptor 2 (CR2) that binds to a C3 degradation product C3d has been linked to a reduced pool of peritoneal B1a cells, the primary source of natural antibodies (40). We thus tested the possibility of whether the lack of C3 results in decreased levels of natural antibodies, which in part is responsible for the higher susceptibility of C3-/- mice to A. fumigatus infection. Here, we injected (i.p.) heat-inactivated naïve sera (to avoid any role of the complement system) from WT or C3-/- mice to C3-/- mice a day before the infection and at day 4 post-infection. These mice were then challenged with conidia and observed for body weight loss and mortality. The infected mice that received naïve sera from WT mice showed a significantly delayed mortality compared to mice that received the sera from C3-/- mice (Figure 6). We, therefore, suggest that one of the ways by which complement C3 contributes to innate immunity against A. fumigatus is by enhancing the natural antibody pool.
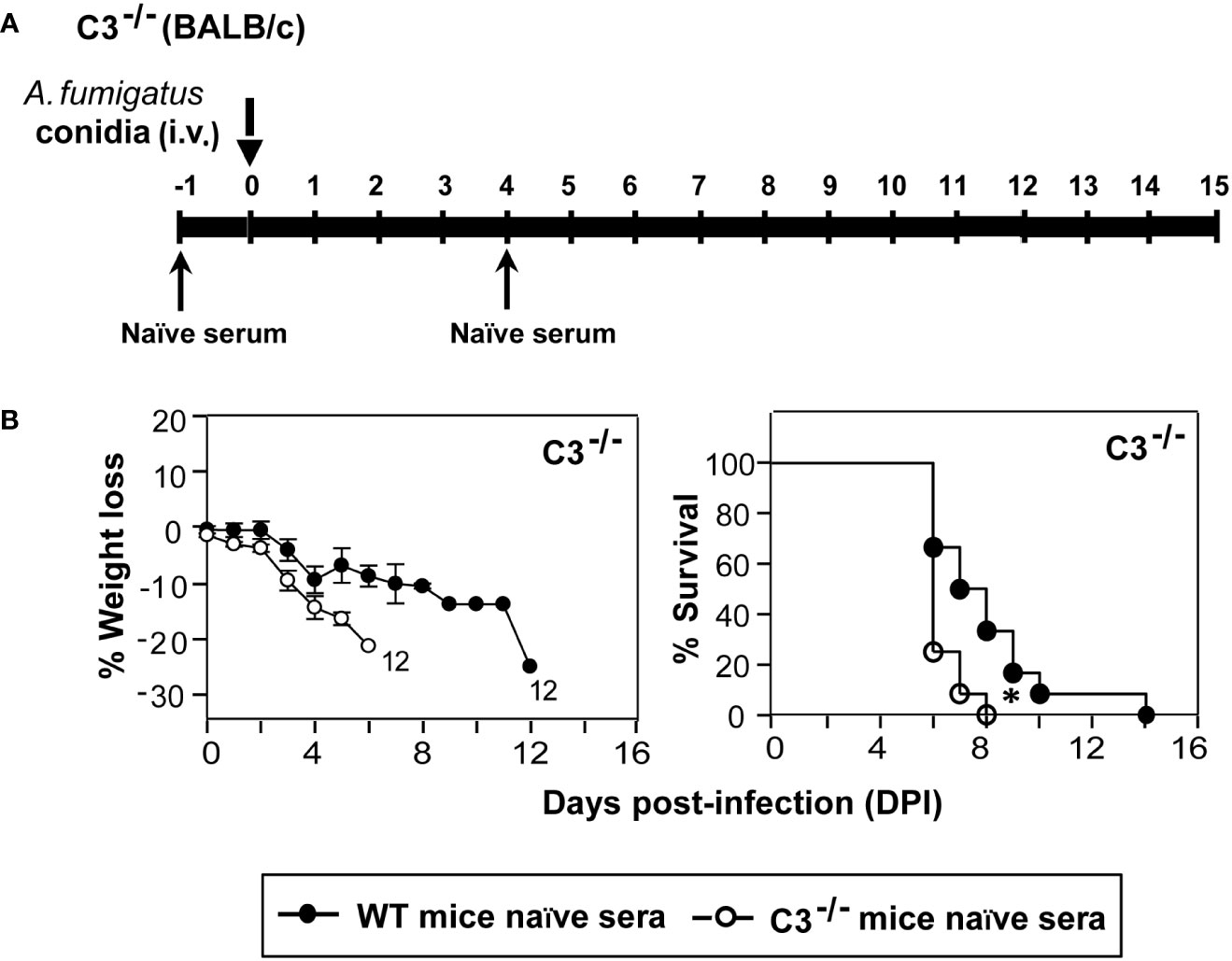
Figure 6 Natural antibodies aid in protection against systemic A fumigatus infection. (A) Diagrammatic depiction of the experimental setup. C3-/- mice received 250 µl serum (i.p.) of naïve heat-inactivated sera from WT (BALB/c) or C3 deficient (C3-/-; BALB/c) at day -1 and day 4. These mice were challenged intravenously with 1x105 conidia on day 0 and observed for body weight loss and mortality till the end of the experiment. (B) Body weight loss and survival of the infected complement C3-/- mice that received heat-inactivated naïve sera either from WT or from C3-/- mice. Passive transfer of naïve sera from WT mice extended the survival of infected C3-/- mice (*p < 0.01). Results shown are mean ± SEM of two independent experiments.
The contribution of the complement system in sequestering conidia during systemic A. fumigatus infection
Another plausible reason for the higher susceptibility of C3-/- mice to systemic A. fumigatus infection could be the lack of conidial opsonization by C3b, affecting their uptake by neutrophils, monocytes, and eosinophils via complement receptors, and thereby reducing conidial clearance. To test this possibility, WT and C3-/- mice were intravenously infected with CFSE-labeled conidia, and blood lymphocytes were analyzed for the uptake of labeled conidia. As speculated, immediately after infection (5 min post-infection), neutrophils (Ly6G+Ly6CInt), eosinophils (Ly6G+Ly6Clow), and monocytes (Ly6G-Ly6Clow and Ly6G-Ly6Chi) from WT mice engulfed a significantly higher number of conidia compared to that from C3-/- mice (Figure 7). The uptake of conidia reduced with time. There was no difference in the uptake between WT and C3-/- mice following 15 min post-infection (Figure 7), possibly due to other modes of phagocytosis. Nevertheless, these data imply that C3 opsonization affects conidial uptake by monocytes, neutrophils, and eosinophils in vivo, and this in part is likely responsible for reduced immune clearance of conidia in C3-/- mice.
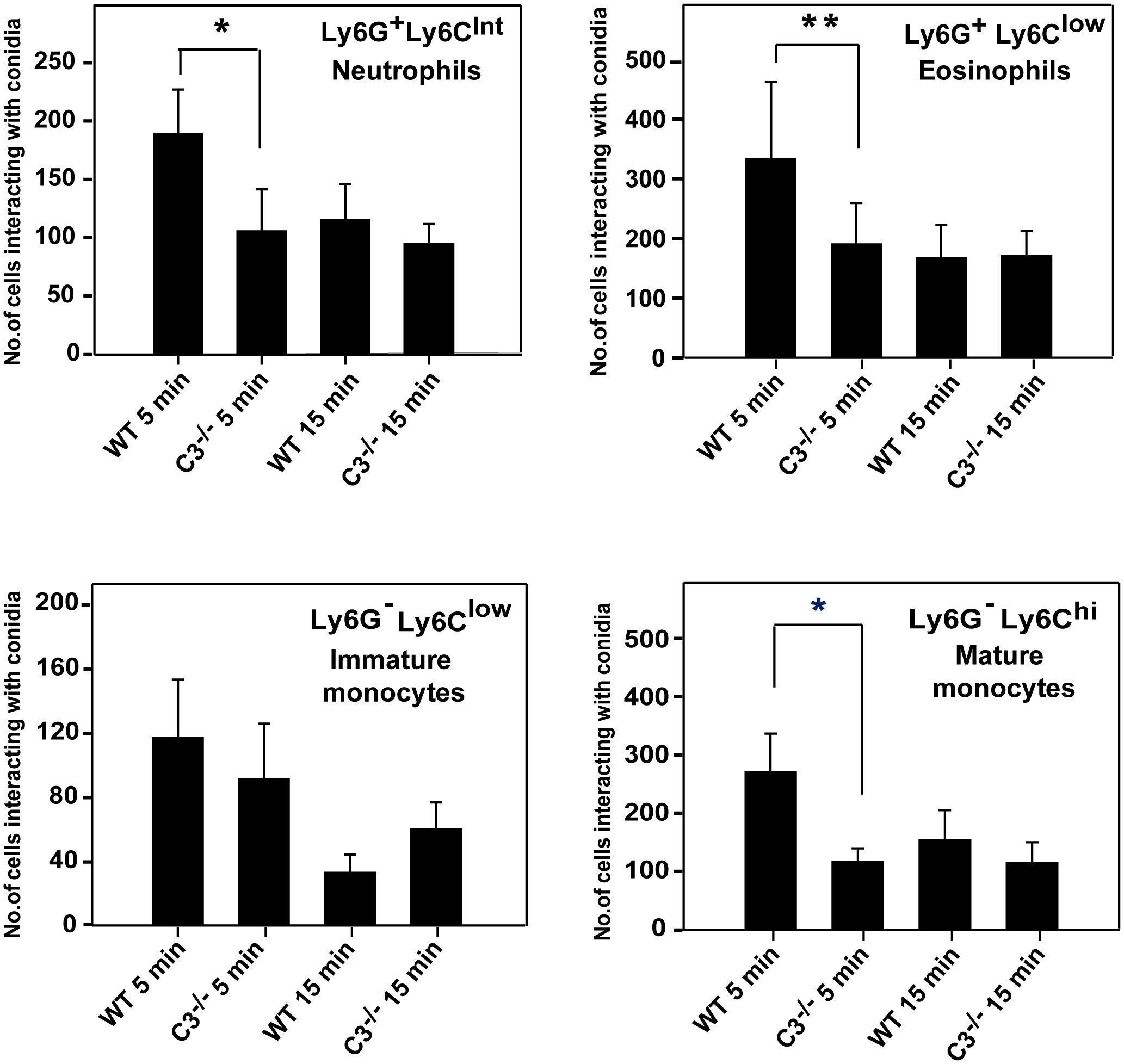
Figure 7 In vivo uptake of A. fumigatus conidia by monocyte subsets, eosinophils, and neutrophils. A. fumigatus conidia 1x106 labelled with CFSE were injected intravenously in wild-type (WT) and C3-/- mice (both on BALB/c background), and blood was collected from infected mice from the retro-orbital sinus at 5 min and 15 min post-infection. Following RBC lysis, cells were stained for neutrophils (Ly6G+/Ly6CInt), eosinophils (Ly6G+/Ly6Clow), and monocyte subsets (Ly6G-/Ly6Clow and Ly6G-/Ly6Chi) with fluorescent antibody and uptake/interaction of conidia was examined by FACS. Results represent the mean ± SD of three independent experiments. Immune cells from WT mice showed more interaction with conidia in 5 min than the cells from C3-/- mice. The statistical significance in complement deficient mice was evaluated by Mann Whitney test (**p < 0.01 *p < 0.05).
Expression of other effectors during systemic A. fumigatus infection in C3-/-and C5aR1-/- mice
It is clear from our data that complement deficiency results in heightened susceptibility to A. fumigatus infection. We thus asked whether increased susceptibility of C3-/- and C5aR1-/- mice to A. fumigatus infection is also due to reduced generation of other effectors in these mice known to control A. fumigatus infection. For example, deficiency in elastase released by neutrophils has been illustrated to delay the clearance of A. fumigatus (41). Nonetheless, estimation and comparison of neutrophil elastase levels between WT, C3-/- and C5aR1-/-mice (all on BALB/c background) infected with A. fumigatus conidia showed that all the three groups of mice produce similar levels of elastase (Figure 8A). TNF-α and IL-6 are the cytokines involved in the defense against A. fumigatus, and both TNF-α-/- and IL-6-/- mice display increased susceptibility to Aspergillus infection (42–44). However, the quantitation of TNF-α levels in WT and C3-/-or C5aR1-/- mice in our study illustrated that its level increased after infection (day 4) and remained high even on day 7 (Figure 8B). The IL-6 levels also increased with the time of infection but did not vary between WT and complement knockout (C3-/- and C5aR1-/-) mice groups (Figure 8C). We, therefore, conclude that the increased mortality in the C3-/- and C5aR1-/- mice are not due to generalized failure in generating some of the salient effectors against A. fumigatus.
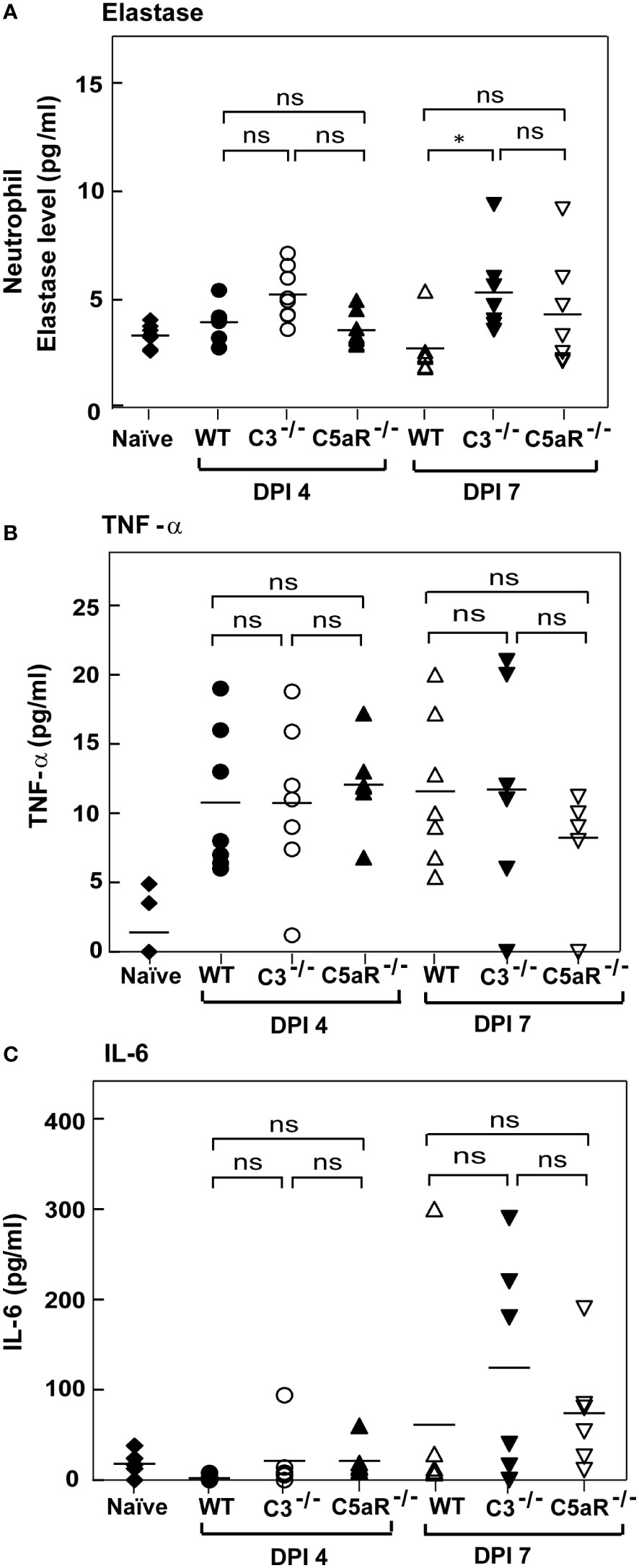
Figure 8 Complement deficient mice with systemic Aspergillus infection show elastase, TNF-alpha and IL-6 comparable to wild-type mice. (A) BALB/c WT, C3-/- and C5aR-/- mice were infected intravenously with 1x105 conidia. Plasma samples from A fumigatus infected mice were obtained on day four and day seven post-infection. Levels of elastase in the infected samples were determined by ELISAs and compared with the WT mice infected with A fumigatus conidia. Results shown are mean concentrations ± SD obtained from six individual mice samples. The statistical significance in complement deficient mice was evaluated by Tukey’s multiple comparison test (*p < 0.05). (B, C) BALB/c WT, C3-/- and C5aR-/- mice were infected intravenously with 1x105 conidia. Serum samples from A fumigatus infected mice were obtained on day four and day seven post-infections. No significant difference was observed with the TNF-α levels. IL-6 levels were increased in C3-/- and C5aR-/- mice on 7 post-infection day compared with infected WT mice, but the difference is not significant. The statistical significance was evaluated by One way ANOVA, followed by Tukey’s multiple comparison test (ns, not significant).
Discussion
A high mortality rate and a limited number of drugs available for treating invasive aspergillosis solicit increased knowledge on the antifungal immune response that controls A. fumigatus infection. The innate immune system forms the first barrier against pathogens, wherein the complement system plays a central role. Earlier in vitro studies have established the role of complement components and complement receptors in detecting and eliminating A. fumigatus conidia. However, the contribution of these entities during in vivo infection is not fully understood. In this study, we assessed the role of the complement system in a mouse model of experimental systemic aspergillosis (26, 27). Our in vivo model mimics the scenario of systemic aspergillosis, where the spores cross the lung barrier to establish systemic infection. In this situation, the complement system is likely to have a more prominent role, as complement proteins are readily available in circulation. In agreement, our data show that the complement system plays an indispensable role during systemic aspergillosis. We demonstrate that the deficiencies of complement component C3 enhance lethality due to decreased uptake-clearance of conidia by the phagocytes. We also show that the C5a-C5aR1 axis plays a critical role in resistance against A. fumigatus, as C5-deficiency increased susceptibility to systemic A. fumigatus infection.
Earlier in vitro studies have shown that all the morphotypes of A. fumigatus are capable of activating the complement system. Dormant conidia primarily activate the alternative pathway, while hyphal morphotypes activate the classical and lectin pathways (13, 14). Further, it has also been documented in vitro that the activation results in the deposition of C3b on the A. fumigatus conidial surface, which facilitate conidial phagocytosis when recognized by the complement receptors CR3 and/or CR4 on the immune cells (20). Whether complement plays a role in protection against systemic aspergillosis has not yet been adequately tested. An earlier study noted a higher degree of lethality in a systemic model of aspergillosis in DBA/2N mice lacking complement C5, compared to complement sufficient outbred CFW mice (26). Activation of C3 is the central step in the complement cascade. Therefore, to investigate the in vivo role of intact complement during systemic infection, we utilized C3-/- mice. We also examined if C3 deficiency on different genetic backgrounds alters the susceptibility to aspergillosis, particularly on Th1-biased C57BL/6 and Th2-biased BALB/c mice. We observed that the C3 deficiency enhances the susceptibility to experimental systemic aspergillosis on both genetic backgrounds. However, the mortality was higher on BALB/c background, which is expected due to the dominance of Th2 response. These results are consistent with the observation that during aspergillosis, administration of Th1 cytokines results in a better outcome (45). It is relevant to point out that an earlier study showed that C3 deficiency enhances susceptibility to systemic Candida infection in mice (46).
Pathogens are known to activate single or multiple complement pathways, offering protection via complement-mediated mechanisms. For example, the classical pathway activation is required for effective immune defense against Streptococcus pneumoniae infection (47) and polymicrobial peritonitis (48), while the lectin pathway is a major defense against pneumococcal infection (49). However, in some pathogens, multiple pathways synergize to provide effective protection (33, 50). To determine the contribution of each of the pathways during systemic A. fumigatus infection, we infected FB-/- (deficient in the alternative pathway) and C4-/- (deficient in the classical/lectin pathways) mice. To our surprise, we observed no difference in the mortality of these mice compared to that in the WT mice. The data thus suggested a possibility of activation of complement without the involvement of C4 and C2. The existence of such a pathway has been known for over four decades now (51) and has been demonstrated in the classical (52, 53) and lectin (54) pathways. Such activation, however, requires an intact alternative complement pathway. Of note, Aspergillus conidia have been shown to activate complement by mannan-binding lectin (MBL) C2 bypass mechanism (15). MBL is a C-type lectin important for activating the lectin pathway. In an earlier study, upon intravenous infection with A. fumigatus conidia, both MBL knockout (both A and C genes deletion on B6.129S4 background) and WT mice (C57BL/6) showed comparable mortality profiles at higher doses of conidia. At the lowest dose, though the death of knockout mice was slower, the survival of MBL-/- mice was not significantly different from that of WT mice (55).
Since C4/C2 bypass pathways are known to involve the alternative pathway and we did not observe increased mortality in FB-/- mice infected with A. fumigatus, we considered a possibility of direct activation of complement by the pathogen-associated protease. To probe this further, we looked for the presence of a conidial protease that can cleave C3 and C5. We indeed found the presence of such a protease associated with conidia that can cleave C3 into a C3b-like fragment and C5 into smaller fragments generating C5a. Apparently, it looks like conidia facilitate their clearance by converting C3 into C3b-like fragment that leads to opsonization-mediated conidial phagocytosis and C5 into C5a that mobilize phagocytes. The interesting question is, then, how does A. fumigatus balances its survival from complement destruction? It should be noted that A. fumigatus produces proteases like Mep1p and Alp1p that are capable of inactivating C3 and C5 and their activation products (C3a and C5a). These proteases are released into the medium containing albumin/collagen (24, 25), suggesting that A. fumigatus conidia escaping from phagocytotic clearance (as in immunosuppressed condition) are well equipped to protect themself in the lung environment where the complement concentration is relatively lower compared to that in the blood.
The late complement components (C5-C9) are essential to clear most infections. In the context of fungal infection, complement C5 has been identified as the dominant gene responsible for altering the susceptibility to systemic Candida infection (56). The late complement components provide protection by two mechanisms: i) by forming a membrane attack complex (MAC; C5b-9) that kills pathogens by pore formation, and ii) as mentioned above, by generating C5a anaphylatoxin that causes the mobilization of neutrophils (57) and monocytes to the site of infection for clearance. Besides, C5a also stimulates neutrophils to produce toxic oxygen-derived free radicals and monocytes to produce inflammatory cytokines. Our infection studies in congenic C5-sufficient and C5-deficient mice indicated that C5 deficiency exhibits significantly higher mortality following systemic A. fumigatus infection. It is therefore clear that C5 contributes to host resistance to A. fumigatus. The next obvious question was how it contributes to the host resistance – owing to MAC-mediated lysis or C5a-mediated immune potentiation. The thick cell wall of conidia/hyphae is expected to block the MAC-mediated lysis of A. fumigatus (18, 58); therefore, it is unlikely that C5 contributes via MAC formation. We thus asked, does the C5a-C5aR1 axis play a part in immune protection? We also evaluated the participation of the C3a-C3aR axis, which has been reported to inhibit neutrophil mobilization (59). Survival analysis of the infected mice revealed enhanced mortality in C5aR1-/-, but not C3aR-/- mice compared to the WT mice. We thus conclude that C5-mediated protection against experimental systemic aspergillosis is owing to C5a-C5aR signaling. Having said that, we would like to point out that the severity of infection in C5aR1-/- mice was comparatively less than in C3-/- mice (see mortality at 1x105 doses of conidia in Figure 1B and Figure 5; both mice on BALB/c background), and therefore signaling through the C5aR1 receptor is only partially responsible for providing complement-mediated protection.
The upregulation of Th1 and proinflammatory cytokines such as IFN-γ, TNF-α, IL-6, IL-12, GM-CSF, IL-1, and IL-18 are associated with a better prognosis of systemic aspergillosis (45). Nagai et al. (42) showed that BALB/c mice upon intravenous A. fumigatus infection showed reduced death from 40-80% to zero when treated with IFN-γ or TNF-α. Camargo et al. (44), on the other hand, showed that the levels of IL-6 were higher after in vitro culturing the peripheral blood mononuclear cell from the healthy and hematological controls but not from the patients with invasive aspergillosis implicating the importance of the IL-6/STAT3 pathway in protective immunity against Aspergillus. Coherent with this, Cenci et al. (43) reported that IL-6-/- mice were more susceptible than WT mice to invasive pulmonary aspergillosis. Intriguingly, the Th1 cytokines IL-6 and TNF-α have a direct link with C5a in that it induces the synthesis of these cytokines (60–63). We thus sought to determine whether the serum levels of these cytokines differ in infected C3-/- and C5aR1-/- mice compared to that in infected wild-type mice. However, the levels of these cytokines were comparable in knockout and wild-type mice, suggesting higher mortality in complement knockout mice is not due to decreased production of these protective cytokines. Next, we examined the uptake of the conidia by phagocytes. Our data revealed that the rate of association of conidia with the phagocytes is significantly higher in WT mice compared to C3-/- mice. Therefore, it appears that the complement system contributes to the clearance of conidia during systemic A. fumigatus infection.
The complement receptors CR1/CR2, which bind to C3-derived ligands, have been shown to shape the natural antibody (nAbs) repertoire (64). These polyreactive low-affinity nAbs contribute to the defense against various infections, including that caused by A. fumigatus (37, 65). It is believed that these nAbs mediate protection by enhancing complement activation and deposition, promoting phagocytosis and antibody-dependent cellular cytotoxicity. Moreover, they inhibit fungal growth, adherence, germination, and biofilm formation (66). We thus investigated whether the C3 deficiency results in a decreased nAbs pool, which in part causes higher lethality in C3-/- mice following systemic A. fumigatus infection. To test this, we passively transferred the naïve heat-inactivated sera from WT or C3-/- mice to C3-/- mice and challenged them with the conidia a day after the transfer. Although the WT mice sera could not rescue the infected mice from lethal infection, it prolonged survival compared to C3-/- mice sera. Consequently, the decreased levels of natural antibodies in C3-/- mice might be another reason responsible for their higher susceptibility to Aspergillus infection.
In summary, our data uncovered the importance of the complement system, an integral part of the humoral immune system of the host, during experimental systemic aspergillosis. Based on our data, we propose the following mechanism for complement-mediated protection against A. fumigatus infection: (i) A. fumigatus conidia are endowed with a protease, which can activate the complement system by a non-canonical pathway, (ii) C3b opsonization that occurs as a result of complement activation enhances the rate of phagocytosis via complement receptors CR3/CR4 (67), and iii) C5a generated owing to complement activation triggers the activation of phagocytic cells via C5aR1, and this results in migration and tissue penetration of phagocytes for fungal clearance. The effect of C5a, however, is not owing to increased synthesis of the cytokines IL-6 and TNF-α.
Data availability statement
The original contributions presented in the study are included in the article/Supplementary Material. Further inquiries can be directed to the corresponding authors.
Ethics statement
The animal study was reviewed and approved by Institutional animal ethics committee (IAEC) of NCCS, Pune.
Author contributions
AS, RS, VA, JP, and GL designed and planned this study. RS, SW, and HM performed different experiments. RS and AS analyzed the data. VA, SW, TM, and AS contributed the reagents, materials, and/or analysis tools. RS and AS drafted the manuscript. All authors contributed to the article and approved the submitted version.
Funding
AS is a J.C. Bose National Fellow. His lab is supported by the Department of Biotechnology, India, Project Grant BT/PR28506/MED/29/1307/2018. VA was supported by FUNHYDRO (ANR-16S-CE110020-01) and FUNPOLYVAC (ANR-21-CE17-0032) grants. SW was supported by Pasteur-Roux-Cantarini postdoctoral fellowship.
Acknowledgments
We thank Mr. Sandip Sonar (National Centre for Cell Science, Pune) for his help during intravenous injections and Dr. B. Ramanamurthy for providing mice. A part of this work was done in partial fulfilment of the Ph.D. thesis of RS submitted to Dr. D.Y. Patil Vidyapeeth, Pune.
Conflict of interest
The authors declare that the research was conducted in the absence of any commercial or financial relationships that could be construed as a potential conflict of interest.
Publisher’s note
All claims expressed in this article are solely those of the authors and do not necessarily represent those of their affiliated organizations, or those of the publisher, the editors and the reviewers. Any product that may be evaluated in this article, or claim that may be made by its manufacturer, is not guaranteed or endorsed by the publisher.
Supplementary material
The Supplementary Material for this article can be found online at: https://www.frontiersin.org/articles/10.3389/fimmu.2022.978152/full#supplementary-material
Supplementary Figure 1 | Experimental strategy for in vivo uptake of conidia by monocyte subsets, eosinophils, and neutrophils.
Supplementary Figure 2 | Histopathological changes in the sections of lung and spleen tissues of mice challenged with A. fumigatus at day 7 post-infection. WT (left side) and C3-/- (right side) mice were infected intravenously with 1 X 105 dose of A. fumigatus conidia and sacrificed at day 7 post-infection. The lungs and spleen were harvested for histopathology. Sections were stained with H & E and are representative of each group (n = 4 to 5, in each group). (A) Typical histopathological changes for lung include Emphysema (), Peri-vascular aggregation of MNCs (>), acute inflammatory status (
). B = bronchi. (B) Typical histopathological changes for the spleen include: hyperplasia of lymphoid tissue in white pulp (
).
Supplementary Figure 3 | Histopathological changes in the sections of liver and kidney tissues of mice challenged with A. fumigatus at day 7 post-infection. WT (left side) and C3-/- (right side) mice were infected intravenously with 1 X 105 dose of A. fumigatus conidia and sacrificed at day 7 post-infection. The liver and kidneys were harvested for histopathology. Sections were stained with H & E and are representative of each group (n = 4 to 5, in each group). a) Examination of kidney section on day 7 showed more promptly focal cellular swelling of renal tubular epithelium in cortico-medullary region in C3-/- mice than WT mice. The changes include cellular swelling of renal tubular epithelium (), and vascular congestion (VC). b) Hepatocyte with intact cellular borders and nucleus was detected in the liver section for WT and C3-/- mice. The nuclear swelling was graded high for liver histopathology in C3-/- mice compared to WT mice liver. The changes include: cellular swelling of hepatocytes (
), granular degeneration (
), nuclear swelling of hepatocytes (
) and vascular congestion (VC).
Supplementary Figure 4 | Periodic acid-Schiff (PAS) staining of the sections of lung, spleen, liver, and kidney tissues of mice challenged with A. fumigatus at day 7 post-infection. WT (upper panel) and C3-/- (lower panel) mice were infected intravenously with 1 X 105 dose of A. fumigatus conidia, sacrificed at day 7 post-infection. The lungs, spleen, liver and kidneys were harvested for histopathology. Representative image of PAS-staining for each group (n = 4 to 5, in each group) for (A) lung, (B) spleen, (C) kidney, and (D) liver. The sections showed mass of hyphae (), hyphae (
), area of very high density hyphae (
) and branched hyphae (
).
References
1. Bader Al, N. and Sheppard DC. Aspergillosis and stem cell transplantation: An overview of experimental pathogenesis studies. Virulence (2016) 7:950–66. doi: 10.1080/21505594.2016.1231278
2. van de Veerdonk FL, Gresnigt MS, Romani L, Netea MG, Latge JP. Aspergillus fumigatus morphology and dynamic host interactions. Nat Rev Microbiol (2017) 15:661–74. doi: 10.1038/nrmicro.2017.90
3. Margalit A, Kavanagh K. The innate immune response to aspergillus fumigatus at the alveolar surface. FEMS Microbiol Rev (2015) 39:670–87. doi: 10.1093/femsre/fuv018
4. Askew DS, Kontoyiannis DP, Clemons KV. Advances against aspergillosis: Biology, host response, diagnosis and treatment. Mycopathologia (2014) 178:321–4. doi: 10.1007/s11046-014-9819-4
5. Carroll MC, Isenman DE. Regulation of humoral immunity by complement. Immunity (2012) 37:199–207. doi: 10.1016/j.immuni.2012.08.002
6. Heeger PS, Kemper C. Novel roles of complement in T effector cell regulation. Immunobiology (2012) 217:216–24. doi: 10.1016/j.imbio.2011.06.004
7. Lyubchenko T, dal Porto J, Cambier JC, Holers VM. Coligation of the b cell receptor with complement receptor type 2 (CR2/CD21) using its natural ligand C3dg: Activation without engagement of an inhibitory signaling pathway. J.Immunol (2005) 174:3264–72. doi: 10.4049/jimmunol.174.6.3264
8. Dunkelberger JR, Song WC. Role and mechanism of action of complement in regulating T cell immunity. Mol.Immunol (2010) 47:2176–86. doi: 10.1016/j.molimm.2010.05.008
9. Schonheyder H, Andersen P. Complement-binding antibodies to aspergillus fumigatus in patients with pulmonary aspergillosis. Acta Pathol Microbiol Immunol Scand B (1983) 91:1–7. doi: 10.1111/j.1699-0463.1983.tb00001.x
10. Neth O, Jack DL, Dodds AW, Holzel H, Klein NJ, Turner MW. Mannose-binding lectin binds to a range of clinically relevant microorganisms and promotes complement deposition. Infect.Immun (2000) 68:688–93. doi: 10.1128/IAI.68.2.688-693.2000
11. Hummelshoj T, Ma YJ, Munthe-Fog L, Bjarnsholt T, Moser C, Skjoedt MO, et al. The interaction pattern of murine serum ficolin-a with microorganisms. PLoS One (2012) 7:e38196. doi: 10.1371/journal.pone.0038196
12. Moalli F, Doni A, Deban L, Zelante T, Zagarella S, Bottazzi B, et al. Role of complement and fc{gamma} receptors in the protective activity of the long pentraxin PTX3 against aspergillus fumigatus. Blood (2010) 116:5170–80. doi: 10.1182/blood-2009-12-258376
13. Kozel TR, Wilson MA, Farrell TP, Levitz SM. Activation of C3 and binding to aspergillus fumigatus conidia and hyphae. Infect Immun (1989) 57:3412–7. doi: 10.1128/iai.57.11.3412-3417.1989
14. Sturtevant JE, Latge JP. Interactions between conidia of aspergillus fumigatus and human complement component C3. Infect Immun (1992) 60:1913–8. doi: 10.1128/iai.60.5.1913-1918.1992
15. Dumestre-Perard C, Lamy B, Aldebert D, Lemaire-Vieille C, Grillot R, Brion JP, et al. Aspergillus conidia activate the complement by the mannan-binding lectin C2 bypass mechanism. J Immunol (2008) 181:7100–5. doi: 10.4049/jimmunol.181.10.7100
16. Braem SG, Rooijakkers SH, Van Kessel KP, de Cock H, Wosten HA, Van Strijp JA, et al. Effective neutrophil phagocytosis of aspergillus fumigatus is mediated by classical pathway complement activation. J Innate Immun (2015) 7:364–74. doi: 10.1159/000369493
17. Rosbjerg A, Genster N, Pilely K, Skjoedt MO, Stahl GL, Garred P. Complementary roles of the classical and lectin complement pathways in the defense against aspergillus fumigatus. Front Immunol (2016) 7:473. doi: 10.3389/fimmu.2016.00473
18. Speth C, Rambach G. Complement attack against aspergillus and corresponding evasion mechanisms. Interdiscip Perspect Infect Dis (2012) 2012:463794. doi: 10.1155/2012/463794
19. Hunniger K, Bieber K, Martin R, Lehnert T, Figge MT, Loffler J, et al. A second stimulus required for enhanced antifungal activity of human neutrophils in blood is provided by anaphylatoxin C5a. J Immunol (2015) 194:1199–210. doi: 10.4049/jimmunol.1401845
21. Tsai HF, Chang YC, Washburn RG, Wheeler MH, Kwon-Chung KJ. The developmentally regulated alb1 gene of aspergillus fumigatus: Its role in modulation of conidial morphology and virulence. J Bacteriol (1998) 180:3031–8. doi: 10.1128/JB.180.12.3031-3038.1998
22. Behnsen J, Hartmann A, Schmaler J, Gehrke A, Brakhage AA, Zipfel PF. The opportunistic human pathogenic fungus aspergillus fumigatus evades the host complement system. Infect Immun (2008) 76:820–7. doi: 10.1128/IAI.01037-07
23. Vogl G, Lesiak I, Jensen DB, Perkhofer S, Eck R, Speth C, et al. Immune evasion by acquisition of complement inhibitors: The mould aspergillus binds both factor h and C4b binding protein. Mol Immunol (2008) 45:1485–93. doi: 10.1016/j.molimm.2007.08.011
24. Shende R, Wong SSW, Rapole S, Beau R, Ibrahim-Granet O, Monod M, et al. Aspergillus fumigatus conidial metalloprotease Mep1p cleaves host complement proteins. J Biol Chem (2018) 293:15538–55. doi: 10.1074/jbc.RA117.001476
25. Behnsen J, Lessing F, Schindler S, Wartenberg D, Jacobsen ID, Thoen M, et al. Secreted aspergillus fumigatus protease Alp1 degrades human complement proteins C3, C4, and C5. Infect Immun (2010) 78:3585–94. doi: 10.1128/IAI.01353-09
26. Hector RF, Yee E, Collins MS. Use of DBA/2N mice in models of systemic candidiasis and pulmonary and systemic aspergillosis. Infect Immun (1990) 58:1476–8. doi: 10.1128/iai.58.5.1476-1478.1990
27. Mirkov I, Stojanovic I, Glamoclija J, Stosic-Grujicic S, Zolotarevski L, Kataranovski D, et al. Differential mechanisms of resistance to sublethal systemic aspergillus fumigatus infection in immunocompetent BALB/c and C57BL/6 mice. Immunobiology (2011) 216:234–42. doi: 10.1016/j.imbio.2010.04.007
28. Thau N, Monod M, Crestani B, Rolland C, Tronchin G, Latge JP, et al. Rodletless mutants of aspergillus fumigatus. Infect Immun (1994) 62:4380–8. doi: 10.1128/iai.62.10.4380-4388.1994
29. Sahu A, Lambris JD. Structure and biology of complement protein C3, a connecting link between innate and acquired immunity. Immunol Rev (2001) 180:35–48. doi: 10.1034/j.1600-065X.2001.1800103.x
30. Watanabe H, Numata K, Ito T, Takagi K, Matsukawa A. Innate immune response in Th1- and Th2-dominant mouse strains. SHOCK (2004) 22:460–6. doi: 10.1097/01.shk.0000142249.08135.e9
31. Bidula S, Sexton DW, Yates M, Abdolrasouli A, Shah A, Wallis R, et al. H-ficolin binds aspergillus fumigatus leading to activation of the lectin complement pathway and modulation of lung epithelial immune responses. Immunology (2015) 146:281–91. doi: 10.1111/imm.12501
32. Abad A, Fernandez-Molina JV, Bikandi J, Ramirez A, Margareto J, Sendino J, et al. What makes aspergillus fumigatus a successful pathogen? Genes and molecules involved in invasive aspergillosis. Rev Iberoam Micol (2010) 27:155–82. doi: 10.1016/j.riam.2010.10.003
33. Rattan A, Pawar SD, Nawadkar R, Kulkarni N, Lal G, Mullick J, et al. Synergy between the classical and alternative pathways of complement is essential for conferring effective protection against the pandemic influenza A(H1N1) 2009 virus infection. PLoS Pathog (2017) 13:e1006248. doi: 10.1371/journal.ppat.1006248
34. Horst SA, Itzek A, Klos A, Beineke A, Medina E. Differential contributions of the complement anaphylotoxin receptors C5aR1 and C5aR2 to the early innate immune response against staphylococcus aureus infection. Pathogens (2015) 4:722–38. doi: 10.3390/pathogens4040722
35. Calame DG, Mueller-Ortiz SL, Morales JE, Wetsel RA. The C5a anaphylatoxin receptor (C5aR1) protects against listeria monocytogenes infection by inhibiting type 1 IFN expression. J Immunol (2014) 193:5099–107. doi: 10.4049/jimmunol.1401750
36. Luo L, Li X, Hu X, Hu C, Tang W, Deng S, et al. Anaphylatoxins enhance recruitment of nonclassical monocytes via chemokines produced by pleural mesothelial cells in tuberculous pleural effusion. Am J Respir Cell Mol Biol (2019) 60:454–64. doi: 10.1165/rcmb.2018-0075OC
37. Rapaka RR, Ricks DM, Alcorn JF, Chen K, Khader SA, Zheng M, et al. Conserved natural IgM antibodies mediate innate and adaptive immunity against the opportunistic fungus pneumocystis murina. J Exp Med (2010) 207:2907–19. doi: 10.1084/jem.20100034
38. Boes M. Role of natural and immune IgM antibodies in immune responses. Mol Immunol (2000) 37:1141–9. doi: 10.1016/S0161-5890(01)00025-6
39. Boes M, Prodeus AP, Schmidt T, Carroll MC, Chen J. A critical role of natural immunoglobulin m in immediate defense against systemic bacterial infection. J Exp Med (1998) 188:2381–6. doi: 10.1084/jem.188.12.2381
40. Ahearn JM, Fischer MB, Croix D, Goerg S, Ma M, Xia J, et al. Disruption of the Cr2 locus results in a reduction in b-1a cells and in an impaired b cell response to T-dependent antigen. Immunity (1996) 4:251–62. doi: 10.1016/S1074-7613(00)80433-1
41. Prufer S, Weber M, Stein P, Bosmann M, Stassen M, Kreft A, et al. Oxidative burst and neutrophil elastase contribute to clearance of aspergillus fumigatus pneumonia in mice. Immunobiology (2014) 219:87–96. doi: 10.1016/j.imbio.2013.08.010
42. Nagai H, Guo J, Choi H, Kurup V. Interferon-gamma and tumor necrosis factor-alpha protect mice from invasive aspergillosis. J Infect Dis (1995) 172:1554–60. doi: 10.1093/infdis/172.6.1554
43. Cenci E, Mencacci A, Casagrande A, Mosci P, Bistoni F, Romani L. Impaired antifungal effector activity but not inflammatory cell recruitment in interleukin-6-deficient mice with invasive pulmonary aspergillosis. J Infect Dis (2001) 184:610–7. doi: 10.1086/322793
44. Camargo JF, Bhimji A, Kumar D, Kaul R, Pavan R, Schuh A, et al. Impaired T cell responsiveness to interleukin-6 in hematological patients with invasive aspergillosis. PLoS One (2015) 10:e0123171. doi: 10.1371/journal.pone.0123171
45. Stevens DA. Th1/Th2 in aspergillosis. Med Mycol (2006) 44:S229–35. doi: 10.1080/13693780600760773
46. Tsoni SV, Kerrigan AM, Marakalala MJ, Srinivasan N, Duffield M, Taylor PR, et al. Complement C3 plays an essential role in the control of opportunistic fungal infections. Infect Immun (2009) 77:3679–85. doi: 10.1128/IAI.00233-09
47. Brown JS, Hussell T, Gilliland SM, Holden DW, Paton JC, Ehrenstein MR, et al. The classical pathway is the dominant complement pathway required for innate immunity to streptococcus pneumoniae infection in mice. Proc Natl Acad Sci U S A (2002) 99:16969–74. doi: 10.1073/pnas.012669199
48. Celik I, Stover C, Botto M, Thiel S, Tzima S, Kunkel D, et al. Role of the classical pathway of complement activation in experimentally induced polymicrobial peritonitis. Infect Immun (2001) 69:7304–9. doi: 10.1128/IAI.69.12.7304-7309.2001
49. Ali YM, Lynch NJ, Haleem KS, Fujita T, Endo Y, Hansen S, et al. The lectin pathway of complement activation is a critical component of the innate immune response to pneumococcal infection. PLoS Pathog (2012) 8:e1002793. doi: 10.1371/journal.ppat.1002793
50. Moulton EA, Atkinson JP, Buller RM. Surviving mousepox infection requires the complement system. PLoS Pathog (2008) 4:e1000249. doi: 10.1371/journal.ppat.1000249
51. Atkinson JP, Frank MM. Bypassing complement: Evolutionary lessons and future implications. J Clin Invest (2006) 116:1215–8. doi: 10.1172/JCI28622
52. Matsushita M, Okada H. Alternative complement pathway activation by C4b deposited during classical pathway activation. J Immunol (1986) 136:2994–8.
53. Knutzen Steuer KL, Sloan LB, Oglesby TJ, Farries TC, Nickells MW, Densen P, et al. Lysis of sensitized sheep erythrocytes in human sera deficient in the second component of complement. J Immunol (1989) 143:2256–61.
54. Selander B, Martensson U, Weintraub A, Holmstrom E, Matsushita M, Thiel S, et al. Mannan-binding lectin activates C3 and the alternative complement pathway without involvement of C2. J Clin Invest (2006) 116:1425–34. doi: 10.1172/JCI25982
55. Clemons KV, Martinez M, Tong AJ, Stevens DA. Resistance of MBL gene-knockout mice to experimental systemic aspergillosis. Immunol Lett (2010) 128:105–7. doi: 10.1016/j.imlet.2009.12.021
56. Tuite A, Elias M, Picard S, Mullick A, Gros P. Genetic control of susceptibility to candida albicans in susceptible A/J and resistant C57BL/6J mice. Genes Immun (2005) 6:672–82. doi: 10.1038/sj.gene.6364254
57. Lee EKS, Gillrie MR, Li L, Arnason JW, Kim JH, Babes L, et al. Leukotriene B4-mediated neutrophil recruitment causes pulmonary capillaritis during lethal fungal sepsis. Cell Host Microbe (2018) 23:121–33. doi: 10.1016/j.chom.2017.11.009
58. Kozel TR. Activation of the complement system by pathogenic fungi. Clin Microbiol Rev (1996) 9:34–46. doi: 10.1128/CMR.9.1.34
59. Wu MC, Brennan FH, Lynch JP, Mantovani S, Phipps S, Wetsel RA, et al. The receptor for complement component C3a mediates protection from intestinal ischemia-reperfusion injuries by inhibiting neutrophil mobilization. Proc Natl Acad Sci U S A (2013) 110:9439–44. doi: 10.1073/pnas.1218815110
60. Strey CW, Markiewski M, Mastellos D, Tudoran R, Spruce LA, Greenbaum LE, et al. The proinflammatory mediators C3a and C5a are essential for liver regeneration. J Exp Med (2003) 198:913–23. doi: 10.1084/jem.20030374
61. Ji M, Lu Y, Zhao C, Gao W, He F, Zhang J, et al. C5a induces the synthesis of IL-6 and TNF-alpha in rat glomerular mesangial cells through MAPK signaling pathways. PLoS One (2016) 11:e0161867. doi: 10.1371/journal.pone.0161867
62. Riedemann NC, Guo RF, Hollmann TJ, Gao H, Neff TA, Reuben JS, et al. Regulatory role of C5a in LPS-induced IL-6 production by neutrophils during sepsis. FASEB J (2004) 18:370–2. doi: 10.1096/fj.03-0708fje
63. Kacani L, Banki Z, Zwirner J, Schennach H, Bajtay Z, Erdei A, et al. C5a and C5a(desArg) enhance the susceptibility of monocyte-derived macrophages to HIV infection. J Immunol (2001) 166:3410–5. doi: 10.4049/jimmunol.166.5.3410
64. Holers VM. Complement receptors and the shaping of the natural antibody repertoire. Springer Semin.Immunopathol (2005) 26:405–23. doi: 10.1007/s00281-004-0186-y
65. Bellocchio S, Bozza S, Montagnoli C, Perruccio K, Gaziano R, Pitzurra L, et al. Immunity to aspergillus fumigatus: the basis for immunotherapy and vaccination. Med Mycol (2005) 43 Suppl 1:S181–8. doi: 10.1080/14789940500051417
66. Casadevall A, Pirofski LA. Antibody-mediated protection through cross-reactivity introduces a fungal heresy into immunological dogma. Infect Immun (2007) 75:5074–8. doi: 10.1128/IAI.01001-07
Keywords: Aspergillus fumigatus, complement system, infectious disease, innate immunity, systemic aspergillosis
Citation: Shende R, Wong SSW, Meitei HT, Lal G, Madan T, Aimanianda V, Pal JK and Sahu A (2022) Protective role of host complement system in Aspergillus fumigatus infection. Front. Immunol. 13:978152. doi: 10.3389/fimmu.2022.978152
Received: 25 June 2022; Accepted: 05 September 2022;
Published: 23 September 2022.
Edited by:
Dimitrios C. Mastellos, National Centre of Scientific Research Demokritos, GreeceReviewed by:
Peter Garred, University of Copenhagen, DenmarkMatteo Stravalaci, Humanitas University, Italy
Copyright © 2022 Shende, Wong, Meitei, Lal, Madan, Aimanianda, Pal and Sahu. This is an open-access article distributed under the terms of the Creative Commons Attribution License (CC BY). The use, distribution or reproduction in other forums is permitted, provided the original author(s) and the copyright owner(s) are credited and that the original publication in this journal is cited, in accordance with accepted academic practice. No use, distribution or reproduction is permitted which does not comply with these terms.
*Correspondence: Arvind Sahu, YXJ2aW5kc2FodUBuY2NzLnJlcy5pbg==; Vishukumar Aimanianda, dmt1bWFyQHBhc3RldXIuZnI=