- 1Zoologische Staatssammlung München (ZSM-SNSB), München, Germany
- 2Johann-Friedrich-Blumenbach-Institut für Zoologie und Anthropologie, Georg-August-Universität Göttingen, Göttingen, Germany
Achrioptera is a taxon of extremely large and exceptionally colorful stick insects endemic to Madagascar and the Comoros Archipelago. We studied the phylogenetic position of the Achriopterini, comprising the genera Achrioptera and Glawiana, based on a multigene phylogeny and concluded that it is a sister group to other Madagascan phasmids (Anisacanthidae) rather than to Neotropical or Australo-Pacific groups as was suggested in a previous study based on morphology. Our results also point to unresolved relationships (potential paraphyly of Achrioptera), taxonomic issues (elevation of A. punctipes cliquennoisi to species level), and detection of cryptic diversity (in A. impennis), demonstrating the need of additional research. A DNA barcoding approach based on COI sequences of Achrioptera species revealed a clear discrimination between closely related and morphologically similar species. Applying integrative taxonomy using multiple lines of evidence, we demonstrated that the well-known species with blue males from Montagne des Français and Foret d'Orangea in the far north of Madagascar, previously attributed to Achrioptera fallax, represents a new species, which we describe as Achrioptera manga sp. nov. based on morphological, chromatic, and genetic (mitochondrial and nuclear) differences. We also describe a second new giant species from this massif: Achrioptera maroloko sp. nov. is among the largest insects (females reaching up to 24 cm total length) and differs from its sister species A. spinosissima from western Madagascar in morphology, coloration, and substantial DNA barcode divergence. These magnificent new species confirm the significance of the Montagne des Français area as a hotspot of biodiversity and microendemism. The biogeographic pattern of the species pair A. fallax/A. manga is paralleled by species pairs of reptiles and amphibians suggesting a similar evolutionary history. Finally, we discuss the sexual dichromatism of Achrioptera species with conspicuous males and mostly cryptic females. As possible reasons, we consider female mate choice and divergent habits of males and females, but aposematism combined with toxic substances produced in defense glands or accumulated in the insect's body from nutritional plants are more plausible explanations for this phenomenon.
Introduction
Madagascar is one of the largest islands and a global biodiversity hotspot (Myers et al., 2000; Goodman and Benstead, 2003). Its biota has evolved in isolation from other major landmasses for more than 65 million years, making it a prime model region to explore mechanisms of evolutionary diversification (Vences et al., 2009). Insular environments (including large islands) generally favor the evolution of unusual morphology and behavior, including extreme sexual dimorphism, miniaturization, and gigantism (e.g., Lomolino, 2005; Meiri et al., 2008; Miralles et al., 2017).
Stick insects (order Phasmatodea) are widespread herbivores in tropical environments and famous for their excellent plant mimesis. Walking leaves (family Phylliidae) almost perfectly mimic green leaves of plants, whereas most other stick insects are brownish and imitate branches and twigs. Mimesis effectively reduces the predation risk of these insects and—together with other key innovations (Shelomi et al., 2016)—might have significantly contributed to the evolutionary success of the Phasmatodea. Most of the ca. 3,100 known species (Bradler and Buckley, 2018) are entirely cryptically colored. The warning colors of the few aposematic taxa are often restricted to the hindwings (alae) and become only visible when the insects are threatened and display their open wings (Bedford, 1978). This display can be associated with other behaviors including stridulation (e.g., in Achrioptera) or spraying chemical defense liquids from glands in the prothorax (e.g., in Peruphasma schultei). Only very few aposematic phasmid species show their coloration also when undisturbed (e.g., Oreophoetes peruana, Eisner et al., 1997), including the adult males of several large Achrioptera species, which permanently present their extremely colorful bodies to the environment. Remarkably, their juvenile stages and their much larger females are cryptically colored.
Over the last decades, substantial progress in the alpha-taxonomy of phasmids has led to the discovery and description of many new species (e.g., Conle et al., 2011) especially from tropical environments, and large-scale molecular studies of phasmids have started to elucidate their phylogenetic relationships (Buckley et al., 2009, 2010; Bradler et al., 2014, 2015; Robertson et al., 2018). However, the stick insects of many regions remain poorly studied and, despite a significant increase of publications in recent years (Hennemann and Conle, 2004; Cliquennois, 2007, 2008), the knowledge of Malagasy Phasmatodea is exceptionally poor. This is also reflected in the low number of described species (77 species according to Brock et al., 2017, Phasmida Species File online) and by the absence of any summarizing paper or book chapter (e.g., this insect group is not covered by Goodman and Benstead, 2003). The only exception is the charismatic genus Achrioptera Coquerel, 1861, which is widespread in Madagascar and has one endemic species on the Comoro Islands. This genus, which includes the largest insects of Madagascar, was revised by Hennemann and Conle (2004), who recognized nine species and provided hypotheses on the phylogenetic relationships of the Achriopterini based on morphological characters. These authors already studied two Achrioptera species from Montagne des Français in north Madagascar and identified them as A. spinosissima (Kirby, 1891) and A. fallax Coquerel, 1861, respectively, interpreting the slight differences to previously known populations from other localities as intraspecific variation. Subsequent fieldwork, captive breeding, as well as additional morphological and molecular studies, led to the paper presented here. We investigate the phylogenetic position and internal phylogeny of the Achriopterini based on a multigene approach and apply DNA barcoding for the identification of species within this group. Based on an integrative approach, using multiple lines of evidence, we describe two new giant species from the far north of Madagascar with extremely colorful males and discuss possible evolutionary scenarios to explain these unusual traits.
Materials and Methods
Morphological Measurements
For better comparison, the terminology and morphological description scheme follows the Achrioptera revision of Hennemann and Conle (2004). Since these authors studied all available type specimens in the genus Achrioptera in great detail, we refrained from re-examining the type material and relied on the identification keys in this revision for the diagnosis of the new species described herein. Bragg (1997) was used for clarification when the definitions of terms were unclear. All specimens of the newly described species that were studied are stored in the Zoologische Staatssammlung München (ZSM) and the Mention Zoologie et Biodiversité Animale of the University of Antananarivo (UADBA). We used field numbers of F. Glaw (FGZC) and M. Vences (ZCMV) to identify all type specimens and other individuals used for this study. For morphological measurements we used a digital caliper to the nearest 0.1 mm and a ruler to the nearest 1.0 mm.
Molecular Laboratory Work and Computational Analyses
We extracted genomic DNA from eggs or muscle tissue using the NucleoSpin® Tissue kit (Macherey-Nagel) following the standard protocol provided by the manufacturer. Following the protocol used in our previous studies (Buckley et al., 2009, 2010; Goldberg et al., 2014; Bradler et al., 2015) we amplified two mitochondrial (COI, COII) and nuclear gene regions (H3, 28S), ~2.3 kb in total, for 33 new samples. We sequenced COI of 32 further specimens using the primers Jerry (Simon et al., 1994) and TOM1 (Ribera et al., 2010) with the protocol of Hebert et al. (2003). We then edited and quality-checked sequences and generated an alignment of a combination of newly and previously sequenced taxa (see Supplementary Tables S1,S2) in Geneious v7.0.1 (available at http://www.geneious.com) and BioEdit v.7.05.3 (Hall, 1999) and then aligned datasets of individual genes in Muscle v.3.6 (Edgar, 2004). We employed the Akaike information criteria (AIC) as implemented in Modeltest v3.745 to select a suitable model of sequence evolution for the combined data.
We separately analyzed two datasets: (1) The DNA barcoding dataset consisting of 49 sequences of Achrioptera and Glawiana with Heteropteryx dilatata as outgroup, and (2) the multigene dataset comprising sequences of 71 taxa of Phasmatodea. The multigene dataset was rooted with the North American stick insect taxon Timema, which has been repeatedly demonstrated to form the sister group of all remaining stick and leaf insects (= Euphasmatodea) (Whiting et al., 2003; Klug and Bradler, 2006; Bradler, 2009; Tomita et al., 2011; Gottardo et al., 2012). Phylogenetic trees of both datasets were reconstructed with the GTR substitution model selected for all partitions using Maximum Likelihood (ML) analyses in MEGA 5.2.2 (Tamura et al., 2011) and Bayesian Inference (BI) analyses in MrBayes 3.1.247 (Ronquist and Huelsenbeck, 2003). ML analyses used 1,000 bootstrap repeats. BI used four independent Markov Chain Monte Carlo (MCMC) runs for 10 million generations with a burn-in of 25% and a tree sampling frequency of 1,000. Trees sampled after burn-in of the four runs were merged and used to construct a 50% majority rule consensus tree.
Newly obtained DNA sequences were deposited on GenBank and are listed in Supplementary Tables S1,S2.
Registration of Nomenclature
The electronic version of this article in Portable Document Format (PDF) will represent a published work according to the International Commission on Zoological Nomenclature (ICZN), and hence the new names contained in the electronic version are effectively published under that Code from the electronic edition alone. This published work and the nomenclatural acts it contains have been registered in ZooBank, the online registration system for the ICZN. The ZooBank LSIDs (Life Science Identifiers) can be resolved and the associated information viewed through any standard web browser by appending the LSID to the prefix http://zoobank.org/. The LSID for this publication is: urn:lsid:zoobank.org:pub:8DAF68FE-4BDA-4511-B9B0-20B7E1CEB3CD. The online version of this work will be archived and made available from the following digital repositories: Biotaxa (http://biotaxa.org/).
Results and Discussion
DNA Barcoding and Multigene Phylogeny of the Achriopterini
The final DNA barcoding tree (Figure 1) comprised 49 sequences of 416 bp each. Base frequencies were not significantly different from equal (Chi-square tests, df = 123; p = 1.0). The final alignments are available on DRYAD (http://www.datadryad.org/). ML and BI topologies were largely congruent, though BI did not resolve the monophyly of Achrioptera fallax vs. A. manga sp. nov. Otherwise, barcoding data revealed a clear distinction between all studied taxa including the new species described herein. Pairwise sequence divergences are provided in Supplementary Table S3.
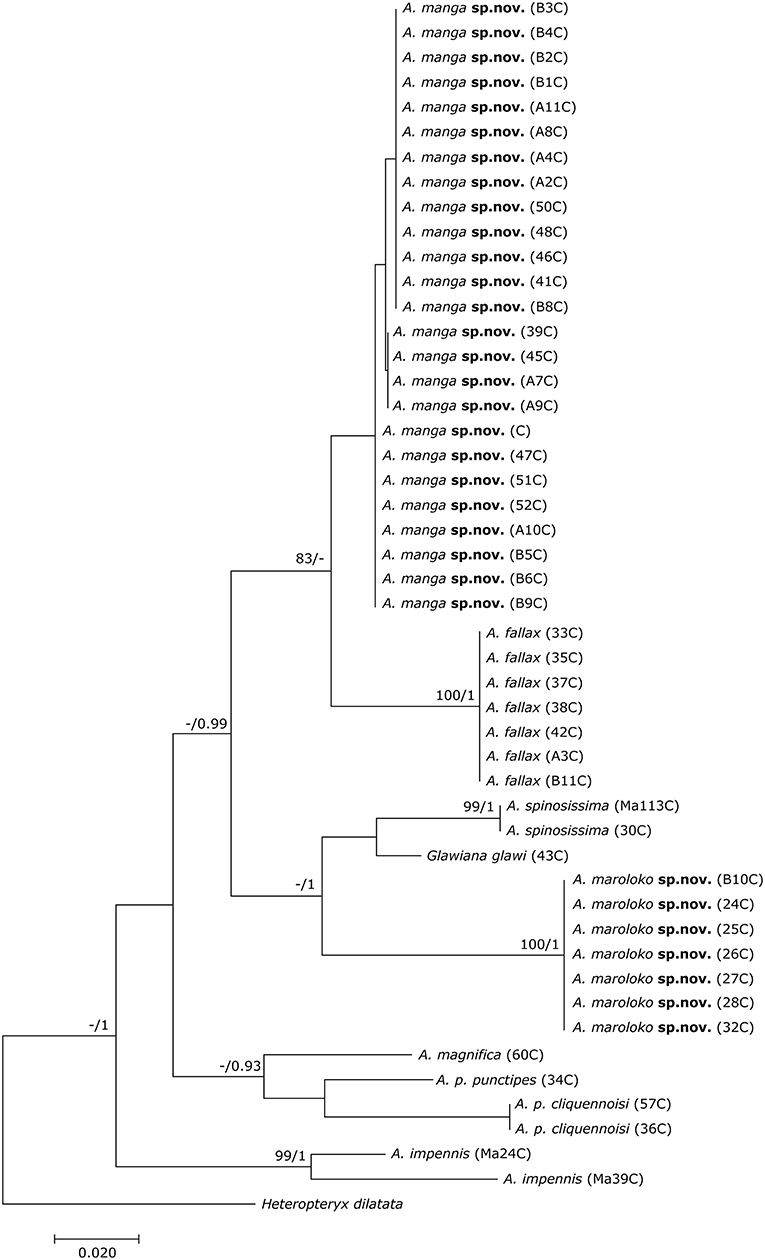
Figure 1. Phylogenetic tree of 48 individuals of Achrioptera and Glawiana, based on DNA barcode sequences of a fragment of the mitochondrial COI gene (Bayesian inference). Heteropteryx dilatata was used as outgroup. ML Bootstrap / BI Posterior Probability support values are given above branches.
The multigene tree (Figure 2) consisted of up to 2.3 kb of nuclear and mitochondrial sequence data for each specimen, depending on gene coverage. We observed excellent support (1.0 BPP) for Aschiphasmatinae, Cladomorphinae, Diapheromerinae, Lanceocercata, Pseudophasmatinae, and Stephanacridini in agreement with previous studies (Whiting et al., 2003; Buckley et al., 2009, 2010; Bradler et al., 2014; Goldberg et al., 2015; Büscher et al., 2018; Robertson et al., 2018). Lonchodinae sensu Bradler et al. (2014) were also supported (0.97 BPP). In contrast to Whiting et al. (2003), Bradler et al. (2014, 2015), Goldberg et al. (2015), and Robertson et al. (2018), Necrosciinae (represented by Sipyloidea and Oxyartes + Phaenopharos) were recovered as polyphyletic. The sister group relationship between Lanceocercata and Stephanacridini was supported (0.92 BPP) as previously suggested (Buckley et al., 2009, 2010; Bradler et al., 2014). Diapheromerinae as sister group to the majority of phasmatodean lineages (0.96 BPP) is in accordance with results obtained by Whiting et al. (2003) and Bradler et al. (2014). The early branches are entirely formed by wingless taxa (Timema, Bacillus, Diapheromerinae, Zehntneria, Spinonemia, and Sceptrophasma), a pattern also observed by Whiting et al. (2003). Agathemera was recovered as sister group to Cladomorphinae (1.0 BPP). The Heteropteryginae were supported as monophyletic (0.99 BPP). Its subgroups Datamini and Heteropteryginae were also well-supported (1.0 BPP). However, Obrimini was recovered as paraphyletic in respect of Datamini with Hoploclonia as sister to the latter (1.0 BPP).
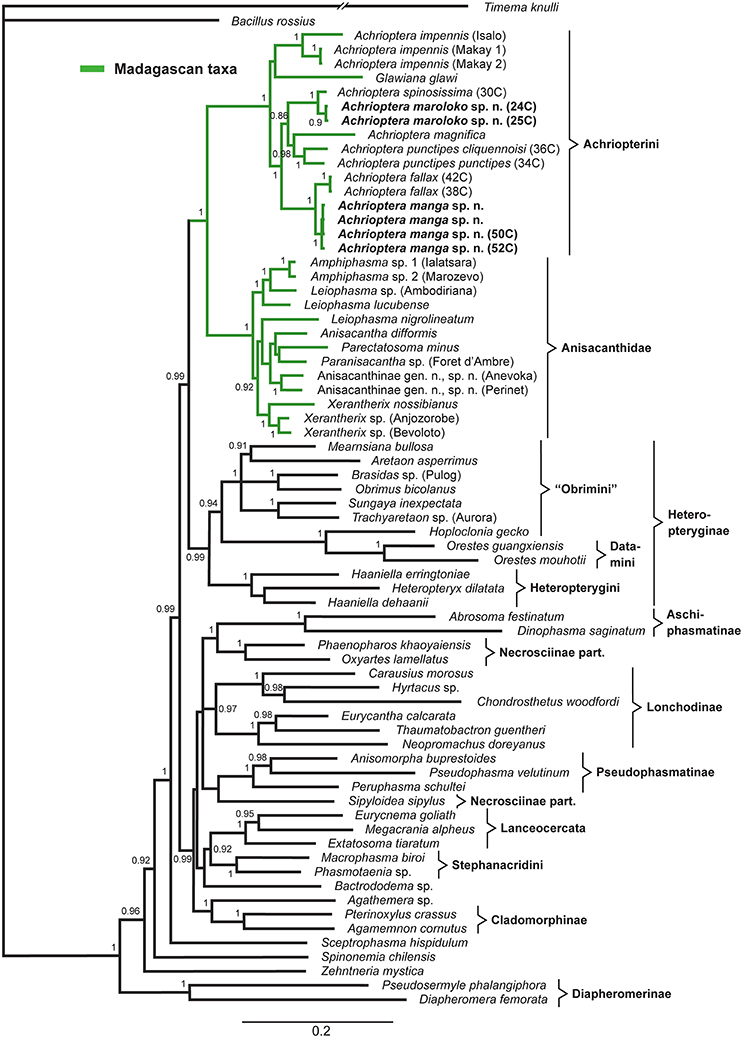
Figure 2. Bayesian phylogenetic tree of 71 phasmatodean specimens showing relationships of the Achriopterini (based on ca. 2.3 kb of mitochondrial and nuclear sequence data). Bayesian Posterior Probability (PP) is given as support value above nodes.
Both the Achriopterini and Anisacanthidae were supported as monophyletic (1.0 BPP) and as sister groups to each other (1.0 BPP), as suggested earlier (Bradler et al., 2015; Goldberg et al., 2015; Büscher et al., 2018). We recovered Achriopterini + Anisacanthidae to be a sister group of Heteropteryginae (1.0 BPP). Within Anisacanthidae, the genera Amphiphasma and Xerantherix appear to be well-established (1.0 BPP), whereas Leiophasma was recovered as paraphyletic. Within Achriopterini, we found no support for a sister group relationship between Achrioptera and the monotypic Glawiana, the latter being possibly more closely related to A. impennis. However, due to lack of support, the phylogenetic position of Glawiana could not be conclusively established. The remaining Achrioptera spp. formed a well-supported lineage (1.0 BPP) with three pairs of sister taxa (1.0 BPP each): (1) A. fallax + A. manga sp. nov., (2) A. magnifica + A. punctipes, and (3) A. maroloko sp. nov. + A. spinosissima. Within this clade, A. fallax + A. manga sp. nov. are sisters to the remaining four species. A. impennis from Makay and from Isalo show distinct genetic differentiation in both mitochondrial and nuclear genes, suggesting that they may belong to different species. The same is true for the two subspecies A. punctipes punctipes and A. p. cliquennoisi. We therefore elevate the subspecies A. punctipes cliquennoisi to species level (A. cliquennoisi).
Identity, Morphology, Coloration, and Reproduction of Achrioptera fallax
Achrioptera fallax was described by Coquerel (1861) from the type locality “Port Leven,” a coastal locality in northeast Madagascar, which is apparently close to the small island Nosy Manambiby (12°46′00″S, 49°47′00″E), one of the northernmost islands in the Nosy Ankao group (Goodman et al., 2017). Hennemann and Conle (2004) could not trace the male holotype of A. fallax in the collection of the Museum nationale d'histoire naturelle (MNHN) in Paris, but the original description by Coquerel (1861) is accompanied by an excellent color figure of the holotype (reproduced in Figure 3A), which clearly shows its morphological and chromatic characteristics. Photographs of males from Ankarana, Andrafiamena, and Daraina agree with the holotype in coloration whereas the populations from further north differ substantially in color (Figures 3B, 4D). Based on the distinctive color differences of males from northern and southern populations, the name A. fallax can be unambiguously applied to the greenish southern populations whereas no name is available for the blueish northern form, which will be described below.
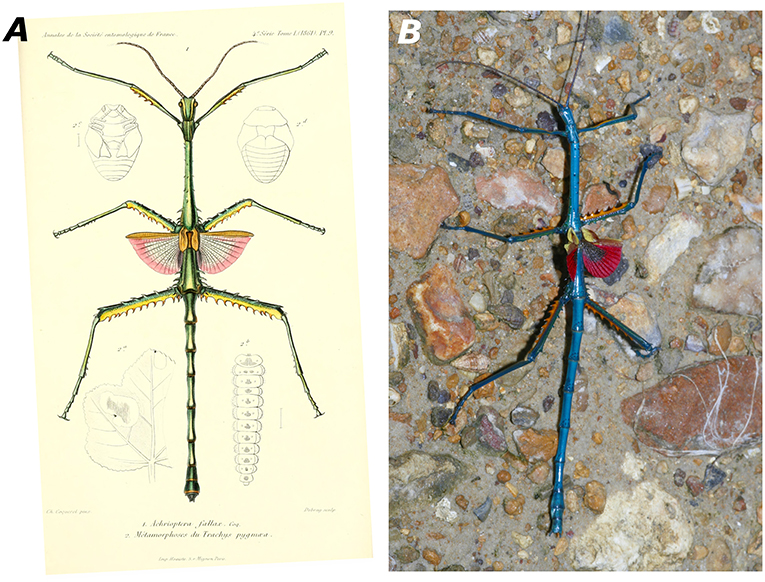
Figure 3. (A) Male holotype of Achrioptera fallax from Port Leven (specimen probably lost) as depicted in the original description (Coquerel, 1861, Pl. 9, Figure 1). (B) Male paratype of A. manga sp. nov. (FGZC 1334) from Foret d'Orangea. With the discovery that the name A. fallax has to be applied to the green rather than to the blue species, the epithet “fallax” (meaning spurious) is now finally and truly fitting.
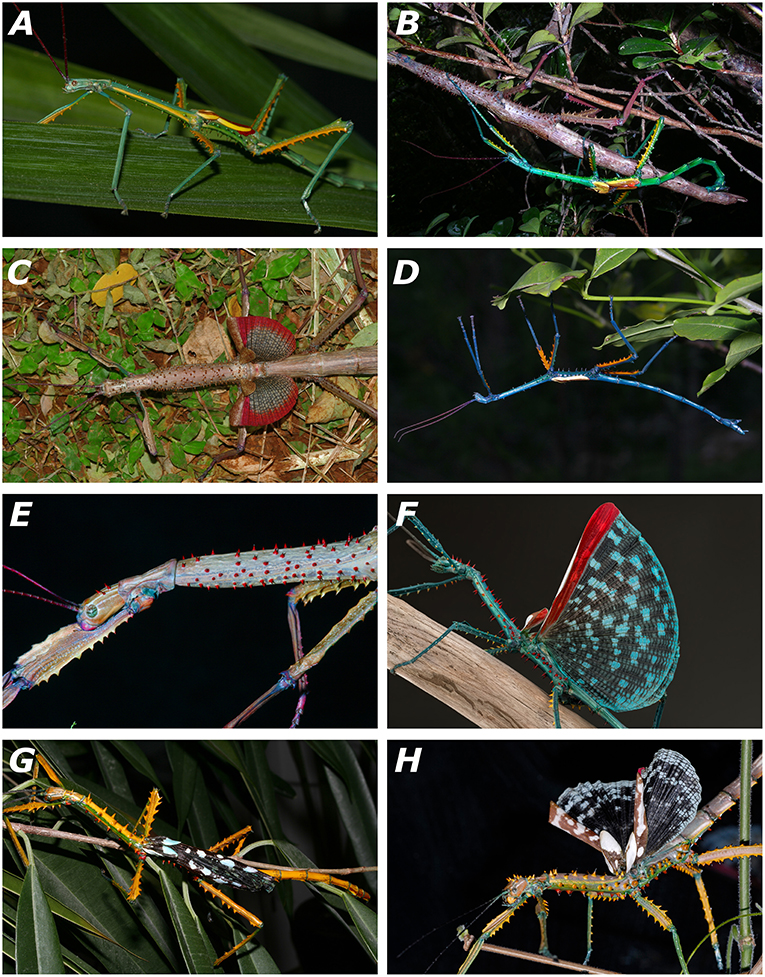
Figure 4. Living specimens of the newly described species of Achrioptera. (A) A. fallax, adult male, captive-bred, reared from eggs of a female from Ankarana (FGZC 1853); (B) A. fallax, mating couple from Daraina (Bekaraoka) (photo by Louis Nusbaumer); (C) A. fallax, adult female with exposed wings from Ankarana National Park (FGZC 1853); (D) A. manga, adult male paratype (FGZC 1334) from Foret d'Orangea; (E) A. manga, adult female; (F) A. spinosissima, adult male reared from eggs collected at Marofandilia, near the type locality in west Madagascar (photo by Bruno Kneubühler); (G) A. maroloko sp. nov., adult male paratype (FGZC 4052) reared from eggs collected at Montagne des Français in dorsolateral view; (H) A. maroloko, adult female paratype (FGZC 4055), bred in captivity from ancestors of Montagne des Français (photo by Moritz Grubenmann).
Females of A. fallax were first described by Hennemann and Conle (2004), but four of the five females studied by them (those from Orangea, Montagne des Français, and Diego Suarez) are from northern populations and therefore refer to the new species described below, whereas the remaining fifth female has no locality data. We therefore characterize the only available adult female specimen from a southern population (A. fallax sensu stricto, FGZC 1853 from Ankarana, Figure 4C): total length (head plus body) 207 mm, including subgenital plate 216 mm. Generally very similar to the females of the northern populations (A. manga sp. nov.) as described by Hennemann and Conle (2004), but generally with more spines (7 spines on pronotum, 17 spines on head, 4 spines on tergum II, 7 spines on sternum III, 1 spine on sternum IV, 1 distinct pair of spines on sternum 7) and a light brown base of the spines on head and thorax. Photographs of another female from Daraina (Bekaraoka, Figure 4B) show at least 2 spines on the pronotum, many spines on head, 4 spines on tergum II, 4 spines on tergum III, and also a light brown base of the spines on head and thorax.
The female from Ankarana (collected on 13 February 2008) laid several eggs, which appeared very similar but slightly thicker than eggs of A. manga. Eggs were incubated at room temperature. Most juveniles hatched (usually in the early morning) after ca. 120–140 days and appeared slightly darker and slightly larger (ca. 19 mm total length) than those of A. manga. Juveniles were fed with bramble leaves. Both males and females became adult as early as 3 months after hatching (faster than A. manga). Newly molted adult males were cryptically colored (brown) and reached the final coloration (greenish body with yellow lateral stripe) after ca. 1 week. Two young males secreted a blue liquid (probably hemolyph) from articulations of the limbs for unknown reasons. The first adult female did not show any spines on the pronotum (in contrast to its mother). Subsequently, all insects died for unknown reason and the specimens were lost.
Achrioptera manga sp. nov.
ZooBank LSID: urn:lsid:zoobank.org:act:0D7185AB-E205-4CFC-962F-DB0D681E0471urn:lsid:zoobank.org:act:0D7185AB-E205-4CFC-962F-DB0D681E0471
Holotype: FGZC 4058 (ZSM DNA Bank Pha-FG-058), adult dried male in good condition, reared in captivity in 2008 from eggs of one of the three females (ZCMV 5356–5358), which were collected at Foret d'Orangea, near Antsiranana, north Madagascar in 2007.
Paratypes: FGZC 4056, adult dried female, collected at Montagne des Français (12°20′02″S, 49°21′31″E, ca. 100 m a.s.l.), near Antsiranana, north Madagascar in February 2004, by F. Glaw, Jaques, Jaquesamis, M. Puente, and R. Randrianiaina; FGZC 1334 and FGZC 4057, two adult males, collected in the Foret d'Orangea, by F. Glaw, P. Bora, H. Enting, Jaques, J. Köhler, and A. Knoll in March 2007; ZCMV 5356–5358, three dried adult females, and UADBA uncataloged, adult female, same locality and collectors as FGZC 1334, collected in February or March 2007; FGZC 1775, adult male, fixed and preserved in ethanol, collected in foret d'Orangea (pitfall line 2), on 22 February 2008 by S. Megson; FGZC 1896, adult male, fixed and preserved in ethanol, collected in Montagne des Français, base camp, on 1 March 2008 by Jaques. The following paratypes were all reared from several eggs laid by the three females ZCMV 5356–5358 from Orangea and bred in several generations in captivity by F. Glaw: FGZC 4059–4068 and FGZC 4104–4125, all adult females; FGZC 4069–4075, FGZC 4078–4102, all adult males; FGZC 4127–4135, all juveniles.
Diagnosis: Females differ (according to the identification key of Hennemann and Conle, 2004, in which they are treated under the name A. fallax) from known females of all other Achrioptera species except A. spinosissima, A. fallax and A. maroloko sp. nov. (females are unknown from A. pygmaea and A. lobipes) by a pair of small spines on the prosternum (normally present vs. absent); from A. spinosissima and A. maroloko sp. nov. by smaller body length (<220 mm) and shorter alae (<30 vs. >50 mm). Females of A. manga sp. nov. differ from those of the most similar A. fallax by differences in coloration of the anal region of the alae (proximally blackish with indistinct reticulations, bordered by a dark red band distally vs. proximally gray with darker reticulations, bordered by a light red band distally), by a reddish base of the spines on head and thorax (Figure 4E) vs. light brown (Figure 4C), and generally by fewer spines (0 vs. 2–7 on pronotum, 0–10 vs. 17 on head, mostly 2 (rarely 4) vs. 4 on sternum II, 0–3 vs. 4–7 on sternum III, 0 vs. 1 on sternum IV).
Males differ (according to the identification key of Hennemann and Conle, 2004) from known males of all other Achrioptera species (males are unknown from A. gracilis and A. griveaudi) as follows: From A. impennis by fully developed alae (vs. rudimentary, hardly visible alae) and by blue dorsal body coloration (vs. cryptic brown coloration); from A. pygmaea and A. lobipes by larger body length (>129 vs. <96 mm); from A. spinosissima and A. maroloko sp. nov. by unarmed pronotum, prosternum and sterna IV–VI (vs. spinose); from A. fallax by blue dorsal body coloration (vs. green), by orange ventral parts of femora (vs. yellow), and by coloration of anal region of alae (proximally gray, distally light red to rosy, overall with dark reticulations, becoming less distinct distally vs. proximally blackish, distally dark red, with indistinct reticulation); from all other species (including the junior synonyms A. intermedia and A. composita, see Redtenbacher, 1908; Carl, 1913) by short alae (<20 mm vs. >33 mm).
In addition to the morphological and chromatic differences, A. manga differs from its sister species A. fallax by distinct differentiation in mitochondrial and nuclear genes (Figures 1, 2).
Description of the male holotype FGZC 4058: dried specimen in good condition. Total length (from tip of head to end of abdomen) 154.7 mm; head length 6.2 mm. Body slender (maximum body width 5.4 mm) with long antennae (slightly bent 43.9 mm, stretched length ~45 mm) reaching to posterior margin of mesonotum. Short tegmina (8.1 mm) and short alae (19.5 mm from insertion of tegmina to end of folded alae). Body surface glabrous and smooth.
Coloration of dried holotype: overall color of body and legs bluish green; ventral surfaces of femora including carinae and spines bright orange. Tibiae and dorsal surface including spines of femora bluish green. All femora irregularly covered with minute white dots. Head with a thin white median line in posterior half, and two lateral lines on each side of which the inner is much more prominent and broader than the outer, starting above the eyes and running along the complete length of the head capsule; another very short white line directly behind the eye. Antennae brownish, scapus, and pedicellus bluish green. Pronotum with a bold, longitudinal white stripe along lateral margins with an incomplete transverse connection, forming a “H-like” marking. Median segment with indistinct, irregular whitish markings. Eyes brownish. Folded tegmina yellowish with a brownish lateral margin, broadening knob-like distally. Folded alae yellowish with broad brown margin.
Head: elongate, 1.5× longer than wide, cylindrical, slightly narrowing toward posterior margin. Dorsal side of head flat and smooth. Eyes strongly convex and prominently projecting from head capsule. Antennae with 28 segments. Scapus and pedicellus almost quadrate, pedicellus smaller than scapus.
Thorax: pronotum slightly longer than head, 2.0× longer than wide, and with a pair of lateral gland openings at front margin. Pronotum and prosternum without spines. Mesothorax elongate, 2.5× longer than length of head and pronotum combined, slightly broadened at posterior margin. Mesonotum anteriorly with two small spines, mesosternum with five small spines posteriorly, and mesopleurae with each one slightly backcurving spine in posterior quarter. Metasternum densely covered with long pointed spines. Spines of meso- and metapleurae large, slightly backcurving, arranged in one longitudinal line of nine spines on each side. Tegmina oval, narrowing toward base. Alae not reaching posterior margin of median segment.
Abdomen: segments II–VI cylindrical, slightly medially constricted. VII slightly shorter than VI, distinctly medially constricted and of bone-like appearance. Sternum II with a posterior pair of medium sized, straight spines, and an additional isolated spine in the middle of the sternum. Terga VIII strongly widening toward posterior margin, trapezoidal, slightly more than half the length of VII. IX 2/3 of the size of VIII. Tergum X as long as IX, almost 1.5 × longer than wide, posterior margin raised and with a broad but flat medial notch. Cerci obtuse, slightly projecting over abdominal tergum X. Vomer broad, triangular, lateral margins raised.
Legs: all slender, mid-legs projecting over tergum III, hind-legs reaching posterior margin of abdominal tergum VII. Carinae of profemora raised and smooth. Probasitarsus longer than following two tarsomeres combined. Mesofemora laterally compressed, ventral carinae with seven large, irregular spines, postero-dorsal carina with five spines. Ventral carinae of mesotibiae very minutely tuberculate, dorsal carinae smooth. Metafemora broadened, ventral carinae with 8–10 very prominent, long and pointed spines, dorsal carinae each with 3–4 spines in basal half. Each dorsal carina with an enlarged and slightly lobe-like apical tooth. Ventral carinae of metatibiae with several minute teeth in apical half, dorsal carinae smooth. Meso- and metabasitarsus longer than the following two tarsomeres combined, ventral carinae usually with a single, minute tooth.
Description of the female paratype ZCMV 5356: dried specimen in good condition. Total length (from tip of head to end of subgenital plate) 204.1 mm; head length 9.8 mm. Body moderately slender (maximum body width 8.8 mm) with long antennae (slightly bent 46.1 mm, stretched length ~48 mm; left antenna partly missing) almost reaching to posterior margin of mesonotum. Short tegmina (13.2 mm) and short alae (29.4 mm from insertion of tegmina to end of folded alae). Body surface glabrous, thorax spinose.
Coloration of dried specimen after several years of preservation in ethanol: general color of body and legs in various shades of brown and gray. Head brown with seven white longitudinal lines, region between the eyes brown without lines. Eyes brownish. Antennae, scapus, and pedicellus brown. Pronotum largely light gray to whitish with five longitudinal brown lines in the posterior half. Mesonotum brownish, darker than abdomen, posterior region with whitish speckles. Spines on body orange brown with dark tips. Tegmina and alae brown with distinctly lighter yellowish veining.
Head: elongate, 1.7× longer than wide, cylindrical, slightly narrowing toward posterior margin. Dorsal side of head flat with one distinct spine. Eyes strongly convex and prominently projecting from head capsule. Antennae with 29 segments. Scapus and pedicellus almost quadrate, pedicellus smaller than scapus.
Thorax: pronotum slightly shorter than head, 1.5× longer than wide. Pronotum with a pair of lateral gland openings at front margin, without spines. Prosternum with a pair of small spines. Mesothorax elongate, 2.0× combined length of head and pronotum, slightly convex in dorsal view, broadest in middle, all over and densely set with numerous pointed spines. Metasternum very densely covered with long pointed spines. Spines of meso- and metapleurae large, slightly backcurving, spines of metapleurae arranged in two longitudinal lines of seven spines on each side, spines of ventral line pointing downwards. Tegmina oval, narrowing toward base. Alae reaching exactly posterior margin of median segment.
Abdomen: segments II–VI cylindrical, parallel-sided, slightly decreasing in width posteriorly. VII slightly shorter than VI. Sternum II with a posterior pair of medium sized, straight spines. Sternum III similar, but only right spine visible, very small, left spine indistinct. Sternum VII with narrow pair of distinct spines. Tergum VIII mediolaterally compressed, slightly less than half the length of VII. IX slightly less than half size of previous. Tergum X as long as IX, with a clear median keel in posterior half, slightly truncate. Cerci not reaching posterior margin of abdominal tergum X. Subgenital plate keeled, but becoming more flattened toward pointed apex, projecting over tergum X by almost the length of the three terminal terga combined (projecting part 15.1 mm).
Legs: all slender, mid-legs projecting over tergum III, hind-legs projecting over posterior margin of tergum VI. Antero-dorsal carina of profemora strongly raised and with five rough, truncate serrations, postero-ventral carina with 11 medium-sized spines. Carinae of protibiae raised and smooth. Probasitarsus longer than following two tarsomeres combined. Mesofemora laterally compressed, ventral carinae with 8 large, irregular spines, antero-dorsal carina with 2 and postero-dorsal carina with 4 (left)/5 (right) spines. Each dorsal carina with an enlarged slightly lobe-like apical tooth. Ventral carinae of mesotibiae with five small spines in apical half, slightly serrate in basal half, dorsal carinae smooth. Metafemora broadened, ventral carinae with 9–10 very prominent, long and pointed spines, dorsal carinae each with 5–4 spines in basal half. Each dorsal carina with an enlarged slightly lobe-like apical tooth, that of the postero-dorsal carina larger. Ventral carinae of metatibiae with five spines in apical half, dorsal carinae smooth. Meso- and metabasitarsus longer than following two tarsomeres combined, ventral carinae of metabasitarsus usually with a single, minute tooth.
Variation: the color of the dried specimens, which were originally preserved in alcohol (FGZC 1334, 4056, 4057, 5356, 5357, 5358), has faded to cream with distally yellow alae. The colors of specimens still stored in 70% ethanol (FGZC 1775, 1896) have faded to dark brown. The coloration of the freshly dried specimens is mostly similar to the types described in detail. In addition to the orange or deeply yellow ventral surfaces of the femora their basis can have a red band. The number of spines on the head is always 0 in males (0–10 in females) and on the pronotum always 0 in males and females. A pair of spines on the prosternum is present in most A. manga females, but can also be absent (this character is used in the identification key of Hennemann and Conle, 2004). The body length varies from 129 to 157 mm in males (total length 135–164 mm) and 176–209 mm in females (total length 189–227 mm).
Eggs: eggs were already described, measured and figured by Hennemann and Conle (2004) based mainly on 14 eggs from a female from Montagne des Français (FGZC 4056) and one dehydrated egg extracted from the ovipositor of a female from Orangea, both here attributed to A. manga.
Etymology: the specific epithet “manga” is an adjective in Malagasy language and means blue, referring to the predominantly blue body color of males. It is used here as an invariable noun in apposition.
Distribution and conservation status: Achrioptera manga has a small distribution range and is currently only known from Montagne des Français (50 km2) and the adjacent Foret d'Orangea (16.4 km2) in far northern Madagascar. Both areas are covered by deciduous dry forest and were threatened by heavy logging until several years ago, but now Montagne des Français appears to be a well-protected communal reserve (F. Glaw, pers. obs. in August 2016) and recently the Orangea region was protected as “Nouvelle aire protégée Oronjia” (http://www.fapbm.org/beneficiaires/nouvelle-aire-protegee-doronjia), indicating that A. manga might not be “Critically Endangered” at current. Hennemann and Conle (2004) mapped an additional (unvouchered) locality of A. fallax in the Sambirano region, which might indeed refer to A. fallax if it can be confirmed. These autors also listed A. fallax specimens from two additional localities, in particular one male from Tsivory and one male from Toirory and located both localities in southern Madagascar. Tsivory is indeed a large village in southeastern Madagascar (−24.068419°, 46.074708°), whereas we could not locate the position of Toirory. For biogeographical reasons we consider these southern localities as unlikely to refer to A. manga, but we cannot exclude that (1) species morphologically similar to A. fallax and A. manga occur in southern Madagascar, (2) localities with these names exist in the north or (3) the historical localities were misspelled. The localities Port Leven, Daraina, Andrafiamena and Ankarana refer to A. fallax.
Reproduction and behavior in capitivity: eggs laid by the three females ZCMV 5356–5358 in February/March 2007 were incubated at room temperature and most juveniles hatched after 120–140 days, with the exception of one juvenile, which only hatched more than 8 months after egg deposition. Both males and females became adult ca. 4.5–5 months after hatching. Newly hatched adult males were cryptically colored (brown) and fully reached their blue coloration after ca. 1 week when they also started first mating attempts. We assume that most (or even all) A. manga currently in culture are descendants of the three paratypes ZCMV 5356–5358, which represented three different COI haplotypes (see Figure 1). When threatened or disturbed, both males and females can open their colorful alae and produce stridulating noises.
Achrioptera maroloko sp. nov.
ZooBank LSID: urn:lsid:zoobank.org:act:52DC7014-DEB7-4095-B56C-2C2D9AB2B26B
Remark: the two wild-captured female paratypes from Montagne des Français (FGZC 4053 and 4051) and some of their eggs were already described and depicted by Hennemann and Conle (2004: Figures 81, 82) in their section “Variation” of Achrioptera spinosissima. These authors already noticed that these two females differ from the holotype of A. spinosissima (and photographs of further females) from the Morondava region in western Madagascar by (l) fewer spines of the mesothorax; (2) posterior spines of head indistinct or lacking; (3) spine on posterolateral angle of abdominal terga II–VI more prominent; (4) smaller number of serrations on the postero-ventral carina of the profemora; (5) white longitudinal line on costal region of alae indistinct, bold white markings instead and (6) pattern of anal region of alae less regular, darker toward bases and black markings of anal areas 1–2 more prominent. Furthermore, the pronotum of both specimens lacks the posterior pair of spines and in one even lacks the anterolateral spines, both seen in the holotype.
Holotype: FGZC 4049 (ZSM DNA Bank Pha-FG-025), adult dried female, reared in captivity from eggs of the female paratype (FGZC 4053), which was collected in 2003 at Montagne des Français (12°18′45″S 49°20′23″E, 100–200 m a.s.l.), north Madagascar.
Paratypes: FGZC 4053, adult female, originally fixed and preserved in ethanol (coloration faded) and subsequently dried, collected at Montagne des Français (12°18′45″S 49°20′23″E, 100–200 m a.s.l.), north Madagascar in February or March 2003 by F. Glaw and Jaqueamis; FGZC 4051 (ZSM DNA Bank Pha-FG-028), adult female (slightly damaged by ants), originally fixed and preserved in ethanol (coloration faded) and subsequently dried, collected at Montagne des Français (southwest of Andavakoera, no coordinates available), north Madagascar on 28 February 2004 by F. Glaw, M. Puente, R. D. Randrianiaina and Jaques; FGZC 4050 (ZSM DNA Bank Pha-FG-026), adult female, reared in captivity from eggs of the female FGZC 4053; FGZC 4052 (ZSM DNA Bank Pha-FG-024), adult male, reared in captivity from eggs of the female FGZC 4051; FGZC 4055, adult female, fixed and preserved in ca. 70% ethanol (grandparents collected at Montagne des Français), reared in captivity by Moritz Grubenmann from parthenogenetically produced eggs laid by either FGZC 4050 or FGZC 4049.
Additional material: FGZC 4054 (ZSM DNA Bank Pha-FG-032), juveniles, preserved in ethanol, captive-bred (mother from Montagne des Français); two dried juveniles without number (offspring of FGZC 4053, hatched in January or February 2004); 6 eggs (one of these eggs sampled: ZSM DNA Bank Pha-FG-027), offspring of FGZC 4053, hatched in January or February 2004; 5 boxes with numerous eggs (partly hatched), offspring of FGZC 4053, one of the eggs unsuccessfully sampled (ZSM DNA Bank Pha-FG-029).
Diagnosis: females differ (according to the identification key of Hennemann and Conle, 2004) from known females of all other species of Achrioptera except A. spinosissima, A. fallax and A. manga (females are unknown from A. pygmaea and A. lobipes) by a pair of small spines on prosternum (vs. no spines on prosternum) and from A. gracilis (155 mm), A. griveaudi (185 mm), A. impennis (144–182 mm), and A. magnifica (156.5–176 mm) by larger size (206–219 mm body length). In addition, they can be distinguished from the poorly known A. griveaudi by the presence of 4–6 spines on the pronotum (vs. absence), and from A. p. punctipes and A. p. cliquennoisi by numerous differences in the coloration of body and wings (e.g., dorsal spines on body yellowish vs. bright green). They differ from A. fallax and A. manga sp. nov. by reduced alae (vs. fully developed) and from A. spinosissima by anal region of alae with dark proximal zone vs. regular pattern of light and dark blotches throughout both proximal and distal zones; possibly by fewer spines on the mesothorax and slightly more colorful body. Further differences in comparison to the holotype of A. spinosissima were noticed by Hennemann and Conle (2004) and are provided above.
Males differ (according to the identification key of Hennemann and Conle, 2004) from known males of all other species of Achrioptera (males are unknown from A. gracilis and A. griveaudi) as follows: from A. impennis by fully developed alae (vs. rudimentary, hardly visible alae) and by yellow dorsal base coloration on body and limbs (vs. cryptic brown coloration); from A. pygmaea and A. lobipes by larger body length (>150 mm vs. <96 mm); and from all other species of Achrioptera (including the junior synonyms A. intermedia and A. composita, see Redtenbacher, 1908; Carl, Hennemann and Conle (1913) except A. spinosissima by spinose pronotum, prosternum and sterna IV–VI (vs. unarmed). The single male of A. maroloko (Figure 4G) differs from A. spinosissima (Figure 4F) by strong differences in coloration (dorsal coloration in life of body and all femora predominantly yellow vs. blue, see Figures 4F,G; mesonotum with yellow spines vs. red spines), dorsal side of head without spines but with a single minute and indistinct tubercle (vs. dorsal side of head with 5–9 distinct spines of red color, Figures 4F,G), pronotum with single pair of yellow spines (vs. pronotum with 2–7 red spines); and anal region of alae with dark proximal zone vs. regular pattern of light and dark blotches throughout both proximal and distal zones.
In addition to the morphological and chromatic differences, A. maroloko differs from its sister species A. spinosissima by distinct differentiation of mitochondrial genes (Figures 1, 2).
Description of the female holotype FGZC 4049: dried specimen in good condition. Total length (from tip of head to end of subgenital plate, body slightly bent, subgenital plate slightly bent upwards) 226.4 mm (approximate total length 232.0 mm); head length 10.9 mm. Body moderately slender (maximum body width 12.1 mm) with long antennae (50.4 mm, incomplete) not reaching to posterior margin of mesonotum. Short tegmina (18.4 mm) and fully developed alae (62.1 mm from insertion of tegmina to end of folded ala). Body surface glabrous, head and thorax densely spinose, abdomen smooth dorsally, spinose ventrally.
Coloration of dried holotype: general color of body in various shades of brown and gray. Head cream with a median white line on vertex and each one white line running from posterior edge of eye to posterior end of head on each side. Eyes brownish. Antennae dark greenish, scapus, and pedicellus slightly lighter. Pronotum cream, with one indistinct dorsolateral band of more than 1 mm width per side. Mesonotum reddish brown, lighter than abdomen, with one indistinct white, narrow mid-dorsal line. Abdomen greenish brown. Coxae reddish ventrally. Pro- and mesotibiae, and pro- and mesotarsi dark green; tibiae and basitarsi with numerous irregular white spots. Pro- and mesofemora olive green with indistinct white dots arranged in one regular line between carinae. Metatibiae and metatarsi dull olive green with very indistinct white dots. Metafemora dark brown. Spines on mesonotum with dark base, darker tip, and orange ring in between. Spines on head and pronotum similar, but less distinct. Costal region of tegmina white (positioned laterally in folded wing), anal region brownish proximally, whitish distally. Costal region of alae reddish with lighter rosy blotches. Anal region dark brown, slightly transparent and with numerous transparent pale turquoise spots arranged in irregular transverse bands; spots becoming larger and more numerous toward the outer margin. Anal regions 1–2 with two distinct, elongate rectangular blackish markings.
Head: slightly elongate, 1.4× longer than wide, slightly narrowing toward posterior margin. Dorsal side of head with five spines on each side. Eyes strongly convex and prominently projecting from head capsule. Antennae broken, left antenna with 16 segments. Scapus and pedicellus almost quadrate, pedicellus smaller than scapus.
Thorax: pronotum slightly longer than head, 1.6× longer than wide. Pronotum with a pair of lateral gland openings and 4 spines at anterior margin and a pair of spines near the middle. Prosternum with a pair of small spines. Mesothorax elongate, 2.0× combined length of head and pronotum, parallel sided, slightly broadening posteriorly, all over and densely set with numerous long and pointed spines. Spines on metasternum arranged in five partly irregular pairs. Spines of meso- and metapleurae large, spines of metapleurae arranged in two longitudinal lines of six spines on each side, posterior most pair reduced, spines of ventral line pointing downwards. Tegmina oval, narrowing toward base, costal region always folded downward. Alae almost reaching posterior margin of abdominal tergum III.
Abdomen: segments II–VII cylindrical, parallel-sided. VII slightly shorter than VI. Sterna II to VII with 2 pairs of medium sized, straight spines. Sternum III similar, but only right spine visible, very small, left spine indistinct. Sternum VII with narrow pair of distinct spines. Terga II–V with a ± prominent spine on the posterolateral edge. Tergum VIII mediolaterally compressed, about 2/3 the length of VII. IX slightly less than half size of previous. Tergum X 1/3 longer than IX, with a moderately distinct median keel in posterior half, slightly broadening toward apex, posterior margin rounded. Cerci distinct, spine-like, not projecting over posterior margin of tergum X. Subgenital plate keeled, but becoming more flattened toward pointed apex, projecting far over tergum X.
Legs: all moderately slender, hind-legs projecting over posterior margin of abdominal tergum VI. Antero-dorsal carina of profemora with 5 distinct serrations, postero-ventral carina with 10 medium-sized spines. Inner carina of profemora smooth, with one proximal small spine. Carinae of protibiae raised, undulate. Probasitarsus longer than following two tarsomeres combined. Mesofemora laterally compressed, ventral carinae with 6–8 large, irregular spines, dorsal carinae with 7–8 large spines. Ventral carinae of mesotibiae with 6–7 minute spines, dorsal carinae slightly undulate. Metafemora broadened, ventral carinae with 9–10 large spines, dorsal carinae each with 8–9 spines. Ventral carinae of metatibiae with 9–10, dorsal carinae smooth. Meso- and metabasitarsus longer than following two tarsomeres combined, ventral carinae of metabasitarsus with 2 minute teeth.
Description of the male paratype FGZC 4052: dried specimen in moderate condition (right ala damaged, right protarsus missing). Medium-sized, total length (from tip of head to end of abdomen) 155.1 mm; head length 7.4 mm. Body slender (maximum body width 6.6 mm) with long antennae (48.5 mm, incomplete) reaching beyond posterior margin of mesonotum. Short tegmina (14.9 mm) and fully developed alae (49.8 mm from insertion of tegmina to end of folded ala). Body surface glabrous, head smooth, thorax densely spinose, abdomen smooth dorsally, spinose ventrally.
Coloration of dried paratype: body and legs with areas of dark green and of dull orange to brown, legs covered with minute white spots. All femora dull orange. All tibiae with shades of dark green and of dull orange, portion of orange higher in pro- and metatibiae than in mesotibiae. All tarsi dark green. Head with a thin white median line in posterior half, and two white lateral lines on each side. Upper line starting above the eyes, much more prominent and broader than lower but fading toward posterior end of head. Lower line starting at posterior end of eyes and running along complete length of head capsule. Antennae dark green, scapus and pedicellus slightly lighter. Pronotum with a bold, longitudinal white stripe along lateral margins with an incomplete transverse connection, forming an incomplete 'H-like' marking. Distinct brown blotch between the eyes. Eyes brownish. Mesonotum dull orange dorsally, green toward the lateral margins. Mesopleurae yellowish. Mesosternum green laterally, wine red ventrally. Metanotum dark brown, metasternum green laterally, ventrally with a very dark red zone covered with darker red spines. Costal region of tegmina white (positioned laterally in folded wing), anal region brown with a distal whitish to turquoise marking. Costal region of alae wine red with lighter rosy to whitish blotches increasing in size distally. Anal region dark brown, slightly transparent and with numerous transparent pale turquoise spots arranged in irregular transverse bands; spots becoming larger and more numerous toward the outer margin. Anal regions 1–2 with three distinct, elongate rectangular blackish markings. Distal part of right wing damaged.
Head: slightly elongate, 1.5× longer than wide, cylindrical, slightly narrowing toward posterior margin. Dorsal side of head flat and smooth, with a single minute and indistinct tubercle. Eyes strongly convex and prominently projecting from head capsule. Antennae incomplete, 17 segments in left antenna. Scapus and pedicellus almost quadrate, pedicellus smaller than scapus.
Thorax: pronotum slightly longer than head, 1.5× longer than wide. Pronotum with a pair of lateral gland openings at front margin, with one dorsal pair of spines, prosternum with one ventral pair of very small spines. Mesothorax elongate, 2× combined length of head and pronotum, slightly broadened at posterior margin, all over covered with numerous long pointed spines (paired on mesosternum). Metapleurae and metasternum densely covered with long pointed spines, spines on metasternum longer and directed ventrally. Tegmina oval, narrowing toward base. Alae reaching abdominal tergum IV.
Abdomen: segments II–VI cylindrical, slightly medially constricted. VII as long as VI, distinctly medially constricted and of bone-like appearance. Sterna II–VII with a pair of medium-sized spines at the middle of the segment, II–VI with an additional pair at posterior end of segment. Pleura II–VI on each side with a single small lateral spine at posterior end, reduced on VI. Terga VIII half the length of VII, strongly widening toward posterior margin, trapezoidal. IX 3/4 of the size of previous, narrowing toward posterior margin. Tergum X longer than VIII and IX, distal margin concave, with a slight dorsal median keel. Cerci spiniform, directed ventrally, not projecting over tergum X. Vomer indistinctly triangular, lateral margins light brownish, slightly raised.
Legs: all slender, hind legs reaching to anterior margin of abdominal tergum IX. Antero-dorsal carina of profemora with four rough serrations, postero-ventral carina with 10 long and straight pointed spines (decreasing in length toward apex of femora). All carinae of protibiae indistinct, without serrations or spines. Mesofemora laterally compressed, carinae with 5–7 large spines. Ventral carinae of mesotibiae with six very small spines, dorsal carinae smooth. Ventral carinae of metafemora with 7–9 prominent, pointed spines. Dorsal carina of right led with irregularly arranged spines, of left leg almost smooth with 2 minute spines. Ventral carinae of metatibiae with 6–7 medium-sized spines, dorsal carinae smooth. Meso- and metabasitarsus longer than following two tarsomeres combined, ventral carinae usually with a single, minute tooth.
Variation: the colors of the two dried females that were originally preserved in alcohol (FGZC 4051 and 4053), and that of FGZC 4055 (still stored in 70% ethanol) have faded. The coloration of FGZC 4050 (a dried female) is similar to the holotype. The number of spines on the head of females varies from 6 to 10 and on the pronotum from 4 to 6. The three dried female paratypes vary in body length from 206 to 219 mm, and in total length from 227 to 242 mm. The single male paratype (FGZC 4052) is described in detail above.
Eggs: eggs were already described, measured and depicted by Hennemann and Conle (2004) based on eggs from Montagne des Français, here attributed to A. maroloko.
Etymology: the specific epithet “maroloko” is an adjective in Malagasy language and means colorful, but is used here as an invariable noun in apposition.
Reproduction and behavior in capitivity: ca. 20 eggs were obtained from the female captured in February/ March 2003 (FGZC 4053) and incubated in a plastic dish on a wet sponge at room temperature. Almost exactly 1 year later four brown juveniles hatched, but two of them died a few days later. The two remaining juveniles were fed successfully with leaves of bramble, dog roses and in later stages also with oaks. Larger juveniles were brown with branch-like skin texture and displayed a characteristic resting position (resulting in mimesis of a branch): the mid legs (mesofemora and mesotibia) were folded and adpressed along the body and both front legs were stretched forward in parallel. In this position the insects became very similar to a small branch and almost disappeared from human perception. After the penultimate ecdysis of the two female juveniles rudimentary spines became recognizable on the head. All juvenile stages displayed a cryptic brown, branch-like, coloration, but after the last ecdysis the insects became colorful with fully developed spines. Disturbed adult females opened their colorful alae and sometimes also lifted their front legs and secreted liquid from the prothoracic defense glands, but did no longer show the mimetic resting position of the juveniles.
The first female (either FGZC 4049 or FGZC 4050) had its penultimate ecdysis on 1 July and the ultimate ecdysis on 28 July (<5 months after hatching). It started laying of (parthenogenetically produced) eggs 38 days later (on 4 September) and laid ca. 504 eggs in the following 198 days (resulting in an average of 2.5 eggs per day) until its death on 20 March 2005, more than 12 months after hatching. The second female (ultimate ecdysis ca. 28 August) started egg laying on 11 October and laid at least 449 eggs in the following 198 days (on average 2.27 eggs per day) until its death on 26 April 2005, more than 13 months after hatching. The parthenogenetically produced eggs of these two females partly hatched, but the juveniles refused to feed and died quickly. However, at least one of the parthogenetically produced juveniles (FGZC 4055) was successfully reared to an adult female by M. Grubenmann and returned to the ZSM after its death. Eggs obtained from the female FGZC 4051, collected on 28 February 2004, were initially incubated at room temperature and later transferred to an incubator for reptile eggs. Seven juveniles hatched between 11 January and 6 April 2005, ca. 10–13 months (but mostly 12 months) after egg laying. Most juveniles refused to feed and died quickly, but FGZC 4052 was successfully reared to an adult male.
Distribution and conservation status: Achrioptera maroloko is currently only known from Montagne des Français in northern Madagascar (Figure 5). This limestone massif is covered by deciduous dry forest and was threatened by heavy logging until a few years ago, but now appears to be a well-protected communal reserve (F. Glaw, pers. obs. in August 2016). Despite intensive surveys of this massif by the first author and other herpetologists in the period 2000–2016, including numerous nocturnal search excursions, we encountered only two females (although a third female was discovered by N. Cliquennois, pers. comm.), suggesting that this splendid stick insect has low population densities and/or a very cryptic habits, perhaps in the canopy of higher trees. However, based on additional localities listed in Hennemann and Conle (2004) and photographs on the internet, the species complex including A. maroloko and A. spinosissima might be widespread in the dry forest areas of northern and western Madagascar. Photographs taken by C. Foucault in the Anjajavy reserve (−15.005819, 47.23413) in northwestern Madagascar (see http://www.inaturalist.org/taxa/429526-Achrioptera-spinosissima), showing a dark proximal zone of the alae and a relatively colorful body of females, suggest that this population might belong to A. maroloko rather than to A. spinosissima. A “giant green and red Achrioptera walking stick” was mentioned by Preston-Mafham (1991: 203) for the Ampijoroa Forestry (Ankarafantsika National Park) and could also refer to A. maroloko. However, the actual area of occupancy and the range limits of both species are unknown and can only be clarified by future studies. We therefore suggest considering the IUCN conservation of A. maroloko status as Data Deficient.
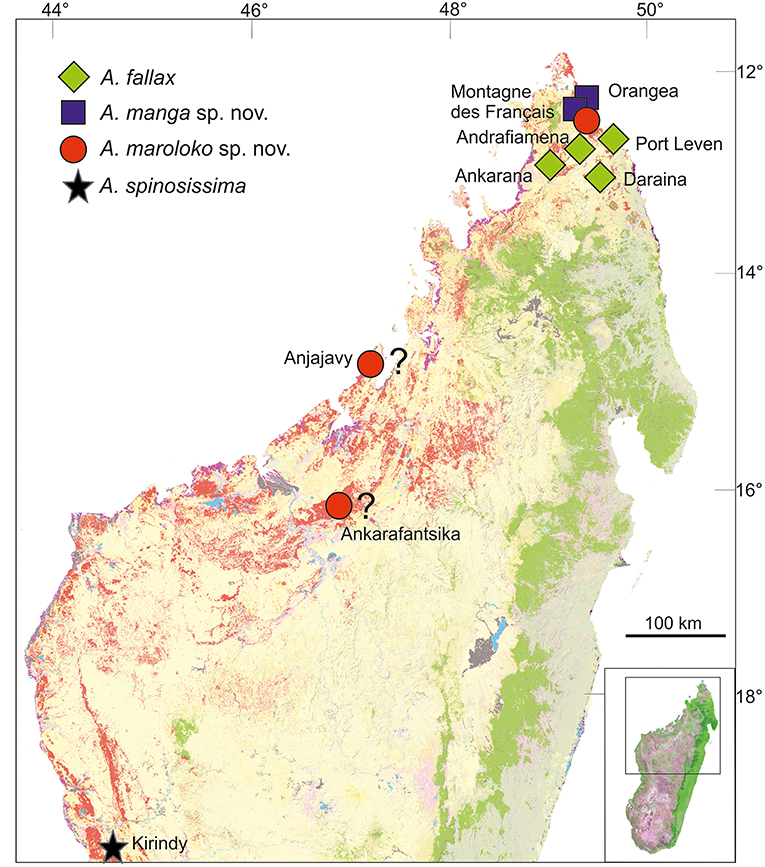
Figure 5. Distribution of Achrioptera fallax, A. manga sp. nov., A. maroloko sp. nov., and A. spinosissima. The localities Ankarafantsika and Anjajavy are uncertain. Orange (dry forest) and green (rainforest) show remaining primary vegetation in 2003–2006, modified from the Madagascar Vegetation Mapping Project (http://www.vegmad.org).
Sexual Dichromatism in Achrioptera and the Conspicuous Coloration of Males
Most phasmids resemble sticks and, typically, their coloration is highly cryptic. Only very few species show conspicuous coloration when undisturbed, though some use their conspicuously colored wings to startle potential predators. The genus Achrioptera is an exception, since the males of most large species permanently present their extremely colorful bodies to the environment and potential predators. There are several possible explanations for this phenomenon, including the following:
1. Female mate choice: only adult males of Achrioptera species are conspicuously colored, while females and juveniles show the typical brown colors that have a strong camouflaging effect and help them mimicking sticks. This could indicate that the male coloration has a signaling function in sexual selection. However, there is no behavioral data that suggests any form of female mate choice in Achrioptera and without an efficient defense mechanism the conspicuous coloration would be prone to strong natural selection by predators, making this explanation unlikely.
2. Cryptic functionality of aposematic coloration combined with different habits of males and females: while appearing conspicuous to the human eye, the green and yellow-black male colorations of A. fallax and A. maroloko (Figure 4) may also have a cryptic function for individuals moving among leaves. In searching for mates, males may be more vagile, which reduces the effect of the stick mimesis that works well for the more sedentary females and juveniles. Disruptive coloration may be more beneficial than mimesis in this case. However, at least for the blue males of A. manga and A. spinosissima it appears unlikely that their coloration can provide any substantial camouflage in the foliage.
3. Aposematism combined with toxic substances produced in defense glands: Achrioptera species possess a pair of prothoracic defense glands, which is a defining autapomorphic character of Phasmatodea and is supposed to be present in all stick and leaf insects (Bradler and Buckley, 2018). These glands are usually well-developed in species exhibiting aposematic coloration [e.g., Oreophoetes, (Eisner et al., 1997); Peruphasma, (Stolz et al., 2015)] and contain repellent substances synthesized by the insects. When disturbed or threatened, both male and female Achrioptera open their colorful wings and often produce stridulating noise simultaneously, which might confuse potential predators and warn them about defensive substances. However, this does not explain why males are conspicuously colored when unthreatened whereas females and juveniles show a cryptic coloration. In Parectatosoma, a member of the closely related Anisacanthidae, highly effective defensive substances are produced (Dossey et al., 2007), but no studies have been conducted yet to detect potential repulsive or toxic substances in Achrioptera spp.
4. Aposematism combined with accumulation of toxic substances from nutritional plants: cryptically colored organism groups like phasmids or frogs are under strong coloration-based selection pressure and accordingly, their freedom in the evolution of deviant colorations is usually very limited. Some frogs (e.g., in the Neotropical family Dendrobatidae and in the genus Mantella from Madagascar) are famous exceptions and display a wide variety of conspicuous and aposematic color pattern (Vences et al., 2003a; Brown et al., 2011). Several studies have demonstrated that the aposematically colored frogs contain potent skin toxins (e.g., Daly et al., 1996) and their chemical components are ingested and accumulated with their food (e.g., ants and termites). Their conspicuous coloration therefore serves as a warning signal of toxicity and enables these frogs relatively safe diurnal activity. A similar mechanism could be hypothesized for the conspicuous coloration of Achrioptera males. Many species of toxic plants occur in the dry forests of Madagascar including Montagne des Français (Lavranos et al., 2001) and Achrioptera might have acquired the ability to digest toxic leaves and to accumulate toxic substances in their bodies, making them uneatable for most predators and allowing them to evolve extreme body colorations like the blue A. manga. Blue body color has also evolved in Neotropical poison frogs (Wollenberg et al., 2006) and such a color change can, at least in frogs, happen rather rapidly in aposematic species (Wang and Shaffer, 2008).
The mechanisms producing warning colorations are diverse and phenotypes are often multifunctional, and subject to biotic and abiotic selection pressures (Briolat et al., 2018). In the absence of substantial data on mate selection, habits, predators, natural food plants, toxins produced by defense glands and possible accumulation of toxins of any Achrioptera species, finding evidence for these ideas and hypotheses, may prove difficult and substantial efforts would be required, also because more than one factor may have played a role in the evolution of this remarkably conspicuous coloration.
Origin and Systematic Position of Achriopterini and Anisacanthidae
Traditionally, the genus Achrioptera was considered to represent a monotypic tribe, Achriopterini, among the species-rich subfamily Phasmatinae (Günther, 1953). However, Phasmatinae has been demonstrated to represent an artificial assemblage, recovered repeatedly as polyphyletic in numerous phylogenetic studies (Whiting et al., 2003; Bradler, 2009; Buckley et al., 2009, 2010; Dunning et al., 2013; Bradler et al., 2014). In more recent molecular phylogenies, Achrioptera is placed as a sister group to the likewise Malagasy stick insect lineage Anisacanthidae (Bradler et al., 2015; Goldberg et al., 2015). Furthermore, a second genus of the Achriopterini, Glawiana, was described (Hennemann and Conle, 2004), and the Achriopterini was discussed either as sister group to the Australo-Pacific Stephanacridini or to the Neotropical Pterinoxylus (Cladomorphinae) (Hennemann and Conle, 2004). We found no support for a close relationship of Achrioptera to either of these groups, namely to Macrophasma + Phasmotaenia (Stephanacridini) or to Pterinoxylus (Cladomorphinae). Instead, Achriopterini is strongly corroborated as a sister group to Anisacanthidae. With both taxa being endemic to Madagascar and the adjacent Comoro Islands, this finding makes sense from a biogeographic point of view. Nonetheless, this result is particularly noteworthy, since Anisacanthidae and Achriopterini are considered to belong to the two different suborders of Phasmatodea, Areolatae, and Anareolatae (Günther, 1953; Zompro, 2004; Cliquennois, 2007, 2008). This traditional subdivision is based on the presence (areolate) or absence (anareolate) of the area apicalis, a demarcated triangular area located ventrally on the apex of the tibiae (Günther, 1953). Numerous previous studies demonstrated that neither Areolatae nor Anareolatae are monophylectic (Whiting et al., 2003; Bradler, 2009; Buckley et al., 2009; Kômoto et al., 2011, 2012; Tomita et al., 2011; Bradler et al., 2014), yet a close relationship between the anareolate Achriopterini and the areolate Anisacanthidae has never been assumed before based on morphological evidence and comes as a major surprise.
Remarkably, the sister groups Achriopterini and Anisacanthidae display an obvious lack of balance in the degree of splitting at the genus and subfamily levels. Although branch lengths between terminal taxa in the studied Achriopterini are generally similar or even longer than in the studied Anisacanthidae (Figure 2), Achriopterini includes only two genera whereas the studied Anisacanthidae includes six described (plus one unnamed) genera and is furthermore divided into three different subfamilies (Anisacanthinae including Anisacantha, Paranisacantha, Parectatosoma, and a presumably unnamed genus; Leiophasmatinae including Amphiphasma and Leiophasma; and Xerantherinae including Xerantherix). Future studies are needed to clarify if this lack of balance correlates with conservatism of morphological evolution in the Achriopterini or indicates shortcomings of the current taxonomy of these groups (e.g., due to oversplitting in the Anisacanthidae). Furthermore, both groups have traditionally been placed at different taxonomic levels (as family in Anisancanthidae and as tribe in Achriopterini), and should be adjusted to the same level once their relationships are sufficiently resolved.
Furthermore, we recovered the areolate Oriental Heteropteryginae to be a sister group of the Malagasy Achriopterini + Anisacanthidae, leading to the assumption of an independent secondary loss of the area apicalis in Achriopterini. The presence of the area apicalis is considered to represent the plesiomorphic condition among stick insects (Bradler, 2009). The hypothesis of a close relationship between Anisacanthidae and Heteropteryginae, with Anisacanthini forming a subgroup of Heteropteryginae, is old (Günther, 1953; Klante, 1976), but was revised by Zompro (2004) who excluded Anisacanthini from Heteropteryginae. This assumption gained acceptance in subsequent studies (Cliquennois, 2007, 2008) and is also supported here.
Hennemann and Conle (2004) argued that Achriopterini “is an evolutionary rather old and unspecialized group of Phasmatodea” due to the animal's large size, long abdomen, strong sexual dimorphism, and presence of wings. These authors proposed a middle to late Jurassic origin of the Achriopterini, when Madagascar was still connected to India, Antarctica and Australia. In contrast, Bradler et al. (2015) estimated a much younger divergence time of 50–35 my between a phylogenetically highly subordinate Achrioptera and Anisacanthidae. Our phylogenetic analysis also resolved Achriopterini to be deeply nested among Phasmatodea as part of a previously unexpected Malagasy radiation. Large size and strong sexual dimorphism as exhibited by Achriopterini might represent a secondary modern trait rather than the plesiomorphic condition within Phasmatodea. Recent descriptions of fossil stick insects further corroborate this assumption, as Mesozoic forms were rather small, ranging from 24 to 56 mm, and lacked noteworthy sexual dimorphism (Nel and Delfosse, 2011; Wang et al., 2014).
Large-Scale Biogeography
A sister-group relationship between Heteropteryginae and Achriopterini + Anisacanthidae indicates a single colonization of Madagascar after transoceanic dispersal from the Asian region, as demonstrated before for various plant and animal species (Warren et al., 2009).
However, this sister group relationship was not suggested before, and Bradler et al. (2015) recovered a monophyletic lineage comprising Anisacanthidae + Achriopterini + further Malagasy species and some African and South American taxa, which could indicate a colonization from Africa (most recently also corroborated by Robertson et al., 2018). Traditionally, the gross phylogeny of Phasmatodea was explained by continental drift vicariance (Günther, 1953). Lately, this view was challenged by molecular clock analyses (Buckley et al., 2009; Bradler et al., 2015), favoring a much younger origin of extant taxa (probably after the Tertiary–Cretaceous transition or K-T boundary, ~66 Ma). Consequently, a high number of transoceanic dispersal events must have shaped the present distribution of stick and leaf insects. The historical biogeography of Malagasy phasmatodeans needs to be addressed in future studies including a more comprehensive taxon sampling from Africa and Madagascar, two largely understudied regions in regards of phasmid systematics.
Apart from unpublished observations (Cliquennois pers. comm.) Achrioptera griveaudi is the only known Comoran species of the genus. It is only known from two female specimens, which were extracted from the stomach of a bird (Leptosomus discolor) at Moheli Island (Paulian, 1960). The volcanic Comoro archipelago consists of oceanic islands surrounded by deep waters, excluding past physical contact to other land masses through fluctuating sea levels (Vences et al., 2003b). Aside from an unlikely aerial transmarine transport by birds (e.g., as a lost or digested prey with surviving eggs; Suetsugu et al., 2018) overseas dispersal from north Madagascar is thus the most plausible hypothesis to explain the presence of this endemic and flightless species on the Comoros and its similarity with A. maroloko. This dispersal mechanism was also used by many other Comoran endemics, including amphibians and reptiles, and is facilitated by prevailing ocean currents (Vences et al., 2003b; Hawlitschek et al., 2017a,b).
Regional Biogeography and Conservation
Northern Madagascar is a mosaic of different geological formations, including old limestone massifs, volcanoes, coastal sands, and other structures. Depending on the topography, climate and vegetation vary substantially as well, ranging from grassland and dry deciduous forest at low altitudes to montane rainforest at high elevations of Montagne d'Ambre. These and other factors have contributed to a remarkable species diversity and endemism in this area. However, the region of Montagne des Français/Orangea has an exceptionally high degree of microendemism, including many species of plants, amphibians and reptiles (Lavranos et al., 2001; D'Cruze et al., 2007; Glaw et al., 2012), and even one microendemic family of blind-snakes (Wegener et al., 2013). The distribution maps of A. fallax and A. manga (Figure 5) point to the existence of a biogeographical border between the Montagne des Français/Orangea region in the north and the adjacent limestone massifs (Ankarana-Andrafiamena-Analamera) further south. Similar distribution patterns of numerous allopatrically distributed sister species pairs suggests a general biogeographic border for different organism groups, such as geckos of the genus Paroedura (Jackman et al., 2008), snakes of the genus Madagascarophis (Glaw et al., 2013; Ruane et al., 2016), and dwarf frogs of the genus Stumpffia (Köhler et al., 2010).
The Montagne des Français region is currently a hotspot for conservation priorities. The massif was unprotected and affected by heavy logging until several years ago (D'Cruze et al., 2007), but is now an apparently well-protected community-based reserve. The hitherto unprotected, degraded forest of Orangea is now being transformed into the National Park Oronjia. The protection of this area is of particular concern not only for the survival of the microendemic A. manga, but also harbors the highest density of critically endangered reptiles in Madagascar (Jenkins et al., 2014) and is home to Lepilemur septentrionalis, one of the most threatened primate species in the world (Ranaivoarisoa et al., 2013).
The two new giant insects described in this paper are among the most spectacular insects in the world. The blue A. manga, hitherto known as A. fallax, has become one of the most famous stick insects after its first successful husbandry and breeding. It is highly desired in the phasmid community and has already served as a model for artwork, paintings, T-shirt prints and others during the past few years. A. maroloko, perhaps even more impressive because of its enormous size, its large spines, and its splendid coloration, might be similarly attractive. These splendid phasmids could therefore be used as flagship species to promote local ecotourism in their habitats, awareness of the unique biodiversity of Madagascar, and the need for its protection.
Data Availability
The datasets generated for this study can be found in NCBI GenBank, https://www.ncbi.nlm.nih.gov/genbank/ (the numerous single accession numbers are to be found in Supplementary Tables S1, S2).
Author Contributions
FG, OH, and SB designed the study. FG, OH, AD, JG, and SB collected the data and wrote the paper. FG, OH, AD, and JG analyzed the data. All authors read, commented on, and approved the final version of the manuscript.
Funding
This work was financially supported by the Volkswagen Foundation, the European Association of Zoos and Aquaria and by Act for Nature to FG, and by the German Science Foundation (DFG grant BR 2930/2-1 and BR 2930/5-1) to SB.
Conflict of Interest Statement
The authors declare that the research was conducted in the absence of any commercial or financial relationships that could be construed as a potential conflict of interest.
Acknowledgments
We are grateful to numerous friends and colleagues: to Moritz Grubenmann for rearing parthenogenetic A. maroloko; to Olivier Arnoult, Nicolas Cliquennois, Moritz Grubenmann, Bruno Kneubühler, and Louis Nusbaumer for providing photographs; to Neil D'Cruze, Falk Sebastian Eckhardt, Arne Hartig, and Bruno Kneubühler for providing crucial tissue samples; to Parfait Bora, Neil D'Cruze, Hildegard Enting, Michael Franzen, Jaques, Jaqueamis, Peter Kappeler, Angelika Knoll, Jörn Köhler, Marta Puente, Steven Megson, and Roger-Daniel Randrianirina for their help in the field work; to York Pareik for his hospitality; to Nicolas Cliquennois, Oskar Conle, Frank Hennemann, Bruno Kneubühler, and Miguel Vences for stimulating discussions; to Tanja Kothe and Bärbel Stock for the preparation of numerous specimens and to Klaus Schönitzer for access to the collection. We are grateful to the Malagasy authorities who kindly issued research and export permits.
Supplementary Material
The Supplementary Material for this article can be found online at: https://www.frontiersin.org/articles/10.3389/fevo.2019.00105/full#supplementary-material
Supplementary Table S1. Specimens and samples used for DNA barcoding (COI) GenBank accession numbers.
Supplementary Table S2. Specimens and samples used for the multigene phylogeny with GenBank accession numbers for each gene.
Supplementary Table S3. Pairwise genetic distances among sequences of Achrioptera taxa in the COI DNA barcoding fragment.
References
Bedford, G. O. (1978). Biology and ecology of the Phasmatodea. Ann. Rev. Entomol. 23, 125–149. doi: 10.1146/annurev.en.23.010178.001013
Bradler, S. (2009). Die Phylogenie der Stab- und Gespenstschrecken (Insecta: Phasmatodea). Spec. Phyl. Evol. 2, 3–139. doi: 10.17875/gup2009-710
Bradler, S., and Buckley, T. R. (2018). “Biodiversity of phasmatodea,” in Insect Biodiversity: Science and Society, Vol. II, eds R. G. Foottit and P. H. Adler (Hoboken, NJ: Wiley-Blackwell), 281–313.
Bradler, S., Cliquennois, N., and Buckley, T. R. (2015). Single origin of the Mascarene stick insects: ancient radiation on sunken islands? BMC Evol. Biol. 15:196. doi: 10.1186/s12862-015-0478-y
Bradler, S., Robertson, J. A., and Whiting, M. F. (2014). A molecular phylogeny of Phasmatodea with emphasis on Necrosciinae, the most species-rich subfamily of stick insect. Syst. Entomol. 39, 205–222. doi: 10.1111/syen.12055
Briolat, E. S., Burdfield-Steel, E. R., Paul, S. C., Rönkä, K. H., Seymoure, B. M., Stankowich, T., et al. (2018). Diversity in warning coloration: selective paradox or the norm? Biol. Rev. 94, 388–414. doi: 10.1111/brv.12460
Brock, P. D., Büscher, T., and Baker, E. (2017). Phasmida Species File Online. Available online at: http://phasmida.Speciesfile.org, version 5.0/5.0 (accessed December 30, 2017).
Brown, J. L., Twomey, E., Amézquita, A., de Souza, M. B., Caldwell, J. P., Lötters, S., et al. (2011). A taxonomic revision of the Neotropical poison frog genus Ranitomeya (Amphibia: Dendrobatidae). Zootaxa 3083, 1–120. doi: 10.11646/zootaxa.3083.1.1
Buckley, T. R., Attanayake, D., and Bradler, S. (2009). Extreme convergence in stick insect evolution: phylogenetic placement of the Lord Howe Island tree lobster. Proc. R. Soc. B 276, 1055–1062. doi: 10.1098/rspb.2008.1552
Buckley, T. R., Attanayake, D., Nylander, J. A. A., and Bradler, S. (2010). The phylogenetic placement and biogeographical origins of the New Zealand stick insects (Phasmatodea). Sys. Ent. 35, 207–225. doi: 10.1111/j.1365-3113.2009.00505.x
Büscher, T. H., Buckley, T. R., Grohmann, C., Gorb, S. N., and Bradler, S. (2018). The evolution of tarsal adhesive microstructures in stick and leaf insects (Phasmatodea). Front. Ecol. Evol. 6:69. doi: 10.3389/fevo.2018.00069
Carl, J. (1913). Phasmides_nouveaux_ou_peu_connus_du_Musém de Genève. Rev. Suisse Zool. 21, 1–56. doi: 10.5962/bhl.part.37151
Cliquennois, N. (2007). Aperçu général de la diversité des phasmes de Madagascar (Insecta, Phasmatodea). Bull. Arthropoda 32, 3–16. Available online at: https://www.researchgate.net/publication/265848904Apercu_general_de_la_diversite_des_phasmes_de_Madagascar_Insecta_Phasmatodea
Cliquennois, N. (2008). Révision des Anisacanthidae, famille endémique de phasmes de Madagascar (Phasmatodea: Bacilloidea). Ann. Soc. Entomol. Fr. 44, 59–85. doi: 10.1080/00379271.2008.10697545
Conle, O. V., Hennemann, F. H., and Gutièrrez, Y. (2011). The Stick Insects of Colombia. Norderstedt: Books on Demand.
Coquerel, C. (1861). Orthoptères de bourbon et de Madagaskar. Ann. Soc. Entomol. Fr. 4, 495–499, pl. 9.
Daly, J. W., Andriamaharavo, N. R., Andriantsiferana, M., and Myers, C. W. (1996). Madagascan poison frogs (Mantella) and their skin alkaloids. Am. Museum Novitates 3177, 1–34.
D'Cruze, N., Sabel, J., Green, K., Dawson, J., Gardner, C., Robinson, J., et al. (2007). The first comprehensive survey of amphibians and reptiles at Montagne des Français, Madagascar. Herpetol. Conserv. Biol. 2, 87–99.
Dossey, A. T., Walse, S. S., Conle, O. V., and Edison, A. S. (2007). Parectadial, a monoterpenoid from the defensive spray of Parectatosoma mocquerysi. J. Nat. Prod. 70, 1335–1338. doi: 10.1021/np070151g
Dunning, L. T., Thomson, G., Dennis, A. B., Sinclair, B. J., Newcomb, R. D., and Buckley, T. R. (2013). Positive selection in glycolysis among Australasian stick insects. BMC Evol. Biol. 13:215. doi: 10.1186/1471-2148-13-215
Edgar, R. C. (2004). MUSCLE: a multiple sequence alignment method with reduced time and space complexity. BMC Bioinformatics 5:113. doi: 10.1186/1471-2105-5-113
Eisner, T., Morgan, R. C., Attygalle, A. B., Smedley, S. R., Herath, K. B., and Meinwald, J. (1997). Defensive production of quinoline by a phasmid insect (Oreophoetes peruana). J. Exp. Biol. 200, 2493–2500.
Glaw, F., Köhler, J., Townsend, T. M., and Vences, M. (2012). Rivaling the world's smallest reptiles: discovery of miniaturized and microendemic new species of leaf chameleons (Brookesia) from northern Madagascar. PLoS ONE 7:e31314. doi: 10.1371/journal.pone.0031314
Glaw, F., Kucharzewski, C., Köhler, J., Vences, M., and Nagy, Z. T. (2013). Resolving an enigma by integrative taxonomy: Madagascarophis fuchsi (Serpentes: Lamprophiidae), a new opisthoglyphous and microendemic snake from northern Madagascar. Zootaxa 3630, 317–322. doi: 10.11646/zootaxa.3630.2.7
Goldberg, J., Bresseel, J., Constant, J., Kneubühler, B., Leubner, F., Michalik, P., et al. (2015). Extreme convergence in egg-laying strategy across insect orders. Sci. Rep. 5:7825. doi: 10.1038/srep07825
Goldberg, J., Knapp, M., Emberson, R. M., Townsend, J. I., and Trewick, S. A. (2014). Species radiation of carabid beetles (Broscini: Mecodema) in New Zealand. PLoS ONE 9:e86185. doi: 10.1371/journal.pone.0086185
Goodman, S. M., Anbdou, Y., Andriamiarantsoa, Y., Fisher, B. L., Griffiths, O., Keitt, B., et al. (2017). Results of a biological inventory of the Nosy Ankao island group, Parc National de Loky-Manambato, northeastern Madagascar. Malagasy Nat. 11, 1–59. Available online at: https://hal.archives-ouvertes.fr/hal-01493362/document
Goodman, S. M., and Benstead, J. P. (eds.) (2003). The Natural History of Madagascar. Chicago, IL; London: University of Chicago Press.
Gottardo, M., Mercati, D., and Dallai, R. (2012). The spermatogenesis and sperm structure of Timema poppensis (Insecta: Phasmatodea). Zoomorph. 131, 209–223. doi: 10.1007/s00435-012-0158-z
Günther, K. (1953). Über die taxonomische Gliederung und geographische Verbreitung der Insektenordnung der Phasmatodea. Beitr. Entomol. 3, 541–563.
Hall, T. A. (1999). BioEdit: a user-friendly biological sequence alignment editor and analysis program for Windows 95/98/NT. Nucl. Acids. Symp. Ser. 41, 95–98.
Hawlitschek, O., Ramirez Garrido, S., and Glaw, F. (2017a). How marine currents influenced the widespread natural overseas dispersal of reptiles in the Western Indian Ocean region. J. Biogeogr. 44, 1435–1440. doi: 10.1111/jbi.12940
Hawlitschek, O., Toussaint, E. F. A., Gehring, P.-S., Ratsoavina, F. M., Cole, N., Crottini, A., et al. (2017b). Gecko phylogeography in the Western Indian Ocean region: the oldest clade of Ebenavia inunguis lives on the youngest island. J. Biogeogr. 44, 409–420. doi: 10.1111/jbi.12912
Hebert, P. D., Cywinska, A., Ball, S. L., and deWaard, J. R. (2003). Biological identifications through DNA barcodes. Proc. R. Soc. Lond. B 270, 313–321. doi: 10.1098/rspb.2002.2218
Hennemann, F. H., and Conle, O. V. (2004). Revision of the tribe Achriopterini Bradley & Galil, 1977, with the description of a new genus, three new species and a new subspecies from Madagascar (Phasmatodea: Phasmatidae: Phasmatinae). Mitt. Münch. Ent. Ges. 94, 5–54.
Jackman, T. R., Bauer, A. M., Greenbaum, E., Glaw, F., and Vences, M. (2008). Molecular phylogenetic relationships among species of the Malagasy-Comoran gecko genus Paroedura (Squamata: Gekkonidae). Mol. Phylogenet. Evol. 46, 74–81. doi: 10.1016/j.ympev.2007.10.018
Jenkins, R. K., Tognelli, M. F., Bowles, P., Cox, N., Brown, J. L., et al. (2014). Extinction risks and the conservation of Madagascar's reptiles. PLoS ONE 9:e100173. doi: 10.1371/journal.pone.0100173
Kômoto, N., Yukuhiro, K., and Tomita, S. (2012). Novel gene rearrangements in the mitochondrial genome of a webspinner, Aposthonia japonica (Insecta: Embioptera). Genome 55, 222–233. doi: 10.1139/g2012-007
Kômoto, N., Yukuhiro, K., Ueda, K., and Tomita, S. (2011). Exploring the molecular phylogeny of phasmids with whole mitochondrial genome sequences. Mol. Phylogenet. Evol. 58, 43–52. doi: 10.1016/j.ympev.2010.10.013
Kirby, W. F. (1891). On the Phasmidae of Madagascar, with the description of a new genus and species in the collection of the British Museum. Ann. Mag. Nat. Hist. 8, 150–152. doi: 10.1080/00222939109460409
Klante, H. (1976). Die “Wandelnden Blätter”. Eine taxonomische Revision der Gattung Phyllium Ill. (Insecta Orthoptera, Phasmatoptera). Zool. Beitr. 22, 49–79.
Klug, R., and Bradler, S. (2006). The pregenital abdominal musculature in phasmids and its implications for the basal phylogeny of Phasmatodea (Insecta: Polyneoptera). Org. Divers. Evol. 6, 171–184. doi: 10.1016/j.ode.2005.08.004
Köhler, J., Vences, M., D'Cruze, N., and Glaw, F. (2010). Giant dwarfs: discovery of a radiation of large-bodied 'stump-toed frogs' from karstic cave environments of northern Madagascar. J. Zool. 282, 21–38. doi: 10.1111/j.1469-7998.2010.00708.x
Lavranos, J. J., Röösli, W., and Hoffmann, R. (2001). Montagne des Français - an ultimate paradise in Madagascar. Cactus Succulent J. 73, 4–11.
Lomolino, M. V. (2005). Body size evolution in insular vertebrates: generality of the island rule. J. Biogeogr. 32, 1683–1699. doi: 10.1111/j.1365-2699.2005.01314.x
Meiri, S., Cooper, N., and Purvis, A. (2008). The island rule: made to be broken? Proc. R. Soc. B 275, 141–148. doi: 10.1098/rspb.2007.1056
Miralles, A., Macleod, A., Rodríguez, A., Ibáñez, A., Jiménez-Uzcategui, G., Quezada, G., et al. (2017). Shedding light on the Imps of Darkness: an integrative taxonomic revision of the Galápagos marine iguanas (genus Amblyrhynchus). Zool. J. Linn. Soc. 20, 1–33. doi: 10.1093/zoolinnean/zlx007
Myers, N., Mittermeier, R. A., Mittermeier, C. G., da Fonseca, G. A., and Kent, J. (2000). Biodiversity hotspots for conservation priorities. Nature 403, 853–858. doi: 10.1038/35002501
Nel, A., and Delfosse, E. (2011). A new Chinese Mesozoic stick insect. Acta Pal. Pol. 56, 429–432. doi: 10.4202/app.2009.1108
Ranaivoarisoa, J. F., Zaonarivelo, J. R., Lei, R., Johnson, S. E., Wyman, T. M., et al. (2013). Rapid survey and assessment of the Northern Sportive Lemur, Lepilemur septentrionalis, in northern Madagascar. Primate Conserv. 27, 23–31. doi: 10.1896/052.027.0109
Redtenbacher, J. (1908). Die Insektenfamilie der Phasmiden, III. Phasmidae Anareolatae (Phibalosomini, Acrophyllini, Necrosciini). Leipzig: Wilhelm Engelmann. p. 341–589, pls. 16–27.
Ribera, I., Fresneda, J., Bucur, R., Izquierdo, A., Vogler, A. P., Salgado, J. M., et al. (2010). Ancient origin of a Western Mediterranean radiation of subterranean beetles. BMC Evol. Biol. 10:29. doi: 10.1186/1471-2148-10-29
Robertson, J. A., Bradler, S., and Whiting, M. F. (2018). Evolution of oviposition techniquesin stick and leaf insects (Phasmatodea). Front. Ecol. Evol. 6:216. doi: 10.3389/fevo.2018.00216
Ronquist, F., and Huelsenbeck, J. P. (2003). MrBayes 3: Bayesian phylogenetic inference under mixed models. Bioinformatics 19, 1572–1574 doi: 10.1093/bioinformatics/btg180
Ruane, S., Burbrink, F. T., Randriamahatantsoa, B., and Raxworthy, C. J. (2016). The cat-eyed snakes of Madagascar: phylogeny and description of a new species of Madagascarophis (Serpentes: Lamprophiidae) from the Tsingy of Ankarana. Copeia 104, 712–721. doi: 10.1643/CH-15-346
Shelomi, M., Danchin, E. G., Heckel, D., Wipfler, B., Bradler, S., Zhou, X., et al. (2016). Horizontal gene transfer of pectinases from bacteria preceded the diversification of stick and leaf insects. Sci. Rep. 6:26388. doi: 10.1038/srep26388
Simon, C., Frati, F., Beckenbach, A. T., Crespi, B., Liu, H., and Flook, P. K. (1994). Evolution, weighting, and phylogenetic utility of mitochondrial gene sequences and a compilation of conserved polymerase chain reaction primers. Ann. Entomol. Soc. Am. 87, 651–701. doi: 10.1093/aesa/87.6.651
Stolz, K., von Bredow, C.-R., von Bredow, Y. M., Lakes-Harlan, R., Trenczek, T. E., and Strau,ß, J. (2015). Neurons of self-defence: neuronal innervation of the exocrine defence glands in stick insects. Front. Zool. 12:29. doi: 10.1186/s12983-015-0122-0
Suetsugu, K., Funaki, S., Takahashi, A., Ito, K., and Yokoyama, T. (2018). Potential role of bird predation in the dispersal of otherwise flightless stick insects. Ecology 99, 1504–1506. doi: 10.1002/ecy.2230
Tamura, K., Peterson, D., Peterson, N., Stecher, G., Nei, M., and Kumar, S. (2011). MEGA5 molecular evolutionary genetics analysis using maximum likelihood, evolutionary distance, and maximum parsimony methods. Mol. Biol. Evol. 28, 2731–2739. doi: 10.1093/molbev/msr121
Tomita, S., Yukuhiro, K., and Kômoto, N. (2011). The mitochondrial genome of a stick insect Extatosoma tiaratum (Phasmatodea) and the phylogeny of polyneopteran insects. J. Ins. Biotech. Sericol. 80, 79–88.
Vences, M., Kosuch, J., Boistel, R., Haddad, C. F. B., La Marca, E., Lötters, S., et al. (2003a). Convergent evolution of aposematic coloration in Neotropical poison frogs: a molecular phylogenetic perspective. Org. Divers. Evol. 3, 215–226. doi: 10.1078/1439-6092-00076
Vences, M., Vieites, D. R., Glaw, F., Brinkmann, H., Kosuch, J., Veith, M., et al. (2003b). Multiple overseas dispersal in amphibians. Proc. R. Soc. Lond. B 270, 2435–2442. doi: 10.1098/rspb.2003.2516
Vences, M., Wollenberg, K. C., Vieites, D. R., and Lees, D. C. (2009). Madagascar as a model region of species diversification. Trends Ecol. Evol. 24, 456–465. doi: 10.1016/j.tree.2009.03.011
Wang, I. J., and Shaffer, H. B. (2008). Rapid color evolution in an aposematic species: a phylogenetic analysis of color variation in the strikingly polymorphic strawberry poison-dart frog. Evolution 62, 2742–2759. doi: 10.1111/j.1558-5646.2008.00507.x
Wang, M., Béthoux, O., Bradler, S., Jacques, F., Cui, Y., and Ren, D. (2014). Under cover at pre-angiosperm times: a cloaked phasmatodean insect from the Early Cretaceous Jehol biota. PLoS ONE 9:e91290. doi: 10.1371/journal.pone.0091290
Warren, B. H., Strasberg, D., Bruggemann, J. H., Prys-Jones, R. P., and Thébaud, C. (2009). Why does the biota of the Madagascar region have such a strong Asiatic flavour? Cladistics 26, 526–538. doi: 10.1111/j.1096-0031.2009.00300.x
Wegener, J. E., Swoboda, S., Hawlitschek, O., Franzen, M., Wallach, V., et al. (2013). Morphological variation and taxonomic reassessment of the endemic Malagasy blind snake family Xenotyphlopidae (Serpentes, Scolecophidia). Spixiana 36, 269–282.
Whiting, M. F., Bradler, S., and Maxwell, T. (2003). Loss and recovery of wings in stick insects. Nature 421, 264–267. doi: 10.1038/nature01313
Wollenberg, K. C., Veith, M., Noonan, B. P., and Lötters, S. (2006). Polymorphism versus species richness - systematics of large Dendrobates from the Eastern Guiana Shield (Amphibia: Dendrobatidae). Copeia 2006, 623–629. doi: 10.1643/0045-8511(2006)6[623:PVSROL]2.0.CO;2
Keywords: Achrioptera, aposematic coloration, biogeography, DNA barcoding, Madagascar, mate choice, new species, multigene phylogeny
Citation: Glaw F, Hawlitschek O, Dunz A, Goldberg J and Bradler S (2019) When Giant Stick Insects Play With Colors: Molecular Phylogeny of the Achriopterini and Description of Two New Splendid Species (Phasmatodea: Achrioptera) From Madagascar. Front. Ecol. Evol. 7:105. doi: 10.3389/fevo.2019.00105
Received: 05 December 2018; Accepted: 15 March 2019;
Published: 02 April 2019.
Edited by:
Anthony I. Cognato, Michigan State University, United StatesReviewed by:
Bjarte Henry Jordal, University of Bergen, NorwayRebecca Barr Simmons, University of North Dakota, United States
Copyright © 2019 Glaw, Hawlitschek, Dunz, Goldberg and Bradler. This is an open-access article distributed under the terms of the Creative Commons Attribution License (CC BY). The use, distribution or reproduction in other forums is permitted, provided the original author(s) and the copyright owner(s) are credited and that the original publication in this journal is cited, in accordance with accepted academic practice. No use, distribution or reproduction is permitted which does not comply with these terms.
*Correspondence: Sven Bradler, sbradle@gwdg.de