- 1Departamento de Química, Universidad de Guanajuato, Guanajuato, Mexico
- 2Departamento de Química, Universidad Autónoma Metropolitana-Iztapalapa, Mexico City, Mexico
- 3Department of Chemistry, Harvey Mudd College, Claremont, CA, United States
6-Triazolylmethyl-pyrrolo[3,4-b]pyridin-5-one tris-heterocycles were synthesized in 43–57% overall yields. The two-stage synthesis involved a cascade process (Ugi-3CR/aza Diels-Alder/N-acylation/aromatization) followed by a copper-assisted alkyne-azide [3+2] cycloaddition (CuAAC). This efficient and convergent strategy proceeded via complex terminal alkynes functionalized with a fused bis-heterocycle at the α-position. The final products are ideal candidates for SAR studies as they possess two privileged scaffolds in medicinal chemistry: 4-substituted or 1,4-substituted 1H-1,2,3-triazoles and pyrrolo[3,4-b]pyridin-5-ones.
Introduction
Polyheterocycles are organic molecules containing three or more heterocyclic moieties, which may be joined by one or more different kinds of connectivity (Ibarra et al., 2018). Nitrogen-containing polyheterocycles are of particular interest in the synthesis of bioactive molecules (Dener et al., 2006; Dolle et al., 2008; Atobe et al., 2013). Tris-heterocyclic molecules have been reported in optics and in coordination chemistry (Stibrany et al., 2003; Burling et al., 2007; Tahara et al., 2009).
The 4-substituted 1H-1,2,3-triazole is a heterocycle of high interest in medicinal chemistry, and it is well-documented that incorporation of this moiety into several bioactive compounds has resulted in advantages such as reduced toxicity or increased antibacterial or antimalarial activity (Shchepin et al., 2008; Zhou et al., 2008) [e.g., Figure 1, compound A (Dixit et al., 2012)]. Triazoles may also increase the stability and polarity of compounds by coordination of the N1 and N2 triazole nitrogens to active-site metal atoms in metalloproteases. The N3 nitrogen appears not to be directly involved in binding metals but can form hydrogen bonds with amino acid residues (Kallander et al., 2005; Huang et al., 2011; Röhri et al., 2012; Borkin et al., 2016). Additionally, 4-substituted 1H-1,2,3-triazoles are precursors of bioactive disubstituted 1,2,3-triazoles (Duan et al., 2009; Oh et al., 2010; Yan et al., 2010; Hsu et al., 2013; Bakulev and Beryozkina, 2016) and of pyridyl-1H-1,2,3-triazolate complexes that have applications in optics and coordination chemistry (Sinn et al., 2014; Prabhath et al., 2015). On the other hand, 1,4-disubstituted 1H-1,2,3-triazoles display structural and electronic similarities with the trans-amide bond. Their overall dipolar moment and hydrogen-bonding properties are greater than those of an amide bond, making these heterocycles effective peptidomimetics (Tron et al., 2008). These triazoles also function as flat bivalent elements, imitating the rigid conformational constraints of double bonds in alkyl chains. 1,4-Disubstituted 1H-1,2,3-triazoles are also capable substitutes for other five-membered nitrogen-containing heterocycles such as imidazoles, pyrazoles, 1,2,4-triazoles, oxazoles, isoxazoles, and oxazolidinones. Finally, these rings can act as more stable isosteres of phosphate linkers (Bonandi et al., 2017).
Common synthetic approaches to 4-substituted 1H-1,2,3-triazoles involve a [3+2] cycloaddition between sodium azide (NaN3) or hydrazoic acid (HN3) and terminal alkynes activated with electron-withdrawing groups (EWGs) (Hartzel and Benson, 1954; Balle et al., 2003; Blass et al., 2006; Koszytkowska-Stawińska et al., 2012). A variation of this classical methodology using trimethylsilyl azide (TMSN3) provides a much safer procedure. The use of a copper(I) catalyst allows the use of non-activated terminal alkynes in shorter reaction times and under milder conditions (Jin et al., 2004). For preparing 1,4-disubstituted 1-H-1,2,3-triazoles, the classic synthetic approach is the regiospecific copper-assisted [3+2] cycloaddition between terminal alkynes with organic azides (Rostovtsev et al., 2002; Tornøe et al., 2002). The most common technique is in situ reduction of copper(II) salts, such as CuSO4•5H2O or Cu(OAc)2, forming copper(I) salts using sodium ascorbate as the reducing agent. A second option is to use a copper(I) salt such as CuCl, CuBr, CuI, [Cu(CH3CN)4]OTf, or Cu(CH3CN)4PF6 in a deoxygenated environment and in organic solvent, typically with an amine such as TEA, DIEA, DIPEA, or PMDETA (Bock et al., 2006; Hein and Fokin, 2010; Lauria et al., 2014).
On the other hand, the fused heterocycle pyrrolo[3,4-b]pyridin-5-one is an aza-analog of isoindolin-1-one natural products and is present in several bioactive molecules; for example: hypoglycemic [Figure 1, compound B (Devasthale et al., 2013)], analgesic, anticancer, and therapeutic agents for CNS-related diseases like Alzheimer's, epilepsy, and schizophrenia (Unverferth et al., 2002; Chang et al., 2008; Pajouhesh et al., 2012; Lindsley et al., 2013). The synthesis of analogs with a brain-selective radioligand has also been reported [Figure 1, compound C (Wager et al., 2017)].
There are no previous reports of molecules containing 4-substituted or 1,4-disubstituted 1H-1,2,3-triazoles and pyrrolo[3,4-b]pyridin-5-ones, though there are a few examples of each of these ring systems connected to other heterocycles using multistep approaches [Figure 1, compounds A-C) (Dixit et al., 2012; Devasthale et al., 2013; Mallemula et al., 2015; Maračić et al., 2015; Wager et al., 2017)].
Isocyanide-based multicomponent reactions (IMCRs) are the most efficient strategies to synthesize pyrrolo[3,4-b]pyridin-5-ones, and Zhu first reported a one-pot synthesis in 2001 (Sun et al., 2001). We synthesized various annulated polyheterocycles containing this fused bis-heterocycle via IMCR strategies (Islas-Jácome et al., 2011, 2012). However, there are no published reports of pyrrolo[3,4-b]pyridin-5-ones linked to other heterocycles in a non-annulated fashion using IMCRs. Recently, Van der Eycken reported a one-pot synthesis of disubstituted pyrrolo[3,4-b]pyridin-5-ones by an Ugi four-component reaction (Ugi-4CR)/carbocyclization/deacylation sequence (Scheme 1; Trang et al., 2015). While that work can generate oxidized 7-hydroxy derivatives, we sought a route that would feature improved atom economy, shorter reaction times, and milder conditions in addition to greater structural complexity with more functionalized products (Scheme 1).
Besson reported the two-stage synthesis of tris-heterocycles with pyrido[2,3-d]pyrimidin-4(3H)-one linked to 1,2,3-triazoles via a one-pot DMFDMA-mediated cyclization followed by a CuAAC (Scheme 1; Hédou et al., 2013). However, only two heterocycles are constructed in this process and the scope of the alkynes is limited.
Our ongoing research program focuses on the design of rapid, convergent and efficient IMCR/post-transformation strategies toward novel molecules containing privileged heterocycles: azepino[4,5-b]indol-4-ones (Rentería-Gómez et al., 2016b), 2-tetrazolylmethyl-isoindolin-1-ones (Rentería-Gómez et al., 2016a), tetrazolo[1,5-a]quinolines (Unnamatla et al., 2016), 3-tetrazolylmethyl-azepino[4,5-b]indol-4-ones (Gordillo-Cruz et al., 2013), 2,3,4,9-tetrahydro-1H-β-carbolines (Cárdenas-Galindo et al., 2014), 4-(pyridine-3-yl)pyrimidines (Cortes-García et al., 2016), and spiro[pyrrolidine-3,3′-oxindoles] (Alvárez-Rodríguez et al., 2018). Herein we describe the first cascade IMCR process/post-transformation strategy toward the synthesis of 4-substituted 1H-1,2,3-triazoles linked to a fused, bis-heterocyclic peptidomimetic. A key aspect of this work is that the cascade IMCR process rapidly generates a complex alkyne for the subsequent [3+2] cycloaddition (Scheme 2).
Results and Discussion
In this work, we report the two-step synthesis of compounds 9a-m and 19a-d, which contain three different heterocycles: pyridine, pyrrolidin-2-one, and 1H-1,2,3-triazole (4-substituted and 1,4-disubstituted) (Scheme 3). The use of orthogonal, bifunctional reagents plays a central role in the IMCR/post-transformation strategy, leading to the rapid generation of molecular complexity in both bis-heterocycles 6a-m and final products 9a-l. In the first step, the synthesis of 6a-m occurs by a cascade process combining an Ugi-3CR with aza Diels-Alder, N-acylation, and aromatization reactions to give a complex terminal alkyne functionalized at the α-position with a fused bis-heterocycle. Two fused rings were created in the process, resulting in pyrrolo[3,4-b]pyridin-5-ones 6a-m.
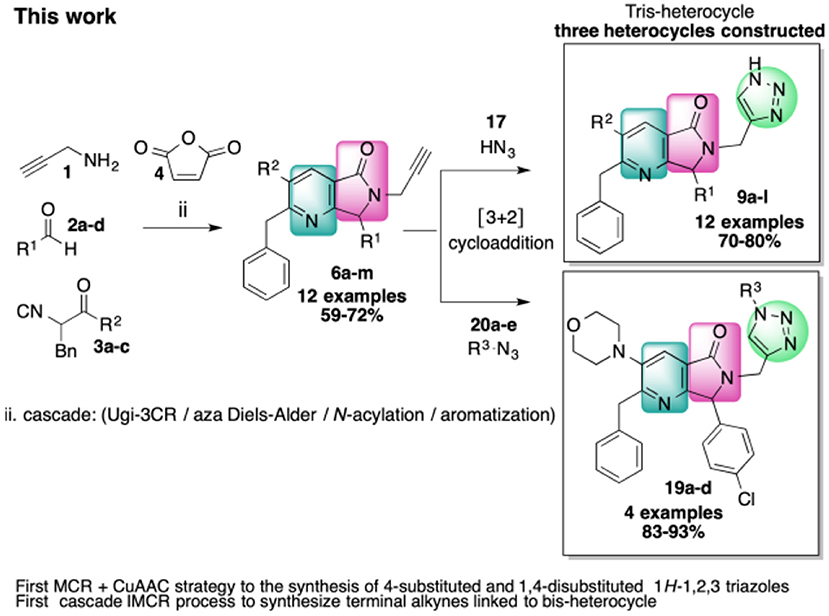
Scheme 3. Synthesis of 4-substituted 1H-1,2,3-triazoles 9a-l and 1,4-disubstituted 1H-1,2,3-triazoles 19a-l via an IMCR/aza Diels-Alder/N-acylation/aromatization/[3+2] cycloaddition sequence.
To optimize the cascade process, we attempted to synthesize complex alkyne 6a by sequentially reacting propargylamine (1), benzaldehyde (2a), isocyanide 3a, and maleic anhydride (4) using toluene (Table 1). Performing the reaction without catalyst at 60–80 °C under conventional conditions produced 6a in 13% yield (entry 1, Table 1). When the reaction was carried out using catalytic amounts of NH4Cl at 60–80 °C (Janvier et al., 2002), the product was isolated in 43% yield (entry 2, Table 1). Under microwave conditions, the yield increased to 57% (entry 3, Table 1). In previous reports, we used TsOH for the aromatization process after a Diels-Alder cycloaddition to construct the isoindolin-1-one moiety (Rentería-Gómez et al., 2016a). Unfortunately, bis-heterocycle 6a was not detected when TsOH was used; only decomposition was observed by TLC.
Using catalytic Sc(OTf)3 under conventional heating, 6a was obtained in 42% yield (entry 5, Table 1). Microwave heating with Sc(OTf)3 or Yb(OTf)3 (Islas-Jácome et al., 2011, 2012) raised the yield to 69 and 62%, respectively (entries 6-7, Table 1). We therefore used the optimal conditions (entry 6: 3 mol% Sc(OTf)3, microwave heating 60–80 °C, 1.5 h) to synthesize the series of fused bis-heterocycles 6a-l (Table 2).
The scope of this cascade process was explored using alkyl and aryl aldehydes 2a-d and amide-containing isocyanides 3a-c (Table 2). The role of fluorine atoms in improving bioavailability, lipophilicity and metabolic resistance in bioactive molecules is well-documented (Purser et al., 2008). The products 6c, 6g, and 6k containing fluorine atom was synthesized. Piperidine, morpholine, and diethylamine were incorporated as substituents of isocyanides 3a-c. These fragments can act as structural bioisosteres, preferably interacting with some amino acids allowing in some cases improve biological activity (Kalinsky and Weinstein, 1954; Sander et al., 2008; Meng et al., 2011; El-Nassan, 2015; Yu et al., 2015; Sato et al., 2017).
The highest yield (72%) was obtained for product 6b, which contains 2,3-dimethoxyphenyl and piperidine as substituents at R1 and R2, respectively. Contrarily, bis-heterocycle 6i, with phenyl and diethylamine substituents, was obtained in the lowest yield (59%). In fact, among all products, the diethylamine-containing analogs 6i-l were synthesized in lower yields, which can be attributed to the lower stability of this isocyanide in acidic media. In all cases, the primary byproducts were the corresponding 5-aminooxazoles resulting from Lewis-acid-catalyzed chain-ring tautomerization of the isocyanides 3a-c (Gao et al., 2016). Consistent with reports by Zhu (Cuny et al., 2004; Wang et al., 2007), we also observed, as minor byproducts, the alcohols resulting from isocyanide addition to the aldehydes prior to oxazole formation (7) (Scheme 4). The plausible reaction mechanism for the formation of pyrrolo[3,4-b]pyridin-5-ones 6a-l is supported by computational calculations performed previously using DFT methods (Scheme 4) (Islas-Jácome et al., 2016).
Conditions were screened for the [3+2] cycloaddition using terminal alkyne 6a (Table 3). Heating at 100 °C for 12 h with 1.0 equiv. TMSN3 and 3 mol% CuI provided tris-heterocycle 9a in a modest 48% yield (entry 1, Table 2). Increasing the equivalents of the volatile TMSN3 to 1.5 or 2.0 raised the yield of 9a to 69 and 77%, respectively (entries 2-3, Table 2). Using additional CuI (5% mol) and increasing the reaction time to 18 h did not improve the yield (entry 4, Table 2). Microwave heating reduced both the reaction time and the yield to 52%, as high amounts of byproducts were detected (entry 5, Table 2).
Using the optimized conditions, a series of tris-heterocycles (9a-l) was synthesized from complex alkynes 6a-I via the [3+2] cycloaddition in good yields (70–80%, Table 4). The highest yields were obtained for the 4-fluorophenyl analogs. Alkynes 6a-l and triazole products 9a-l were fully characterized by IR, 1H and 13C NMR, and HRMS (see the Supplementary Material for further details). Several attempts to obtain adequate crystals for X-ray analysis were performed without success.
In Scheme 5, we show a plausible reaction mechanism for the alkyne-azide [3+2] cycloaddition to produce 4-substituted 1H-1,2,3-triazoles. The reaction likely proceeds through the formation of copper acetylide species 13a-l from terminal alkynes 6a-l with CuI (12) and in situ generation of HN3 (17) from the reaction of TMSN3 (15) and MeOH (14). Copper-assisted cycloaddition between 13a-l and HN3 (17) takes place to form intermediates 18a-l. Protonolysis of the C-Cu bond of 18a-l by terminal alkynes 6a-l, HI, or MeOH affords 4-substituted 1H-1,2,3-triazoles 9a-l (Jin et al., 2004).
Having the methodology for the synthesis of compounds 9a-l, we next explored the scope of use de terminal alkynes linked to bis-heterocycles using organic azides to obtain the 1,4-disubstituted 1,2,3-triazoles (19a-d) via CuAAC. Compound 6m was synthetized (60% yield) and selected as model. Phenyl azides with different stereo-electronic natures (20a-d) were prepared from aromatic amines via diazotization with sodium nitrite in water in the presence of p-TsOH followed by reaction with sodium azide at room temperature (Kutonova et al., 2013).
First, the reaction was carried out under constant stirring, at room temperature, using 6m and azide 20a obtaining an 85% yield of 1,4-disubstituted 1,2,3-triazole 19a after 5 h. When the reaction was carried out using ultrasound-assisted irradiation (USI) at room temperature, the product 19a was obtained in 1.5 h, with a yield of 83%. For this reason, we decided to use the USI protocol for the synthesis of 1,4-disubstituted 1-H-1,2,3-triazoles (19a-d). The reactions under USI resulted in reduced reaction times (30–90 min) and good yields (83–93%) in the CuAAC for the synthesis of 19a-e (Table 5).
Conclusions
We have developed a new and efficient strategy to synthesize 4-substituted and 1,4-disubstituted 1H-1,2,3-triazoles linked to pyrrolo[3,4-b]pyridin-5-ones. Molecules containing these heterocycles together are novel. The molecules synthesized contain privileged tris-heterocycles which could have applications in medicinal chemistry and coordination chemistry.
The IMCR based cascade process coupled with CuAAC strategy, as convergent and powerful tool toward the synthesis of bis and tris heterocycles is unreported.
Data Availability
All datasets generated for this study are included in the manuscript and/or the Supplementary Files.
Author Contributions
MR-G, RG-M, and DV have made a substantial, direct and intellectual contribution to the work. SP was responsible for performing the initial experiments. AI-J was responsible for designing and analyzing the results. All authors discussed the whole project, wrote the publication, and approved it for publication.
Conflict of Interest Statement
The authors declare that the research was conducted in the absence of any commercial or financial relationships that could be construed as a potential conflict of interest.
Acknowledgments
RG-M is grateful for financial support from DAIP (193/2017) and CONACYT (CB-2016-28562) projects. MR-G (707974/585367) and SP (636753/573230) thank CONACYT for scholarships. DV is thankful for a Fulbright-García Robles grant. The authors acknowledge Dr. Murali V. Unnamatla and Dr. Eduardo González-Zamora (UAM-I) for technical support with HRMS and NMR. All authors acknowledge the Laboratorio Nacional de Caracterizacioń de Propiedades Fisicoquímícas y Estructura Molecular (CONACYT-México, Project: 123732) for the instrumentation time provided.
Supplementary Material
The Supplementary Material for this article can be found online at: https://www.frontiersin.org/articles/10.3389/fchem.2019.00546/full#supplementary-material
References
Alvárez-Rodríguez, N. V., Islas-Jácome, A., Rentería-Gómez, A., Cárdenas-Galindo, L. E., Unnamatla, M. V. B., and Gámez-Montaño, R. (2018). Synthesis of 1′-tetrazolylmethyl-spiro[pyrrolidine-3,3′-oxindoles] via two coupled one-pot processes Ugi-azide/Pictet–Spengler and oxidative spiro-rearrangement. New J. Chem. 42, 1600–1603. doi: 10.1039/C7NJ03829A
Atobe, M., Maekawara, N., Ishiguro, N., Sogame, S., Suenaga, Y., Kawanishi, M., et al. (2013). A series of thiazole derivatives bearing thiazolidin-4-one as non-competitive ADAMTS-5 (aggrecanase-2) inhibitors. Bioorg. Med. Chem. Lett. 23, 2106–2110. doi: 10.1016/j.bmcl.2013.01.121
Bakulev, V. A., and Beryozkina, T. A. (2016). NH-1,2,3-triazoles: synthesis and reactions with electrophilic agents. Chem. Heterocycl. Comp. 52, 4–6. doi: 10.1007/s10593-016-1821-y
Balle, T., Perregaard, J., Ramirez, M. T., Larsen, A. K., Søby, K. K., Liljefors, T., et al. (2003). Synthesis and structure–affinity relationship investigations of 5-heteroaryl-substituted analogues of the antipsychotic sertindole. A new class of highly selective α1 edrenoceptor antagonists. J. Med. Chem. 46, 265–283. doi: 10.1021/jm020938y
Blass, B. E., Coburn, K., Lee, W., Fairweather, N., Fluxe, A., Wu, S., et al. (2006). Synthesis and evaluation of (2-phenethyl-2H-1,2,3-triazol-4-yl)(phenyl)methanones as Kv1.5 channel blockers for the treatment of atrial fibrillation. Bioorg. Med. Chem. Lett. 16, 4629–4632. doi: 10.1016/j.bmcl.2006.06.001
Bock, V. D., Hiemstra, H., and van Maarseveen, J. H. (2006). CuI-catalyzed alkyne–azide “Click” cycloadditions from a mechanistic and synthetic perspective. Eur. J. Org. Chem. 51–68. doi: 10.1002/ejoc.200500483
Bonandi, E., Christodoulou, M. S., Fumagalli, G., Perdicchia, D., Rastelli, G., and Passarella, D. (2017). The 1,2,3-triazole ring as a bioisostere in medicinal chemistry. Drug Discov Today. 22, 1572–1581. doi: 10.1016/j.drudis.2017.05.014
Borkin, D., Pollock, J., Kempinska, K., Purohit, T., Li, X., Wen, B., et al. (2016). Property focused structure-based optimization of small molecule inhibitors of the protein–protein interaction between menin and mixed lineage leukemia (MLL). J. Med. Chem. 59, 892–913. doi: 10.1021/acs.jmedchem.5b01305
Burling, S., Field, L. D., Messerle, B. A., and Rumble, S. L. (2007). Late transition metal catalyzed intramolecular hydroamination: The effect of ligand and substrate structure. Organometallics 26, 4335–4343. doi: 10.1021/om700428q
Cárdenas-Galindo, L. E., Islas-Jácome, A., Alvárez-Rodríguez, N. V., El Kaim, L., and Gámez-Montaño, R. (2014). Synthesis of 2-tetrazolylmethyl-2,3,4,9-tetrahydro-1H-β-carbolines by a one-pot Ugi-azide/Pictet–Spengler process. Synthesis 46, 49–56. doi: 10.1055/s-0033-1340051
Chang, H.-F., Chapdelaine, M., Dembofsky, B. T., Herzog, K. J., Horschler, C., and Schmiesing, R. J. (2008). Compounds and Uses Thereof−848. Patent Cooperation Treaty (PCT). WO 2008/155572 A1. Geneva: World Intellectual Property Organization.
Cortes-García, C. J., Islas-Jácome, A., Rentería-Gómez, A., and Gámez-Montaño, R. (2016). Synthesis of 1,5-disubstituted tetrazoles containing a fragment of the anticancer drug imatinib via a microwave-assisted Ugi-azide reaction. Monatsh. Chem. 147, 1277–1290. doi: 10.1007/s00706-016-1686-x
Cuny, G., Gámez-Montaño, R., and Zhu, J. (2004). Truncated diastereoselective Passerini reaction, a rapid construction of polysubstituted oxazole and peptides having an α-hydroxy-β-amino acid component. Tetrahedron 60, 4879–4885. doi: 10.1016/j.tet.2004.03.084
Dener, J. M., O'Bryan, C., Yee, R., Shelton, E. J., Sperandio, D., Mahajan, T., et al. (2006). Development of a scalable synthesis of a nonbasic inhibitor of the serine protease tryptase. Tetrahedron Lett. 47, 4591–4595. doi: 10.1016/j.tetlet.2006.04.149
Devasthale, P., Wang, Y., Wang, W., Fevig, J., Feng, J., Wang, A., et al. (2013). Optimization of activity, selectivity, and liability profiles in 5-oxopyrrolopyridine DPP4 inhibitors leading to clinical candidate (Sa)-2-(3-(aminomethyl)-4-(2,4-dichlorophenyl)-2-methyl-5-oxo-5H-pyrrolo[3,4-b]pyridin-6(7H)-yl)-N,N-dimethylacetamide (BMS-767778). J. Med. Chem. 56, 7343–7357. doi: 10.1021/jm4008906
Dixit, S. K., Mishra, N., Sharma, M., Singh, S., Agarwal, A., Awasthi, S. K., et al. (2012). Synthesis and in vitro antiplasmodial activities of fluoroquinolone analogs. Eur. J. Med. Chem. 51, 52–59. doi: 10.1016/j.ejmech.2012.02.006
Dolle, R. E., Le Bourdonnec, B., Goodman, A. J., Morales, G. A., Thomas, C. J., and Zhang, W. (2008). Comprehensive survey of chemical libraries for drug discovery and chemical biology: 2007. J. Comb. Chem. 10, 753–802. doi: 10.1021/cc800119z
Duan, H., Yan, W., Sengupta, S., and Shi, X. (2009). Highly efficient synthesis of vinyl substituted triazoles by Au(I) catalyzed alkyne activation. Bioorg. Med. Chem. Lett. 19, 3899–3902. doi: 10.1016/j.bmcl.2009.03.096
El-Nassan, H. B. (2015). Synthesis and antitumor activity of tetrahydrocarbazole hybridized with dithioate derivatives. J. Enzyme Inhib Med Chem. 30, 308–315. doi: 10.3109/14756366.2014.922554
Gao, S., Xu, X., Yuan, Z., Zhou, H., Yao, H., and Lin, A. (2016). 1,6-Addition arylation of para-quinone methides: an approach to unsymmetrical triarylmethanes. Eur. J. Org. Chem. 2016, 3006–3012. doi: 10.1002/ejoc.201600385
Gordillo-Cruz, R. E., Rentería-Gómez, A., Islas-Jácome, A., Cortes-García, C. J., Díaz-Cervantes, E., Robles, J., et al. (2013). Synthesis of 3-tetrazolylmethyl-azepino[4,5-b]indol-4-ones in two reaction steps: (Ugi-azide/N-acylation/SN2)/free-radical cyclization and docking studies to a 5-Ht6 model. Org. Biomol. Chem. 11, 6470–6476. doi: 10.1039/C3OB41349G
Hartzel, L. M., and Benson, F. R. (1954). Synthesis of 4-alkyl-v-triazoles from acetylenic compounds and hydrogen azide. J. Am. Chem. Soc. 76, 667–670. doi: 10.1021/ja01632a010
Hédou, D., Deau, E., Dubouilh-Benard, C., Sanselme, M., Martinet, A., Chosson, E., et al. (2013). Microwave-assisted [3+2] cycloaddition and Suzuki–Miyaura cross-coupling for a concise access to polyaromatic scaffolds. Eur. J. Org. Chem. 7533–7545. doi: 10.1002/ejoc.201301014
Hein, J. E., and Fokin, V. V. (2010). Copper-catalyzed azide–alkyne cycloaddition (CuAAC) and beyond: new reactivity of copper(I) acetylides. Chem. Soc. Rev., 39, 1302–1315. doi: 10.1039/b904091a
Hsu, K. L., Tsuboi, K., Whitby, L. R., Aspeers, A. E., Pugh, H., Inloes, J., et al. (2013). Development and optimization of piperidyl-1,2,3-triazole ureas as selective chemical probes of endocannabinoid biosynthesis. J. Med. Chem. 56, 8257–8269. doi: 10.1021/jm400898x
Huang, Q., Zheng, M., Yang, S., Kuang, C., Yu, C., and Yang, Q. (2011). Structure–activity relationship and enzyme kinetic studies on 4-aryl-1H-1,2,3-triazoles as indoleamine 2,3-dioxygenase (IDO) inhibitors. Eur. J. Med. Chem. 46, 5680–5687. doi: 10.1016/j.ejmech.2011.08.044
Ibarra, I. A., Islas-Jácome, A., and González-Zamora, E. (2018). Synthesis of polyheterocycles via multicomponent reactions. Org. Biomol. Chem. 16, 1402–1418. doi: 10.1039/C7OB02305G
Islas-Jácome, A., Cárdenas-Galindo, L. E., Jerezano, A. V., Tamariz, J., González-Zamora, E., and Gámez-Montaño, R. (2012). Synthesis of nuevamine aza-analogues by a sequence: I-MCR–aza-Diels–Alder–Pictet–Spengler. Synlett 23, 2951–2956. doi: 10.1055/s-0032-1317622
Islas-Jácome, A., González-Zamora, E., and Gámez-Montaño, R. (2011). A short microwave-assisted synthesis of tetrahydroisoquinolin-pyrrolopyridinones by a triple process: Ugi-3CR–aza Diels–Alder/S-oxidation/Pummerer. Tetrahedron Lett. 52, 5245–5248. doi: 10.1016/j.tetlet.2011.07.134
Islas-Jácome, A., Rentería-Gómez, A., Rentería-Gómez, M. A., González-Zamora, E., Jiménez-Halla, J. O. C., and Gámez-Montaño, R. (2016). Selective reaction route in the construction of the pyrrolo[3,4-b]pyridin-5-one core from a variety of 5-aminooxazoles and maleic anhydride. A DFT study. Tetrahedron Lett. 57, 3496–3500. doi: 10.1016/j.tetlet.2016.06.099
Janvier, P., Sun, X., Bienaym,é, H., and Zhu, J. (2002). Ammonium chloride-promoted four-component Synthesis of Pyrrolo[3,4-b]pyridin-5-one. J. Am. Chem. Soc. 124, 2560–2567. doi: 10.1021/ja017563a
Jin, T., Kamijo, S., and Yamamoto, Y. (2004). Copper-catalyzed synthesis of N-Unsubstituted 1,2,3-triazoles from nonactivated terminal alkynes. Eur. J. Org. Chem. 3789–3791. doi: 10.1002/ejoc.200400442
Kalinsky, J. L., and Weinstein, A. (1954). The synthesis of 2-ethylhexanol-1-C14 and esters. J. Am. Chem. Soc. 76, 3730–3730. doi: 10.1021/ja01643a042
Kallander, L. S., Lu, Q., Chen, W., Tomaszek, T., Yang, G., Tew, D., et al. (2005). 4-Aryl-1,2,3-triazole: a novel template for a reversible methionine aminopeptidase 2 inhibitor, optimized to inhibit angiogenesis in vivo. J. Med. Chem. 48, 5644–5647. doi: 10.1021/jm050408c
Koszytkowska-Stawińska, M., Mironiuk-Puchalska, E., and Rowicki, T. (2012). Synthesis of 1,2,3-triazolo-nucleosides via the post-triazole N-alkylation. Tetrahedron 68, 214–225. doi: 10.1016/j.tet.2011.10.067
Kutonova, K. V., Trusova, M. E., Postnikov, P. S., Filimonov, V. D., and Parello, J. (2013). A simple and effective synthesis of Aryl Azides via arenediazonium tosylates Synthesis 45, 2706–2710. doi: 10.1055/s-0033-1339648
Lauria, A., Delisi, R., Mingoia, F., Terenzi, A., Martorana, A., Barone, G., et al. (2014). 1,2,3-Triazole in heterocyclic compounds, endowed with biological activity, through 1,3-dipolar cycloadditions. Eur. J. Org. Chem. 3289–3306. doi: 10.1002/ejoc.201301695
Lindsley, C. W., Conn, P. J., Wood, M. R., Hopkins, C. R., Melancon, B. J., Poslusney, M. S., et al. (2013). Substituted 2-(4-heterocyclylbenzyl)Isoindolin-1-One Analogs as Positive Allosteric Modulators of Muscarinic Acethylcholine Receptor M1. (PCT). WO 2013/063549 A1. Geneva: World Intellectual Property Organization.
Mallemula, V. R., Sanghai, N. N., Himabindu, V., and Chakravarthy, A. K. (2015). Synthesis and characterization of antibacterial 2-(pyridin-3-yl)-1H-benzo[d]imidazoles and 2-(pyridin-3-yl)-3H-imidazo[4,5-b]pyridine derivatives. Res. Chem. Intermed. 41, 2125–2138. doi: 10.1007/s11164-013-1335-5
Maračić, S., Kraljević, T. G., Paljetak, H. C., Perić, M., Matijašić, M., Verbanac, D., et al. (2015). 1,2,3-Triazole pharmacophore-based benzofused nitrogen/sulfur heterocycles with potential anti-Moraxella catarrhalis activity. Bioorg. Med. Chem. 23, 7448–7463. doi: 10.1016/j.bmc.2015.10.042
Meng, Z., Kulkarni, B. A., Kerekes, A. D., Mandal, A. K., Esposite, S. J., Belanger, D. B., et al. (2011). Bioisosteric approach to the discovery of imidazo[1,2-a]pyrazines as potent Aurora kinase inhibitors. Bioorg. Med. Chem. Lett. 21, 592–598 doi: 10.1016/j.bmcl.2010.10.008
Oh, S., Shin, W., Ham, J., and Lee, S. (2010). Acid-catalyzed synthesis of 10-substituted triazolyl artemisinins and their growth inhibitory activity against various cancer cells. Bioorg. Med. Chem. Lett. 20, 4112–4115. doi: 10.1016/j.bmcl.2010.05.074
Pajouhesh, H., Hollan, R., Zhou, Y., Zhu, Y, and Grimwood, M. E. (2012). Bisaylsufone and Dialkylarylsulfone Compounds as Calcium Channel Blockers. PatentCooperation Treaty (PCT). WO 2012/061926 A1.
Prabhath, M. R., Romanova, J., Curry, R. J., Silva, S. R., and Jarowski, P. D. (2015). The role of substituent effects in tuning metallophilic interactions and emission energy of bis-4-(2-pyridyl)-1,2,3-triazolatoplatinum(II) complexes. Angew. Chem. Int. Ed. 54, 7949–7953. doi: 10.1002/anie.201502390
Purser, S., Moore, P. R., Swallow, S., and Gouverneur, V. (2008). Fluorine in medicinal chemistry. Chem. Soc. Rev. 37, 320–330. doi: 10.1039/B610213C
Rentería-Gómez, A., Islas-Jácome, A., Cruz-Jiménez, A. E., Manzano-Velázquez, J. C., Rojas-Lima, S., Jiménez-Halla, J. O. C., et al. (2016a). Synthesis of 2-tetrazolylmethyl-isoindolin-1-ones via a one-pot Ugi-azide/(N-acylation/exo-Diels–Alder)/dehydration process. ACS Omega 1, 943–951. doi: 10.1021/acsomega.6b00281
Rentería-Gómez, A., Islas-Jácome, A., Díaz-Cervantes, E., Villaseñor-Granados, T., Robles, J., and Gámez-Montaño, R. (2016b). Synthesis of azepino[4,5-b]indol-4-ones via MCR/free radical cyclization and in vitro–in silico studies as 5-Ht6R ligands. Bioorg. Med. Chem. 26, 2333–2338. doi: 10.1016/j.bmcl.2016.03.036
Röhri, U. F., Majjigapu, S. R., Grosdidier, A., Bron, S., Stroobant, V., Pilotte, L., et al. (2012). Rational design of 4-aryl-1,2,3-triazoles for indoleamine 2,3-dioxygenase 1 inhibition. J. Med. Chem. 55, 5270–5290. doi: 10.1021/jm300260v
Rostovtsev, V. V., Green, L. G., Fokin, V. V., and Sharpless, K. B. (2002). A stepwise Huisgen cycloaddition process: Copper(I)-catalyzed regioselective “ligation” of azides and terminal alkynes. Angew. Chem. Int. Ed. 41, 2596–2599. doi: 10.1002/1521-3773(20020715)41:14<2596::AID-ANIE2596>3.0.CO;2-4
Sander, K., Kottke, T., and Stark, H. (2008). Histamine H3 receptor antagonists go to clinics. Biol. Pharm. Bull. 31, 2163–2181. doi: 10.1248/bpb.31.2163
Sato, T., Hashimoto, N., and Honna, T. (2017). J. Chem. Inf. Model. 57, 2938–2947. doi: 10.1021/acs.jcim.7b00092
Shchepin, R., Navarathna, D. H., Dumitru, R., Lippold, S., Nickerson, K. W., and Dussaut, P. H. (2008). Influence of heterocyclic and oxime-containing farnesol analogs on quorum sensing and pathogenicity in Candida albicans. Bioorg. Med. Chem. 16, 1842–1848. doi: 10.1016/j.bmc.2007.11.011
Sinn, S., Schulze, B., Friebe, C., Brown, D. G., Jag¨er, M., Kübel, J., et al. (2014). A heteroleptic bis(tridentate) ruthenium(II) platform featuring an anionic 1,2,3-triazolate-based ligand for application in the dye-sensitized solar cell. Inorg. Chem. 53, 1637–1645. doi: 10.1021/ic402701v
Stibrany, R. T., Schulz, D. N., Kacker, S., Patil, A. O., Baugh, L. S., Rucker, S. P., et al. (2003). Polymerization and copolymerization of olefins and acrylates by Bis(benzimidazole) copper catalysts. Macromolecules 36, 8584–8586. doi: 10.1021/ma034548c
Sun, X., Janvier, P., Zhao, G., Bienaym,é, H., and Zhu, J. (2001). A novel multicomponent synthesis of polysubstituted 5-aminooxazole and its new scaffold-generating reaction to pyrrolo[3,4-b]pyridine. Org. Lett. 3, 877–880. doi: 10.1021/ol007055q
Tahara, S., Shibahara, F., Maruyama, T., and Murai, T. (2009). Iodine-mediated cyclization of N-thioacyl-1-(2-pyridyl)-1,2-aminoalcohols and their subsequent condensation leading to the formation of novel bis(1-imidazo[1,5-a]pyridyl)arylmethanes. Chem. Commun. 7009–7011. doi: 10.1039/B910172A
Tornøe, C. W., Christensen, C., and Meldal, M. (2002). Peptidotriazoles on solid phase: [1,2,3]-Triazoles by regiospecific copper(I)-catalyzed 1,3-dipolar cycloadditions of terminal alkynes to azides. J Org. Chem. 67, 3057–3064.
Trang, T. T. T., Peshkov, A. A., Jacobs, J., Meervelt, L. V., Peshkov, V. A., and Van der Eycken, E. V. (2015). Post-Ugi carbocyclization/fragmentation sequence for the synthesis of 6,7-dihydro-5H-pyrrolo[3,4-b]pyridin-5-ones. Tetrahedron Lett. 56, 2882–2886. doi: 10.1016/j.tetlet.2015.03.092
Tron, G. C., Pirali, T., Billington, R. A., Canonico, P. L., Sorba, G., and Genazzani, A. A. (2008). Click chemistry reactions in medicinal chemistry: applications of the 1,3-dipolar cycloaddition between azides and alkynes. Med. Res. Rev. 28, 278–308. doi: 10.1002/med.20107
Unnamatla, M. V., Islas-Jácome, A., Quezada-Soto, A., Ramírez-Lopez, S. C., Flores-Alamo, M., and Gámez-Montaño, R. (2016). Multicomponent one-pot synthesis of 3-tetrazolyl and 3-imidazo[1,2-a]pyridin tetrazolo[1,5-a]quinolines. J. Org. Chem. 81, 10576–10583. doi: 10.1021/acs.joc.6b01576
Unverferth, K., Arnold, T., Lankau, H., Rostock, A., Tober, C., Dost, R., et al. (2002). 6,7-Dihydro-Pirrolo[3,4-b]Pyridin-5-One With an Anticonvulsive Action and Methods for the Same. WO 02/18381 A1. Geneva: World Intellectual Property Organization.
Wager, T. T., Galatsis, P., Chandrasekaran, R. Y., Butler, T. W., Li, J., Zhang, L., et al. (2017). Identification and profiling of a selective and brain penetrant radioligand for in vivo target occupancy measurement of casein kinase 1 (CK1) inhibitors. ACS Chem. Neurosci. 8, 1995–2004. doi: 10.1021/acschemneuro.7b00155
Wang, S., Wang, M.-X., Wang, D.-X., and Zhu, J. (2007). Asymmetric Lewis acid catalyzed addition of isocyanides to aldehydes – synthesis of 5-Amino-2-(1-hydroxyalkyl)oxazoles. Eur. J. Org. Chem. 2007, 4076–4080. doi: 10.1002/ejoc.200700340
Yan, W., Wang, Q., Chen, Y., Petersen, J. L., and Shi, X. (2010). Iron-catalyzed C–O bond activation for the synthesis of propargyl-1,2,3-triazoles and 1,1-bis-triazoles. Org. Lett. 12, 3308–3311. doi: 10.1021/ol101082v
Yu, B., Yu, Z., Qi, P., Yu, D., and Liu, H. (2015). Discovery of orally active anticancer candidate CFI-400945 derived from biologically promising spirooxindoles: Success and challenges. Eur. J. Med. Chem. 95, 35–40. doi: 10.1016/j.ejmech.2015.03.020
Keywords: nitrogen tris-heterocycles, cascade IMCR process, CuAAC, Ugi-3CR, aza Diels-Alder
Citation: Rentería-Gómez MA, Islas-Jácome A, Pharande SG, Vosburg DA and Gámez-Montaño R (2019) Synthesis of Tris-Heterocycles via a Cascade IMCR/Aza Diels-Alder + CuAAC Strategy. Front. Chem. 7:546. doi: 10.3389/fchem.2019.00546
Received: 08 April 2019; Accepted: 18 July 2019;
Published: 06 August 2019.
Edited by:
Alexander Dömling, University of Groningen, NetherlandsReviewed by:
Fabio De Moliner, University of Edinburgh, United KingdomValentine Nenajdenko, Lomonosov Moscow State University, Russia
Copyright © 2019 Rentería-Gómez, Islas-Jácome, Pharande, Vosburg and Gámez-Montaño. This is an open-access article distributed under the terms of the Creative Commons Attribution License (CC BY). The use, distribution or reproduction in other forums is permitted, provided the original author(s) and the copyright owner(s) are credited and that the original publication in this journal is cited, in accordance with accepted academic practice. No use, distribution or reproduction is permitted which does not comply with these terms.
*Correspondence: David A. Vosburg, dm9zYnVyZyYjeDAwMDQwO2htYy5lZHU=; Rocío Gámez-Montaño, cm9jaW9nbSYjeDAwMDQwO3VndG8ubXg=